- 1Department of Pathology and Laboratory Medicine, Children’s Hospital Los Angeles, Los Angeles, CA, United States
- 2The Saban Research Institute, Children’s Hospital Los Angeles, Los Angeles, CA, United States
- 3Keck School of Medicine, University of Southern California, Los Angeles, CA, United States
The mitochondrion is a multifunctional organelle that modulates multiple systems critical for homeostasis during pathophysiological stress. Variation in mitochondrial DNA (mtDNA) copy number (mtDNAcn), a key mitochondrial change associated with chronic stress, is an emerging biomarker for disease pathology and progression. mtDNAcn can be quantified from whole blood samples using qPCR to determine the ratio of mtDNA to nuclear DNA. However, the collection of blood samples in pediatric populations, particularly in infants and young children, can be technically challenging, yield much smaller volume samples, and can be distressing for the patients and their caregivers. Therefore, we have validated a mtDNAcn assay utilizing DNA from simple buccal swabs (Isohelix SK-2S) and report here it's performance in specimens from infants (age = <12 months). Utilizing qPCR to amplify ∼200 bp regions from two mitochondrial (ND1, ND6) and two nuclear (BECN1, NEB) genes, we demonstrated absolute (100%) concordance with results from low-pass whole genome sequencing (lpWGS). We believe that this method overcomes key obstacles to measuring mtDNAcn in pediatric populations and creates the possibility for development of clinical assays to measure mitochondrial change during pathophysiological stress.
1 Introduction
The mitochondrion is an essential organelle producing cellular energy through oxidative metabolism. In addition to being the “powerhouse” of the cell, mitochondria are increasingly recognized for their roles in a broad range of cellular processes. Damage-associated molecular patterns (DAMPs) are pathophysiological changes that occur on the cellular level that can be mediated by the release of mitochondrial DNA and mitochondrial double stranded RNA into the cytoplasm during mitophagy (1, 2). This may lead to increased oxidative stress and changes in nuclear gene expression, triggering inflammation-related signaling pathways and systemic inflammatory responses (3–5).
One of the most well-studied associations between mtDNAcn and pathology is in cancer (6). Increased mtDNAcn has been observed in various cancer types, including breast, lung, and colorectal cancer. Known as mitochondrial biogenesis, this is thought to support the high energy demands of rapidly dividing cancer cells and may also contribute to chemoresistance, making it a potential therapeutic target (7–9). Diseases may also be characterized by a reduced mtDNAcn. Mitochondrial diseases often involve mutations in nuclear genes or mtDNA that disrupt a variety of cellular processes including but not limited to energy production, causing a wide range of symptoms (1, 10, 11). Other diseases associated with changes in mtDNAcn include neurodegenerative and cardiovascular diseases, and metabolic disorders including type 2 diabetes and obesity (12–14).
Chronic stress, a persistent state of emotional and psychological strain, has been closely linked with changes in mtDNAcn and altered mitochondrial functioning in pre-clinical models of early adversity and in human adults (15–17). Disordered mitochondrial function may be an important contributor to the molecular mechanisms of human disease, including several mental and physical health conditions. In chronic stress, dysregulation of the hypothalamic-pituitary-adrenal (HPA) axis and increased cortisol can affect mitochondrial function and mtDNAcn (18, 19). These changes highlight the need for a reliable means of assessing mtDNAcn.
A variety of methods have been described to quantify mtDNAcn, beginning with use of a combination of restriction enzymes and southern blotting to evaluate the intensity of nuclear to mitochondrial DNA probes (20). Molecular methods including PCR, qPCR, digital PCR and genomic methods including whole exome sequencing (WES) and whole genome sequencing (WGS) have all been used to establish a relative mtDNAcn value by comparing nuclear to mitochondrial intensity, CT values, copies, number of reads, respectively (3, 21, 22). PCR based approaches are broadly cost effective for implementation of routine clinical testing. While WES/WGS are becoming more cost-effective and are used in some specialized settings, they remain impractical for routine clinical testing of mtDNAcn. However, in this methods paper we show that low pass whole genome sequencing (lpWGS) can serve as a complementary method to qPCR, establishing the robustness of the developed qPCR assay. lpWGS, also known as shallow whole genome sequencing, involves sequencing the entire genome at low coverage depth. In our study, lpWGS was performed with an average coverage of 1X. In this case where the nuclear genome is covered at 1X, the average coverage depth for mtDNA was observed to be between 100–1000X, depending on the exact number of mtDNA copies per cell and the sample. While we did not assess mitochondrial mutations as part of this assay, utilizing lpWGS has the added advantage of detecting potentially low-level heteroplasmic variants, improving the accuracy of mtDNA variant calling and haplogroups in a cost-efficient and comprehensive manner. Therefore, lpWGS is sufficient for quantifying mtDNAcn and is significantly less expensive than standard WES/WGS in settings with the capacity for clinical genomic testing (23).
To obtain sufficient volume and quality of DNA for mtDNAcn testing, conventional methods utilize venipuncture, from which up to 10 mls of whole blood is collected (24). However, this exceeds the recommended volume limits for infants and children (25), and venipuncture can be technically challenging and can generate anxiety and discomfort in pediatric populations (26–28). Therefore, we utilized buccal swabs (Isohelix SK-2S buccal swab kit and BuccalFix buffer BFX25 to stabilize DNA and RNA) for specimen collection as it was minimally invasive, simple to administer and cost effective. Using DNA extracted from these swabs, we developed a mtDNAcn qPCR assay and evaluated its performance in comparison to a commercially available mtDNAcn qPCR kit (NovaQUANT Human Mitochondrial to Nuclear DNA ratio kit, Millipore Sigma, USA) and lpWGS.
2 Materials and methods
2.1 Participants and study design
Buccal swab samples collected through Children's Hospital Los Angeles as part of the California Initiative to Advance Precision Medicine (CIAPM)—Adverse Childhood Experiences (ACEs) program (Scalable Measurement and Clinical Deployment of Mitochondrial Biomarkers of Toxic Stress—IRB# CHLA-21-00174) and the Your Baby: Healthy Development and Resiliency (IRB# CHLA-18-00547) studies were used for assay development. The ACEs study samples used in this study were from 164 mothers and their infants recruited to participate at the 6-month timepoint of this ongoing study that also includes a 12-month infant visit. The Your Baby study samples used in this study were from 19 mothers and 21 infants at the 6-month timepoint. A total of six samples collected as part of the CIAPM study were used to validate the commercial (samples a1–a3) and our own (samples b1–b3) qPCR based mtDNAcn assay. The commercial assay and lpWGS were performed on 40 samples (Your Baby study, 19 mothers and their 21 infants) to assess the concordance of these two techniques. However, further use of the commercial qPCR mtDNAcn assay was precluded by supply chain issues which limited the availability of kits (at the time of manuscript submission, the NovaQUANT assay is currently described as “limited availability” from Millipore Sigma). Therefore, we established the concordance of our assay (developed with CIAPM samples b1–b3) using three samples from the Your Baby study that were previously used for the commercial assay and lpWGS. We then performed our mtDNAcn qPCR assay on 164 mother and 164 infant ACEs study samples and, finally assessed concordance using lpWGS on a subset of 36 samples (18 mothers and their 18 infants) from the ACEs study. The workflows and study samples are summarized in Figure 1.
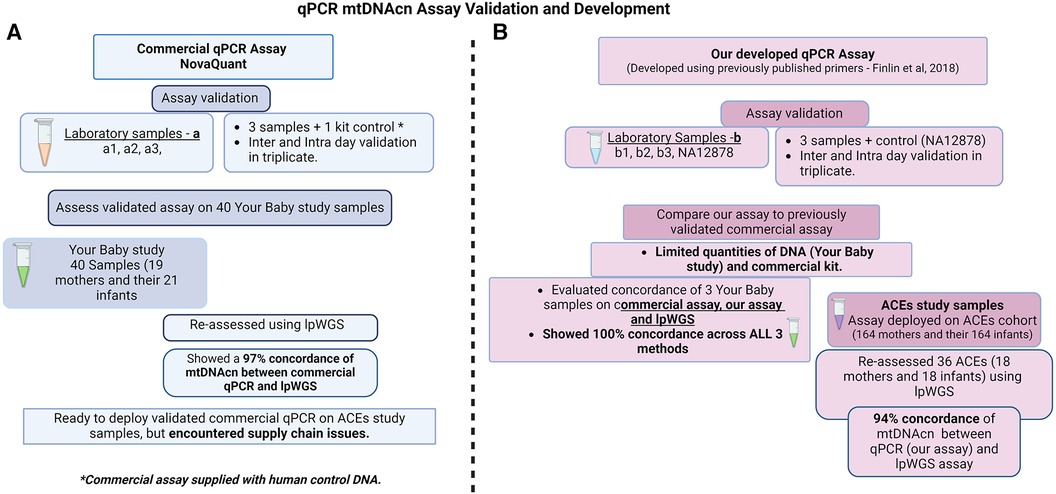
Figure 1. Study design for qPCR mtDNA assay validation and development. (A) Commercial, NovaQUANT (Millipore Sigma, USA) mtDNAcn qPCR assay validation and study samples utilized; (B) our mtDNAcn qPCR assay development, validation and study samples utilized.
2.2 Specimen collection, DNA isolation and extraction
The IsoHelix SK-2S kit (IsoHelix—Division of Cell Projects LTD) in combination with the BFX-25 stabilizing buffer can be used for the isolation of both DNA and RNA from a single buccal swab. Caregivers were instructed not to give infants food or milk for 60 min prior to specimen collection. Mothers utilized a gentle “bear hug technique” to hold the infant while a member of the research staff swabbed the buccal mucosa of each cheek for 30 s (total of 60 s, timed), and then placed the swab into the collection tube. Maternal buccal swabs were self-collected during the same visit following an identical procedure. While some infants tolerated a shorter period of time (30–45 s), we found that moderate pressure applied during swabbing for any time between 30 and 60 s is the most important element for sufficient sample collection. No mother-infant pairs were excluded from the study due to inadequate swab times. Collection tubes received a 500 μl aliquot of BuccalFix buffer (BFX-25) and were inverted 10 times before being barcoded and stored at −80°C in the CHLA Pediatric Research Biorepository. DNA and RNA were extracted using the Maxwell RSC Blood DNA kit &RSC miRNA from Tissue and Plasma Kit (Promega) and stored at 4°C and −80°C, respectively, until required. DNA/RNA quality (nanodrop) and quantity (Qubit) was assessed prior to use.
Samples collected for the ACEs and Your Baby study were assigned study specific identifiers followed by a 5-digit number. Once received at the laboratory, all samples were given a separate laboratory 10-digit identifier. Thus, all samples had two specific identifiers to maintain sample integrity and ensure de-identification and study participant privacy. All data of the study subjects was captured and stored in a HIPAA-compliant REDCap database. Laboratory staff conducting assay validation were not able to access clinical or sociodemographic information at any time.
2.3 mtDNAcn assays and lpWGS
NovaQUANT (Millipore Sigma, USA) is a commercial qPCR assay that assesses relative mtDNAcn by comparing the ratio of mitochondrial to nuclear DNA using the Ct (ΔCt) levels of two mitochondrial genes (ND1 and ND6) and two nuclear genes (BECN1 and NEB). Real-time PCR of 4 targets (2 mitochondrial, 2 nuclear) was performed following the manufacturer's protocol. All samples were run in triplicate (DNA—2 ng/replicate), including nuclease-free water as a no template control (NTC). For analysis, we used the manufacturer's recommended method in which relative mtDNAcn was calculated by assessing the ratio of mtDNA/nDNA for the MT-ND1 and MT-ND4, by the geometric mean of BECN1 and NEB1.
Due to supply chain issues that limited availability of the commercial assay, we designed a mtDNAcn qPCR assay utilizing prior published primers and mitochondrial gene target normalization against nuclear genes (29) (Table 1). The Ct (ΔCt) levels of two mitochondrial genes (ND1 and ND6) and two nuclear genes (BECN1 and NEB) were assessed for each DNA sample. A reference DNA-sample (NA12878) which has been broadly used in validating many genomic assays was included in all runs. Real-time PCR of the four target genes was performed on DNA obtained from the buccal swab specimens using the following cycling parameters: 95 °C for 10 min; 40 cycles of 95 °C for 15 s, 60°C for 60 s on the StepOnePlus™ Real-Time PCR System (Applied Biosystems, USA) and using RT² SYBR Green ROX FAST Master mix (Qiagen, USA) consisting of HotStart DNA taq polymerase, nucleotides, ROX as the reference dye, and SYBR Green dye. All samples were run in triplicate (DNA—2 ng/replicate), including nuclease-free water as a no template control (NTC). Once normalized, the mitochondrial gene target Ct value was analyzed using the 2-Delta-Delta method (30) to convert the Ct value to a linear form for analysis of gene expression and calculate the relative change in mitochondrial copy number. Briefly, Cts from the ND1 gene are subtracted from that of the BECN1 gene to obtain ΔCt1 (ΔCt1 = CtNuc1-CtMito1), while ND6 Ct is subtracted from NEB Ct to obtain ΔCt2 (ΔCt2 = CtNuc2-CtMito2), then copy numbers are calculated based on the ΔCt of the matched mitochondrial to nuclear DNA Cts (N = 2ΔCt). The average of the two copy number results provides the final relative mtDNAcn per sample.
We performed lpWGS to evaluate the performance of both the commercial and our own mtDNAcn assay (Figure 1). DNA (5 ng) obtained from a subset of the same buccal swab specimens was used to prepare NGS libraries using xGen™ cfDNA & FFPE DNA Library Prep (Integrated DNA Technologies Coralville, IA). NGS libraries were barcoded, pooled and sequenced on Illumina NextSeq 500 at 2 × 100 bp to an average read-depth ranging from 0.5–1X. Relative mtDNAcn was then calculated using the formula mtDNAcn = (Average mitochondrial genome coverage/Average autosomal chromosome coverage)*2, as previously described (31).
We assessed the performance of our mtDNAcn assay by three-way comparison to the commercial assay and lpWGS results obtained from three samples using DNA (2 ng/replicate) obtained from a subset of the same buccal swab specimens from the Your Baby study. We were only able to perform this 3-way comparison on this subset of samples due to (1) limited amounts of DNA remaining and (2) limited quantities of the commercial assay.
2.4 Data analysis
Correlation analysis and graphing were done using GraphPad Prism (version 9.5.1). In our study, we utilized the Pearson correlation coefficient to assess the relationship between mitochondrial DNA copy number (mtDNAcn) measured using different methods. Pearson correlation in this context enabled us to quantify the strength and direction of a linear relationship between two continuous variables. R (version 4.3.1) running in RStudio was utilized to graph Figures 3B, 6. Data are presented as means with error bars depicting standard deviation (SD) unless otherwise specified.
3 Results
3.1 Commercial assay validation and assessment
We initially assessed buccal swab specimens from the Your Baby study comprised of 19 mothers and 21 infants. As shown in Figure 2, the IsoHelix SK-2S kit in combination with the BFX-25 stabilizing buffer produced high-quality DNA and RNA as assessed by 260/280 and 260/230 ratios. The collection method yielded a range of 20–2,000 ng DNA across infant samples, and 200–4,000 ng DNA across maternal samples (Figure 2C). We were able to elute an average of 600 ng of DNA and RNA in a 25 μl volume, providing sufficient nucleic acid concentrations for multiple assays on each sample (new Figures 2C,D). Prior to assessing mtDNAcn in the Your Baby study samples, we conducted an inter and intra-day assay validation of the commercial NovaQUANT mtDNA qPCR assay utilizing DNA obtained from three specimens (a1, a2 and a3). Inter-day variability was determined by running a1, a2 and a3 in triplicate on consecutive days; intra-day variability was determined by running the same samples in triplicate a second time on the same plate. (Supplementary Figures S1–S3).
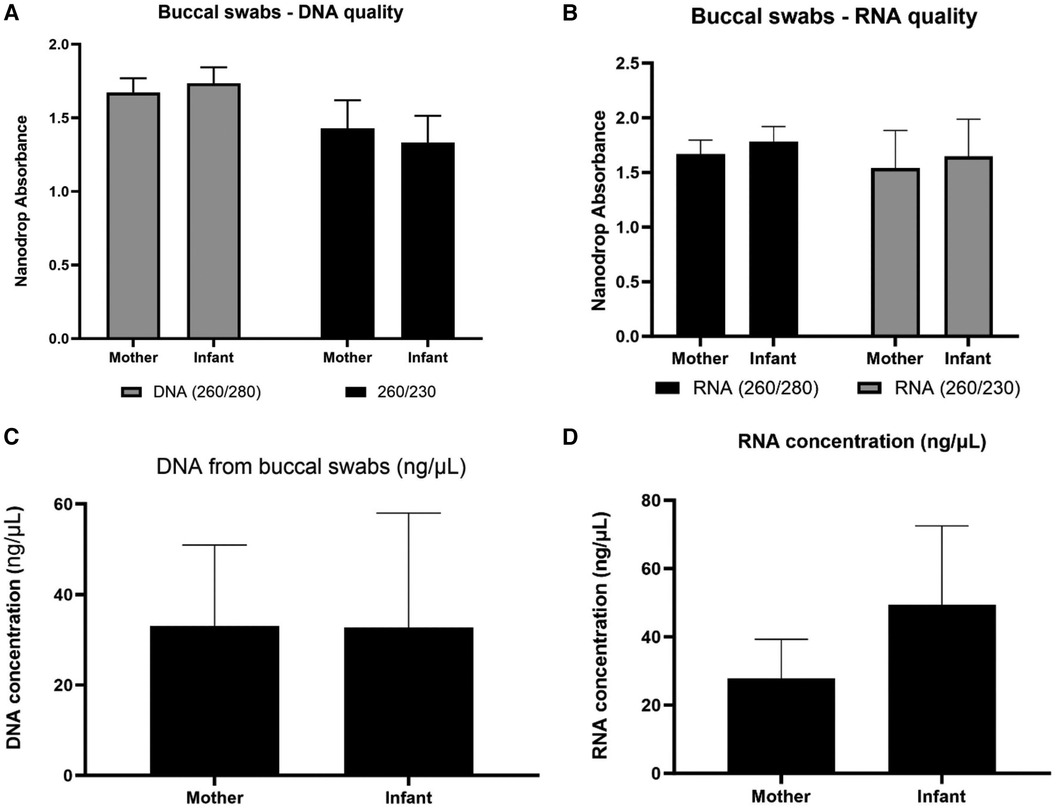
Figure 2. Nucleic acid collection results. Assessment of DNA and RNA quality (panels A,B) and quantity (panels C,D) of the IsoHelix® SK-2S kit in combination with the BFX-25® stabilizing buffer on 19 mother and 21 infant samples from the Your Baby study.
We then used the commercial assay to determine mtDNAcn for Your Baby study samples utilizing DNA available for 19 mothers and 21 infants. Our results shown in Figure 3A illustrate the mean mtDNAcn level was higher in infants (229.2 ± 66.4 S.D) than maternal samples (136.7 ± 41.8 S.D) (Figure 3A). The Your Baby samples showed a 97% concordance (Figure 3B) between the commercial mtDNAcn qPCR assay and lpWGS results.
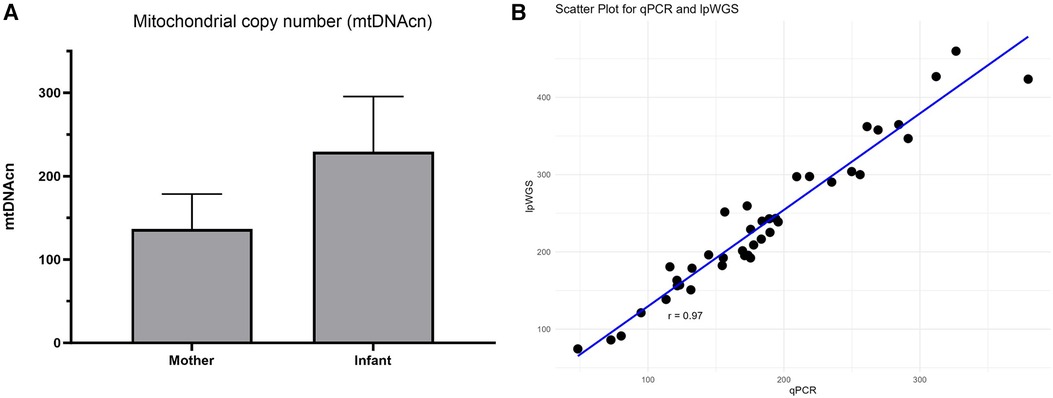
Figure 3. mtDNAcn results for commercial assay and concordance with lpWGS. Mother and infant mtDNAcn assessment using the commercial assay (panel A). Strong (Pearson r = 0.97) concordance of the commercial qPCR assay and lpWGS for mtDNAcn assessment (panel B).
3.2 Validation and performance characterization of our qPCR assay
We developed our own custom mtDNAcn qPCR assay combining methods from the commercial assay and published primers as previously described. We performed inter- and intra-day validation for our assay as described previously (Supplementary Figures S4–S6). Using three samples from the Your Baby Study, we performed a three-way comparison of our assay, the commercial assay and lpWGS assay, and showed a 100% concordance (Pearson r of 1) for all three assays (Figure 4).
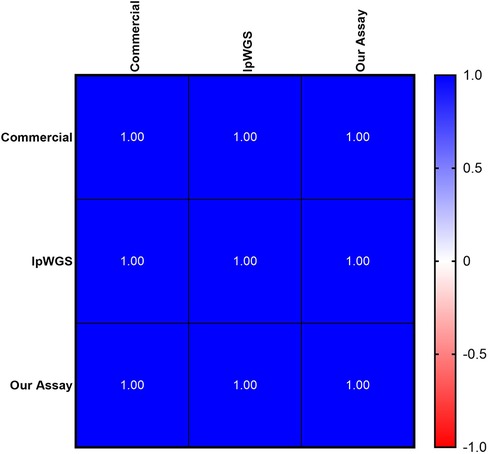
Figure 4. Concordance of mtDNAcn between commercial, lpWGS and our own assays. High (100%) concordance, depicted as Pearson's r values, of all three methodologies used to assess mtDNAcn.
Our own assay then was utilized to evaluate DNA from 164 mothers and 164 six-month old infant samples collected for the ACEs study. Figure 5 shows DNA quality, quantity and mtDNAcn from this cohort. In addition, we showed a high concordance between our assay and lpWGS on a subset of 36 ACEs samples (Pearson r = 0.94).
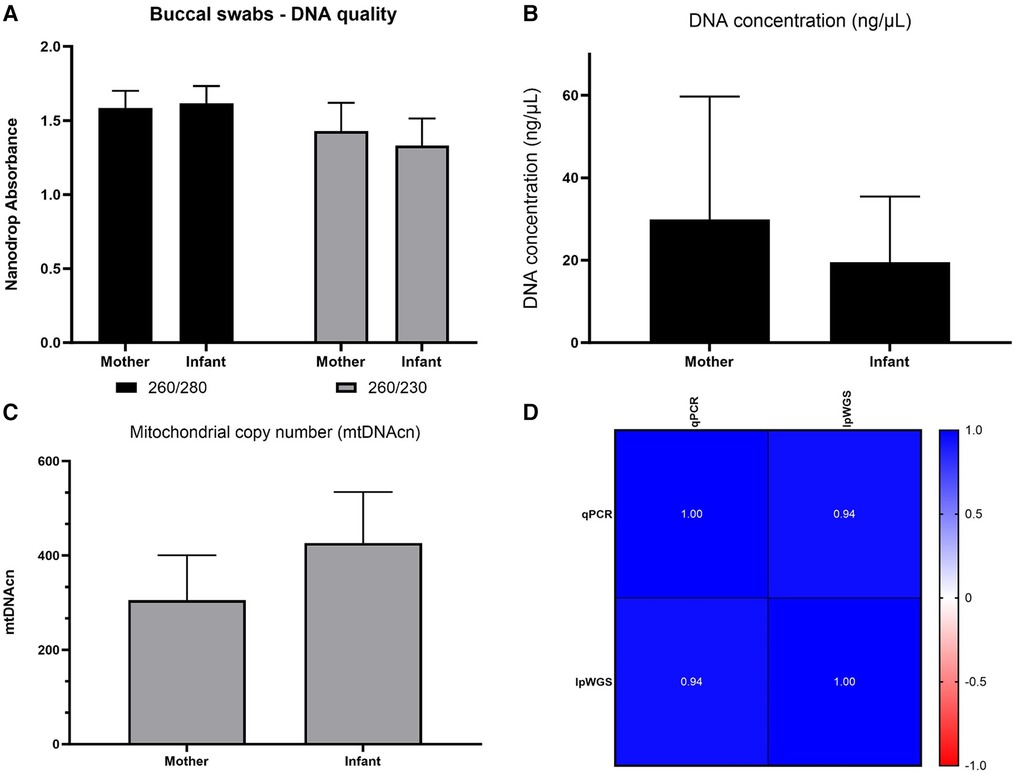
Figure 5. ACEs study: nucleic acid collection results and mtDNAcn results from our qPCR and lpWGS assays. DNA quality (Panel A), DNA quantity (Panel B), average mtDNAcn in mothers (164) and infants (164) assessed using our qPCR assay (panel C), and concordance of 36 samples re-assessed for mtDNAcn using lpWGS (panel D), depicted as Pearson's r values. RNA quality and quantity was also assessed and is shown in Supplementary Figure S7.
3.3 Mitochondrial haplogroup assessment
Mitochondrial haplogroups are defined by a set of variants in the mitochondrial genome that form specific branches of the mitochondrial phylogenetic tree (32, 33). These may be useful as markers of genetic ancestry, population migrations and environmental adaptation, and certain haplogroups have been associated with susceptibility or resistance to specific diseases (34, 35). We used the Phy-mer tool that we previously developed (33) to evaluate the mitochondrial haplogroups and determine haplogroup regions for 18 mothers for which we had lpWGS data (Figure 6). The majority of maternal haplogroups were from the “Americas” region (North and South America).
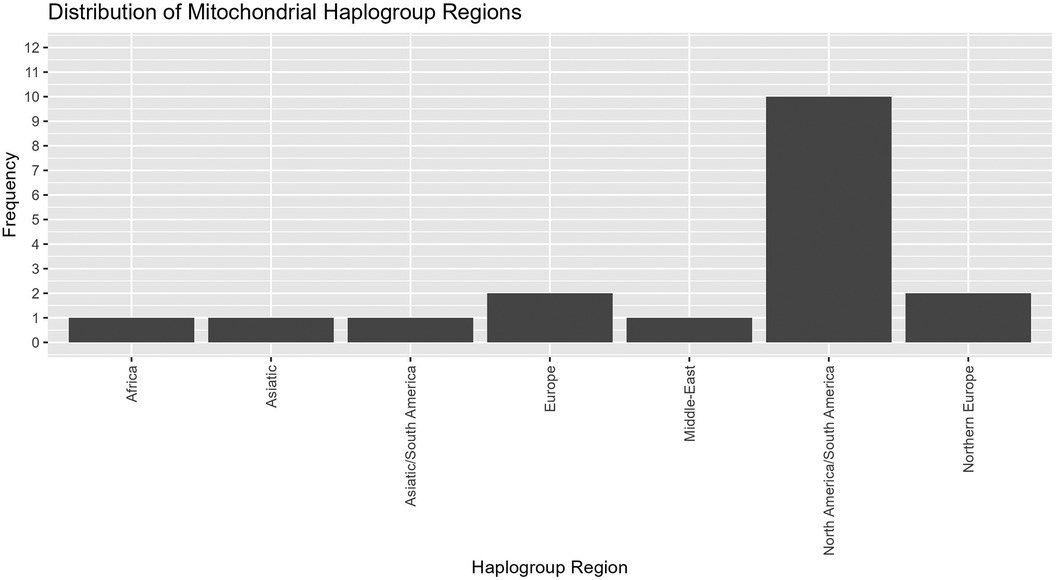
Figure 6. Mitochondrial haplogroups regions. Evaluation of mitochondrial haplogroups for 18 maternal samples that underwent lpWGS showed most falling into North America/South America, with small numbers from other haplogroup regions.
4 Discussion
We report the development and validation of mtDNA copy number assays that provide highly reliable and reproducible results from an oral biospecimen. Using the IsoHelix SK-2S buccal swab kit we were able to isolate DNA and RNA of sufficient quality and quantity from infants and adults to perform qPCR based mtDNAcn and lpWGS assays from a single sample. This makes these assays particularly useful for infants and young children for whom specimen collection and sample limitations may effectively preclude existing methods. Our own qPCR assay required as little as 2 ng of DNA per qPCR replicate and our lpWGS required 5 ng for library preparation. We demonstrated high concordance of results from our qPCR mtDNAcn assay, a commercial qPCR mtDNAcn assay, and lpWGS.
Different methods of measuring mtDNAcn have been evaluated previously (21). One study used data from two adult cohorts, the Atherosclerosis Risk in Communities (ARIC) study and the Multi-Ethnic Study of Atherosclerosis (MESA), and evaluated mtDNAcn calculated from qPCR, two microarray platforms, whole genome sequencing (WGS), and whole exome sequencing (WES) (21). The results showed that mtDNAcn calculated from WGS data had the strongest associations with known mtDNAcn correlates, such as age, sex, white blood cell count, and incident cardiovascular disease. mtDNAcn calculated from WGS also was more significantly associated with these traits compared to all other methods, including qPCR. While the current study only evaluated one DNA extraction method, the ARIC study also examined the impact of DNA extraction methods on mtDNAcn estimation and found that measuring mtDNAcn from cell lysate resulted in less variability compared to traditional methods using phenol-chloroform-isoamyl alcohol and silica-based column selection (21).
While we have demonstrated excellent reproducibility with our developed qPCR mtDNAcn assay, there are limitations to consider when using buccal swabs. Population-level variation; It is important to note that buccal swabs contain a heterogeneous mixture of cell types, including leukocytes and squamous epithelial cells. This variation reflects the physiological and genetic diversity within the population. Thus, cell type-specific alternations of mtDNAcn will not be captured by this method. Methods that are not clinically scalable can obtain such experimental data, but was not the goal of the current methods development study.
There is growing evidence that mitochondrial adaptation as part of the cell danger response (36, 37) may play a central role in various pathophysiological states, including cancer, cardiovascular disease, metabolic disorders and chronic stress (16, 19, 38–43). Mitochondrial DNA copy number is a critical determinant of mitochondrial function, influencing cellular energy production and metabolism (3). Developing mtDNAcn assays that can be used in infants and young children provides the opportunity to study whether atypical mtDNAcn may be an indicator of early pathophysiological changes that are currently challenging to identify using other measures. Use of buccal swabs for sample collection and a qPCR based method make our assay potentially cost effective and scalable, two key features for any clinical test. Our developed qPCR mtDNAcn method is likely to be cost-effective over currently available commercial kits and lpWGS, keeping in mind lpWGS methodology and technology may not be readily available to all scientists, especially in a clinical setting.
This assay could be used in the future for studies in which researchers are interested in measuring pediatric mtDNAcn. For example, longitudinal studies will be crucial (but expensive) in understanding the dynamics of mitochondrial DNA copy number (mtDNAcn) in health and disease. Future research to investigate changes in mtDNAcn over time in pediatric populations, both in healthy individuals and in those with specific health conditions. The method can be applied to determine the relation between temporal changes in mtDNAcn and child developmental milestones. This could provide insights into the natural progression of mtDNAcn and its potential as a biomarker for typical development, disease progression or treatment responsiveness. This method could also be applied in clinical settings to monitor disease progression and response to treatment in pediatric populations. For example, in pediatric oncology, mtDNAcn has been implicated in the progression of certain cancers and response to chemotherapy (8). Our method could be used to monitor changes in mtDNAcn as a potential indicator of treatment response or disease recurrence. This in turn could lead to biomarker development studies to determine the utility of mtDNAcn as a diagnostic or prognostic biomarker.
While this test has broad potential application, we are particularly interested in its utility for measuring mtDNAcn changes in infants and young children to better understand whether and how mtDNAcn may reflect developmental disruption due to early adversity. This requires study of larger populations which hopefully can be more readily accomplished using this assay.
Data availability statement
The original contributions presented in the study are included in the article/Supplementary Material, further inquiries can be directed to the corresponding author.
Ethics statement
The studies involving humans were approved by Childrens Hospital Los Angeles. The studies were conducted in accordance with the local legislation and institutional requirements. Written informed consent for participation in this study was provided by the participants’ legal guardians/next of kin.
Author contributions
SM: Data curation, Formal Analysis, Investigation, Methodology, Project administration, Software, Supervision, Validation, Visualization, Writing – original draft, Writing – review & editing. LN: Investigation, Methodology, Validation, Writing – review & editing. AO: Investigation, Methodology, Project administration, Writing – review & editing. DO: Conceptualization, Data curation, Methodology, Supervision, Validation, Writing – review & editing. YG: Data curation, Formal Analysis, Methodology, Validation, Writing – review & editing. HH: Data curation, Formal Analysis, Writing – review & editing. JC: Conceptualization, Funding acquisition, Writing – review & editing. AJ: Funding acquisition, Writing – original draft, Writing – review & editing. PL: Conceptualization, Data curation, Formal Analysis, Funding acquisition, Investigation, Methodology, Project administration, Resources, Software, Supervision, Validation, Visualization, Writing – original draft, Writing – review & editing. XG: Conceptualization, Data curation, Formal Analysis, Funding acquisition, Investigation, Methodology, Project administration, Resources, Software, Supervision, Validation, Visualization, Writing – original draft, Writing – review & editing.
Funding
The author(s) declare financial support was received for the research, authorship, and/or publication of this article.
This research project was supported by the California Initiative to Advance Precision Medicine—ACES Program (OPR20139) and The JPB Research Network on Toxic Stress: A project of the Center on the Developing Child at Harvard University. The Saban Research Institute Developmental Neuroscience and Neurogenetics Program, the Simms/Mann Family Foundation and the CHLA Center for Personalized Medicine.
Acknowledgments
We are grateful to Drs. Alma Gharib and Sahana Nagabhushan Kalburgi for guidance in establishing the full research protocol and to Suchi Patel for assisting with initial data generation for the NovaQUANT assay.
Conflict of interest
The authors declare that the research was conducted in the absence of any commercial or financial relationships that could be construed as a potential conflict of interest.
Publisher's note
All claims expressed in this article are solely those of the authors and do not necessarily represent those of their affiliated organizations, or those of the publisher, the editors and the reviewers. Any product that may be evaluated in this article, or claim that may be made by its manufacturer, is not guaranteed or endorsed by the publisher.
Supplementary material
The Supplementary Material for this article can be found online at: https://www.frontiersin.org/articles/10.3389/fped.2024.1401737/full#supplementary-material
References
1. Grochowska J, Czerwinska J, Borowski LS, Szczesny RJ. Mitochondrial RNA, a new trigger of the innate immune system. Wiley Interdiscip Rev RNA. (2022) 13(3):e1690. doi: 10.1002/wrna.1690
2. Qiu Y, Huang Y, Chen M, Yang Y, Li X, Zhang W. Mitochondrial DNA in NLRP3 inflammasome activation. Int Immunopharmacol. (2022) 108:108719. doi: 10.1016/j.intimp.2022.108719
3. Filograna R, Mennuni M, Alsina D, Larsson NG. Mitochondrial DNA copy number in human disease: the more the better? FEBS Lett. (2021) 595(8):976–1002. doi: 10.1002/1873-3468.14021
4. Picard M, Wallace DC, Burelle Y. The rise of mitochondria in medicine. Mitochondrion. (2016) 30:105–16. doi: 10.1016/j.mito.2016.07.003
6. Wallace DC. Mitochondria and cancer: Warburg addressed. Cold Spring Harb Symp Quant Biol. (2005) 70:363–74. doi: 10.1101/sqb.2005.70.035
7. Audano M, Pedretti S, Ligorio S, Crestani M, Caruso D, De Fabiani E, et al. “The loss of golden touch": mitochondria-organelle interactions, metabolism, and cancer. Cells. (2020) 9(11). doi: 10.3390/cells9112519
8. Kaneva K, Merkurjev D, Ostrow D, Ryutov A, Triska P, Stachelek K, et al. Detection of mitochondrial DNA variants at low level heteroplasmy in pediatric CNS and extra-CNS solid tumors with three different enrichment methods. Mitochondrion. (2020) 51:97–103. doi: 10.1016/j.mito.2020.01.006
9. Kopinski PK, Singh LN, Zhang S, Lott MT, Wallace DC. Mitochondrial DNA variation and cancer. Nat Rev Cancer. (2021) 21(7):431–45. doi: 10.1038/s41568-021-00358-w
10. Breda CNS, Davanzo GG, Basso PJ, Saraiva Câmara NO, Moraes-Vieira PMM. Mitochondria as central hub of the immune system. Redox Biol. (2019) 26:101255. doi: 10.1016/j.redox.2019.101255
11. Petruzzella V, Sardanelli AM, Scacco S, Panelli D, Papa F, Trentadue R, et al. Dysfunction of mitochondrial respiratory chain complex I in neurological disorders: genetics and pathogenetic mechanisms. Adv Exp Med Biol. (2012) 942:371–84. doi: 10.1007/978-94-007-2869-1_17
12. Kong C, Song W, Fu T. Systemic inflammatory response syndrome is triggered by mitochondrial damage (review). Mol Med Rep. (2022) 25(4). doi: 10.3892/mmr.2022.12663
13. Norat P, Soldozy S, Sokolowski JD, Gorick CM, Kumar JS, Chae Y, et al. Mitochondrial dysfunction in neurological disorders: exploring mitochondrial transplantation. NPJ Regen Med. (2020) 5(1):22. doi: 10.1038/s41536-020-00107-x
14. Pyle A, Anugrha H, Kurzawa-Akanbi M, Yarnall A, Burn D, Hudson G. Reduced mitochondrial DNA copy number is a biomarker of Parkinson’s disease. Neurobiol Aging. (2016) 38:216.e7–.e10. doi: 10.1016/j.neurobiolaging.2015.10.033
15. Eagleson KL, Villaneuva M, Southern RM, Levitt P. Proteomic and mitochondrial adaptations to early-life stress are distinct in juveniles and adults. Neurobiol Stress. (2020) 13:100251. doi: 10.1016/j.ynstr.2020.100251
16. Picard M, McEwen BS. Psychological stress and mitochondria: a conceptual framework. Psychosom Med. (2018) 80(2):126–40. doi: 10.1097/PSY.0000000000000544
17. Tyrka AR, Parade SH, Price LH, Kao HT, Porton B, Philip NS, et al. Alterations of mitochondrial DNA copy number and telomere length with early adversity and psychopathology. Biol Psychiatry. (2016) 79(2):78–86. doi: 10.1016/j.biopsych.2014.12.025
18. Lapp HE, Ahmed S, Moore CL, Hunter RG. Toxic stress history and hypothalamic-pituitary-adrenal axis function in a social stress task: genetic and epigenetic factors. Neurotoxicol Teratol. (2019) 71:41–9. doi: 10.1016/j.ntt.2018.01.011
19. Zitkovsky EK, Daniels TE, Tyrka AR. Mitochondria and early-life adversity. Mitochondrion. (2021) 57:213–21. doi: 10.1016/j.mito.2021.01.005
20. Memon AA, Zoller B, Hedelius A, Wang X, Stenman E, Sundquist J, et al. Quantification of mitochondrial DNA copy number in suspected cancer patients by a well optimized ddPCR method. Biomol Detect Quantif. (2017) 13:32–9. doi: 10.1016/j.bdq.2017.08.001
21. Longchamps RJ, Castellani CA, Yang SY, Newcomb CE, Sumpter JA, Lane J, et al. Evaluation of mitochondrial DNA copy number estimation techniques. PLoS One. (2020) 15(1):e0228166. doi: 10.1371/journal.pone.0228166
22. Picard M. Blood mitochondrial DNA copy number: what are we counting? Mitochondrion. (2021) 60:1–11. doi: 10.1016/j.mito.2021.06.010
23. Dong Z, Zhang J, Hu P, Chen H, Xu J, Tian Q, et al. Low-pass whole-genome sequencing in clinical cytogenetics: a validated approach. Genet Med. (2016) 18(9):940–8. doi: 10.1038/gim.2015.199
24. Gillio-Meina C, Cepinskas G, Cecchini EL, Fraser DD. Translational research in pediatrics II: blood collection, processing, shipping, and storage. Pediatrics. (2013) 131(4):754–66. doi: 10.1542/peds.2012-1181
25. Howie SR. Blood sample volumes in child health research: review of safe limits. Bull World Health Organ. (2011) 89(1):46–53. doi: 10.2471/BLT.10.080010
26. Cohen LL. Behavioral approaches to anxiety and pain management for pediatric venous access. Pediatrics. (2008) 122(Suppl 3):S134–9. doi: 10.1542/peds.2008-1055f
27. Kennedy RM, Luhmann J, Zempsky WT. Clinical implications of unmanaged needle-insertion pain and distress in children. Pediatrics. (2008) 122(Suppl 3):S130–3. doi: 10.1542/peds.2008-1055e
28. Meyer A, Paroni F, Gunther K, Dharmadhikari G, Ahrens W, Kelm S, et al. Evaluation of existing methods for human blood mRNA isolation and analysis for large studies. PLoS One. (2016) 11(8):e0161778. doi: 10.1371/journal.pone.0161778
29. Finlin BS, Memetimin H, Confides AL, Kasza I, Zhu B, Vekaria HJ, et al. Human adipose beiging in response to cold and mirabegron. JCI Insight. (2018) 3(15). doi: 10.1172/jci.insight.121510
30. Livak KJ, Schmittgen TD. Analysis of relative gene expression data using real-time quantitative PCR and the 2[-Delta Delta C(T)] method. Methods. (2001) 25(4):402–8. doi: 10.1006/meth.2001.1262
31. Qian Y, Butler TJ, Opsahl-Ong K, Giroux NS, Sidore C, Nagaraja R, et al. Fastmitocalc: an ultra-fast program to estimate mitochondrial DNA copy number from whole-genome sequences. Bioinformatics. (2017) 33(9):1399–401. doi: 10.1093/bioinformatics/btw835
32. Kaneva K, Schurr TG, Tatarinova TV, Buckley J, Merkurjev D, Triska P, et al. Mitochondrial DNA haplogroup, genetic ancestry, and susceptibility to Ewing sarcoma. Mitochondrion. (2022) 67:6–14. doi: 10.1016/j.mito.2022.09.002
33. Navarro-Gomez D, Leipzig J, Shen L, Lott M, Stassen AP, Wallace DC, et al. Phy-Mer: a novel alignment-free and reference-independent mitochondrial haplogroup classifier. Bioinformatics. (2015) 31(8):1310–2. doi: 10.1093/bioinformatics/btu825
34. Alwehaidah MS, Bakhiet M, AlFadhli S. Mitochondrial haplogroup reveals the genetic basis of diabetes mellitus type 2 comorbidity in psoriasis. Med Princ Pract. (2021) 30(1):62–8. doi: 10.1159/000509937
35. Canter JA, Haas DW, Kallianpur AR, Ritchie MD, Robbins GK, Shafer RW, et al. The mitochondrial pharmacogenomics of haplogroup T: MTND2*LHON4917G and antiretroviral therapy-associated peripheral neuropathy. Pharmacogenomics J. (2008) 8(1):71–7. doi: 10.1038/sj.tpj.6500470
36. Naviaux RK. Metabolic features and regulation of the healing cycle-A new model for chronic disease pathogenesis and treatment. Mitochondrion. (2019) 46:278–97. doi: 10.1016/j.mito.2018.08.001
37. Naviaux RK. Metabolic features of the cell danger response. Mitochondrion. (2014) 16:7–17. doi: 10.1016/j.mito.2013.08.006
38. Trumpff C, Michelson J, Lagranha CJ, Taleon V, Karan KR, Sturm G, et al. Stress and circulating cell-free mitochondrial DNA: a systematic review of human studies, physiological considerations, and technical recommendations. Mitochondrion. (2021) 59:225–45. doi: 10.1016/j.mito.2021.04.002
39. Trumpff C, Marsland AL, Basualto-Alarcon C, Martin JL, Carroll JE, Sturm G, et al. Acute psychological stress increases serum circulating cell-free mitochondrial DNA. Psychoneuroendocrinology. (2019) 106:268–76. doi: 10.1016/j.psyneuen.2019.03.026
40. Sharma P, Sampath H. Mitochondrial DNA integrity: role in health and disease. Cells. (2019) 8(2):21. doi: 10.3390/cells8020100
41. Daniels TE, Olsen EM, Tyrka AR. Stress and psychiatric disorders: the role of mitochondria. Annu Rev Clin Psychol. (2020) 16:165–86. doi: 10.1146/annurev-clinpsy-082719-104030
42. Chaudhari PR, Singla A, Vaidya VA. Early adversity and accelerated brain aging: a mini-review. Front Mol Neurosci. (2022) 15:822917. doi: 10.3389/fnmol.2022.822917
Keywords: mitochondrial DNA copy number, adversity, lpWGS, adverse childhood experience (ACE), DNA RNA extraction
Citation: Maggo S, North LY, Ozuna A, Ostrow D, Grajeda YR, Hakimjavadi H, Cotter JA, Judkins AR, Levitt P and Gai X (2024) A method for measuring mitochondrial DNA copy number in pediatric populations. Front. Pediatr. 12:1401737. doi: 10.3389/fped.2024.1401737
Received: 15 March 2024; Accepted: 31 May 2024;
Published: 13 June 2024.
Edited by:
Enrico Baruffini, University of Parma, ItalyReviewed by:
Yolanda Cámara, Vall d'Hebron Research Institute (VHIR), SpainHakan Öğütlü, Cognitive Behavioral Psychotherapies Association, Türkiye
© 2024 Maggo, North, Ozuna, Ostrow, Grajeda, Hakimjavadi, Cotter, Judkins, Levitt and Gai. This is an open-access article distributed under the terms of the Creative Commons Attribution License (CC BY). The use, distribution or reproduction in other forums is permitted, provided the original author(s) and the copyright owner(s) are credited and that the original publication in this journal is cited, in accordance with accepted academic practice. No use, distribution or reproduction is permitted which does not comply with these terms.
*Correspondence: Xiaowu Gai, eGdhaUBjaGxhLnVzYy5lZHU=