- 1Department of Neonatology, KK Women’s and Children’s Hospital, Singapore, Singapore
- 2Yong Loo Ling School of Medicine, Singapore, Singapore
- 3Lee Kong Chian School of Medicine, Singapore, Singapore
- 4Duke—NUS Medical School, Singapore, Singapore
- 5Department of Paediatric Cardiology, KK Women’s and Children’s Hospital, Singapore, Singapore
Objectives: To evaluate serial tissue Doppler cardiac imaging (TDI) in the evolution of bronchopulmonary dysplasia-associated pulmonary hypertension (BPD-PH) among extremely preterm infants.
Design: Prospective observational study.
Setting: Single-center, tertiary-level neonatal intensive care unit.
Patients: Infant born <28 weeks gestation.
Main outcome measures: Utility of TDI in the early diagnosis and prediction of BPD-PH and optimal timing for screening of BPD-PH.
Results: A total of 79 infants were included. Of them, 17 (23%) had BPD-PH. The mean gestational age was 25.9 ± 1.1 weeks, and mean birth weight was 830 ± 174 g. The BPD-PH group had a high incidence of hemodynamically significant patent ductus arteriosus (83% vs. 56%, p < 0.018), longer oxygen days (96.16 ± 68.09 vs. 59.35 ± 52.1, p < 0.008), and prolonged hospital stay (133.8 ± 45.9 vs. 106.5 ± 37.9 days, p < 0.005). The left ventricular eccentricity index (0.99 ± 0.1 vs. 1.1 ± 0.7, p < 0.01) and the ratio of acceleration time to right ventricular ejection time showed a statistically significant trend from 33 weeks (0.24 ± 0.05 vs. 0.28 ± 0.05, p < 0.05). At 33 weeks, the BPD-PH group showed prolonged isovolumetric contraction time (27.84 ± 5.5 vs. 22.77 ± 4, p < 0.001), prolonged isovolumetric relaxation time (40.3 ± 7.1 vs. 34.9 ± 5.3, p < 0.003), and abnormal myocardial performance index (0.39 ± 0.05 vs. 0.32 ± 0.03, p < 0.001). These differences persisted at 36 weeks after conceptional gestational age.
Conclusions: TDI parameters are sensitive in the early evolution of BPD-PH. Diagnostic accuracy can be increased by combining the TDI parameters with conventional echocardiographic parameters. BPD-PH can be recognizable as early as 33–34 weeks of gestation.
Introduction
Bronchopulmonary dysplasia (BPD), which is currently defined by continued oxygen/respiratory support beyond 36 weeks of gestation, continues to be the most common respiratory morbidity in surviving preterm infants (1). The development of pulmonary hypertension (PH) among infants with BPD often complicates the respiratory course in the vulnerable population. The complex interaction of abnormal vascular remodeling, arrested vascular growth, reduced vascular surface, immature repair mechanisms, reduced expression of pro-angiogenic factors, or over-expression of anti-angiogenic factors have all been linked to abnormal pulmonary vascular resistance in patients with BPD (2–4).
The incidence of BPD-PH in the premature population is approximately 17%–24% but can vary between 27% and 40% with gestation less than 25 weeks and other associated co-morbidities (5–7). There is increased mortality when BPD is complicated by PH (5, 8). The true burden of PH in BPD is unknown due to the poor sensitivity of conventional echocardiographic parameters.
The gold standard for measuring pulmonary arterial pressure is cardiac catheterization; however, this is not practical in the preterm population due to the inherent high risk of invasive nature. Two-dimensional echocardiography is most widely used as an indirect measurement of pulmonary artery pressure and diagnosis of pulmonary hypertension. Although the individual conventional parameters lack sensitivity, the combination of multiple echocardiographic parameters is thought to increase the sensitivity of diagnosis of BPD-PH (9). The proposed timeline for screening PH in BPD is 36 weeks in high-risk infants, but there is limited information on serial echocardiographic evaluation to study the evolution of PH in BPD (10, 11).
Tissue Doppler imaging (TDI) is a newer echocardiographic technique that uses Doppler principles to measure the higher amplitude and lower velocity signals from myocardial tissue motion. TDI can be used to assess myocardial systolic and diastolic function by measuring long-axis ventricular motion. TDI has been validated in various pathological conditions in preterm infants, but longitudinal studies in BPD-PH are lacking (12, 13).
The primary aim of the study was to ascertain the value of serial right ventricular (RV) functional assessment in extremely premature infants when evaluating BPD-PH. We also aimed to evaluate the clinical predictors of BPD-PH and the optimal timing of echocardiographic screening in high-risk infants.
We hypothesize that serial TDI in premature infants will enable the early detection of pulmonary hypertension and increase the sensitivity of diagnosis when coupled with conventional echocardiography.
Methodology
This is a single-center prospective observational study conducted in a large tertiary neonatal unit in Singapore. The study was initiated in September 2018 and the last participant was recruited in March 2021.
Patient cohort
Infants born at <28 weeks of gestation were recruited into the study. We excluded infants born with major congenital anomalies. Each recruited infant had their first comprehensive echocardiogram involving both conventional and TDI at 7–10 days of life (scan 1) and 4-weekly scans (scan 2: 29.4 ± 1.17 weeks, scan 3: 33.8 ± 1 weeks, scan 4: 36.7 ± 0.5 weeks) thereafter until 36 weeks of corrected age. At a postmenstrual age of 36 weeks, the presence of BPD-PH was diagnosed using the conventional echocardiographic parameters. The infant was diagnosed with BPD-PH if there was a tricuspid regurgitation (TR) jet maximum velocity >2.8 m/s or a combination of left ventricular eccentricity index of more than 1.15 and the ratio of acceleration time to RV ejection time (ACT/RVET) <0.3 (14–16). The TDI markers were then compared between BPD-no PH and BPD-PH groups at each period.
Hemodynamically significant patent ductus arteriosus (Hs PDA) was defined as size >1.6 mm, left heart dilatation, reversal of flow in descending aorta, clinical signs of congestive heart failure, and needing treatment. The severity of BPD was defined as per the National Institute of Child Health and Human Development (NICHD) criteria (17). Severity of retinopathy of prematurity (ROP) was defined as per the International Classification of Retinopathy of Prematurity.
Clinical data
Baseline clinical parameters were collected including maternal antenatal details and infants’ clinical and respiratory characteristics.
Echocardiography
A Vivo S6 machine (GE Medical, Milwaukee, WI, USA) with 7 and 10 MHz probes was used for the cardiac scans. All study measurements were averaged for three to five cardiac cycles. The echocardiogram scans were performed by two senior cardiac technologists and all parameters were analyzed by a single study member (KG). The image acquisition and TDI measurements were standardized by a single senior pediatric cardiologist (SS). The initial standardization of scans and measurements was performed on six well preterm infants without any respiratory support before the study commencement. The calculated interclass correlation coefficient (ICC) between the study member and the cardiologist was 0.89. The ICC between cardiac technologists was 0.84. The unstable infants had their scans postponed to a later date. No infants received diuretics (>1 week) and pulmonary vasodilators during the study period.
We measured tricuspid jet velocity, TR systolic to diastolic ratio, ratio of pulmonary artery acceleration time to RV ejection time, RV dimensions on four-chamber, short axis, and long-axis views, LV eccentricity index (LVEI) (end diastole, end systole, average), and tricuspid and mitral E/A ratio as conventional echocardiographic parameters. Tissue Doppler images were obtained at the lateral mitral and tricuspid annulus (pulse wave on color-coded TDI). TDI measurements collected were isovolumetric contraction time, isovolumetric relaxation time, and ejection time. Other TDI parameters were S’, E’, A’, isovolumetric velocity, and E/E’ ratio. All the images were analyzed on site.
Statistical analysis
Categorical variables were analyzed using the chi-square test, and continuous variables were analyzed with the Mann–Whitney U-test. We constructed a receiver operating characteristic (ROC) curve for echo parameters for statistically significant conventional and TDI parameters to predict the cut-off values in predicting BPD-PH. Clustered box plots were used to show the trend of significant cardiac parameters. The data were analyzed using SPSS statistics (Version 26; IBM Corp.) and a two-sided p-value <0.05 was considered statistically significant.
The study was approved by the centralized institute review board (CIRB 2018/2077).
Results
A total of 80 infants were recruited into the study, and 74 infants were included in the final analysis, as shown in Figure 1. Five infants died before the post-conceptual age of 36 weeks and one infant withdrew from the study. The incidence of BPD-PH was 23% in our cohort. An additional 14 infants showed PH on continued screening beyond 36 weeks of gestation (mean age of diagnosis 39.5 ± 6.42 weeks) making the total incidence of BPD-PH 41%.
The mean gestational age of the study population was 25.9 ± 1.1 weeks and the mean birth weight was 830 ± 174 g. Table 1 shows that 3 (18%) infants in the mild BPD group had evidence of PH, whereas the incidence was 82.4% (14/17) in moderate to severe BPD.
The BPD-PH group had a high incidence of Hs PDA compared to the BPD-no PH group (83% vs. 56%, p < 0.018), longer oxygen days (96.16 ± 68.09 vs. 59.35 ± 52.1, p < 0.008), invasive ventilation days (31.03 ± 40.4 vs. 13.77 ± 19.2, p < 0.01), and hospital stay (133.8 ± 45.9 days vs. 106.5 ± 37.9 days, p < 0.005).
In total, 13 infants in the PH group required home oxygen (13 vs. 9, p < 0.02). More infants in the BPD-PH group required postnatal steroid therapy (18 (58.1%) vs. 14 (29.2%), p < 0.01) and there was higher mortality in the BPD-PH group [6 (20%) vs. 2 (4.2%), p < 0.02]. There was no statistical difference in the incidence of severe BPD in both groups, though a higher number of infants in the severe BPD group were affected by PH. The rest of the study characteristics were not significant between the groups, as shown in Table 1.
Among the conventional echocardiographic parameters, the left ventricular eccentricity index (end-diastolic, end-systolic, and average) was higher in infants with BPD-PH (1.24 ± 0.1 vs. 0.97 ± 0.1, p < 0.001) and lower ACT/RVET ratio from 33 weeks (0.28 ± 0.05 vs. 0.24 ± 0.05, p < 0.05). These differences persisted at the 36-week scan. Infants with BPD-PH showed higher basal RV dimension from 33 weeks (11.8 ± 2.3 vs. 10.6 ± 1.75 mm, p < 0.04) and 36 weeks (13.6 ± 1.95 vs. 12.5 ± 1.8 mm, p < 0.03). The conventional echocardiographic features are summarized in Table 2.
The RV TDI parameters isovolumetric contraction time (IVCT), isovolumetric relaxation time (IVRT), and RV myocardial performance index (MPI) showed significant differences between the groups. At the 33-week scan, the BPD-PH group showed prolonged IVCT (27.84 ± 5.5 vs. 22.77 ± 4 ms, p < 0.001), IVRT (40.3 ± 7.1 vs. 34.9 ± 5.3 ms p < 0.003), and abnormal MPI (0.39 ± 0.05 vs. 0.32 ± 0.03, p < 0.001). The ejection time was shorter in infants with BPD-PH at the 36-week scan (168 ± 12.3 vs. 183 ± 18.5 ms, p < 0.004). These differences persisted at 36 weeks, as shown in Table 3. The TDI parameters showed a similar trend when adjusted for gestational age at birth.
The ROC curve showed end-systolic LV EI >1.16 had a sensitivity of 94% and specificity of 98% when diagnosing BPD-PH [area under the curve (AUC) 0.734, p < 0.001]. The RV internal diameter of >8.46 mm at 36 weeks had a sensitivity of 76% and specificity of 75% when diagnosing BPD-PH. The ACT/RVET ratio of 0.25 had a low sensitivity (41%) and specificity (40%) when diagnosing PH (AUC 0.304, p < 0.016), as shown in Figure 2A.
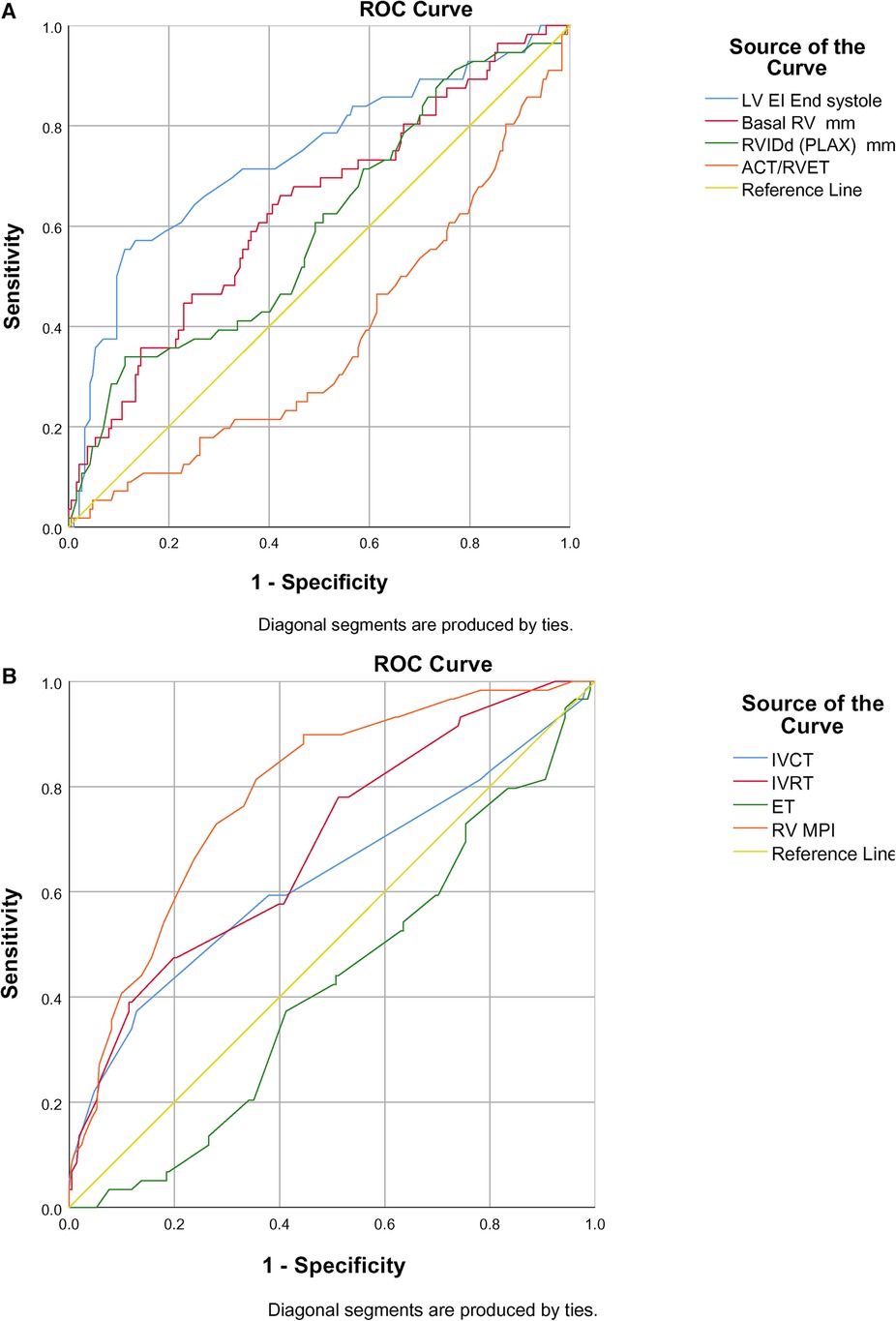
Figure 2. (A) ROC curve of conventional echocardiographic parameters in predicting BPD-PH. (B) ROC curve of TDI parameters in predicting BPD-PH.
Among the TDI parameters, the RV MPI had better predictability (AUC 0.786, p < 0.001) followed by IVRT (AUC 0.688, p < 0.001) and IVCT (AUC 0.628, p < 0.003), as shown in Figure 2B. The RV MPI of 0.38 had a sensitivity of 66% and specificity of 76%, the IVCT of >25 ms had a sensitivity of 59% and specificity of 62%, and the IVRT >40 ms had a sensitivity and specificity of 57% and 60%, respectively, when diagnosing BPD-PH. The clustered box plots for echo parameter (LV EI, IVCT, IVRT, RV MPI) trends at different time points is shown in Figures 3A–D.
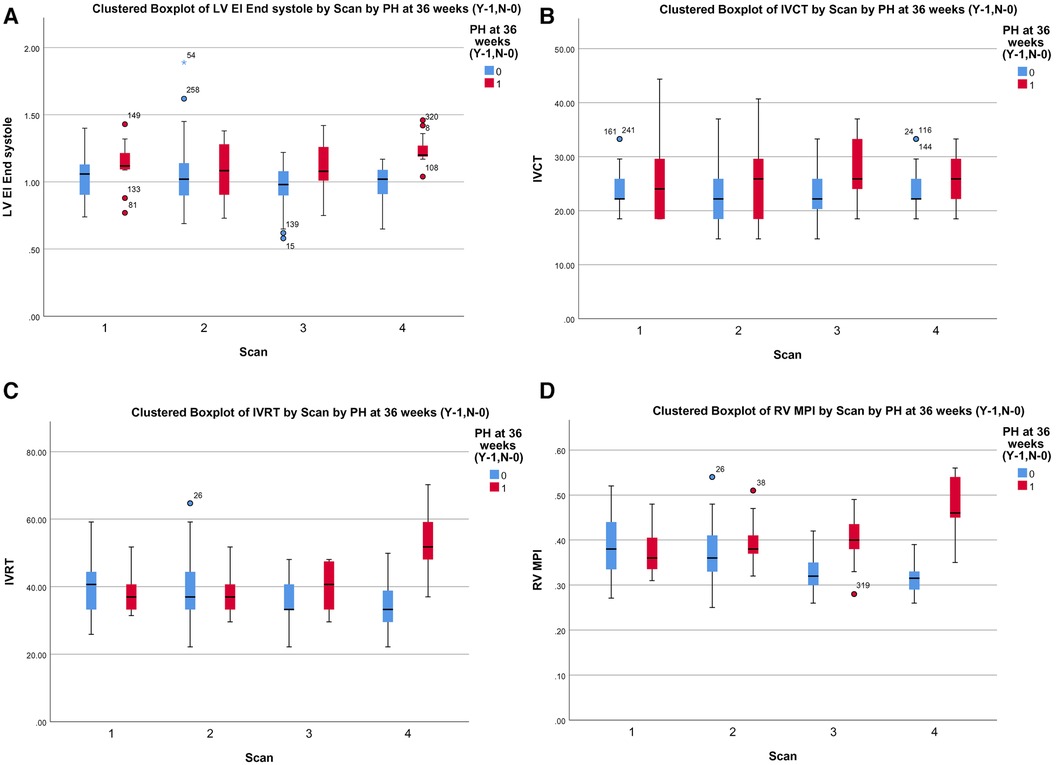
Figure 3. (A) Cluster box plot of LV EI. (B) Cluster box plot of IVCT. (C) Cluster box plot of IVRT. (D) Cluster box plot of MPI.
Discussion
The incidence of PH in our study was 23% (17/74) at the 36-week scan. The total incidence of PH increased to 41% on echocardiogram screening beyond 36 weeks of post-conceptual age. Our results are similar to those of previously reported prospective studies (7, 18, 19): 3 (18%) infants with mild BPD had PH, which is higher than in previously published studies except in a study by Sun et al., which showed a 33% incidence of mild BPD (6, 20). The abnormal fetal vascular modeling might be the reason for elevated vascular resistance in this group (21). This highlights the importance of screening infants with all grades of BPD for PH.
In our study, BPD-PH had a higher incidence of hemodynamically significant PDA. PDA as a significant predictor of BPD-PH has been described by others (5, 7, 22). The prolonged exposure to PDA results in structural and functional changes in developing pulmonary arterioles resulting in elevated pulmonary arterial pressures (23). Our study demonstrated the worst outcome of respiratory morbidities in terms of the need for prolonged respiratory support, oxygen therapy, hospital stay, and higher mortality in the BPD-PH cohort (19, 20, 24, 25).
The LV EI is an objective measure of septal configuration at a modified short-axis view (Figure 1, echo) and is a useful marker of RV systolic hypertension (26). In our study, LVEI, ACT/RVET, and basal RV dimensions were statistically significantly different from 33 weeks onwards. Abraham and Weismann showed LV EI >1.15 correlates with systolic septal flattening and a ratio >1.3 was associated with RV functional impairment (14). The utility of age-dependent LV EI and cut-off values in predicting PH has also been validated by Schweintzger et al. (27). Our study showed an end-systolic LV EI >1.16 had high sensitivity and specificity in diagnosing BPD-PH.
The ACT/RVET has been correlated with catheter-measured pulmonary pressure (28). The lower ratio <0.31 reliably detected elevated pulmonary vascular resistance with high sensitivity and specificity in older children (9, 29). In our study, the BPD-PH group had a lower ratio (<25) but had lower sensitivity and specificity. Nonetheless, this is a simple cardiac parameter that can be used in conjunction with other cardiac parameters in the diagnosis of BPD-PH. The ACT time was not significant between the two cohorts in our study. ACT is known to be shorter in neonates due to the faster heart rate and is well validated in older children with PH (30).
TR Vmax >2.8 m/s, which has high sensitivity in the diagnosis of PH, was present in only 16 (43%) infants. Other TR-derived measures, such as the SD/DD ratio and TR/VTI ratio, were not significant among the study groups.
Tricuspid annular plane systolic excursion (TAPSE) is one of the widely studied parameters to measure longitudinal RV lateral wall performance, which correlates with RV ejection fraction (31). TAPSE linearly increases with gestational age in preterm neonates and was significantly lower in patients with PH (32, 33). We did not find a difference in TAPSE between the groups, though the PH group had lower values. A recent meta-analysis showed Fractional area change (FAC) may have a better correlation with RV systolic function compared to TAPSE (34). TDI has been shown to correlate with invasive pulmonary hemodynamics in pediatric PH (35). IVCT, measured by the end of the a’ wave to the beginning of the S’ wave, has shown a good correlation with ventricular systolic dysfunction in various neonatal conditions (36). IVRT is measured by the end of the S’ wave to the beginning of e’ wave, is a marker of diastolic dysfunction. It is inversely correlated with the cardiac index (37). It has been well validated in pulmonary artery systolic pressure in adult patients (38).
The utility of TDI parameters, such as MPI, E/e’ ratio, RV peak systolic, isovolumic velocities, IVCT, and IVRT, has been well documented in infants with BPD-PH (39–42). Our study showed higher IVCT, IVRT, and higher MPI in the BPD-PH cohort. These changes were consistent from 33 weeks.
We observed a decreased RV E’ and E/E’, a marker of diastolic dysfunction in the BPD-PH group, but the difference was not statistically significant. The myocardial performance (Tei) index is a measure of global ventricular function (43). Patel et al. showed significantly higher RV MPI in infants with PH but a poor correlation with pulmonary artery pressure measured by TR (44). We found that RV MPI had a high predictive value followed by IVRT and IVCT. To the best of our knowledge, this is the first study to show the serial RV functional assessment in the evolution of BPD-PH. Figure 4 shows the sample 2D echocardiogram images of various conventional and TDI images from the study.
Limitations
The present study has some limitations. The smaller sample size is one of the limitations. Our study lacks the direct comparison of TDI with gold standard cardiac catheterization in the diagnosis of PHT, though we attempted to increase the robustness of diagnosis by incorporating multiple echo parameters.
Conclusions
Serial TDI along with conventional echocardiogram increases the diagnostic accuracy of BPD-PH. The TDI parameters MPI and IVRT are sensitive in recognizing early RV stress and should be incorporated in routine screening. LV end-systolic EI, ACT/RVET ratio, and RV dimensions can be incorporated into the PH screening protocol in the absence of TR. We recommend initiating PH screening from 32 to 34 weeks and continuing to screen beyond 36 weeks in high-risk infants.
Data availability statement
The original contributions presented in the study are included in the article/Supplementary Material, further inquiries can be directed to the corresponding author.
Ethics statement
The studies involving humans were approved by the Centralized Institutional Review Board. The studies were conducted in accordance with the local legislation and institutional requirements. Written informed consent for participation in this study was provided by the participants’ legal guardians/next of kin.
Author contributions
KG: Conceptualization, Data curation, Formal Analysis, Funding acquisition, Writing – original draft. AA: Investigation, Supervision, Validation, Writing – review & editing. SV: Investigation, Supervision, Writing – review & editing. SS: Data curation, Validation, Writing – review & editing. WN: Data curation, Investigation, Writing – review & editing. TC: Supervision, Validation, Writing – review & editing. WA: Investigation, Resources, Writing – review & editing. NB: Investigation, Resources, Writing – review & editing. NW: Data curation, Writing – review & editing. VR: Supervision, Validation, Writing – review & editing. KY: Conceptualization, Supervision, Validation, Writing – review & editing. TT: Supervision, Validation, Writing – review & editing.
Funding
The authors declare financial support was received for the research, authorship, and/or publication of this article.
This study was funded by Tan Cheng Lim fund/Paeds ACP (PAEDSACP-TCL/2018/RES/003), Singapore. The funder had no role in the design, data collection, data analysis, and reporting of this study.
Acknowledgments
The authors would like to thank all the parents who consented to the study. We are grateful to Miss Wendy Chin Yi Wen for her help with the preparation of the tables and figures in the manuscript.
Conflict of interest
The authors declare that the research was conducted in the absence of any commercial or financial relationships that could be construed as a potential conflict of interest.
Publisher's note
All claims expressed in this article are solely those of the authors and do not necessarily represent those of their affiliated organizations, or those of the publisher, the editors and the reviewers. Any product that may be evaluated in this article, or claim that may be made by its manufacturer, is not guaranteed or endorsed by the publisher.
References
1. Islam JY, Keller RL, Aschner JL, Hartert TV, Moore PE. Understanding the short- and long-term respiratory outcomes of prematurity and bronchopulmonary dysplasia. Am J Respir Crit Care Med. (2015) 192(2):134–56. doi: 10.1164/rccm.201412-2142PP
2. Lignelli E, Palumbo F, Myti D, Morty RE. Recent advances in our understanding of the mechanisms of lung alveolarization and bronchopulmonary dysplasia. Am J Physiol Lung Cell Mol Physiol. (2019) 317(6):L832–87. doi: 10.1152/ajplung.00369.2019
3. Baker CD, Abman SH, Mourani PM. Pulmonary hypertension in preterm infants with bronchopulmonary dysplasia. Pediatr Allergy Immunol Pulmonol. (2014) 27(1):8–16. doi: 10.1089/ped.2013.0323
4. Levy PT, Levin J, Leeman KT, Mullen MP, Hansmann G, Kourembanas S. Diagnosis and management of pulmonary hypertension in infants with bronchopulmonary dysplasia. Semin Fetal Neonatal Med. (2022) 27(4):101351. doi: 10.1016/j.siny.2022.101351
5. Arjaans S, Zwart EAH, Ploegstra M, Bos AF, Kooi EMW, Hillege HL, et al. Identification of gaps in the current knowledge on pulmonary hypertension in extremely preterm infants: a systematic review and meta-analysis. Paediatr Perinat Epidemiol. (2018) 32(3):258–67. doi: 10.1111/ppe.12444
6. Khemani E, McElhinney DB, Rhein L, Andrade O, Lacro RV, Thomas KC, et al. Pulmonary artery hypertension in formerly premature infants with bronchopulmonary dysplasia: clinical features and outcomes in the surfactant era. Pediatrics. (2007) 120(6):1260–9. doi: 10.1542/peds.2007-0971
7. Mourani PM, Sontag MK, Younoszai A, Miller JI, Kinsella JP, Baker CD, et al. Early pulmonary vascular disease in preterm infants at risk for bronchopulmonary dysplasia. Am J Respir Crit Care Med. (2015) 191(1):87–95. doi: 10.1164/rccm.201409-1594OC
8. Kim DH, Kim HS, Choi CW, Kim EK, Kim BI, Choi JH. Risk factors for pulmonary artery hypertension in preterm infants with moderate or severe bronchopulmonary dysplasia. Neonatology. (2012) 101(1):40–6. doi: 10.1159/000327891
9. Revanna GK, Kunjunju A, Sehgal A. Bronchopulmonary dysplasia associated pulmonary hypertension: making the best use of bedside echocardiography. Prog Pediatr Cardiol. (2017) 46:39–43. doi: 10.1016/j.ppedcard.2016.12.003
10. Mourani PM, Abman SH. Pulmonary hypertension and vascular abnormalities in bronchopulmonary dysplasia. Clin Perinatol. (2015) 42(4):839–55. doi: 10.1016/j.clp.2015.08.010
11. Krishnan U, Feinstein JA, Adatia I, Austin ED, Mullen MP, Hopper RK, et al. Evaluation and management of pulmonary hypertension in children with bronchopulmonary dysplasia. J Pediatr. (2017) 188:24–34.1. doi: 10.1016/j.jpeds.2017.05.029
12. Breatnach CR, EL-Khuffash A, James A, McCallion N, Franklin O. Serial measures of cardiac performance using tissue Doppler imaging velocity in preterm infants <29 weeks gestations. Early Hum Dev. (2017) 108:33–9. doi: 10.1016/j.earlhumdev.2017.03.012
13. Nestaas E, Schubert U, de Boode WP, El-Khuffash A, on behalf of the European Special Interest Group “Neonatologist Performed Echocardiography’ (NPE). Tissue Doppler velocity imaging and event timings in neonates: a guide to image acquisition, measurement, interpretation, and reference values. Pediatr Res. (2018) 84(S1):18–29. doi: 10.1038/s41390-018-0079-8
14. Abraham S, Weismann CG. Left ventricular end-systolic eccentricity index for assessment of pulmonary hypertension in infants. Echocardiography. (2016) 33(6):910–5. doi: 10.1111/echo.13171
15. Parasuraman S, Walker S, Loudon BL, Gollop ND, Wilson AM, Lowery C, et al. Assessment of pulmonary artery pressure by echocardiography—a comprehensive review. IJC Heart Vasc. (2016) 12:45–51. doi: 10.1016/j.ijcha.2016.05.011
16. Nagiub M, Lee S, Guglani L. Echocardiographic assessment of pulmonary hypertension in infants with bronchopulmonary dysplasia: systematic review of literature and a proposed algorithm for assessment. Echocardiography. (2015) 32(5):819–33. doi: 10.1111/echo.12738
17. Jobe AH, Bancalari E. Bronchopulmonary dysplasia. Am J Respir Crit Care Med. (2001) 163(7):1723–9. doi: 10.1164/ajrccm.163.7.2011060
18. An HS, Bae EJ, Kim GB, Kwon BS, Beak JS, Kim EK, et al. Pulmonary hypertension in preterm infants with bronchopulmonary dysplasia. Korean Circ J. (2010) 40(3):131–6. doi: 10.4070/kcj.2010.40.3.131
19. Bhat R, Salas AA, Foster C, Carlo WA, Ambalavanan N. Prospective analysis of pulmonary hypertension in extremely low birth weight infants. Pediatrics. (2012) 129(3):e682–9. doi: 10.1542/peds.2011-1827
20. Sun J, Weng B, Zhang X, Chu X, Cai C. Risk factors and clinical characteristics for bronchopulmonary dysplasia associated pulmonary hypertension in very-low-birth-weight infants. BMC Cardiovasc Disord. (2021) 21(1):514. doi: 10.1186/s12872-021-02330-w
21. Pierro M, Villamor-Martinez E, van Westering-Kroon E, Alvarez-Fuente M, Abman SH, Villamor E. Association of the dysfunctional placentation endotype of prematurity with bronchopulmonary dysplasia: a systematic review, meta-analysis and meta-regression. Thorax. (2022) 77(3):268. doi: 10.1136/thoraxjnl-2020-216485
22. Gentle SJ, Travers CP, Clark M, Carlo WA, Ambalavanan N. Patent ductus arteriosus and development of bronchopulmonary dysplasia-associated pulmonary hypertension. Am J Respir Crit Care Med. (2023) 207(7):921–8. doi: 10.1164/rccm.202203-0570OC
23. Philip R, Lamba V, Talati A, Sathanandam S. Pulmonary hypertension with prolonged patency of the ductus arteriosus in preterm infants. Child Basel Switz. (2020) 7(9):139. doi: 10.3390/children7090139
24. Ali Z, Schmidt P, Dodd J, Jeppesen DL. Predictors of bronchopulmonary dysplasia and pulmonary hypertension in newborn children. Dan Med J. (2013) 60(8):A4688.23905570
25. Chen Y, Zhang D, Li Y, Yan A, Wang X, Hu X, et al. Risk factors and outcomes of pulmonary hypertension in infants with bronchopulmonary dysplasia: a meta-analysis. Front Pediatr. (2021) 9:695610. doi: 10.3389/fped.2021.695610
26. King ME, Braun H, Goldblatt A, Liberthson R, Weyman AE. Interventricular septal configuration as a predictor of right ventricular systolic hypertension in children: a cross-sectional echocardiographic study. Circulation. (1983) 68(1):68–75. doi: 10.1161/01.CIR.68.1.68
27. Schweintzger S, Kurath-Koller S, Burmas A, Grangl G, Fandl A, Noessler N, et al. Normal echocardiographic reference values of the right ventricular to left ventricular endsystolic diameter ratio and the left ventricular endsystolic eccentricity index in healthy children and in children with pulmonary hypertension. Front Cardiovasc Med. (2022) 9:950765. doi: 10.3389/fcvm.2022.950765
28. Kosturakis D, Goldberg SJ, Allen HD, Loeber C. Doppler echocardiographic prediction of pulmonary arterial hypertension in congenital heart disease. Am J Cardiol. (1984) 53(8):1110–5. doi: 10.1016/0002-9149(84)90646-5
29. Levy PT, Patel MD, Groh G, Choudhry S, Murphy J, Holland MR, et al. Pulmonary artery acceleration time provides a reliable estimate of invasive pulmonary hemodynamics in children. J Am Soc Echocardiogr. (2016) 29(11):1056–65. doi: 10.1016/j.echo.2016.08.013
30. Koestenberger M, Grangl G, Avian A, Gamillscheg A, Grillitsch M, Cvirn G, et al. Normal reference values and z scores of the pulmonary artery acceleration time in children and its importance for the assessment of pulmonary hypertension. Circ Cardiovasc Imaging. (2017) 10(1):e005336. doi: 10.1161/CIRCIMAGING.116.005336
31. Sato T, Tsujino I, Oyama-Manabe N, Ohira H, Ito YM, Sugimori H, et al. Simple prediction of right ventricular ejection fraction using tricuspid annular plane systolic excursion in pulmonary hypertension. Int J Cardiovasc Imaging. (2013) 29(8):1799–805. doi: 10.1007/s10554-013-0286-7
32. Zakaria D, Sachdeva R, Gossett JM, Tang X, O’Connor MJ. Tricuspid annular plane systolic excursion is reduced in infants with pulmonary hypertension. Echocardiography. (2015) 32(5):834–8. doi: 10.1111/echo.12797
33. Seo YH, Choi HJ. Clinical utility of echocardiography for early and late pulmonary hypertension in preterm infants: relation with bronchopulmonary dysplasia. J Cardiovasc Ultrasound. (2017) 25(4):124–30. doi: 10.4250/jcu.2017.25.4.124
34. Lee JZ, Low SW, Pasha AK, Howe CL, Lee KS, Suryanarayana PG. Comparison of tricuspid annular plane systolic excursion with fractional area change for the evaluation of right ventricular systolic function: a meta-analysis. Open Heart. (2018) 5(1):e000667. doi: 10.1136/openhrt-2017-000667
35. Lammers AE, Haworth SG, Riley G, Maslin K, Diller GP, Marek J. Value of tissue Doppler echocardiography in children with pulmonary hypertension. J Am Soc Echocardiogr. (2012) 25(5):504–10. doi: 10.1016/j.echo.2012.01.017
36. Hirschfeld S, Meyer R, Korfhagen J, Kaplan S, Liebman J. The isovolumic contraction time of the left ventricle. An echographic study. Circulation. (1976) 54(5):751–6. doi: 10.1161/01.CIR.54.5.751
37. Schmitz L, Stiller B, Koch H, Koehne P, Lange P. Diastolic left ventricular function in preterm infants with a patent ductus arteriosus: a serial Doppler echocardiography study. Early Hum Dev. (2004) 76(2):91–100. doi: 10.1016/j.earlhumdev.2003.11.002
38. Zimbarra Cabrita I, Ruisanchez C, Grapsa J, Dawson D, North B, Pinto FJ, et al. Validation of the isovolumetric relaxation time for the estimation of pulmonary systolic arterial blood pressure in chronic pulmonary hypertension. Eur Heart J Cardiovasc Imaging. (2013) 14(1):51–5. doi: 10.1093/ehjci/jes093
39. Yates AR, Welty SE, Gest AL, Cua CL. Myocardial tissue Doppler changes in patients with bronchopulmonary dysplasia. J Pediatr. (2008) 152(6):766–70. doi: 10.1016/j.jpeds.2007.11.039
40. Czernik C, Rhode S, Metze B, Schmalisch G, Bührer C. Persistently elevated right ventricular index of myocardial performance in preterm infants with incipient bronchopulmonary dysplasia. PLoS One. (2012) 7(6):e38352. doi: 10.1371/journal.pone.0038352
41. Sehgal A, Malikiwi A, Paul E, Tan K, Menahem S. Right ventricular function in infants with bronchopulmonary dysplasia: association with respiratory sequelae. Neonatology. (2016) 109(4):289–96. doi: 10.1159/000442967
42. Yajamanyam PK, Negrine RJS, Rasiah SV, Zamora J, Ewer AK. Assessment of myocardial function in preterm infants with chronic lung disease using tissue Doppler imaging. Arch Dis Child Fetal Neonatal Ed. (2016) 101(6):527–32. doi: 10.1136/archdischild-2015-308929
43. Pellett AA, Tolar WG, Merwin DG, Kerut EK. The Tei index: methodology and disease state values. Echocardiography. (2004) 21(7):669–72. doi: 10.1111/j.0742-2822.2004.04052.x
Keywords: bronchopulmonary dysplasia, pulmonary hypertension, tissue Doppler imaging, extreme prematurity, conventional echocardiogram
Citation: Gopagondanahalli KR, Abdul Haium AA, Vora SJ, Sundararaghavan S, Ng WD, Choo TLJ, Ang WL, Binte Mohamad Taib NQ, Wijedasa NHY, Rajadurai VS, Yeo KT and Tan TH (2024) Serial tissue Doppler imaging in the evaluation of bronchopulmonary dysplasia-associated pulmonary hypertension among extremely preterm infants: a prospective observational study. Front. Pediatr. 12:1349175. doi: 10.3389/fped.2024.1349175
Received: 4 December 2023; Accepted: 18 March 2024;
Published: 5 April 2024.
Edited by:
David Warburton, Children’s Hospital Los Angeles, United StatesReviewed by:
Mahmoud Montasser, Wishaw General Hospital, United KingdomAbhijit Bhattacharya, Royal Hospital for Children, United Kingdom
© 2024 Gopagondanahalli, Abdul Haium, Vora, Sundararaghavan, Ng, Choo, Ang, Binte Mohamad Taib, Wijedasa, Rajadurai, Yeo and Tan. This is an open-access article distributed under the terms of the Creative Commons Attribution License (CC BY). The use, distribution or reproduction in other forums is permitted, provided the original author(s) and the copyright owner(s) are credited and that the original publication in this journal is cited, in accordance with accepted academic practice. No use, distribution or reproduction is permitted which does not comply with these terms.
*Correspondence: Krishna Revanna Gopagondanahalli kris.cta@gmail.com