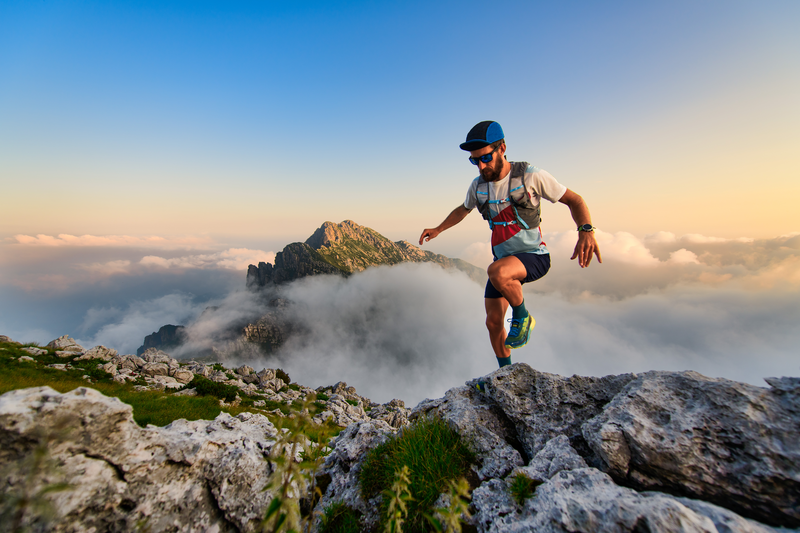
95% of researchers rate our articles as excellent or good
Learn more about the work of our research integrity team to safeguard the quality of each article we publish.
Find out more
BRIEF RESEARCH REPORT article
Front. Pediatr. , 02 July 2024
Sec. Pediatric Infectious Diseases
Volume 12 - 2024 | https://doi.org/10.3389/fped.2024.1381310
Biomarkers play a crucial role in the early identification of high-risk children with infectious diseases. Despite their importance, few studies evaluated biomarkers' capabilities in predicting mortality. The aim of this study was to evaluate the biomarkers' predictive capabilities for mortality in children with infectious diseases. From an inpatient database covering ≥200 acute-care hospitals in Japan, we included children who underwent blood culture, and received antimicrobial treatment between 2012 and 2021. Biomarkers' results from the day of the initial blood culture were used. Biomarker discriminative capabilities were assessed using the area under receiver operating characteristic curves (AUCs). Of 11,365 eligible children with presumed infection, 1,378 (12.1%) required mechanical ventilation or vasoactive agents within 2 days of blood culture, and 100 (0.9%) died during admission. Of all children, 10,348 (91.1%) had community-onset infections and 1,017 (8.9%) had hospital-onset infections. C-reactive protein and white blood cell demonstrated limited discriminatory capabilities with AUCs of 0.44 [95% confidence interval (CI): 0.38–0.51] and 0.45 (95% CI: 0.39–0.52). In contrast, pH, prothrombin time-international normalized ratio, and procalcitonin exhibited strong discriminatory capabilities with AUCs of 0.77 (95% CI: 0.65–0.90), 0.77 (95% CI: 0.70–0.84) and 0.76 (95% CI: 0.29–1.00). In conclusions, our real-world data analysis suggested that C-reactive protein and white blood cell may not be reliable indicators for predicting mortality in children with presumed infection. These findings could warrant future studies exploring promising biomarkers, including those from blood gas analyses, coagulation studies and procalcitonin.
Early identification and prompt treatment of children at elevated risk of mortality from infectious diseases are imperative, especially considering the significant number of pediatric deaths associated with serious infections (1). Several approaches have been proposed, including the use of predictive biomarkers for serious bacterial infections and the definition and identification of pediatric sepsis (2–4). However, the clinical application of these approaches remains challenging (5, 6).
Many of the existing studies evaluating the capabilities of biomarkers have focused primarily on serious bacterial infections as the outcome. Such outcome often has a low positive predictive value for mortality, casting doubts on the robustness of these biomarkers in predicting mortality (7–9). Moreover, although a few studies have evaluated biomarkers' capabilities to predict mortality, they have mostly been conducted in pediatric intensive care units (PICUs), which inherently admit children already deemed at risk of mortality. Consequently, this bias leaves an unaddressed knowledge gap regarding the risk stratification of children presenting with infectious diseases in emergency departments or general wards, where serious infections are often first recognized (4, 7, 10). Therefore, our study aimed to evaluate the predictive capabilities of biomarkers, tested when serious infections were initially suspected, for mortality in children admitted with infectious diseases across diverse settings in over 200 acute-care hospitals.
We performed a retrospective cohort study using an in/outpatient database managed by the Health, Clinic, and Education Information Evaluation Institute (Kyoto, Japan), which is a non-profit research service foundation, with support from Real World Data Co, Ltd (Kyoto, Japan). Details of the database are described elsewhere (11); briefly, it includes medical records, blood test results, and administrative claims data from over 200 hospitals from Kyushu to Hokkaido region of Japan. The database contains the following data: patient characteristics, diagnosis, medications and procedures during admission, and discharge status. This study was approved by the Institutional Review Board of Teikyo University (approval number: 22-019; May 20, 2020), and the procedures were in accordance with the ethical standards of the responsible committee on human experimentation and with the Helsinki Declaration of 1975. Due to the anonymity of the data the requirement of informed consent was waived.
We applied the following inclusion and exclusion criteria. We included children under 18 years of age who were admitted between January 2012 and December 2021, underwent blood culture, and received intravenous antimicrobial agents (antibiotics, antivirus and antifungals) starting within a window of 2 days before and after blood culture. Exclusion criteria were as follows: subsequent infectious events in previously included cases; neonates hospitalized since birth; use of mechanical ventilation or vasoactive agents priori to blood culture.
We stratified the children into the four severity groups: (i) short-term antimicrobial use for <4 days, (ii) antimicrobial treatment for four or more consecutive days, (iii) critical illness, defined as the initiation of mechanical ventilation or vasoactive agents within 2 days after blood culture, (iv) in-hospital death. Vasoactive agents included dopamine, epinephrine, norepinephrine, phenylephrine, or vasopressin.
The primary outcome was in-hospital mortality. We examined the following biomarkers: C-reactive protein (CRP), procalcitonin, white blood cell, platelet, prothrombin time international normalized ratio (PT-INR), pH, base excess, lactate, aspartate aminotransferase, alanine aminotransferase, bilirubin, creatine. The primary test was to calculate the area under a receiver-operator characteristic curve (AUC) of the results of biomarkers tested on the same day as blood culture for predicting in-hospital mortality. For each biomarker analysis, patients with missing data were excluded and no imputation for missing data was performed. We performed sensitivity analyses to assess the robustness of the primary test, by (i) using the worst values of biomarkers within 3 days and 7 days of blood culture, (ii) by time of infection onset, (iii) by dispositions, (iv) by age categories, (v) by excluding immunocompromised children, defined as those with malignant, haematological or immunological diagnoses, and (vi) by using an area under the precision-recall curve. Patients were classified as “community-onset infection” if neither blood culture nor antimicrobial initiation occurred on the first or second day of admission, or both, or “hospital-onset infection” if both blood culture and antimicrobial initiation occurred on the third day of admission or later (12). Patients were classified as “ICU” if admitted to an intensive care unit (ICU) or “ward” if admitted to a non-ICU setting on the day of blood culture collection. All analyses were performed using STATA 17 (StataCorp LLC, College Station, TX, USA).
Of 11,365 eligible children with presumed infectious diseases, 1,378 (12.1%) required mechanical ventilation or vasoactive agents within 2 days of blood culture, and 100 (0.9%) died during admission (Figure 1). The median age was 1 year (interquartile range: 0–5 years) with 4,958 (43.6%) being female. Of all children, 10,348 (91.1%) had community-onset infections and 1,017 (8.9%) had hospital-onset infections. By disposition, 2,024 (17.8%) children were admitted to ICUs on the day of blood culture (Table 1). Six biomarkers were tested in at least 80% of these children: CRP, white blood cell, platelet, aspartate aminotransferase, alanine aminotransferase, creatine. Meanwhile, pH, base excess and lactate were tested in 31.9%, 32.0% and 27.8%. PT-INR and Procalcitonin were tested in 24.1% and 16.6%, respectively (Table 2).
Table 1 Characteristics, therapies and outcomes of 11,365 children with presumed infectious diseases.
CRP and white blood cell showed poor discriminatory capabilities with AUCs of 0.44 [95% confidence interval (CI): 0.38–0.51] and 0.45 (95% CI: 0.39–0.52). On the other hand, pH, PT-INR and procalcitonin demonstrated strong discriminatory capabilities with AUCs of 0.77 (95% CI: 0.65–0.90), 0.77 (95% CI: 0.70–0.84) and 0.76 (95% CI: 0.29–1.00), respectively (Table 2 and Supplementary Figure S1).
Sensitivity analyses by using the worst values of the biomarkers within 3 and 7 days showed similar results. The AUCs of CRP increased slightly to 0.57 (95% CI: 0.51–0.64) and 0.63 (95% CI: 0.45–1.00), while the AUCs of white blood cell experienced negligible increases, standing at 0.49 (95% CI: 0.37–0.61) and 0.50 (95% CI: 0.56–0.69) for 3 and 7 days, respectively. In contrast, pH, PT-INR and procalcitonin consistently demonstrated strong discriminatory capabilities, irrespective of the duration of biomarkers’ tests (Table 2).
Sensitivity analyses by time of infection onset and disposition showed similar results (Supplementary Tables S1 and S2). CRP and white blood cell recorded AUCs ranging between 0.33–0.57 and 0.42–0.50, depending on the patient situation. Meanwhile, pH, PT-INR and procalcitonin exhibited AUCs spanning 0.65–0.87, 0.73–0.77 and 0.53–1.00, respectively. Sensitivity analyses by age and immunocompromised status showed similar results with the primary test (Supplementary Tables S3 and S4). Sensitivity analysis using precision-recall curves showed a 5-fold or greater increase in discriminatory accuracy for procalcitonin, PT-INR, pH, lactate, aspartate aminotransferase, alanine aminotransferase and creatine. In contrast, CRP and white blood cell did not improve the discriminatory accuracy (Supplementary Table S5).
By severity groups, 2,807 (24.7%), 7,155 (63.0%), 1,303 (11.5%), and 100 (0.9%) children fell in (i) antimicrobials <4 days, (ii) antimicrobials ≥4 days, (iii) critical illness, and (iv) in-hospital death, respectively. In the higher severity groups, there were progressively worsening trends in eight biomarkers: procalcitonin, PT-INR, platelet, pH, lactate, base excess, aspartate aminotransferase, alanine aminotransferase (Figure 2). In contrast, CRP and white blood cell did not display consistent trends across varying severities.
Figure 2 Biomarkers’ results by the severity of infection. Children were classified into the four severities groups: (i) short-term antimicrobial use for <4 days, (ii) antimicrobial treatment for ≥4 days, (iii) critical illness, defined as the initiation of mechanical ventilation or vasoactive agents within 2 days after blood culture, (iv) in-hospital death. PT-INR, prothrombin time international normalized ratio.
This analysis of real-world data evaluating biomarkers' capabilities to predict in-hospital mortality in children with presumed infectious diseases, demonstrated poor discriminatory capabilities in CRP and white blood cell and strong discriminatory capabilities in pH, PT-INR and procalcitonin.
While previous studies reported a strong discriminatory capability of CRP for serious bacterial infection, we found its poor discriminatory capability for mortality. One study reported the discriminatory capabilities of CRP with AUCs of 0.81 for serious bacterial infection and 0.43 for mortality in children presenting with suspected meningitis or pneumonia (13). One possible interpretation was that the outcome setting could significantly affect the biomarker's discrimination, given serious bacterial infections, defined as culture-proven invasive infections in many studies, do not necessarily indicate a high risk of death and need for intensive care (14). Another possible reason for our findings could be the delayed rise in CRP levels. A systematic review reported an improved discrimination of mortality with late-phase CRP levels compared to early-phase CRP levels recorded within 48 h. Similarly, our sensitivity analyses showed improved discrimination of CRP over extended observation periods. However, such marginal improvements did not suggest the clinical usefulness of CRP as an early predictor, as early recognition and timely treatment are crucial in managing severely-ill children (15). In addition, the cohort in our study may have included non-infectious cases characterized by high CRP levels and low mortality (e.g., Kawasaki disease, etc.). The international variation in disease incidence may have led to the discrepancy in CRP's performance. Furthermore, elevated CRP levels have been associated with improved outcomes in previous studies of selected cohorts with severe infections. This might be explained by spuriously low CRP levels due to an insufficient hepatic production, as CRP is a protein mainly synthesized in liver hepatocytes (16).
In our study, white blood cell count failed to discriminate the mortality. This aligned with previous studies showing its poor discrimination for mortality, with a limited sensitivity and specificity (8, 17).
Our study identified potentially predictive biomarkers including procalcitonin, pH, and PT-INR. Previous studies have shown that procalcitonin is useful in the early identification of children with serious bacterial infections at risk of mortality. It may be the time to discuss the implementation of procalcitonin testing among severely-ill children at risk of mortality, although the test should be judiciously reserved for properly-selected children due to its high cost. Similar to our findings, some of the biomarkers have been successfully used in ICU severity scores to accurately predict mortality e.g., PT-INR, aspartate aminotransferase, platelet, creatinine and pH in PELOD 2 and PRISM 3, although their validation in the general pediatric cohort hospitalized with infectious diseases is limited (17, 18). Other studies have demonstrated strong discriminatory capabilities of acidosis and PT-INR as a single biomarker or as a part of the prediction model (18–20). However, these studies have predominantly focused on specific conditions such as malaria or non-infectious cohorts (e.g., patients with traumatic injuries). Consequently, there is still a knowledge gap in the application of pH and PT-INR to predict mortality in children with infectious diseases. This gap warrants future studies to explore these potentially promising biomarkers.
To the best of our knowledge, this was the first study to evaluate the biomarkers' capabilities to predict mortality in children in a huge sample size. However, this study had limitations. First, the inclusion criteria based on blood culture test and the initiation of intravenous antimicrobial agents may have resulted in different cohorts from those in other studies. However, we believe that our approach was the best available and reproducible to incorporate children with presumed infectious diseases into this analysis. Secondly, since not all children underwent every type of blood test, we could not directly compare the discriminatory capabilities of biomarkers. Third, in immunocompromised children, the discriminatory capabilities of biomarkers may have been affected due to their decreased baseline levels and suppressed biomarker responses. However, a sensitivity analysis by excluding immunocompromised children showed a similar result (Supplementary Table S2). Lastly, biomarkers that were tested less frequently may have been indicative of severely-ill children, who physicians thought needed more extensive evaluation. This may have influenced the calculated AUCs.
Our real-world data analysis suggested that CRP and white blood cell may not be reliable indicators for predicting mortality in children with presumed infectious diseases. These findings could warrant future studies exploring promising biomarkers, including those from blood gas analyses, coagulation studies and procalcitonin.
The data analyzed in this study was obtained from Real World Data Co, Ltd (Kyoto, Japan). Requests to access these datasets should be directed to https://rwdata.co.jp/contact/.
The studies involving humans were approved by Institutional Review Board of Teikyo University. The studies were conducted in accordance with the local legislation and institutional requirements. The ethics committee/institutional review board waived the requirement of written informed consent for participation from the participants or the participants’ legal guardians/next of kin because of the anonymity of the data in this study.
SM: Conceptualization, Data curation, Formal Analysis, Funding acquisition, Investigation, Methodology, Project administration, Software, Supervision, Validation, Writing – original draft, Writing – review & editing. TK: Conceptualization, Formal Analysis, Methodology, Supervision, Writing – original draft, Writing – review & editing, Validation. YN: Conceptualization, Formal Analysis, Methodology, Supervision, Writing – original draft, Writing – review & editing, Validation.
The author(s) declare financial support was received for the research, authorship, and/or publication of this article.
This study was supported by “The Japan Foundation for Pediatric Research” (Grant No. 21-013) and the Ministry of Health, Labor and Welfare, Japan (Grant No. 24K19510).
The authors thank the Health, Clinic, and Education Information Evaluation Institute, for the database development for the study, and Mrs. E. Sakaguchi and her colleagues at Real World Data Co, Ltd. for providing the patient data.
TK received payment for lectures from Merck Sharp & Dohme Corp.
The remaining authors declare that the research was conducted in the absence of any commercial or financial relationships that could be construed as a potential conflict of interest.
All claims expressed in this article are solely those of the authors and do not necessarily represent those of their affiliated organizations, or those of the publisher, the editors and the reviewers. Any product that may be evaluated in this article, or claim that may be made by its manufacturer, is not guaranteed or endorsed by the publisher.
The Supplementary Material for this article can be found online at: https://www.frontiersin.org/articles/10.3389/fped.2024.1381310/full#supplementary-material
1. Rudd KE, Johnson SC, Agesa KM, Shackelford KA, Tsoi D, Kievlan DR, et al. Global, regional, and national sepsis incidence and mortality, 1990–2017: analysis for the global burden of disease study. Lancet. (2020) 395:200–11. doi: 10.1016/S0140-6736(19)32989-7
2. Matics TJ, Sanchez-Pinto LN. Adaptation and validation of a pediatric sequential organ failure assessment score and evaluation of the sepsis-3 definitions in critically ill children. JAMA Pediatr. (2017) 171:e172352. doi: 10.1001/jamapediatrics.2017.2352
3. Solé-Ribalta A, Launes C, Felipe-Villalobos A, Balaguer M, Luaces C, Garrido R, et al. New multivariable prediction model PEdiatric SEpsis recognition and stratification (PESERS score) shows excellent discriminatory capacity. Acta Paediatr Int J Paediatr. (2022) 111:1209–19. doi: 10.1111/apa.16321
4. Alejandre C, Guitart C, Balaguer M, Torrús I, Bobillo-Perez S, Cambra FJ, et al. Use of procalcitonin and C-reactive protein in the diagnosis of bacterial infection in infants with severe bronchiolitis. Eur J Pediatr. (2021) 180:833–42. doi: 10.1007/s00431-020-03790-6
5. Zallocco F, Osimani P, Carloni I, Romagnoli V, Angeloni S, Cazzato S. Assessment of clinical outcome of children with sepsis outside the intensive care unit. Eur J Pediatr. (2018) 177:1775–83. doi: 10.1007/s00431-018-3247-2
6. Menon K, Schlapbach LJ, Akech S, Argent A, Biban P, Carrol ED, et al. Criteria for pediatric sepsis—a systematic review and meta-analysis by the pediatric sepsis definition taskforce. Crit Care Med. (2022) 50:21–36. doi: 10.1097/CCM.0000000000005294
7. Oruganti S, Evans J, Cromarty T, Javaid A, Roland D. Identification of sepsis in paediatric emergency departments: a scoping review. Acta Paediatr. (2022) 111:2262–77. doi: 10.1111/apa.16536
8. Milcent K, Faesch S, Guen CG Le, Dubos F, Poulalhon C, Badier I, et al. Use of procalcitonin assays to predict serious bacterial infection in young febrile infants. JAMA Pediatr (2016) 170:62–9. doi: 10.1001/jamapediatrics.2015.3210
9. Nijman RG, Moll HA, Smit FJ, Gervaix A, Weerkamp F, Vergouwe Y, et al. C-reactive protein, procalcitonin and the lab-score for detecting serious bacterial infections in febrile children at the emergency department: a prospective observational study. Pediatr Infect Dis J. (2014) 33:e273–9. doi: 10.1097/INF.0000000000000466
10. Rey C, Los Arcos M, Concha A, Medina A, Prieto S, Martinez P, et al. Procalcitonin and C-reactive protein as markers of systemic inflammatory response syndrome severity in critically ill children. Intensive Care Med. (2007) 33:477–84. doi: 10.1007/s00134-006-0509-7
11. Ono Y, Taneda Y, Takeshima T, Iwasaki K, Yasui A. Validity of claims diagnosis codes for cardiovascular diseases in diabetes patients in Japanese administrative database. Clin Epidemiol. (2020) 12:367–75. doi: 10.2147/CLEP.S245555
12. Centers for Disease Control and Prevention. Hospital Toolkit. Atlanta, USA: Centers for Disease Control and Prevention (2018). Available online at: https://www.cdc.gov/sepsis/pdfs/Sepsis-Surveillance-Toolkit-Mar-2018_508.pdf#:∼:text=In (accessed June 9, 2024). 2017%2C a CDC Prevention Epicenters-funded consortium published, objective clinical data elements conceptually analogous to Sepsis-3.
13. Carrol ED, Mankhambo LA, Jeffers G, Parker D, Guiver M, Newland P, et al. The diagnostic and prognostic accuracy of five markers of serious bacterial infection in Malawian children with signs of severe infection. PLoS One. (2009) 4:e6621. doi: 10.1371/journal.pone.0006621
14. Agyeman PKA, Schlapbach LJ, Giannoni E, Stocker M, Posfay-Barbe KM, Heininger U, et al. Epidemiology of blood culture-proven bacterial sepsis in children in Switzerland: a population-based cohort study. Lancet Child Adolesc Health. (2017) 1:124–33. doi: 10.1016/S2352-4642(17)30010-X
15. Egi M, Ogura H, Yatabe T, Atagi K, Inoue S, Iba T, et al. The Japanese clinical practice guidelines for management of sepsis and septic shock 2020 (J-SSCG 2020). J Intensive Care. (2021) 9:53. doi: 10.1186/s40560-021-00555-7
16. Sproston NR, Ashworth JJ. Role of C-reactive protein at sites of inflammation and infection. Front Immunol. (2018) 9:754. doi: 10.3389/fimmu.2018.00754
17. Andreola B, Bressan S, Callegaro S, Liverani A, Plebani M, Da Dalt L. Procalcitonin and C-reactive protein as diagnostic markers of severe bacterial infections in febrile infants and children in the emergency department. Pediatr Infect Dis J. (2007) 26:672–7. doi: 10.1097/INF.0b013e31806215e3
18. Chandna A, Tan R, Carter M, Van Den Bruel A, Verbakel J, Koshiaris C, et al. Predictors of disease severity in children presenting from the community with febrile illnesses: a systematic review of prognostic studies. BMJ Glob Health. (2021) 6:e003451. doi: 10.1136/bmjgh-2020-003451
19. Von Seidlein L, Olaosebikan R, Hendriksen ICE, Lee SJ, Adedoyin OT, Agbenyega T, et al. Predicting the clinical outcome of severe falciparum malaria in African children: findings from a large randomized trial. Clin Infect Dis. (2012) 54:1080–90. doi: 10.1093/cid/cis034
Keywords: biomarker, predict, infection, sepsis, child, mortality
Citation: Miura S, Katsuta T and Nakamura Y (2024) Predictive biomarker of mortality in children with infectious diseases: a nationwide data analysis. Front. Pediatr. 12:1381310. doi: 10.3389/fped.2024.1381310
Received: 3 February 2024; Accepted: 10 June 2024;
Published: 2 July 2024.
Edited by:
Andrew S. Day, University of Otago, New ZealandReviewed by:
Judith Ju Ming Wong, KK Women’s and Children’s Hospital, Singapore© 2024 Miura, Katsuta and Nakamura. This is an open-access article distributed under the terms of the Creative Commons Attribution License (CC BY). The use, distribution or reproduction in other forums is permitted, provided the original author(s) and the copyright owner(s) are credited and that the original publication in this journal is cited, in accordance with accepted academic practice. No use, distribution or reproduction is permitted which does not comply with these terms.
*Correspondence: Shinya Miura, c2hpbi5ueWExOTgyQGdtYWlsLmNvbQ==
Disclaimer: All claims expressed in this article are solely those of the authors and do not necessarily represent those of their affiliated organizations, or those of the publisher, the editors and the reviewers. Any product that may be evaluated in this article or claim that may be made by its manufacturer is not guaranteed or endorsed by the publisher.
Research integrity at Frontiers
Learn more about the work of our research integrity team to safeguard the quality of each article we publish.