- 1Department of Industrial Design, Eindhoven University of Technology, Eindhoven, Netherlands
- 2Obstetrics and Gynaecology, Máxima Medical Centre, Veldhoven, Netherlands
- 3Institute for Applied Medical Engineering and Clinic for Neonatology, University Hospital Aachen, Aachen, Germany
- 4Department of Pediatrics, Leiden University Medical Center, Leiden, Netherlands
- 5Department of Electrical Engineering, Eindhoven University of Technology, Eindhoven, Netherlands
- 6Department of Biomedical Engineering, Eindhoven University of Technology, Eindhoven, Netherlands
To improve care for extremely premature infants, the development of an extrauterine environment for newborn development is being researched, known as Artificial Placenta and Artificial Womb (APAW) technology. APAW facilitates extended development in a liquid-filled incubator with oxygen and nutrient supply through an oxygenator connected to the umbilical vessels. This setup is intended to provide the optimal environment for further development, allowing further lung maturation by delaying gas exposure to oxygen. This innovative treatment necessitates interventions in obstetric procedures to transfer an infant from the native to an artificial womb, while preventing fetal-to-neonatal transition. In this narrative review we analyze relevant fetal physiology literature, provide an overview of insights from APAW studies, and identify considerations for the obstetric procedure from the native uterus to an APAW system. Lastly, this review provides suggestions to improve sterility, fetal and maternal well-being, and the prevention of neonatal transition.
1 Introduction
Current care for extremely premature infants (<28 weeks of gestational age) involves intensive care, including mechanical ventilation and gastrointestinal nutrition support. Due to their fragile and immature organs coupled with the limits of mechanical ventilation, these patients' mortality and morbidity rates remain high (1, 2). The development of extracorporeal life support to prolong infant organ maturation in a womb-like environment, the artificial womb (AW), has been studied since the 1950s (3, 4). This involves maintaining the infant in a fetal physiological state [i.e., as a perinate (5)] with the lungs submerged in amniotic fluid and maintaining a fetal blood circuit through the umbilical vessels via an artificial placenta (AP). Progress on the development of a fluid environment, maintenance of fetal circulation, and physiological placental blood flow have all led to raised hopes that clinical application has come within reach (3, 6–12). Now that human trials are under consideration (12, 13), the methods used in animal studies must be translated to suit human patients. To date, most experimental research on the technology (except Chamberlain (14) and Westin (15)) has been performed on animal models, which are crucial for the translation of the technology to human patients; however, translational success is uncertain (16). The focus has been predominantly on the critical requirements for continuing fetal homeostasis, meaning sustaining fetal animals for an extended period on external life support by developing and controlling key cardiovascular needs in a liquid environment. Future treatment using such a system also requires changes in the preterm birth care procedure, as the infant would need to be cannulated to an oxygenator and placed in an AW instead of a neonatal incubator. In animal studies, transfer to the AW has been controlled using medication and sedation. In case clinical trials demonstrate improvements in care compared with conventional neonatal incubators, it could significantly impact how obstetric and neonatal care is provided to extremely premature infants.
In this review, we analyze the factors that must be considered from an obstetric standpoint. We emphasize the need for studies on the intervention of fetal-to-neonatal transition within an AW context and provide an overview of the relevant transitional physiology, as it applies to various obstetric approaches. We mapped the key aspects of perinatal physiological changes and reflected on how certain measures should be in place when transferring a perinate to an AW. Second, we analyzed current AW studies and patents, and provided an overview of the transfer methods used. We conclude with considerations and research directions for obstetric procedure development and propose to supplement preclinical investigations with simulation technologies to build further knowledge.
2 Data sources
Insights on the physiology of a fetus at birth were gathered through a literature search that informed us about specific milestones requiring intervention, and ideas on how these might be prevented or suppressed. A PubMed search was performed to identify relevant studies on the obstetric considerations. Finally, a manual search of the most relevant literature was conducted. We used a value-sensitive design approach, in which the involvement of a range of stakeholders is emphasized throughout the research (5, 17). This study held expert meetings with medical and technical specialists from obstetrics, gynecology, neonatology, anesthesiology, technical medicine, industrial design, medical engineering, and patient advocates to receive recommendations for the requirements and obtain insights for developing a step-by-step transfer workflow.
Regarding the review of different transfer strategies, since there are only a few published concepts, a manual method was chosen by utilizing PubMed and Google Patents, with search terms written in different combinations of either [artificial AND (placenta OR womb OR uterus OR amnion OR amniotic sac)], [(fetal OR fetus OR preterm OR perinatal OR premature OR infant) AND (extracorporeal OR extrauterine) AND (support OR life support OR environment)]. English articles and patent records were carefully examined to determine whether a procedure describing the transfer of a fetus from a natural womb to an artificial womb was mentioned. First, titles and abstracts were examined from the literature and patent searches to select those that met the selection criteria, followed by full-text screening for the final selection. The reference lists of the included reports were also examined for other relevant articles. For each study, we extracted information on the type of system, animal model, mode of delivery, fetal/maternal medication used during delivery, and a description of the transfer stage. The selection of the studies and patents is presented in Table 1. Not all the published approaches are equal regarding success (e.g., duration of support), validation, research stage (conceptual or experimental), model (e.g., sheep, pig), gestational age, approach (LFL or LFC) and therefore may not be directly comparable or translatable to human application. However, it does provide an overview and demonstrates how knowledge on perinatal physiology is applied in an APAW context.
Much of our understanding of physiological adaptation in the neonatal transition is derived from animal research and is essential for progress in the field. However, lambs at the same developmental stage as extremely premature infants are significantly larger, pigs are too mature, and non-human primates are too small. Therefore, human trials would require additional adjustments to the APAW setup. These studies’ results and surgical approaches must be considered from the perspective of anatomical, physiological, and developmental differences.
Different terminologies of this particular extracorporeal life support for premature infants can be found in the literature: artificial womb technology, Artificial Placenta and Artificial Womb technology (APAW) or the system names EXTrauterine Environment for Neonatal Development therapy (EXTEND) (46), ex vivo uterine environment therapy (EVE) (28), biobag, and Perinatal Life Support (PLS) (5). In this review, we refer to APAW and cite published reports using either a liquid-filled chamber or a liquid-filled lung setup.
3 Discussion
Normal lung development relies on the womb's liquid environment, adequate intrathoracic space, and regulated intrabronchial pressures (47–49). When born at 24 weeks of gestational age, the preterm lung is in the late canicular stage, when alveolar and capillary development begins (50). Normal alveolar development can be disrupted by preterm pulmonary gas exchange, leading to respiratory failure. Using mechanical ventilation, high volume, and pressure can lead to pulmonary and cerebral injury (50–52). By eliminating the need for pulmonary gas exchange using an AP, retaining liquid within the airways serves a dual purpose: it may avoid fetal to neonatal transition, prevent ventilation and oxygen induced injury, and allow continued maturation of the respiratory system.
The APAW system prevents the physiological transition from fetal to neonatal physiology, normally following birth (53–55). The transition from intra- to extra-uterine life is marked by a series of large and abrupt physiological events. Predominantly triggered by lung liquid clearance and aeration, these events lead to a decrease in pulmonary vascular resistance and an increase in pulmonary blood flow. This causes a shift from fetal circulation with placental oxygenation to neonatal circulation with oxygenation through the lungs (53–55). The decrease in pulmonary vascular resistance and cord clamping initiates a sequence of changes that dramatically reorganizes the infant's cardiovascular system (56–58).
Prostaglandins from the placenta, and adenosine from the liver and placenta, may suppress fetal breathing movements. Adamson et al. observed in experiments conducted in intubated and oxygenated lambs that, when the umbilical cord is clamped, fetal sheep start continuous breathing movements and stop when the occlusion is lifted (59). In newborns, breathing suppression can be reversed by treatment with prostaglandin synthetase inhibitors such as indomethacin (58).
The precise initiation of large inspiratory efforts at birth is not fully understood. However, factors such as activation of chemoreceptors, increased PaCO2 levels, prostaglandins and prostaglandin synthetase inhibitors, loss of inhibitory factors on respiratory center activity and physical stimuli (light, temperature and handling) are thought to contribute (58, 60–67).
If the cord is clamped and fetal-placental circulation is ceased before lung aeration, a sudden 30%–50% loss of venous return from the placenta occurs, with an increase in systemic vascular resistance, as demonstrated in fetal lambs (68).
This transition to neonatal physiology in extremely premature infants occurs too early, as their organs are activated before reaching full maturity and are probably functionally and structurally immature (69). Additionally, current treatments involving mechanical ventilation, although necessary, negatively affect normal growth and development (70). Preventing the transition process and allowing the lungs to achieve full development and maturity may be accomplished by maintaining the liquid in the lungs, thereby preventing the aeration-induced cardiovascular transition (54, 56, 71).
The lungs develop as a liquid-filled organ throughout fetal life, with liquid formed by the epithelial cells of the distal airways. The liquid produced in the airways is causing an intraluminal positive pressure, which is a stimulant for lung development (47, 71). Physiological processes during the last weeks of a full-term pregnancy and the onset of spontaneous labor appear to occur simultaneously with changes in fetal and maternal hormonal balance, preparing the fetus for neonatal transition (72, 73). Normally at birth, lung liquid is replaced by air during the first breaths with the rapid movement of liquid through the epithelium into the interstitial space. Studies in rabbits demonstrated nearly complete clearance within 3–5 breaths across the epithelium, followed by absorption by lymphatic and vessels over 4–6 h (63, 74). During a preterm non-spontaneous birth, such as an emergency preterm cesarean section (CS), preparations through stress hormones (activating epithelial sodium channels and reversing the flux of liquid) and mechanical changes (liquid clearance through uterine contractions) (75) would not have begun, disrupting neonatal transition (76). This results in absent liquid clearance antenatally, leading to more lung liquid volume at birth (77). This delay could be advantageous in preventing lung aeration when transferring to an APAW system.
Full immersion in amniotic fluid enables the fetus to maintain balanced lung liquid (swallowing and absorption), supports gut maturation, and shields against external hazards such as temperature variations, sound, trauma, and pathogens. Whether immediate immersion (with no exposure to air) was performed in the included APAW studies and patents was not clearly defined for each case. In experiments of the Philadelphia group, gas was not allowed to enter the lung (78). Flake et al. developed a biobag with a sealable opening, in which the fetus could be placed after cannulation. At stable perfusion, the opening can be sealed and the biobag can be placed in the support system (20). Flake et al. mentioned that direct delivery from the natural uterus to the liquid filled chamber (LFC) would be ideal; however, more infants could qualify for the procedure if they were supported for some time before moving into the LFC (20).
In an APAW study as early as 1968 (14), respiration prevention was deemed crucial, and infants were kept submerged by an “en caul” delivery. Experiments were performed to understand fetal respiration movements when submerged in a tank and demonstrated that once the cardiovascular circuit was halted, respiratory efforts became more prominent (14). In the study by Alexander, lambs were delivered with their heads in liquid-filled polythene bags to suppress respiration (41).
Harned et al. (79) showed that introducing (amniotic) fluid in the laryngeal region in delivered lambs can result in respiratory suppression, as a correlation between the frequency of swallowing liquid and suppression of breathing was found. Lambs who were still on placental support and had no air exposure demonstrated a possible correlation. While 3-month-old lambs showed no signs of respiratory suppression (79).
Instead of immersing the infant entirely in a liquid-filled chamber, another approach is to occlude the trachea via intubation to maintain the liquid-filled-lungs (LFL). Although direct comparisons with LFC studies cannot be made; both methods can provide insights into how perinatal physiology is modulated in extracorporeal life support. The LFL approach has been demonstrated in several animal studies via CS, after which the fetus is injected with sedatives and the umbilical vessels are cannulated. Once a stable circuit exists, the fetus is fully exteriorized and an endotracheal tube is introduced (8, 24, 25). In this approach, fluid with oral chlorhexidine is supplied to the endotracheal tube (ETT) to reduce infection, which is subsequently occluded. Daily ETT insertions of additional amniotic fluid were performed to maintain a constant fluid level without pressurization (24, 25). In another study, perfluorocarbon was used instead and maintained at a set pressure in a closed system (8). Obstruction of the fetal trachea could cause overexpansion of the lung, making it necessary to allow normal fluctuations in intrabronchial pressure and liquid breathing movements to occur.
Several APAW experiments have used medication to suppress fetal (breathing) movements or general anesthesia to control breathing (8, 24, 34–26). If medication to suppress respiration were to be used in humans, it would be ethical to combine breathing suppressants with adequate sedation. Selecting appropriate sedatives and exposure duration is crucial to avoid the risks of adverse neurodevelopmental effects, gastrointestinal motility issues, and lowered arterial blood pressure (80, 81).
Other methods to suppress air-based respiration also need to be considered. In addition, it might not always be possible to have the APAW system and necessary neonatal intensive care staff members present during delivery while in the operating room. Moving the preterm infant to the neonatal ward should therefore ideally be performed while the lungs are submerged in liquid.
Potentially harmful effects of preterm lung aeration, like oxygen toxicity or termination of the fetal circulation while connected to an artificial placenta, suggest that lung aeration during the transfer procedure ought to be avoided. Normally, during the first breathing, the air is inhaled into the (surfactant-rich) mature lungs. Yet, the underdeveloped lungs of extremely premature infants often struggle to effectively aerate the lungs, necessitating some form of respiratory assistance (63). This difficulty may be attributed to factors such as the structural immaturity of the lungs, inadequate respiratory drive, impaired lung liquid clearance, muscular weakness, rib flexibility, and surfactant deficiency (82). This suggests that limited gas intake has a small effect. As oxygenation of the perinate would be ensured via the artificial placenta, the cardiovascular effects are thought to remain unchanged. More research is necessary to elucidate the underlying physiological mechanisms, understanding the associated risks and the potential reversibility by introducing lung liquid, as demonstrated in animal experiments by Gray et al. (25). The Michigan group showcased that lambs could initially receive mechanical gas ventilation, but when respiration failed (57 ± 22 min), they could successfully be transferred to breathing liquid by filling the endotracheal tube with artificial amniotic fluid (25). This raises questions about short-term air intake effects on triggering inflammatory cascades and causing lung injury, the effect on the cardiovascular circuit (e.g., the ductus arteriosus), and whether a transition to neonatal physiology has been initiated and/or whether this transition is reversible. Postmortem analysis confirmed patent ductus arteriosus, foramen ovale, and sinus venosus in all subjects (25). The specific limits of gas breathing determining if small periods of breathing gas during the canalicular phase, may cause lung injury are yet to be determined (83). More (preclinical animal) studies are needed to evaluate functional and structural lung damage, both from lung aeration and efforts to prevent it. Thus, based on theoretical considerations, preventing gas from entering the lungs completely and preserving airway liquid in an APAW context appears desirable. In current care, when at risk of preterm delivery, the mother receives corticosteroids (i.e., betamethasone) to increase lung maturation, thereby improving neonatal outcomes, such as reduction of germinal matrix and intraventricular hemorrhage (84, 85). Further research is needed to investigate and clarify the sequential cascade of effects of antenatal corticosteroids. As the aim of APAW treatment is to maintain fetal physiology, preventing pulmonary gas exchange, further research is necessary to understand whether administering corticosteroids should be standard when transferring to APAW.
1. During the transfer procedure, exposure of the perinate's airways can initiate the breathing reflex. Based on the theoretical insights, it appears logical to prevent this by maintaining liquid in the lungs of infants.
The infant leaves the warm uterus environment and enters the cold hospital room with an ambient temperature of 26°C to 27°C (86). Within several seconds after birth, the infant's temperature decreases by approximately 2°C (87). Fetal temperature is on average 0.3°C to 0.5°C higher than the maternal temperature (86), with mean measurements ranging from 37.1°C at the beginning of labor and increasing to 37.4°C after 22 h (88). A fetal temperature exceeding 41.0°C should be avoided due to risks of protein denaturation in the fetal brain (89).
Preventing hypothermia has become standard care during preterm delivery (90). Because preterm infants have a high surface-area-to-volume ratio and low subcutaneous fat content, they quickly lose body heat (91).
In nearly all APAW studies included, the temperature of the infant was kept in line with in-uterus values (ca. 39.0°C) (11). However, one of the first studies performed decided to keep the fetus at a colder temperature before cannulation to keep the oxygen consumption low (15). After perfusion was established, the infant was placed in warm liquid. Under these conditions, no respiratory movement was observed (15).
2. The liquid environment in which the perinate would be captured should be temperature-controlled to prevent the child from developing hypo- or hyperthermia (89, 91).
A crucial task during transfer is cannulation of the umbilical vessels, thus connecting the umbilical cord to the artificial placenta (11).
Sobotka et al. investigated the degree of heart rate decline upon umbilical cord occlusion in ex-utero and in-utero lambs (92). They suggested that a newborn's immediate environment substantially impacts cardiovascular responses to prenatal hypoxia. They theorized that this may be influenced by the “diving reflex”, which is a vasovagal reaction brought on by contact with water on the face (92, 93). Chamberlain showed that when a fetus is placed in liquid, and the umbilical cord is occluded, gasping movements increase, which is an effort to maintain O2 levels (14).
Maintaining standard placental blood flow is essential for adequate fetal oxygen delivery at normal saturation levels. A single 10-min occlusion of the umbilical cord causes neuronal loss in (predominantly) the hippocampus, as demonstrated in fetal sheep (94–96). Within approximately 4 min of total cord occlusion in fetal sheep, a loss of cerebral blood flow autoregulation can be expected (97).
Although no exact duration of safe cord occlusion can be determined in humans, compression or overstretching of the umbilical cord regularly occurs during normal delivery, resulting in temporary fetal bradycardia as a physiologic response to the hypoxemic state.
Recent studies used sheep models to demonstrate rapid vessel cannulation. Partridge et al. also observed unconstrained fluid breathing, and swallowing movements while ensuring a rapid cannulation setup to ensure timely oxygen delivery for brain oxygenation (3). However, it is important to note that the sheep umbilical cord anatomy, comprising two umbilical arteries and two umbilical veins, enables the support of the native placenta through the second set of vessels while shifting to AP support. In humans, the single umbilical vein does not allow continued native placental support, making studies in pig animal models closer to human translation (22). More research is needed to draw requirements for human patient umbilical vessel cannulation (98).
Studies have performed vessel catheterization in animals in utero or ex utero. In cases of exteriorization before cannulation, the animal was generally placed in a bath, bag, or kept warm. Due to the length of the umbilical cord at 24 weeks' gestational age, cannulation after delivery would need to occur in proximity to the mother.
Knowledge on cannulation strategies can be gained from existing ex utero intrapartum treatment (EXIT), such as those described for EXIT-to-extracorporeal membrane oxygenation (ECMO) procedures (99) and could aid in determining a safe sequence of cannulation. Next to avoiding fetal airway exposure to air, factors such as uterus involution, shear-stress within the utero-placental unit and umbilical cord length, may also need to be considered to determine the optimal position of the fetus during cannulation.
3. Rapid establishment of the artificial placenta circuit is required to maintain arterial pO2 and pCO2 (92).
The supply of oxygen-rich blood from the placenta to the fetus is dependent on patency of the ductus arteriosus. This vascular shunt connects the main pulmonary artery to the aorta (100, 101). This shunt must remain open during pregnancy and birth to bypass the amniotic-fluid-filled fetal lung. Fetal adaptation after delivery necessitates the emergence of spontaneous breathing and increased oxygen tension, which leads to ductus arteriosus closure (102, 103). Although the exact processes regulating ductal patency and closure are unknown, placental prostaglandins (PG) are involved (100, 101). PGE1 and PGE2 keep the ductus arteriosus open (104). Normally, at birth, when the fetus separates from the placenta, the amount of vasodilatory placental PGs in circulation decreases (103). Neonates born with a patent ductus arteriosus can therefore be administered PGSI's (such as indomethacin) (105). PGE1 was administered to lambs in a study by Partridge et al. (3), presumably to maintain patent ductus arteriosus. PGE1 and synthetic PGE1 (misoprostol) are routinely used in clinical practice to induce labor and enhance circulation in neonates with ductal-dependent cardiac lesions before surgery (104, 106). To maintain the fetal cardiovascular circuit and prevent fetal-to-neonatal transition, closure of fetal shunts (such as the ductus arteriosus) needs to be prevented (54). To confirm persistence of the fetal cardiac circulation, previous APAW studies in animals have taken echocardiographs daily, to verify the opening of the ductus arteriosus, ductus venosus, and foramen ovale (3, 4). To ensure fetal cardiac circulation, hormones (such as PGE1) could be administered within the APAW system to prevent shunt closures.
During fetal development, endogenous glucocorticoids (cortisol and corticosterone) are crucial in organ maturation, such as the brain, lungs, kidneys, liver, and thyroid (107). At term, preparation for birth, transition to neonatal physiology, and multiorgan adaptation involve increased catecholamines and cortisol release (58). Administering exogenous glucocorticoids (dexamethasone or betamethasone) to women at risk of preterm labor accelerates fetal maturation—such as stimulation of surfactant production, thereby improving newborn outcomes (108, 109). However, excessive or premature exposure of the fetus to glucocorticoids may disrupt developmental pathways, resulting in disrupted growth, impaired gas exchange, and potential harmful long-term changes in physiological function (107, 108, 110). The fetal hypothalamic–pituitary–adrenal axis is especially prone to glucocorticoid-induced changes with potential long-lasting effects (107).
Small-for-gestational-age fetuses already have high endogenous cortisol levels; therefore, exogenous antenatal corticosteroids before preterm delivery may not provide advantages (110). In growth-restricted fetuses, antenatal exposure to high levels of endogenous glucocorticoids are thought to affect the regulation of cardiovascular development. These infants rely more on the sympathetic nervous system to maintain blood pressure and redistribute cardiac output for vital organ growth, which could be further impaired by exogenous glucocorticoids (111, 112).
Similarly, the use of antenatal dexamethasone to enhance fetal maturation has been associated with reduced birth weight (108). However, antenatal corticosteroids have decreased newborn mortality without affecting morbidity in preterm small-for-gestational-age infants (110).
The impairment in adaptation observed in extremely premature infants and extending fetal physiology using APAW require further investigation to determine whether the suppression or acceleration of neonatal adaptation is appropriate.
Within the context of an APAW system and the maintenance of fetal physiology, the promotion of lung maturation would not be suitable. However, the decision to withhold or administer antenatal corticosteroids is complex given the possibility of treatment redirection to conventional care (rescue procedure) and the uncertainty surrounding the optimal timing of delivery for a preterm infant (113). If a transfer procedure reverts to standard care, preterm care must align with conventional care standards and outcomes, and therefore inform the decision on altering antenatal administrations.
Certain antenatal administrations, such as magnesium sulfate, have shown neuroprotective effects for preterm births, and it would be a reasonable question of research, if this also applies to patients in an APAW context, seen that extremely preterm infants are at increased risk of neurologic injury (114, 115).
To sum up, the application of standard-of-care antenatal pharmacological interventions should be further investigated in cases of APAW treatment.
4. Medication may be necessary before and during transfer to prevent specific physiological cascades related to neonatal physiological transitions, for their (neuro)protective effects and to support organ growth and maturation. Further investigation is required to determine the suitability of administering conventional medications, such as corticosteroids.
Another essential factor for the successful transfer of the perinate is intact umbilical and placental circulation. When blood flow in the umbilical cord is reduced the same holds for oxygen consumption, as shown in fetal lambs (116), which could lead to transfer failure and health risks to the perinate. Umbilical cord obstruction can be caused by the pressure exerted by a physician (leading to hematoma), vasospasm, and interaction with surgical tools and actions. Additionally, the occlusion of the umbilical cord stimulates breathing initiation regardless of blood gases and pH, as demonstrated in fetal sheep (61). When occluded, the pulmonary stretch receptor activity first increases and subsequently decreases to zero at approximately the same time as the breathing reflex (117).
5. The transfer procedure should avoid obstruction or damage of the umbilical cord that could lead to the occlusion of the umbilical cord's blood flow.
Ideally, transfer to the APAW system could be performed via vaginal and CS delivery. Planned CS is always performed before vaginal delivery could occur, thereby increasing the risk of preterm birth. Delaying delivery, by performing vaginal delivery in certain situations could impact survival in cases of extremely premature birth (118). Additionally, preterm birth of an infant cannot always be planned; therefore, the timing does not always allow for CS. A vaginal procedure is preferred for the mother, as additional CS delivery risks, such as incision and uterine infection, pulmonary embolism, increased blood loss, placental growth into the scar in subsequent pregnancies, and uterine rupture, can be avoided (118, 119). Nevertheless, a transfer via vaginal delivery following preterm premature rupture of membranes may affect the feasibility of potential APAW treatments. Potential beneficial effects may be found for patients with lung hypoplasia after re-immersion into an adequate amount of amniotic fluid (120). Nevertheless, research attention should be given to maintaining the sterility of the environment, especially since chorioamnionitis is responsible for triggering roughly half of preterm births (121). An association has been found between chorioamnionitis, or early onset bacterial infection, and an increased risk for germinal matrix intraventricular hemorrhage (122, 123), a risk that has already shown to be increased in ECMO treatment in these infants (124).
To increase the success rate for a perinatal transfer, and because nearly half of premature births are performed via CS, a CS transfer procedure should also be available (125–128). From a fetal perspective, CS transfer offers a more controllable environment throughout the procedure. In contrast to vaginal birth, in a CS the “labor less” placenta should function similarly as during pregnancy, provided specific anesthesia protocols are followed. Relevant surgery has been successfully applied to the human fetus in this condition, i.e., ex utero intrapartum treatment, whilst keeping the fetus on uteroplacental circulation (129).
In a broader context, the delivery mode can impact the transition stage to neonatal physiology. Certain processes involved in neonatal transition leading to spontaneous vaginal labor would be omitted if elective CS is planned before labor begins. Therefore, CS can lead to retained lung fluid and (transient) poor respiratory adaptation (58, 128). However, because the transition is impaired in extreme preterm births, a difference in the stage of neonatal transition between CS and vaginal delivery is less likely.
Previous APAW animal studies controlled umbilical vessel cannulation directly after CS while the animal was still in utero or ex utero, connected to the native placenta, before vasospasm or cord desiccation could occur (Table 1).
Through the CS and a small hysterotomy, the fetus is exposed and can be cannulated in the neck or umbilical cord. After establishing the circuit, the fetus was transferred to a warmed fluidic incubator. A similar approach was demonstrated by the Philadelphia group, where a lamb was placed directly in a biobag after delivery and cannulation, after which the biobag was sealed and transported to a mobile support station (3). They proposed the possibility of vaginal delivery by cannulating at the perineum (12). In contrast to the broader applicability of AP studies in terms of delivery modes, current EXTEND protocols continue to focus on CS (78).
One of the first approaches described for human subjects was to perform CS and keep the native amniotic sac intact, after which the fetus could be submerged in the artificial amniotic fluid in a tank. Subsequently, cannulation was performed. In case of membrane rupture, the fetus is placed in a warmed fluid on the operating table (14). In 1958, a study performed in humans reported transfers by vaginal, spontaneous, and legally induced abortions. After delivery, the umbilical cord was clamped rapidly and the infant was kept in a temperature of 25°C, as this is believed to reduce the oxygen consumption until the circuit is established. After umbilical vessel cannulation, the fetus was placed in a closed liquid-filled chamber on a perforated disc above the heating apparatus (15).
In a patent published in 2004, Cooper suggested two possible transfer methods: CS and birth canal (44). As the only detailed account describing a human obstetric procedure, we included it despite a lack of feasibility studies. A CS can be performed without rupturing the membranes, allowing for an “en caul” delivery where the fetus remains protected within the amniotic sac, subsequently placed in a net and submerged in warmed artificial amniotic fluid (44). If membrane rupture occurs, the medical staff should prevent the infant from breathing by covering the infant's mouth. This approach has also been suggested for VB transfer, with the physician's hand in the infant's mouth before placing it in a submerged net. In the case of VB, the contamination risk is a concern, which can be mitigated by cleaning the fetus through successive antibiotic and antimicrobial baths.
Infants from a multiple pregnancy could particularly benefit from improved preterm care, as they currently face a higher likelihood of being born preterm (130) and have a higher risk of adverse outcomes compared to singletons at similar gestational age (131). Factors to address in future research on this topic may include management of umbilical cord abnormalities and cannulation strategies in twins.
6. Efforts should be made to allow the transfer procedure to be available for both vaginal and CS delivery, and to allow for a multiple birth.
Vaginal delivery is not sterile, exposing the fetus to the mother's vaginal flora during birth. In addition, the uterus is not a sterile environment, as studies have shown that the acquisition and colonization of the human digestive tract begins in utero (132). In extremely preterm births, chorioamnionitis is the most common cause of preterm labor (133).
Some studies have compared vaginally delivered neonates exposed to maternal vaginal flora vs. CS-delivered neonates and argued that exposure to vaginal flora may contribute to developing the newborn immune system (134, 135). Therefore, contact with the vaginal microbiota could be explored, for example, during the birth from the APAW system (136). However, no exposure to vaginal flora should occur throughout the present process, because it is intended to maintain perinatal physiological conditions in the fetus. Exposing the perinate to vaginal flora during transfer may increase the risk of Early Onset Sepsis, which is most often acquired from the mother's genital tract or, less frequently, vertically through the placenta (137). Infection with group B Streptococcus is the leading cause of morbidity in Early Onset Sepsis (138). A vaginal swab and potential antibiotic treatment must be given as per standard practice in managing threatened preterm labor.
Although not all studies have demonstrated significant outcomes (139, 140), intrapartum intravaginal lavage can be regarded as a feasible precaution to limit the risk of newborn contamination with pathogens from the maternal genital canal (141). Specifically, chlorhexidine and povidone-iodine (PI) treatments may be beneficial (142, 143). However, data on the possible adverse effects of chlorhexidine exposure in the birth canal of neonates, particularly preterm newborns, are limited. Multiple examples of adverse skin responses to topical chlorhexidine have been reported, with extremely low birth weight neonates suffering the most serious reactions (i.e., burns) (144, 145). This was most likely caused by compromised skin integrity (146). Perinates may also swallow or inhale any residual chlorhexidine present during delivery. However, there is currently no literature on its impact on preterm newborns. A study on rats showed acute pulmonary inflammation, capillary congestion, edema, and interstitial fibrosis after gradual intratracheal administration of 0.1% and 1% chlorhexidine solutions (147). These histological changes are comparable to those that can be seen in patients who suffer from acute respiratory distress syndrome (148–150). The researchers concluded that there is a reasonable chance that inhalation of chlorhexidine at concentrations of 0.1% or greater could result in this syndrome; thus, using chlorhexidine would ideally be avoided.
PI has also been used to disinfect birth canals prior to cesarean section and vaginal delivery and showed reduced infection rates (141, 151). According to one study, infection rates following standard vaginal saline solution disinfection were comparable to those following PI disinfection (152). The same was true for vaginal cleaning using a diluted baby shampoo (153). Additionally, prolonged exposure to PI (1%–2%) during pregnancy or birth may trigger temporary thyroid dysfunction in infants and mothers (154). According to some studies, this condition may be tolerated if the newborn is well monitored after exposure. However, it may be preferable to use only non-iodine antiseptics.
Alternatively, rather than chemical protection, the use of a physical barrier, such as a retractor between the perinate and birth canal, could be investigated to prevent exposure to vaginal flora.
Multiple techniques have been proposed to reduce the perinatal contamination in liquid-filled incubators. Sakata et al. added antibiotics to lactated Ringer's solution (38). When the perinate is transferred via the birth canal, more attention should be paid so that the vaginal microflora are not taken into the incubator. Cooper suggested performing subsequent rinses in baths containing antibiotics and antimicrobial fluids (44). Antibiotic treatments (meropenem, fluconazole, and cefazolin) have also been regularly administered intravenously to lambs in liquid-filled incubators to prevent infections (4, 6, 29).
APAW studies in animals have shown that some lambs die of pulmonary inflammation (3, 6). Partridge et al. compared multiple options for fluid chamber design and demonstrated that the use of a sealable biobag instead of open or semi-open structures eliminated many problems related to fluid contamination and associated infections (3). Owing to their system's fluid-filtering capacity, the Philadelphia group suggests that any contamination could be removed after placing the infant in the system (12). Adding antimicrobial coatings to an incubator can also aid in reducing bacterial growth on the film (20, 44).
7. Ideally, exposure to vaginal flora should be prevented during the perinate transfer. We argue that a physical barrier between the fetus and birth canal will likely prevent or greatly lower fetal exposure to vaginal flora and possible lavage residues.
For the safety of the perinate, it must be possible to cease transfer to the APAW system at any time during the procedure and proceed with conventional neonatal care. Establishing specific criteria (and parameter thresholds) is essential for determining whether and when a rescue procedure is necessary. The critical phase of vital parameter monitoring is the time between the abdominal incision and attachment of the inserted cannulas to the extracorporeal circuit. This could be regarded as the transition time from fetal heart rate monitoring (CTG) to more comprehensive monitoring of the infant, since data on blood pressure, heart rate, and oxygen saturation are immediately available once a circuit is established. However, if the cannulation procedure fails or is prolonged, monitoring relies solely on inobtrusive methods (e.g., CTG) or monitoring would have to be extended to attach sensors to the fetus in utero.
A protocol that specifies the maximum duration of cannulation, identifies the key clinical parameters that indicate deterioration, and emphasizes the importance of promptly discontinuing the procedure before the onset of irreversible damage, such as decreased cerebral oxygenation, should be established. Future preclinical studies should identify all the relevant parameters and their threshold values, which could be aided by the development of a clinical decision support system (155). It is essential that these criteria are unambiguous, and that the parties responsible for making the decision to cease the transfer are clearly delineated. These may include parents, obstetricians, neonatologists, and/or technicians.
8. The primary objective of monitoring during the transfer is to indicate that everything is proceeding as expected or to indicate promptly that the rescue procedure must be started before irreversible harm occurs.
The effectiveness of prospective APAW treatment should be evaluated based on its improvement over the standard of care for an extremely premature infant in the neonatal incubator on a ventilator, not by comparison with the native womb (156). However, knowledge of the intrauterine conditions should inform APAW treatment to improve preterm care outcomes. General benefits can be gained from further research on the environmental influences that affect development (157). Birth is associated with massive tactile, visual, auditory, and vestibular sensory sensations, when the fetus leaves the warm uterus for a new but cold physical environment. Although no clear conclusions can be drawn from the available research that short-term exposure to the external environment affects perinatal development, attenuation of environmental triggers from the harsh hospital room during delivery would allow a smooth transfer from the protective environment of the native womb to the protective environment of the liquid-filled chamber (LFC). Submerging the fetus in a liquid has the benefit of protecting it from sound, balance disturbances, tactile stimuli, and trauma. Additional efforts could include protection from intense light while allowing visual examination by medical staff. For instance, Charest-Pekeski et al. covered biobags to prevent light transmission to the fetus and mimic uterine conditions more accurately (7). While detailing these requirements is beyond the scope of this review, we believe that the obstetric procedure should be in line with the attenuation of triggers offered in an LFC and therefore avoid exposure to temperature shifts, noise, and harsh directed lights.
9. Despite the transfer taking only a limited duration, it would be optimal to attenuate environmental triggers, as is aimed for in an LFC, yet allow for adequate visual examination of the perinate to make an informed decision to (dis)continue treatment.
Contributions towards health and well-being that account for the emotional impact on mothers and partners should not be overlooked. Maternal comfort could be facilitated by epidural or spinal analgesia as general anesthesia could carry more risk of maternal and neonatal complications (158–160).
In previous studies on APAW with lambs, ewes were premedicated, anesthetized, intubated, and ventilated by administering drugs including buprenorphine or propofol (3, 28). Buprenorphine, an opioid, depresses ventilation, which is beneficial for suppressing the breathing reflex of the fetus (161, 162). Another opioid that is used in clinics for maternal pain relief is pethidine, a morphinomimetic (163). This opioid also suppresses the breathing reflex in the neonate (164). In vaginal births, epidural is the preferred pain relief option, or remifentanil is used as an alternative, which crosses the placenta but is thought to be quickly metabolized. In cesarean sections, spinal anesthesia is preferred. Although fetal surgery can be performed under fetal-direct anesthesia, it is primarily reserved for pain management in critically ill patients and represents a decision between two unfavorable options. If a transfer could be performed without the use of fetal anesthetics (but with maternal anesthetics), it would be more desirable if it did not result in an unreasonable pain experience (165, 166).
10. With appropriate pain relief in place, attempts should be made to avoid general anesthesia.
Although the enclosed environment of the LFC is designed to protect the infant from external factors and sustain fetal physiology, it also prevents direct contact, or potential bonding, between parent and infant. Studies have shown a correlation between postnatal attachment and maternal and newborn outcomes (167). High levels of bonding are related to greater adjustment to the parental role from the mother, and are positively correlated with social, cognitive, and physical development in the newborn (167–170). Insufficient bonding in extremely premature infants can negatively affect hormonal, epigenetic, and neuronal development (170).
Upon delivery, the mother undergoes significant endocrine changes triggered by the detachment of the placenta (171, 172). These hormonal fluctuations, such as a rapid decline in the concentrations of estradiol and progesterone after birth, could contribute to depression, anxiety, or increased stress levels (172, 173). Preterm delivery can turn into a traumatic experience due to fear, helplessness, pain, and loss of control (174, 175). Quality of care, privacy, engagement in decision-making, and support significantly impact a woman's birth experience (176). Unplanned CS and instrumental vaginal deliveries have been associated with increased risk of negative birth experiences and maternal health issues (177, 178). Early maternal-newborn contact directly following delivery, such as seeing, holding, and feeding, has been linked to positive birth experiences for new mothers, especially those with CS (179). Situations may arise in which patients prepared for, or on APAW support do not survive, and parents may not have had the opportunity to hold or witness their infant alive. Adequate parent support guidelines, such as those that exist for stillbirth, should be in place.
Additional research should confirm the direct connection between negative birth experiences and subsequent poor maternal caregiving (180). In any case, adequate obstetric and family centered care policies are imperative to enhance patient well-being.
Regarding birth experience, the LFL approach would be more similar to the current neonatal intensive care, and skin-to-skin contact between parents and infants would still be possible (181). Further (long-term) research will elucidate which configuration is the safest and most preferable for patients.
11. Parent-infant bonding (either through direct contact or technology-enhanced bonding) is advisable, as it could enhance the birth experience and, thereby, parental and fetal well-being.
Finally, integrating treatment using APAW into the current healthcare system will likely demand increased human and material resources. Personnel setups required for novel treatments, such as transfer procedures, require specialized training and may easily exceed staffing availability. For example, the health workforce requirements in German perinatal centers demand the presence of 2 gynecologists, one neonatologist (present or on-call), one fellow pediatrician, one anesthesiologist, one scrub nurse, one surgical technical assistant, and one medical assistant (182). Within the context of a transfer procedure, to cover delivery, cannulation, and placement in the LFC, the majority of this team would likely be needed, thereby preventing them from caring for other patients. This makes treatment with this technology highly specialized, expensive, and inaccessible to many patients, as low- and middle-income countries are already struggling with staffing shortages for newborn care (183). Centralizing care may initially be necessary if this specialized treatment is brought to the clinic, requiring the accumulation of experience and expertise, along with the need to rearrange the NICU ward and purchase of medical equipment.
12. Human and logistical resources should be considered to integrate treatments using APAW within the existing healthcare system.
Research suggests that medical simulation may improve obstetric team performance and maternal and perinatal outcomes (184). Medical simulation has also been proposed within an APAW context to complement the development of procedures tailored to human patients (5, 155, 185). Although in silico studies should ultimately be followed by validation in vivo, the emergence of novel simulation technologies could offer an intermediary step that allows for a controlled and safe environment for procedural development and training. A large body of literature has been written about the ethical development of APAW technology (5), underscoring the crucial role of dialogue and stakeholder engagement during its development.
Figure 1 summarizes the considerations for a transfer procedure, as it relates to standard obstetric care for extremely premature infants, and lists points for future research that focus on obstetric approaches in an APAW context. Future research on the obstetric approaches for APAW treatment is here divided into three stages surrounding preterm care: pre-, during and post-delivery. Apart from obstetric care, prompt initiation of neonatal intensive care monitoring and treatment at cannulation would be crucial for continuous adjustment of gas exchange, nutrition, medical treatment, stabilization of the circulation, countering extracorporeal circulation side-effects, obtaining blood samples for various tests, and initiating infection control if necessary.
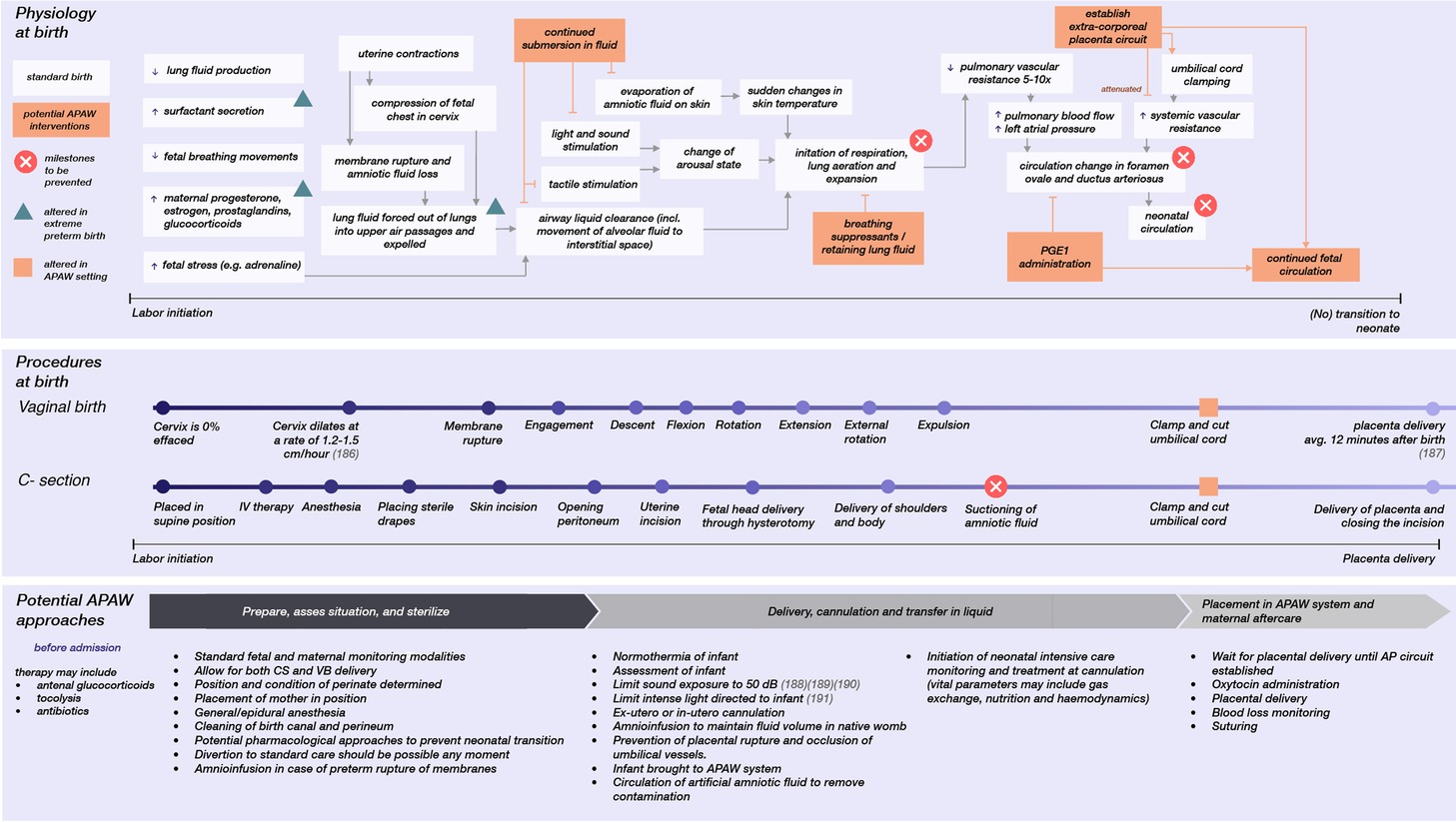
Figure 1. The physiology involved at birth that results in neonatal transition, the different modes of delivery, and potential obstetric approaches for a transfer to an APAW system. Each has a relative timescale (186–191).
4 Conclusion
This narrative review covers the transitional physiology in an APAW context and how it relates to different obstetric approaches and perinatal physiological needs. The majority of APAW studies included in this review, conducted on animals, have been essential in providing valuable insights; however, their applicability in humans remains uncertain. The feasibility of transitioning from placental to extracorporeal circuit support with conventional obstetric care and the associated requirements, such as safety thresholds, remain unclear.
This review aims to outline future research directions and lists considerations for a safe obstetric procedure to mitigate potential risks. This can be achieved by promoting continuous intrauterine conditions through adaptation of conventional preterm delivery. Considerations include preserving fluid in the airways, maintaining sterility, normothermia, anesthetic management, attenuating external stimuli (e.g., light and sound), and ensuring psychological aspects such as patient well-being. Certain requirements may become superfluous as research advances.
Compared to the present preterm care, one challenge associated with the potential clinical use of AW is the necessity of CS—as seen in all successful AW models. Clinical translation may require an EXIT procedure or a modified CS. Vasospasm and reduced placental perfusion at the time of initial vessel cannulation could favor CS delivery over VB, especially in cases of prolonged labor. Still, allowing both vaginal and CS deliveries could benefit maternal outcomes if measures to limit vasospasms, prevent neonatal transition and the promotion of sterility are ensured.
Preclinical studies have shown successful maintenance of APAW support and many learnings can be drawn from its animal studies. As there are still missing links in APAW research that need to be resolved before clinical use (192), animal models can also aid in determining the physiological factors involved in neonatal transition and how they are modulated during extracorporeal life support.
Medical simulation can be complementary to reach this aim, as these can seamlessly integrate a value-sensitive approach to identify and implement patient-centered care and ethical considerations in APAW development, ensuring optimal alignment with the needs and values of stakeholders (5, 17).
In this review, we aimed to provide a comprehensive overview of factors that might come into play in the obstetric care of future treatment using APAW technology and provide suggestions for how these factors might be addressed. Building a collective understanding of essential transitional physiology and obstetric practices informs technological development and can enhance the readiness of a medical team in anticipation of potential future clinical trials.
Author contributions
JvH: Conceptualization, Data curation, Methodology, Visualization, Writing – original draft, Writing – review & editing, Investigation. FD: Conceptualization, Writing – review & editing. MS: Writing – review & editing. AtP: Writing – review & editing. JvL: Writing – review & editing. SO: Writing – review & editing. MvdH: Conceptualization, Writing – review & editing.
Funding
The author(s) declare financial support was received for the research, authorship, and/or publication of this article.
This work was supported by the Project “Perinatal Life Support System: Integration of Enabling Technologies for Clinical Translation” Under Horizon 2020 FET Open Grant EU863087.
Conflict of interest
The authors JvH, FD, SO have a patent application entitled “Transfer of a preterm baby from a natural womb” (no. WO2023027584A1). The authors JvH, FD, MvH, SO have a patent application entitled “Transfer of a preterm baby from a natural womb” (no. WO2022066014).
The remaining authors declare that the research was conducted in the absence of any commercial or financial relationships that could be construed as a potential conflict of interest.
Publisher's note
All claims expressed in this article are solely those of the authors and do not necessarily represent those of their affiliated organizations, or those of the publisher, the editors and the reviewers. Any product that may be evaluated in this article, or claim that may be made by its manufacturer, is not guaranteed or endorsed by the publisher.
References
1. Patel RM. Short- and long-term outcomes for extremely preterm infants. Am J Perinatol. (2016) 33(3):318–28. doi: 10.1055/s-0035-1571202
2. Morgan AS, Mendonca M, Thiele N, David AL. Management and outcomes of extreme preterm birth. BMJ. (2022) 376. doi: 10.1136/bmj-2021-055924
3. Partridge EA, Davey MG, Hornick MA, McGovern PE, Mejaddam AY, Vrecenak JD, et al. An extra-uterine system to physiologically support the extreme premature lamb. Nat Commun. (2017) 8. doi: 10.1038/ncomms15112
4. Usuda H, Watanabe S, Miura Y, Saito M, Musk GC, Rittenschober-Böhm J, et al. Successful maintenance of key physiological parameters in preterm lambs treated with ex vivo uterine environment therapy for a period of 1 week. Am J Obstet Gynecol. (2017) 217(4):457.e1–457.e13. doi: 10.1016/j.ajog.2017.05.046
5. van der Hout-van der Jagt MB, Verweij EJT, Andriessen P, de Boode WP, Bos AF, Delbressine FLM, et al. Interprofessional consensus regarding design requirements for liquid-based perinatal life support (PLS) technology. Front Pediatr. (2022) 9. doi: 10.3389/fped.2021.793531
6. Usuda H, Watanabe S, Saito M, Ikeda H, Koshinami S, Sato S, et al. Successful use of an artificial placenta–based life support system to treat extremely preterm ovine fetuses compromised by intrauterine inflammation. Am J Obstet Gynecol. (2020) 223(5):755.e1–755.e20. doi: 10.1016/j.ajog.2020.04.036
7. Charest-Pekeski AJ, Cho SKS, Aujla T, Sun L, Floh AA, McVey MJ, et al. Impact of the addition of a centrifugal pump in a preterm miniature pig model of the artificial placenta. Front Physiol. (2022) 13. doi: 10.3389/fphys.2022.925772
8. Kading JC, Langley MW, Lautner G, Jeakle MMP, Toomasian JM, Fegan TL, et al. Tidal flow perfusion for the artificial placenta: a paradigm shift. ASAIO J. (2020) 66(7):796–802. doi: 10.1097/MAT.0000000000001077
9. Eixarch E, Illa M, Fucho R, Rezaei K, Hawkins-Villarreal A, Bobillo-Pérez S, et al. An artificial placenta experimental system in sheep: critical issues for successful transition and survival up to one week. Biomedicines. (2023) 11(3):702. doi: 10.3390/biomedicines11030702
10. Schoberer M, Arens J, Lohr A, Seehase M, Jellema RK, Collins JJ, et al. Fifty years of work on the artificial placenta: milestones in the history of extracorporeal support of the premature newborn. Artif Organs. (2012) 36(6):512–6. doi: 10.1111/j.1525-1594.2011.01404.x
11. De Bie FR, Davey MG, Larson AC, Deprest J, Flake AW. Artificial placenta and womb technology: past, current, and future challenges towards clinical translation. Prenat Diagn. (2021) 41(1):145–58. doi: 10.1002/pd.5821
12. Flake AW. A supportive physiologic environment for the extreme premature infant: improving life outside the womb. J Pediatr Surg. (2022) 57(2):167–71. doi: 10.1016/j.jpedsurg.2021.10.025
13. Kozlov M. Human trials of artificial wombs could start soon. Here’s what you need to know. Nature. (2023) 621:458–60. doi: 10.1038/d41586-023-02901-1
14. Chamberlain G. An artificial placenta: the development of an extracorporeal system for maintenance of immature infants with respiratory problems. Am J Obstet Gynecol. (1968) 100(5):615–26. doi: 10.1016/S0002-9378(15)33387-1
15. Westin B, Nyberg R, Enhorning G. A technique for perfusion of the previable human fetus. Acta Paediatr. (1958) 47(4):339–49. doi: 10.1111/j.1651-2227.1958.tb07643.x
16. Leenaars CHC, Kouwenaar C, Stafleu FR, Bleich A, Ritskes-Hoitinga M, De Vries RBM, et al. Animal to human translation: a systematic scoping review of reported concordance rates. J Transl Med. (2019) 17. doi: 10.1186/s12967-019-1976-2
17. Verweij EJ, De Proost L, van Laar JOEH, Frank L, Obermann-Borstn SA, Vermeulen MJ, et al. Ethical development of artificial amniotic sac and placenta technology: a roadmap. Front Pediatr. (2021) 9. doi: 10.3389/fped.2021.793308
18. Hornick MA, Mejaddam AY, McGovern PE, Hwang G, Han J, Peranteau WH, et al. Technical feasibility of umbilical cannulation in midgestation lambs supported by the EXTra-uterine environment for neonatal development (EXTEND). Artif Organs. (2019) 43(12):1154–61. doi: 10.1111/aor.13524
19. Hornick MA, Davey MG, Partridge EA, Mejaddam AY, McGovern PE, Olive AM, et al. Umbilical cannulation optimizes circuit flows in premature lambs supported by the EXTra-uterine environment for neonatal development (EXTEND). J Physiol. (2018) 596(9):1575–85. doi: 10.1113/JP275367
20. Flake A, Marcus D. Method and Apparatus for Extracorporeal Support of Premature Fetus. US (US20210161744A1). U.S. Patent and Trademark Office, Alexandria, VA: World Intellectual Property Organization. W O 2016/205622 A1 (2020).
21. Charest-Pekeski AJ, Sheta A, Taniguchi L, McVey MJ, Floh A, Sun L, et al. Achieving sustained extrauterine life: challenges of an artificial placenta in fetal pigs as a model of the preterm human fetus. Physiol Rep. (2021) 9(5). doi: 10.14814/phy2.14742
22. Darby JRT, Berry MJ, Quinn M, Holman SL, Bradshaw EL, Jesse SM, et al. Haemodynamics and cerebral oxygenation of neonatal piglets in the immediate ex utero period supported by mechanical ventilation or ex utero oxygenator. J Physiol. (2021) 599(10):2751–61. doi: 10.1113/JP280803
23. McLeod JS, Church JT, Coughlin MA, Carr B, Poling C, Sarosi E, et al. Splenic development and injury in premature lambs supported by the artificial placenta. J Pediatr Surg. (2019) 54(6):1147–52. doi: 10.1016/j.jpedsurg.2019.02.041
24. Bryner B, Gray B, Perkins E, Davis R, Hoffman H, Barks J, et al. An extracorporeal artificial placenta supports extremely premature lambs for 1 week. J Pediatr Surg. (2015) 50(1):44–9. doi: 10.1016/j.jpedsurg.2014.10.028
25. Gray BW, El-Sabbagh A, Zakem SJ, Koch KL, Rojas-Pena A, Owens GE, et al. Development of an artificial placenta V: 70 h veno-venous extracorporeal life support after ventilatory failure in premature lambs. J Pediatr Surg. (2013) 48(1):145–53. doi: 10.1016/j.jpedsurg.2012.10.030
26. Gray BW, El-Sabagh A, Rojas-Pena A, Kim AC, Gadepali S, Koch KL, et al. Development of an artificial placenta IV: 24 h venovenous extracorporeal life support in premature lambs. ASAIO J. (2012) 58(2):148–54. doi: 10.1097/MAT.0b013e3182436817
27. Reoma JL, Rojas A, Kim AC, Khouri JS, Boothman E, Brown K, et al. Development of an artificial placenta I: pumpless arterio-venous extracorporeal life support in a neonatal sheep model. J Pediatr Surg. (2009) 44(1):53–9. doi: 10.1016/j.jpedsurg.2008.10.009
28. Usuda H, Watanabe S, Saito M, Sato S, Musk GC, Fee ME, et al. Successful use of an artificial placenta to support extremely preterm ovine fetuses at the border of viability. Am J Obstet Gynecol. (2019) 221(1):69.e1–69.e17. doi: 10.1016/j.ajog.2019.03.001
29. Miura Y, Usuda H, Watanabe S, Woodward E, Saito M, Musk GC, et al. Stable control of physiological parameters, but not infection, in preterm lambs maintained on ex vivo uterine environment therapy. Artif Organs. (2017) 41(10):959–68. doi: 10.1111/aor.12974
30. Miura Y, Matsuda T, Usuda H, Watanabe S, Kitanishi R, Saito M, et al. A parallelized pumpless artificial placenta system significantly prolonged survival time in a preterm lamb model. Artif Organs. (2016) 40(5):E61–8. doi: 10.1111/aor.12656
31. Miura Y, Saito M, Usuda H, Woodward E, Rittenschober-Böhm J, Kannan PS, et al. Ex-vivo uterine environment (EVE) therapy induced limited fetal inflammation in a premature lamb model. PLoS One. (2015) 10(10):1–17. doi: 10.1371/journal.pone.0140701
32. Miura Y, Matsuda T, Funakubo A, Watanabe S, Kitanishi R, Saito M, et al. Novel modification of an artificial placenta: pumpless arteriovenous extracorporeal life support in a premature lamb model. Pediatr Res. (2012) 72(5):490–4. doi: 10.1038/pr.2012.108
33. Pak SC, Song CH, So GY, Jang CH, Lee KH, Kim JY. Extrauterine incubation of fetal goats applying the extracorporeal membrane oxygenation via umbilical artery and vein. J Korean Med Sci. (2002) 17(5):663–8. doi: 10.3346/jkms.2002.17.5.663
34. Unno N, Kuwabara Y, Okai T, Kido K, Nakayama H, Kikuchi A, et al. Development of an artificial placenta: survival of isolated goat fetuses for three weeks with umbilical arteriovenous extracorporeal membrane oxygenation. Artif Organs. (1993) 17(12):996–1003. doi: 10.1111/j.1525-1594.1993.tb03181.x
35. Unno N, Kuwabara Y, Shinozuka N, Akiba K, Okai T, Kozuma S, et al. Development of artificial placenta: oxygen metabolism of isolated goat fetuses with umbilical arteriovenous extracorporeal membrane oxygenation. Fetal Diagn Ther. (1990). 5(3–4):189–95. doi: 10.1159/000263592
36. Kuwabara Y, Okai T, Kozuma S, Unno N, Akiba K, Shinozuka N, et al. Artificial placenta: long-term extrauterine incubation of isolated goat fetuses. Artif Organs. (1989) 13(6):527–31. doi: 10.1111/j.1525-1594.1989.tb01574.x
37. Kuwabara Y, Okai T, Imanishi Y, Muronosono E, Kozuma S, Takeda S, et al. Development of extrauterine fetal incubation system using extracorporeal membrane oxygenator. Artif Organs. (1987) 11(3):224–7. doi: 10.1111/j.1525-1594.1987.tb02663.x
38. Sakata M, Hisano K, Okada M, Yasufuku M. A new artificial placenta with a centrifugal pump: long-term total extrauterine support of goat fetuses. J Thoracic Cardiovasc Surg. (1998) 115(5):1023–31. doi: 10.1016/S0022-5223(98)70401-5
39. Zapol WM, Kolobow T, Pierce JE, Vurek GG, Bowman RL. Artificial placenta: two days of total extrauterine support of the isolated premature lamb fetus. Science. (1969) 166(3905):617–8. doi: 10.1126/science.166.3905.617
40. Alexander DP, Britton HG, Nixon DA. Maintenance of sheep fetuses by an extracorporeal circuit for periods up to 24 h. Am J Obstet Gynecol. (1968) 102(7):969–75. doi: 10.1016/0002-9378(68)90457-2
41. Alexander P, Britton HG, Nixon DA. Survival of the foetal sheep at term following short periods of perfusion through the umbilical vessels. J Physiol. (1964) 175:113–24. doi: 10.1113/jphysiol.1964.sp007506
42. Lawn L, Mccance RA. Ventures with an artificial placenta—i. Principles and preliminary results. Proc R Soc Lond B Biol Sci. (1962) 155(961):500–9. doi: 10.1098/rspb.1962.0015
43. Tchirikov M. Artificial Womb System and Placenta. International Patent Office; WO 2018/171905 AI. (2018).
44. Cooper W. Conceptus Chamber. Vol. 1. USA: United States Patent Office; US 2004/0193096 A1, 2004 [cited 2023 Nov 6]. Available at: https://patents.google.com/patent/US5218958A/en
45. Greenberg EM. Artificial uterus. Vol. 99. USA: US Patent Office; 2,723,660. (1955). Available at: https://patents.google.com/patent/US2723660A/en (cited June 30, 2023).
46. Partridge EA, Davey MG, Hornick MA, Flake AW. An EXTrauterine environment for neonatal development: EXTENDING fetal physiology beyond the womb. Semin Fetal Neonatal Med. (2017) 22:404–9. doi: 10.1016/j.siny.2017.04.006
47. Hooper SB, Han VKM, Harding R. Changes in lung expansion alter pulmonary DNA synthesis and IGF-II gene expression in fetal sheep. Am J Physiol. (1993) 265(4 Pt 1):L403–9. doi: 10.1152/ajplung.1993.265.4.L403
48. Kitterman JA. Physiological factors in fetal lung growth. Can J Physiol Pharmacol. (1988) 66:122–3. doi: 10.1139/y88-184
49. Kotecha S. Lung growth for beginners. Paediatr Respir Rev. (2000) 1(4):308–13. doi: 10.1053/prrv.2000.0069
50. Coalson JJ. Pathology of new bronchopulmonary dysplasia. Semin Neonatol. (2003) 8:73–81. doi: 10.1016/S1084-2756(02)00193-8
51. Polglase GR, Miller SL, Barton SK, Kluckow M, Gill AW, Hooper SB, et al. Respiratory support for premature neonates in the delivery room: effects on cardiovascular function and the development of brain injury. Pediatr Res. (2014) 75:682–8. doi: 10.1038/pr.2014.40
52. Björklund LJ, Ingimarsson J, Curstedt T, John J, Robertson B, Werner O, et al. Manual ventilation with a few large breaths at birth compromises the therapeutic effect of subsequent surfactant replacement in immature lambs. Pediatr Res. (1997) 42:348–55. doi: 10.1203/00006450-199709000-00016
53. Tan CMJ, Lewandowski AJ. The transitional heart: from early embryonic and fetal development to neonatal life. Fetal Diagn Ther. (2020) 47(5):373–86. doi: 10.1159/000501906
54. Morton SU, Brodsky D. Fetal physiology and the transition to extrauterine life. Clin Perinatol. (2016) 43(3):395–407. doi: 10.1016/j.clp.2016.04.001
55. Finnemore A, Groves A. Physiology of the fetal and transitional circulation. Semin Fetal Neonatal Med. (2015) 20(4):210–6. doi: 10.1016/j.siny.2015.04.003
56. Hooper SB, Te Pas AB, Lang J, Van Vonderen JJ, Roehr CC, Kluckow M, et al. Cardiovascular transition at birth: a physiological sequence. Pediatr Res. (2015) 77(5):608–14. doi: 10.1038/pr.2015.21
57. Hooper SB, Roberts C, Dekker J, te Pas AB. Issues in cardiopulmonary transition at birth. Semin Fetal Neonatal Med. (2019) 24(6):101033. doi: 10.1016/j.siny.2019.101033
58. Hillman NH, Kallapur SG, Jobe AH. Physiology of transition from intrauterine to extrauterine life. Clin Perinatol. (2012) 39(4):769–83. doi: 10.1016/j.clp.2012.09.009
59. Adamson SL, Richardson BS, Homan J. Initiation of pulmonary gas exchange by fetal sheep in utero. J Appl Physiol. (1987) 62(3):989–98. doi: 10.1152/jappl.1987.62.3.989
60. Alvaro RE, Rigatto H. Control of breathing in newborns. In: Buonocore G, Bracci R, Weindling M, editors. Neonatology. Cham: Springer (2018). doi: 10.1007/978-3-319-29489-6_198
61. Adamson SL. Regulation of breathing at birth. J Dev Physiol. (1991) 15(1):45–52. PMID: 1908494.1908494
62. Dekker J, van Kaam AH, Roehr CC, Flemmer AW, Foglia EE, Hooper SB, et al. Stimulating and maintaining spontaneous breathing during transition of preterm infants. Pediatr Res. (2019) 90:722–30. doi: 10.1038/s41390-019-0468-7
63. Hooper SB, Te Pas AB, Kitchen MJ. Respiratory transition in the newborn: a three-phase process. Arch Dis Child Fetal Neonatal Ed. (2016) 101(3):F266–71. doi: 10.1136/archdischild-2013-305704
64. te Pas AB, Davis PG, Hooper SB, Morley CJ. From liquid to air: breathing after birth. J Pediatr. (2008) 152(5):607–11. doi: 10.1016/j.jpeds.2007.10.041
65. te Pas AB, Hooper S. Initiation of breathing at birth. In: Jobe AH, Whitsett JA, Abman SH, editors. Fetal and Neonatal Lung Development: Clinical Correlates and Technologies for the Future. New York: Cambridge University Press (2016). p. 164–86.
66. Ronca AE, Abel RA, Alberts JR. Perinatal stimulation and adaptation of the neonate. Acta Paediatr Supp. (1996) 85(416):8–15. doi: 10.1111/j.1651-2227.1996.tb14271.x
67. Purves MJ. Onset of respiration at birth. Arch Dis Child. (1974) 49:333–43. doi: 10.1136/adc.49.5.333
68. Bhatt S, Alison BJ, Wallace EM, Crossley KJ, Gill AW, Kluckow M, et al. Delaying cord clamping until ventilation onset improves cardiovascular function at birth in preterm lambs. J Physiol. (2013) 591(8):2113–26. doi: 10.1113/jphysiol.2012.250084
69. Bensley JG, De Matteo R, Harding R, Black MJ. The effects of preterm birth and its antecedents on the cardiovascular system. Acta Obstet Gynecol Scand. (2016) 95:652–63. doi: 10.1111/aogs.12880
70. Sehgal A, Ruoss JL, Stanford AH, Lakshminrusimha S, McNamara PJ. Hemodynamic consequences of respiratory interventions in preterm infants. J Perinatol. (2022) 42:1153–60. doi: 10.1038/s41372-022-01422-5
71. Hooper SB, Harding R. Fetal lung liquid: a major determinant of the growth and functional development of the fetal lung. Clin Exp Pharmacol Physiol. (1995) 22(4):235–41. doi: 10.1111/j.1440-1681.1995.tb01988.x
72. Jain L, Eaton DC. Physiology of fetal lung fluid clearance and the effect of labor. Semin Perinatol. (2006) 30(1):34–43. doi: 10.1053/j.semperi.2006.01.006
73. Soma-Pillay P, Nelson-Piercy C, Tolppanen H, Mebazaa A. Physiological changes in pregnancy. Cardiovasc J Afr. (2016) 27(2):89–94. doi: 10.5830/CVJA-2016-021
74. Hooper SB, Kitchen MJ, Wallace MJ, Yagi N, Uesugi K, Morgan MJ, et al. Imaging lung aeration and lung liquid clearance at birth. FASEB J. (2007) 21(12):3329–37. doi: 10.1096/fj.07-8208com
75. Van Vonderen JJ, Roest AAW, Siew ML, Walther FJ, Hooper SB, Te Pas AB. Measuring physiological changes during the transition to life after birth. Neonatology. (2014) 105(3):230–42. doi: 10.1159/000356704
76. Jain L, Dudell GG. Respiratory transition in infants delivered by cesarean section. Semin Perinatol. (2006) 30(5):296–304. doi: 10.1053/j.semperi.2006.07.011
77. Houeijeh A, Tourneux P, Mur S, Aubry E, Viard R, Sharma D, et al. Lung liquid clearance in preterm lambs assessed by magnetic resonance imaging. Pediatr Res. (2017) 82(1):114–21. doi: 10.1038/pr.2017.31
78. Flake AW, De Bie FR, Munson DA, Feudtner C. The artificial placenta and EXTEND technologies: one of these things is not like the other. J Perinatol. (2023) 43(11):1343–8. doi: 10.1038/s41372-023-01716-2
79. Harned HS, Myracle J, Ferreiro J. Respiratory suppression and swallowing from introduction of fluids into the laryngeal region of the lamb. Pediat Res. (1978) 12:1003–9. doi: 10.1203/00006450-197810000-00011
80. Puia-Dumitrescu M, Comstock BA, Li S, Heagerty PJ, Perez KM, Law JB, et al. Assessment of 2-year neurodevelopmental outcomes in extremely preterm infants receiving opioids and benzodiazepines. JAMA Netw Open. (2021) 4(7). doi: 10.1001/jamanetworkopen.2021.15998
81. McPherson C, Ortinau CM, Vesoulis Z. Practical approaches to sedation and analgesia in the newborn. J Perinatol. (2021) 41:383–95. doi: 10.1038/s41372-020-00878-7
82. te Pas AB, Wong C, Kamlin COF, Dawson JA, Morley CJ, Davis PG. Breathing patterns in preterm and term infants immediately after birth. Pediatr Res. (2009) 65(3):352–6. doi: 10.1203/PDR.0b013e318193f117
83. Jobe AJ. The new BPD: an arrest of lung development. Pediatr Res. (1999) 46:641–3. doi: 10.1203/00006450-199912000-00007
84. Vafaei H, Kaveh Baghbahadorani F, Asadi N, Kasraeian M, Faraji A, Roozmeh S, et al. The impact of betamethasone on fetal pulmonary, umbilical and middle cerebral artery Doppler velocimetry and its relationship with neonatal respiratory distress syndrome. BMC Pregnancy Childbirth. (2021) 21(1). doi: 10.1186/s12884-021-03655-2
85. Roberts D, Brown J, Medley N, Dalziel SR. Antenatal corticosteroids for accelerating fetal lung maturation for women at risk of preterm birth. Cochrane Database Syst Rev. (2017) 2017. doi: 10.1002/14651858.CD004454.pub3
86. Asakura H. Fetal and neonatal thermoregulation. J Nippon Med Sch. (2004) 71(6):360–70. doi: 10.1272/jnms.71.360
87. Lubkowska A, Szymański S, Chudecka M. Surface body temperature of full-term healthy newborns immediately after birth—pilot study. Int J Environ Res Public Health. (2019) 16(8). doi: 10.3390/ijerph16081312
88. Schouten FD, Wolf H, Smit BJ, Bekedam DJ, De Vos R, Wahlen I. Maternal temperature during labour. BJOG. (2008) 115(9):1131–7. doi: 10.1111/j.1471-0528.2008.01781.x
89. Lavesson T, Källén K, Olofsson P. Fetal and maternal temperatures during labor and delivery: a prospective descriptive study. J Matern Fetal Neonatal Med. (2018) 31(12):1533–41. doi: 10.1080/14767058.2017.1319928
90. Mccall EM, Alderdice F, Halliday HL, Vohra S, Johnston L. Interventions to prevent hypothermia at birth in preterm and/or low birth weight infants. Cochrane Database Syst Rev. (2018) 2018. doi: 10.1002/14651858.CD004210.pub5
91. Mank A, Van Zanten HA, Meyer MP, Pauws S, Lopriore E, Te Pas AB. Hypothermia in preterm infants in the first hours after birth: occurrence, course and risk factors. PLoS One. (2016) 11(11):1–8. doi: 10.1371/journal.pone.0164817
92. Sobotka KS, Morley C, Ong T, Polglase GR, Aridas JDS, Miller SL, et al. Circulatory responses to asphyxia differ if the asphyxia occurs in utero or ex utero in near-term lambs. PLoS One. (2014) 9(11):1–5. doi: 10.1371/journal.pone.0112264
93. Gooden BA. Mechanism of the human diving response. Integr Physiol Behav Sci. (1994) 29(1):6–16. doi: 10.1007/BF02691277
94. Mallard EC, Gunn AJ, Williams CE, Johnston BM, Gluckman PD. Transient umbilical cord occlusion causes hippocampal damage in the fetal sheep. Am J Obstet Gynecol. (1992) 167(5):1423–30. doi: 10.1016/S0002-9378(11)91728-1
95. Mallard EC, Williams CE, Johnston BM, Gluckman PD. Increased vulnerability to neuronal damage after umbilical cord occlusion in fetal sheep with advancing gestation. Am J Obstet Gynecol. (1994) 170(1):206–14. doi: 10.1016/S0002-9378(13)70305-3
96. Keunen H, Deutz NEP, Van Reempts JLH, Hasaart THM. Transient umbilical cord occlusion in late-gestation fetal sheep results in hippocampal damage but not in cerebral arteriovenous difference for nitrite, a stable end product of nitric oxide. J Soc Gynecol Invest. (1999) 6(3):120–6. doi: 10.1016/S1071-5576(99)00006-4
97. Lotgering FK, Bishai JM, Struijk PC, Blood AB, Hunter CJ, Power GG, et al. Ten-minute umbilical cord occlusion markedly reduces cerebral blood flow and heat production in fetal sheep. Am J Obstet Gynecol. (2003) 189(1):233–8. doi: 10.1067/mob.2003.440
98. Verrips M, van Haren JS, Oei SG, Moser A, van der Hout-van der Jagt MB. Clinical aspects of umbilical cord cannulation during transfer from the uterus to a liquid-based perinatal life support system for extremely premature infants a qualitative generic study. PLoS One. (2023) 18(12):e0290659. doi: 10.1371/journal.pone.0290659
99. Matte GS, Connor KR, Toutenel NA, Gottlieb D, Fynn-Thompson F. A modified EXIT-to-ECMO with optional reservoir circuit for use during an EXIT procedure requiring thoracic surgery. J Extra Corpor Technol. (2016) 48(1):35–8. doi: 10.1051/ject/201648035
100. Hammerman C. Patent ductus arteriosus: clinical relevance of prostaglandins and prostaglandin inhibitors in PDA pathophysiology and treatment. Clin Perinatol. (1995) 22:457. doi: 10.1016/S0095-5108(18)30293-8
101. Reese J, Paria BC, Brown N, Zhao X, Morrow JD, Dey SK. Coordinated regulation of fetal and maternal prostaglandins directs successful birth and postnatal adaptation in the mouse. PNAS. (2000) 97(17):9759–64. doi: 10.1073/pnas.97.17.9759
102. Crockett SL, Berger CD, Shelton EL, Reese J. Molecular and mechanical factors contributing to ductus arteriosus patency and closure. Congenit Heart Dis. (2019) 14(1):15–20. doi: 10.1111/chd.12714
103. Ovalı F. Molecular and mechanical mechanisms regulating ductus arteriosus closure in preterm infants. Front Pediatr. (2020) 8. doi: 10.3389/fped.2020.00516
104. Akkinapally S, Hundalani SG, Kulkarni M, Fernandes CJ, Cabrera AG, Shivanna B, et al. Prostaglandin E1 for maintaining ductal patency in neonates with ductal-dependent cardiac lesions. Cochrane Database Syst Rev. (2018) 2018. doi: 10.1002/14651858.CD011417.pub2
105. Pacifici GM. Clinical pharmacology of indomethacin in preterm infants: implications in patent ductus arteriosus closure. Pediatric Drugs. (2013) 15:363–76. doi: 10.1007/s40272-013-0031-7
106. Allen R, O’brien BM. Uses of misoprostol in obstetrics and gynecology. Rev Obstet Gynecol. (2009) 2(3):159–68. doi: 10.1007/s40272-013-0031-7
107. Moisiadis VG, Matthews SG. Glucocorticoids and fetal programming part 1: outcomes. Nat Rev Endocrinol. (2014) 10(7):391–402. doi: 10.1038/nrendo.2014.73
108. Bloom SL, Sheffield JS, Mcintire DD, Leveno KJ. Antenatal dexamethasone and decreased birth weight. Obstet Gynecol. (2001) 97:485–90. doi: 10.1016/s0029-7844(00)01206-0
109. Liggins GC. The role of cortisol in preparing the Fetus for birth. Reprod Fertil Dev. (1994) 6:141–50. doi: 10.1071/RD9940141
110. Blankenship SA, Brown KE, Simon LE, Stout MJ, Tuuli MG. Antenatal corticosteroids in preterm small-for-gestational age infants: a systematic review and meta-analysis. Am J Obstet Gynecol MFM. (2020) 2. doi: 10.1016/j.ajogmf.2020.100215
111. Morrison JL, Orgeig S. Review: antenatal glucocorticoid treatment of the growth-restricted fetus: benefit or cost? Reprod Sci. (2009) 16(6):527–38. doi: 10.1177/1933719109332821
112. Morrison JL, Botting KJ, Soo PS, McGillick E V, Hiscock J, Zhang S, et al. Antenatal steroids and the IUGR fetus: are exposure and physiological effects on the lung and cardiovascular system the same as in normally grown fetuses? J Pregnancy. (2012) 2012. doi: 10.1155/2012/839656
114. Chollat C, Sentilhes L, Marret S. Fetal neuroprotection by magnesium sulfate: from translational research to clinical application. Front Neurol. (2018) 9:247. doi: 10.3389/fneur.2018.00247
115. Volpe JJ. Brain injury in premature infants: a complex amalgam of destructive and developmental disturbances. Lancet Neurol. (2009) 8(1):110–24. doi: 10.1016/S1474-4422(08)70294-1
116. Itskovitz J, LaGamma EF, Rudolph AM. Heart rate and blood pressure responses to umbilical cord compression in fetal lambs with special reference to the mechanism of variable deceleration. Am J Obstet Gynecol. (1983) 147(4):451–7. doi: 10.1016/S0002-9378(16)32243-8
117. Ponte J, Purves MJ. Types of afferent nervous activity which may be measured in the vagus nerve of the sheep foetus. J Physiol. (1973) 229:51–76. doi: 10.1113/jphysiol.1973.sp010126
118. Bodner K, Wierrani F, Grünberger W, Bodner-Adler B. Influence of the mode of delivery on maternal and neonatal outcomes: a comparison between elective cesarean section and planned vaginal delivery in a low-risk obstetric population. Arch Gynecol Obstet. (2011) 283(6):1193–8. doi: 10.1007/s00404-010-1525-y
119. Larsson C, Matsson A, Mooe T, Söderström L, Tunón K, Nordin P. Cardiovascular complications following cesarean section and vaginal delivery: a national population-based study. J Matern Fetal Neonatal Med. (2021) 35(25):8072–9. doi: 10.1080/14767058.2021.1941851
120. Van Teeffelen S, Pajkrt E, Willekes C, Van Kuijk SMJ, Mol BWJ. Transabdominal amnioinfusion for improving fetal outcomes after oligohydramnios secondary to preterm prelabour rupture of membranes before 26 weeks. Cochrane Database Syst Rev. (2013) 2013(8). doi: 10.1002/14651858.CD009952.pub2
121. Jain VG, Willis KA, Jobe A, Ambalavanan N. Chorioamnionitis and neonatal outcomes. Pediatr Res. (2022) 91:289–96. doi: 10.1038/s41390-021-01633-0
122. Linder N, Haskin O, Levit O, Klinger G, Prince T, Naor N, et al. Risk factors for intraventricular hemorrhage in very low birth weight premature infants: a retrospective case-control study. Pediatrics. (2003) 111(5 Pt 1):e590–5. doi: 10.1542/peds.111.5.e590
123. Villamor-Martinez E, Fumagalli M, Rahim OM, Passera S, Cavallaro G, Degraeuwe P, et al. Chorioamnionitis is a risk factor for intraventricular hemorrhage in preterm infants: a systematic review and meta-analysis. Front Physiol. (2018) 9. doi: 10.3389/fphys.2018.01253
124. Wild KT, Burgos CM, Rintoul NE. Expanding neonatal ECMO criteria: when is the premature neonate too premature. Semin Fetal Neonatal Med. (2022) 27. doi: 10.1016/j.siny.2022.101403
125. Högberg U, Håkansson S, Serenius F, Holmgren PÅ. Extremely preterm cesarean delivery: a clinical study. Acta Obstet Gynecol Scand. (2006) 85(12):1442–7. doi: 10.1080/00016340600969366
126. Delnord M, Blondel B, Drewniak N, Klungsøyr K, Bolumar F, Mohangoo A, et al. Varying gestational age patterns in cesarean delivery: an international comparison. BMC Pregnancy Childbirth. (2014) 14(1). doi: 10.1186/1471-2393-14-321
127. Kuper SG, Sievert RA, Steele R, Biggio JR, Tita AT, Harper LM. Maternal and neonatal outcomes in indicated preterm births based on the intended mode of delivery. Obstet Gynecol. (2017) 130(5):1143–51. doi: 10.1097/AOG.0000000000002320
128. Lee HC, Gould JB. Survival rates and mode of delivery for vertex preterm neonates according to small- or appropriate-for-gestational-age status. Pediatrics. (2006) 118(6):e1836–44. doi: 10.1542/peds.2006-1327
129. Bouchard S, Johnson MP, Flake AW, Howell LJ, Myers LB, Adzick NS, et al. The EXIT procedure: experience and outcome in 31 cases. J Pediatr Surg. (2002) 37(3):418–26. doi: 10.1053/jpsu.2002.30839
130. Gardner MO, Goldenberg RL, Cliver SP, Tucker JM, Nelson KG, Copper RL. The origin and outcome of preterm twin pregnancies. Obstet Gynecol. (1995) 85(4):553–7. doi: 10.1016/0029-7844(94)00455-M
131. Papiernik E, Zeitlin J, Delmas D, Blondel B, Künzel W, Cuttini M, et al. Differences in outcome between twins and singletons born very preterm: results from a population-based European cohort. Hum Reprod. (2010) 25(4):1035–43. doi: 10.1093/humrep/dep430. Erratum in: Hum Reprod. (2010) 25(9):2404. PMID: 20118116.20118116
132. Perez-Muñoz ME, Arrieta MC, Ramer-Tait AE, Walter J. A critical assessment of the ’sterile womb’ and ‘in utero colonization’ hypotheses: implications for research on the pioneer infant microbiome. Microbiome. (2017) 5. doi: 10.1186/s40168-017-0268-4
133. Goldenberg RL, Culhane JF, Iams JD, Romero R. Preterm birth 1 epidemiology and causes of preterm birth. Lancet. (2008) 371:75–84. doi: 10.1016/S0140-6736(08)60074-4
134. Dzidic M, Boix-Amorós A, Selma-Royo M, Mira A, Collado M. Gut microbiota and mucosal immunity in the neonate. Med Sci. (2018) 6(3):56. doi: 10.3390/medsci6030056
135. Dunn AB, Jordan S, Baker BJ, Carlson NS. The maternal infant microbiome: considerations for labor and birth. MCN Am J Matern Child Nurs. (2017) 42(6):318–25. doi: 10.1097/NMC.0000000000000373
136. Dominguez-Bello MG, De Jesus-Laboy KM, Shen N, Cox LM, Amir A, Gonzalez A, et al. Partial restoration of the microbiota of cesarean-born infants via vaginal microbial transfer. Nat Med. (2016) 22(3):250–3. doi: 10.1038/nm.4039
137. Dong Y, Speer CP. Late-onset neonatal sepsis:recent developments. Arch Dis Childhood Fetal Neonatal Ed. (2015) 100:F257–63. doi: 10.1136/archdischild-2014-306213
138. Barcaite E, Bartusevicius A, Tameliene R, Kliucinskas M, Maleckiene L, Nadisauskiene R. Prevalence of maternal group B streptococcal colonisation in European countries. Acta Obstet Gynecol Scand. (2008) 87:260–71. doi: 10.1080/00016340801908759
139. Bell C, Hughes L, Akister T, Ramkhelawon V, Wilson A, Lissauer D. What is the result of vaginal cleansing with chlorhexidine during labour on maternal and neonatal infections? A systematic review of randomised trials with meta-analysis. BMC Pregnancy Childbirth. (2018) 18(1). doi: 10.1186/s12884-018-1754-9
140. Lumbiganon P, Thinkhamrop J, Thinkhamrop B, Tolosa JE. Vaginal chlorhexidine during labour for preventing maternal and neonatal infections (excluding group B streptococcal and HIV). Cochrane Database Syst Rev. (2014) 2014. doi: 10.1002/14651858.CD004070.pub3
141. Haas DM, Morgan S, Contreras K, Kimball S. Vaginal preparation with antiseptic solution before cesarean section for preventing postoperative infections. Cochrane Database Syst Rev. (2020) 2020. doi: 10.1002/14651858.CD007892.pub7
142. Burman LG, Fryklund B, Helgesson AM, Christensen P, Christensen K, Svenningsen NW, et al. Prevention of excess neonatal morbidity associated with group B streptococci by vaginal chlorhexidine disinfection during labour. Lancet. (1992) 340(8811):65–9. doi: 10.1016/0140-6736(92)90393-H
143. Adriaanse AH, Kollée LAA, Muytjens HL, Nijhuis JG, de Haan AFJ, Eskes TKAB. Randomized study of vaginal chlorhexidine disinfection during labor to prevent vertical transmission of group B streptococci. Eur J Obstet Gynecol. (1995) 61(2):135–41. doi: 10.1016/0301-2115(95)02134-S
144. Tamma PD, Aucott SW, Milstone AM. Chlorhexidine use in the neonatal intensive care unit: results from a national survey. Infect Control Hosp Epidemiol. (2010) 31(8):846–9. doi: 10.1086/655017
145. Neri I, Ravaioli GM, Faldella G, Capretti MG, Arcuri S, Patrizi A. Chlorhexidine-induced chemical burns in very low birth weight infants. J Pediatr. (2017) 191:262–265.e2. doi: 10.1016/j.jpeds.2017.08.002
146. Nachman RL, Esterly NB. Increased skin permeability in preterm infants. J Pediatr. (1971) 79(4):628–32. doi: 10.1016/s0022-3476(71)80311-6
147. Orito K, Hashida M, Hirata K, Kurokawa A, Shirai M, Akahori F. Effects of single intratracheal exposure to chlorhexidine gluconate on the rat lung. Drug Chem Toxicol. (2006) 29(1):1–9. doi: 10.1080/01480540500408416
148. Hite RD, Morris PE. Acute respiratory distress syndrome pharmacological treatment options in development. Drugs. (2001) 61(7):897–907. doi: 10.2165/00003495-200161070-00001
149. Agrons GA, Courtney SE, Stocker JT, Markowitz RI. Lung disease in premature neonates: radiologic-pathologic correlation. Radiographics. (2005) 25(4):1047–73. doi: 10.1148/rg.254055019
150. Crimi E, Slutsky AS. Inflammation and the acute respiratory distress syndrome. Best Pract Res Clin Anaesthesiol. (2004) 18:477–92. doi: 10.1016/j.bpa.2003.12.007
151. Saling E. A new method to decrease the risk of intrapartum infection. J Perinat Med. (1978) 6:206. doi: 10.1515/jpme.1978.6.4.206
152. Abbas AM, Shaltout AS, Ali SS, Michael A, Altraigey A, Abdelfatah LE. Povidone-iodine versus normal saline solution in preoperative vaginal cleansing before office hysteroscopy: a randomized controlled trial. Int J Reprod Contracept Obstet Gynecol. (2017) 7(1):5. doi: 10.18203/2320-1770.ijrcog20175823
153. Lewis LA, Lathi RB, Crochet P, Nezhat C. Preoperative vaginal preparation with baby shampoo compared with povidone-iodine before gynecologic procedures. J Minim Invasive Gynecol. (2007) 14(6):736–9. doi: 10.1016/j.jmig.2007.05.010
154. Meyler L. Meyler’s side effects of drugs: the international encyclopedia of adverse drug reactions and interactions. 16th edn. Amsterdam: Elsevier Science (2016). p. 875–82. doi: 10.1016/B978-0-444-53717-1.01320-2
155. van Willigen BG, van der Hout-van der Jagt MB, Huberts W, van de Vosse FN. A review study of fetal circulatory models to develop a digital twin of a fetus in a perinatal life support system. Front Pediatr. (2022) 10. doi: 10.3389/fped.2022.915846
156. De Bie FR, Kim SD, Bose SK, Nathanson P, Partridge EA, Flake AW, et al. Ethics considerations regarding artificial womb technology for the fetonate. Am J Bioeth. (2023) 23(5):67–78. doi: 10.1080/15265161.2022.2048738
157. White-Traut RC, Nelson MN, Burns K, Cunningham N. Environmental influences on the developing premature infant: theoretical issues and applications to practice. J Obstet Gynecol Neonatal Nurs. (1994) 23(5):393–401. doi: 10.1111/j.1552-6909.1994.tb01896.x
158. Ring L, Landau R, Delgado C. The current role of general anesthesia for cesarean delivery. Curr Anesthesiol Rep. (2021) 11:18–27. doi: 10.1007/s40140-021-00437-6
159. Lim G, Facco FL, Nathan N, Waters JH, Wong CA, Eltzschig HK. A review of the impact of obstetric anesthesia on maternal and neonatal outcomes. Anesthesiology. (2018) 129(1):192–215. doi: 10.1097/ALN.0000000000002182
160. Sung TY, Jee YS, You HJ, Cho CK. Comparison of the effect of general and spinal anesthesia for elective cesarean section on maternal and fetal outcomes: a retrospective cohort study. Anesth Pain Med. (2021) 16(1):49–55. doi: 10.17085/apm.20072
161. Lee MH, Yang KH, Lee CS, Lee HS, Moon SY, Hwang SIL, et al. The effect-site concentration of propofol producing respiratory depression during spinal anesthesia. Korean J Anesthesiol. (2011) 61(2):122–6. doi: 10.4097/kjae.2011.61.2.122
162. Dahan A, van Lemmen M, Jansen S, Simons P, van der Schrier R. Buprenorphine: a treatment and cause of opioid-induced respiratory depression. Br J Anaesth. (2022) 128(3):402–4. doi: 10.1016/j.bja.2021.12.001
163. Kadirogullari P, Bahat PY, Sahin B, Gonen I, Seckin KD. The effect of pethidine analgesia on labor duration and maternal-fetal outcomes. Acta Biomedica. (2021) 92(2). doi: 10.23750/abm.v92i2.10905
164. Belfrage P, Boréus LO, Hartvig P, Irestedt L, Raabe N. Neonatal depression after obstetrical analgesia with pethidine. The role of the injection-delivery time interval and of the plasma concentrations of pethidine and norpethidine. Acta Obstet Gynecol Scand. (1981) 60(1):43–9. doi: 10.3109/00016348109154108
165. Duci M, Pulvirenti R, Fascetti Leon F, Capolupo I, Veronese P, Gamba P, et al. Anesthesia for fetal operative procedures: a systematic review. Front Pain Res. (2022) 3. doi: 10.3389/fpain.2022.935427
166. Fetal Awareness Review of Research and Recommendations for Practice. United Kingdom: Royal College of Obstetricians and Gynaecologists (2010). Available at: https://www.rcog.org.uk/media/gdtnncdk/rcog-fetal-awareness-evidence-review-dec-2022.pdf
167. de Cock ESA, Henrichs J, Vreeswijk CMJM, Maas AJBM, Rijk CHAM, van Bakel HJA. Continuous feelings of love? The parental bond from pregnancy to toddlerhood. J Fam Psychol. (2016) 30(1):125–34. doi: 10.1037/fam0000138
168. Joas J, Möhler E. Maternal bonding in early infancy predicts childrens social competences in preschool age. Front Psychiatry. (2021) 12. doi: 10.3389/fpsyt.2021.687535
169. Lutkiewicz K, Bieleninik L, Cieślak M, Bidzan M. Maternal–infant bonding and its relationships with maternal depressive symptoms, stress and anxiety in the early postpartum period in a polish sample. Int J Environ Res Public Health. (2020) 17(15):1–12. doi: 10.3390/ijerph17155427
170. Kommers D, Oei G, Chen W, Feijs L, Bambang Oetomo S. Suboptimal bonding impairs hormonal, epigenetic and neuronal development in preterm infants, but these impairments can be reversed. Acta Paediatr. (2016) 105:738–51. doi: 10.1111/apa.13254
171. Asztalos EV. Supporting mothers of very preterm infants and breast milk production: a review of the role of galactogogues. Nutrients. (2018) 10. doi: 10.3390/nu10050600
172. Weigl T, Schneider N, Stein A, Felderhoff-Müser U, Schedlowski M, Engler H. Postpartal affective and endocrine differences between parents of preterm and full-term infants. Front Psychiatry. (2020) 11. doi: 10.3389/fpsyt.2020.00251
173. Skalkidou A, Hellgren C, Comasco E, Sylvén S, Sundström Poromaa I. Biological aspects of postpartum depression. Women’s Health. (2012) 8:659–72. doi: 10.2217/WHE.12.55
174. Misund AR, Nerdrum P, Diseth TH. Mental health in women experiencing preterm birth. BMC Pregnancy Childbirth. (2014) 14(1). doi: 10.1186/1471-2393-14-263
175. Fowler C, Green J, Elliott D, Petty J, Whiting L. The forgotten mothers of extremely preterm babies: a qualitative study. J Clin Nurs. (2019) 28(11–12):2124–34. doi: 10.1111/jocn.14820
176. Bell AF, Andersson E, Goding K, Vonderheid SC. The birth experience and maternal caregiving attitudes and behavior: a systematic review. Sex Reprod Healthc. (2018) 16:67–77. doi: 10.1016/j.srhc.2018.02.007
177. Döblin S, Seefeld L, Weise V, Kopp M, Knappe S, Asselmann E, et al. The impact of mode of delivery on parent-infant-bonding and the mediating role of birth experience: a comparison of mothers and fathers within the longitudinal cohort study DREAM. BMC Pregnancy Childbirth. (2023) 23(1). doi: 10.1186/s12884-023-05611-8
178. Reisz S, Brennan J, Jacobvitz D, George C. Adult attachment and birth experience: importance of a secure base and safe haven during childbirth. J Reprod Infant Psychol. (2019) 37(1):26–43. doi: 10.1080/02646838.2018.1509303
179. Brubaker LH, Paul IM, Repke JT, Kjerulff KH. Early maternal-newborn contact and positive birth experience. Birth. (2019) 46(1):42–50. doi: 10.1111/birt.12378
180. Bell AF, Rubin LH, Davis JM, Golding J, Adejumo OA, Carter CS. The birth experience and subsequent maternal caregiving attitudes and behavior: a birth cohort study. Arch Womens Ment Health. (2019) 22(5):613–20. doi: 10.1007/s00737-018-0921-3
181. Kukora SK, Mychaliska GB, Weiss EM. Ethical challenges in first-in-human trials of the artificial placenta and artificial womb: not all technologies are created equally, ethically. J Perinatol. (2023) 43(11):1337–42. doi: 10.1038/s41372-023-01713-5
182. Rossi R, Bauer NH, Becke-Jakob K, Grab D, Herting E, Kehl S, et al. Empfehlungen für die Strukturellen Voraussetzungen der Perinatologischen Versorgung in Deutschland. Germany: Arbeitsgemeinschaft der Wissenschaftlichen Medizinischen Fachgesellschaften (AWMF) (2021). Available at: https://register.awmf.org/assets/guidelines/087-001l_S2k_Empfehlungen-strukturelle-Voraussetzungen-perinatologische-Versorgung-Deutschland__2021-04_01.pdf
183. World Health Organization. WHO Recommendations for Care of the Preterm or Low-Birth-Weight Infant. Geneva, Switzerland: WHO (2022).
184. Fransen AF, van de Ven J, Banga FR, Mol BWJ, Oei SG. Multi-professional simulation-based team training in obstetric emergencies for improving patient outcomes and trainees’ performance. Cochrane Database Syst Rev. (2020) 2020(12). doi: 10.1002/14651858.CD011545.pub2
185. van Haren JS, van der Hout-van der Jagt MB, Meijer N, Monincx M, Delbressine FLM, Griffith XLG, et al. Simulation-based development: shaping clinical procedures for extra-uterine life support technology. Adv Simul. (2023) 8(1):29. doi: 10.1186/s41077-023-00267-y
186. Hutchison J, Mahdy H, Hutchison J. Stages of labor. In: StatPearls [Internet]. Treasure Island, FL: StatPearls Publishing (2024). Available online at: https://www.ncbi.nlm.nih.gov/books/NBK544290/
187. Shinar S, Shenhav M, Maslovitz S, Many A. Distribution of third-stage length and risk factors for its prolongation. Am J Perinatol. (2016) 33(10):1023–8. doi: 10.1055/s-0036-1572426
188. Philbin MK, Evans JB. Standards for the acoustic environment of the newborn ICU. J Perinatol. (2006) 26:S27–30. doi: 10.1038/sj.jp.7211585
189. Philbin MK. Recommended permissible noise criteria for occupied, newly constructed or renovated hospital nurseries. Adv Neonatal Care. (2008) 8(5):559–63. doi: 10.1097/01.ANC.0000337267.47599.80
190. Graven SN. Sound and the developing infant in the NICU: conclusions and recommendations for care. J Perinatol. (2000) 20(8 Pt 2):S88–93. doi: 10.1038/sj.jp.7200444
191. Rodríguez RG, Pattini AE. Neonatal intensive care unit lighting: update and recommendations. Arch Argent Pediatr. (2017) 115(4):361–7. doi: 10.5546/aap.2017.eng.361
Keywords: extra-uterine life support, perinatal life support, artificial womb, artificial placenta, APAW, transfer procedure, neonatal transition, fetal physiology
Citation: van Haren JS, Delbressine FLM, Schoberer M, te Pas AB, van Laar JOEH, Oei SG and van der Hout-van der Jagt MB (2024) Transferring an extremely premature infant to an extra-uterine life support system: a prospective view on the obstetric procedure. Front. Pediatr. 12:1360111. doi: 10.3389/fped.2024.1360111
Received: 22 December 2023; Accepted: 2 February 2024;
Published: 15 February 2024.
Edited by:
Sascha Meyer, Saarland University Hospital, GermanyReviewed by:
Balaji Govindaswami, California Department of Health Care Services, United StatesDavid Andrew Paul, Christiana Care Health System, United States
© 2024 van Haren, Delbressine, Schoberer, te Pas, van Laar, Oei and van der Hout-van der Jagt. This is an open-access article distributed under the terms of the Creative Commons Attribution License (CC BY). The use, distribution or reproduction in other forums is permitted, provided the original author(s) and the copyright owner(s) are credited and that the original publication in this journal is cited, in accordance with accepted academic practice. No use, distribution or reproduction is permitted which does not comply with these terms.
*Correspondence: Juliette S. van Haren ai5zLnYuaGFyZW5AdHVlLm5s