- 1Epilepsy Center, Children’s Hospital Affiliated to Shandong University, Jinan, Shandong, China
- 2Epilepsy Center, Jinan Children's Hospital, Jinan, Shandong, China
- 3Department of Ophthalmology, Children's Hospital Affiliated to Shandong University, Jinan, Shandong, China
- 4Department of Ophthalmology, Jinan Children's Hospital, Jinan, Shandong, China
The symptoms and signs of infection caused by severe acute respiratory syndrome coronavirus 2 (SARS-CoV-2) are milder in children than in adults. However, in April 2020, British pediatricians first reported that coronavirus disease 2019 (COVID-19) may present as multisystem inflammatory syndrome in children and adolescents (MIS-C), similar to that observed in Kawasaki disease. MIS-C can be associated with multiple systemic injuries and even death in children. In addition to digestive system involvement, cardiac injury is prominent. This article reviews the pathogenesis, clinical manifestations, and treatment of cardiac injury caused by MIS-C, which may help clinicians in early diagnosis and timely commencement of treatment.
Introduction
Coronavirus disease 2019 (COVID-19) caused by severe acute respiratory syndrome coronavirus 2 (SARS-CoV-2) has led to high morbidity and mortality worldwide, which has generated significant global concern. The World Health Organization (WHO) declared COVID-19 a pandemic on March 11, 2020. COVID-19 is less severe in children than in adults because of the lower expression of angiotensin-converting enzyme 2 (ACE2) receptors, a poorly developed immune system, and the slightest possibility of inflammatory cytokine storms causing limited virus invasion, thereby avoiding large-scale outbreaks in children. However, severe cases of COVID-19 in children have been reported in Europe and the United States since April 2020, with symptoms and signs similar to those of incomplete Kawasaki disease or toxic shock syndrome (1–5). The Centers for Disease Control and Prevention (CDC) and the WHO named it a multisystem inflammatory syndrome in children and adolescents (MIS-C), with persistent high fever, digestive tract symptoms, shock, cardiac dysfunction, nervous system disorders, and other multi-organ injuries. Most children develop digestive system disorders, followed by cardiac injury; nonetheless, the manifestations of cardiac injury are variable and potentially fatal. This review focuses on the pathogenesis, clinical manifestations, and treatment of MIS-C and cardiac injury in children.
SARS-CoV-2 structure
Coronavirus is a single-stranded RNA virus, which is divided into four genera: α, β, γ, and δ (6). Coronavirus β includes SARS-CoV, SARS-CoV-2, and the Middle East respiratory syndrome coronavirus. SARS-CoV-2 is transmitted mainly through inhalation of respiratory droplets from infected people or through contact with virus-contaminated surfaces. The spike glycoprotein, S protein, present on the surface of the coronavirus makes this series of viruses appear typical of coronaviruses microscopically (6). Coronavirus entry into host cells is regulated by S protein, which is divided into S1 and S2 subunits. The S1 subunit includes amino-terminal domain and carboxy-terminal domain (CTD); The CTD recognizes and binds to receptors, also known as receptor-binding domain (RBD). The entry of coronavirus into host cells is a complex process that requires the synergistic effect of binding with receptors and catalytic treatment of S protein to promote virus and host cell fusion (7, 8). ACE2 is a necessary enzyme in the renin-angiotensin system (RAS), which can effectively maintain RAS balance (9). ACE2 is a type-I membrane protein. Full-length ACE2 consists of a peptidase domain (PD) and a collagen-like domain. ACE2 mainly regulates angiotensin, controls vasoconstriction and blood pressure, and protects the cardiovascular system and many other tissues and organs (8, 10, 11) (Figure 1). Hamming et al. (12) verified that ACE2 is highly expressed in many other tissues and cells, such as myocardium, vascular endothelial cells, proximal renal tubules, bladder epithelial cells, and human lung and intestinal epithelial cells. Li et al. showed that ACE2 is the receptor of severe acute respiratory syndrome coronavirus (SARS-CoV) in 2003, and Yan et al. found that it is the receptor of SARS-CoV-2 in 2020 (7, 13, 14). The S protein on SARS-CoV-2's surface has a high affinity for ACE2. Novel coronavirus enters the host cell mainly through S protein on the virus surface binding to the ACE2 receptor (15); the spike RBD of SARS-CoV-2 binds to the ACE2 PD to form an RBD-PD complex, allowing the virus to enter the host cell (7). The heart is a high-risk target for SARS-CoV-2 infection. Most children with MIS-C have cardiac injury; malignant arrhythmias and heart failure can occur in severe cases, which is closely related to case mortality (16).
Epidemiological characteristics and clinical manifestations of MIS-C
Multiple research teams have conducted meta-analyses of MIS-C caused by SARS-CoV-2 infection (1, 3, 4, 17), finding that MIS-C is found in children of all ages, mostly 6–15 years old, with a male to female ratio approximating 1:1; black children are at greater risk than Asians; most MIS-C appears 4–6 weeks after SARS-CoV-2 infection in children (18); and respiratory, circulatory, renal, digestive, and nervous system injuries can occur in MIS-C. Among these, digestive system involvement is prominent, followed by cardiovascular system involvement. Statistics showed that 50%–90% of patients had digestive tract disorders, 50%–81% had cardiogenic shock, 53%–80% had cardiovascular diseases, 21%–70% had respiratory disorders, 22%–52% had acute renal injuries, and nervous system disorders occurred in 12%–57%. Most patients with MIS-C have a high incidence of gastrointestinal tract symptoms, such as vomiting and diarrhea (17, 19). The WHO, CDC, and the Royal College of Pediatrics and Child Health have established corresponding standards for the definition and diagnosis of inflammatory response syndrome in children, as shown in Table 1 (20).
Pathophysiological mechanism
Immune-mediated injury
The clinical characteristics of an inflammatory response syndrome, laboratory test findings, and the temporal association with SARS-CoV-2 infection support the hypothesis that SARS-CoV-2 infection causes immune-mediated damage to tissues and organs resulting in MIS-C (21). First, symptoms and signs of MIS-C appear after virus infection, not acutely during COVID-19 (22); second, MIS-C onset lags behind COVID-19 onset by approximately 4–5 weeks, which supports the hypothesis that MIS-C is an immune system disorder associated with SARS-COV-2 infection. A study by Feldstein et al. on 186 patients found that 14 had obvious COVID-19 symptoms before MIS-C onset (21), with an approximate 25-day interval between COVID-19 symptoms and MIS-C onset, and others reporting SARS-CoV-2 infection approximately 1–2 weeks before MIS-C onset. Belot et al. reported that 95 patients with MIS-C were diagnosed post-COVID-19 with a lag time of approximately 4–5 weeks (23). MIS-C, caused by the abnormal immune response in SARS-COV-2 infection, results in multi-organ damage. Gruber et al. reported an immune map of nine cases of MIS-C in which serum antibodies against the S protein on the SARS-CoV-2 surface were detected (24, 25). They found positive serum antibodies in all MIS-C patients, and on evaluating immunoglobulin antibody subtypes, observed increased plasma levels of IgG immunoglobulin antibodies, but decreased levels of plasma IgM antibodies (24). Most patients with MIS-C are positive for anti-SARS-CoV-2 serum antibodies but negative for viral RNA, and only a few patients with MIS-C show PCR positivity. McMurray et al. also found that in most MIS-C cases, PCR nucleic acid detection was negative in most children, with positive serum anti-SARS-CoV-2 antibodies (22). Therefore, these findings show that MIS-C is a type of acquired immune-mediated response following SARS-CoV-2 infection, rather than persistent infection caused by direct virus invasion.
Direct invasion of the virus
Hamming et al. observed that ACE2 receptor expression were higher not only on lung surface and intestinal epithelial cells, but also in cardiomyocytes, indicating that cardiomyocytes are also high-risk targets for novel coronavirus infection (12). The novel coronavirus surface S protein has a high affinity for ACE2 and assists the entry of virus inside host cells by binding with ACE2 (Figure 2). Oudit et al. studied 20 patients who died of SARS-COV infection, and seven of them tested positive for SARS-COV genome in cardiac tissue, suggesting that the virus can directly damage cardiomyocytes (26). SARS-COV-2 and SARS-COV have highly homologous genomes (27, 28). It has been suggested that SARS-COV-2 can also directly invade and injure myocardial cells (29). After binding with ACE2 by S protein, the virus enters cells, where it replicates and destroys host cells in large numbers, and eventually leads to cardiac tissue damage. Rapid replication of the virus may lead to endothelial and epithelial cell apoptosis, blood vessel leakage, and release of proinflammatory regulatory cells and factors.
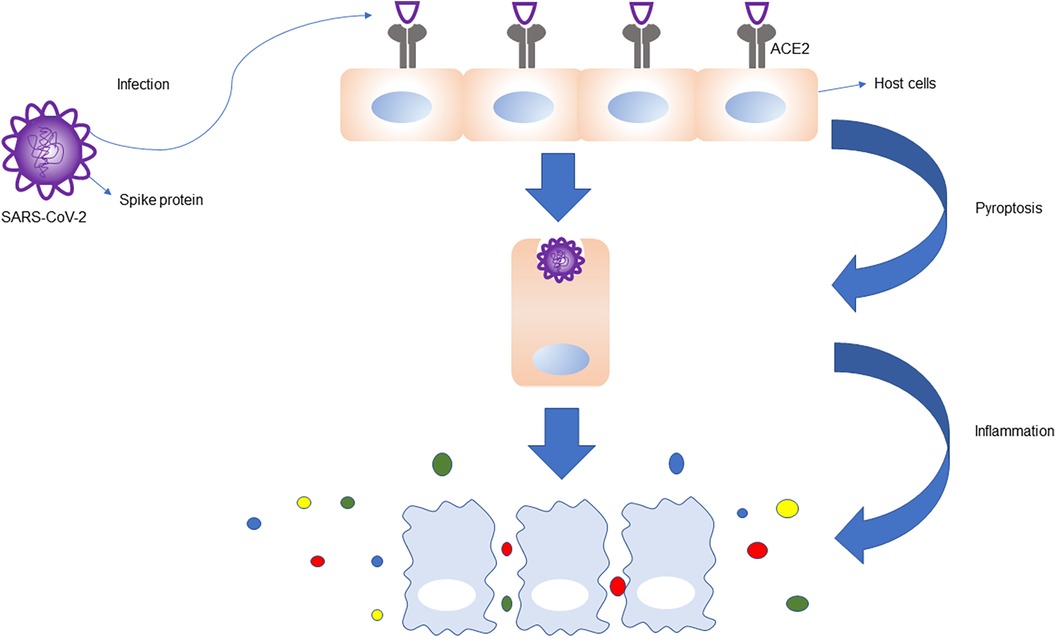
Figure 2. ACE2 receptors are highly expressed in the lung, heart, intestine, vascular endothelial cells, proximal renal tubules, and bladder epithelial cells. S protein on the surface of SARS-CoV-2 binds to ACE2 receptors, which helps the virus to enter the host cells, leading to host cell infection, apoptosis, and inflammation. The immune response is magnified, and the immune cells release a large number of cytokines and chemokines.
Cytokine storm
Many clinical studies have shown that cytokine storms occur in patients with MIS-C (12, 30–36), with upregulation of large numbers of inflammatory cytokines, leading to endothelial cell damage (37, 38). Cytokines are low molecular weight proteins or peptides synthesized and secreted by a variety of cells with many biological activities involved in cell-to-cell signal transduction and interaction. Cytokines have many biological functions, such as innate and adaptive immunity regulation, hematopoiesis, cell growth and differentiation, and tissue repair. Many cytokines promote or restrict each other in the body, forming a complex cytokine immunomodulatory network. Cytokines are double-edged swords; they not only play an immunomodulatory role, but also participate in disease occurrence under certain conditions. They can cause cytokine storm and cytokine storm syndrome, which results in multiple organ damage, function failure, and even death.
Cytokines are produced by many immune cells, including T and B lymphocytes, macrophages, natural killer cells, and dendritic cells. The innate immune system responds to viral invasion by triggering an inflammatory response, activating related signaling pathways, and stimulating interferon (IFN) and other cytokine production. The three most important proinflammatory cytokines in the innate immune response, including interleukin (IL)-1, IL-6, and tumor necrosis factor (TNF)-α, are mainly derived from macrophages, mast cells, epithelial cells, and endothelial cells. The main types and functions of cytokines are as follows: the IL family is responsible for immune cell proliferation and differentiation; IFN regulates innate immunity and activates antiviral properties and anti-proliferation; TNF promotes inflammation and activates cytotoxic T cells; colony-stimulating factor stimulates stem progenitor cell proliferation and differentiation; chemokines mainly control chemotaxis and recruit leukocytes; growth factors promote individual development by promoting cell growth and differentiation.
The main cause of a cytokine storm is immune response imbalance. With disease development, the immune response is dysregulated, and activated T cells or other related immune effector cells release a large number of proinflammatory cytokines and chemokines. These explosive proinflammatory cytokines and chemokines (including IL-1, IL-6, IL-17, IL-12, TNF-α, IFN-α, IFN-β, IFN-γ, and monocyte chemotactic factor 1) lead to the activation of macrophages, mast cells, other immune cells, and endothelial cells; the cytokines released by the latter further activate more immune cells through autocrine and paracrine cascade reactions. This dysregulates the immune regulatory network, forming a cytokines cascade that initiates a cytokine storm, which damages self-immune system homeostasis along with normal tissue and cell function. When cytokine storms occur, inflammatory responses occur in multiple organ systems, which results in pulmonary symptoms (hypoxemia, pulmonary edema caused by vascular leakage, and even acute respiratory distress syndrome), cardiovascular symptoms (hypotension, arrhythmia, myocardial damage, and shock), hematological symptoms (persistent decrease of blood cells, coagulation disorders, and diffuse intravascular coagulation), acute renal injury, multi-organ failure, and even death. This uncontrolled systemic inflammatory response is caused by the release of extreme inflammatory mediators caused by the over-activation and expansion of primary immune cells.
The expression of ACE2 mentioned above was first found in the heart, kidney, and testis, and later in the upper respiratory tract, lung, intestine, and liver. Cells with ACE2 receptors, such as cardiac cells, can be host cells for SARS-CoV-2 infection. When the virus infects cells, immune cells produce a large number of cytokines. Mast cells regulate the expression of proinflammatory cytokines TNF-α and IL-6, and significantly upregulate the expression of inflammatory factors CCL2, CCL3, CCL5, and CXCL10. Macrophages produce IFN and other cytokines, so a surge of cytokines and chemokines leads to host cell damage, and eventually cardiac damage. Cater et al. (39) analyzed the serum of 25 children with acute stage MIS-C and observed significantly increased levels of IL-1, IL-6, IL-10, IL-17, and interferon-γ. The high expression of human leucocyte antigen (HLA)-DR on T cells in the acute phase indicates that the immune cell group is activated. Consiglio et al. examined plasma samples from 11 children with MIS-C and 28 children with Kawasaki disease and found that elevated IL-6, IL-17A, and CXCL10 play a major role in cytokine storms (40). Gruber et al. (24) reported that the increase in cytokines and chemokines in patients with MIS-C is mainly related to the synthesis and secretion of circulating natural killer cells and T cells, as well as the activation and elevation of CCL19, CXCL10, CCL3, CCL4, CDCP1, and colony-stimulating factor 1. Laura et al. analyzed the immune response in the peripheral blood of patients with SARS-CoV-2 infection and MIS-C, finding obvious activation and proliferation of T cells in patients with MIS-C, with greater activation of CX3CR1 + CD8 + T cells (41). This may explain the cardiac injury caused by cytokine storms in patients with MIS-C (Figure 3).
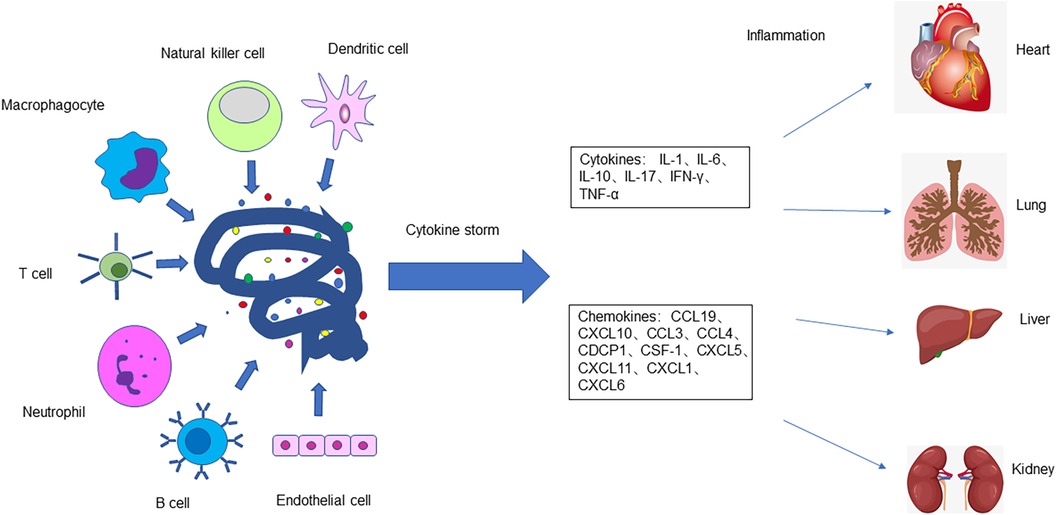
Figure 3. The body's immune response is dysregulated after SARS-CoV-2 infects host cells. T cells, B cells, macrophages, neutrophils, natural killer cells, dendritic cells, epithelial cells, and endothelial cells release a large number of cytokines and chemokines, causing a cytokine storm and severe inflammatory reaction which damages tissues and organs of the body, including the heart, lungs, liver, intestines, kidneys, and testicles.
Innate and adaptive immune dysregulation
SARS-CoV-2 can activate both innate and adaptive immune responses (42). Viral infection can cause an innate immune response, including elevated erythrocyte sedimentation rate (ESR), C-reactive protein (CRP), serum amyloid A, and ferritin levels, and an increase in some inflammatory cytokines (30, 43, 44). The early response of type-I IFN is the first line of defense in suppressing viral replication and spread; however, SARS-CoV-2 can suppress the response of type-I IFN by interfering with pattern recognition receptors or the type-I IFN receptor-signaling pathway (45). In addition, in genetic immune deficiencies or immunoregulation dysfunction, delayed type-I IFN reaction leads to eosinophil and monocyte macrophage release, followed by apoptosis of T cells, epithelial cells, and endothelial cells. Therefore, the intensity and time point at which the innate immune system responds play a crucial role, and poor regulation of the innate immune response leads to disease progression (46–48).
SARS-CoV-2 infection leads to specific antibody formation that binds host autoantigens. The target antigens of autoantibodies are expressed in the mucosa, heart tissue, and endothelial cells. When antibodies bind to antigens, immune complexes form that activate the viral superantigen series of host immune cells and inflammatory response, promote the activation and release of proinflammatory cytokines (20, 49), and finally lead to heart injury. Gruber et al. found that patients with MIS-C had neutralizing antibodies against SARS-CoV-2, which were related to the activation of IL-18, IL-16, lymphocytes, monocytes, and natural killer cells. Elevated mucosal immune dysregulation (IL-17A, CCL20 and CCL28) and neutrophil and macrophage upregulation of intercellular adhesion molecule 1 and FcγR1 indicate enhanced antigen expression and Fc-mediated response (24). Consiglio et al. analyzed the antigen-antibody responses of patients with MIS-C. They identified autoantibodies, including antibodies to MAP2K2 and the casein kinase family (CSNK1A1, CSNK2A1, and CSNK1E1), which appeared in the acute phase of SARS-CoV-2 infection (40). The targets of antibodies are widely expressed in the heart and other tissues, corresponding to the broad, multisystem involvement in patients with MIS-C.
Clinical features
The clinical symptoms of cardiac injury in patients with MIS-C mainly include chest pain, arrhythmias, electrocardiogram (ECG) changes, hypotension, and echocardiographic changes (coronary artery dilatation and aneurysms). Laboratory investigations show increased creatine kinase, troponin, and brain natriuretic peptide (BNP) levels. The possible clinical symptoms, laboratory test results, examinations, and imaging changes of cardiac injury are summarized and analyzed in the following sections (Table 2).
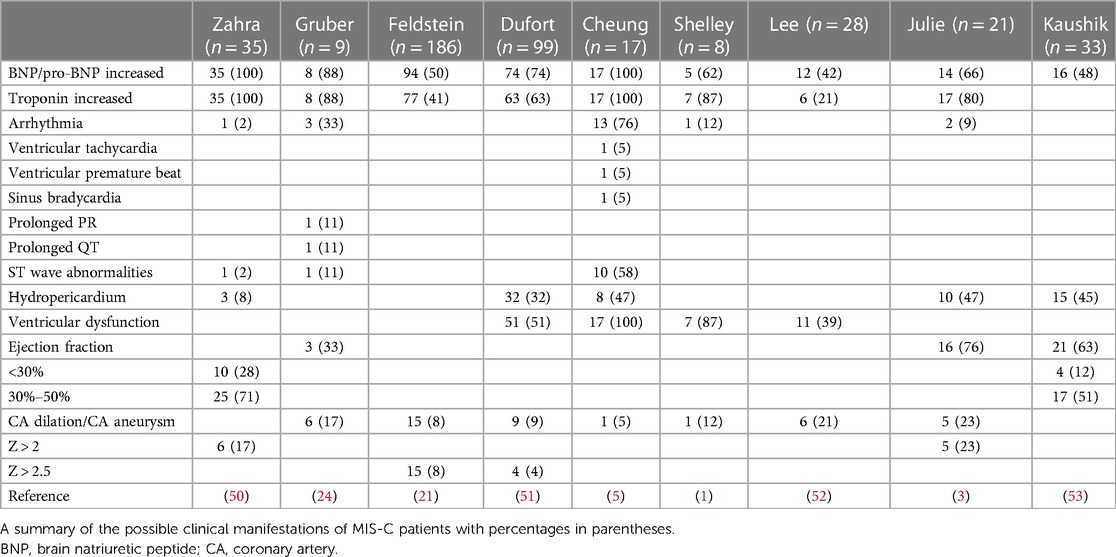
Table 2. Clinical manifestations, laboratory results, and echocardiography findings in MIS-C patients.
BNP and troponin
The troponin complex comprises cTnC, cTnI, and cTnT, cTnT and cTnI are unique to myocardial cells and exist in free and bound forms. A small part is soluble and free in the cytoplasm; most of the rest is insoluble and fixed on myofibrils in the form of structural proteins. When the myocardial cell membrane is intact, troponin cannot enter the human blood circulation through the cell membrane, and its quantity in the blood is very low. The free part can enter human blood rapidly only when the integrity of the myocardial cell membrane is damaged. This lays the foundation for the early diagnosis of myocardial injury. BNP is a member of the natriuretic peptide family, which mainly includes atrial natriuretic peptide, BNP, C-type natriuretic peptide, renal natriuretic peptide, and dendroaspis natriuretic peptide. Zahra et al. (50) collected data on 35 patients and found that NT-proBNP or BNP levels increased in all children. Gruber collected data on nine children from New York, and eight were found to have elevated troponin and BNP levels (24). Feldstein et al. reported data on 149 patients with MIS-C with cardiovascular changes in 26 patients; 73% had increased BNP and 50% had increased troponin levels (21). Dufort et al. summarized data on 99 patients who were diagnosed with MIS-C, 74% of whom had elevated troponin levels (51). Huang et al. reported that 12% of patients with COVID-19 in their study were diagnosed with acute myocardial injury, mainly characterized by increased troponin I levels (30). Therefore, elevated BNP or troponin levels in MIS-C patients suggest the existence of myocardial injury.
Arrhythmia
Under normal conditions, the heart beats regularly within a certain range of frequencies. It originates from the atrionector and conducts to the atrium and ventricle in a certain order and rate. When the heart is damaged, the frequency, rhythm, origin site, conduction velocity, and excitation order of cardiac impulses may be abnormal, resulting in arrhythmia. Whittaker collected data on 58 hospitalized children, of whom four had arrhythmias, including complex tachycardia and conduction block (2). Cheung et al. (5) reported that of 17 children with MIS-C in New York, 16 showed ECG abnormalities; 10 had nonspecific ST/T wave abnormalities, 1 had reduced QRS voltage, and 3 had arrhythmia (ventricular premature contraction, ventricular tachycardia, and sinus bradycardia). Waltuch et al. reported the occurrence of tachycardia in patients with MIS-C (54). Shelley et al. reported arrhythmia in 1 of 8 patients (1). Gruber et al. reported data on nine children from New York, six of whom had significant ECG changes. Therefore, when excluding other arrhythmia causes, arrhythmia after myocardial injury should be noted in patients with MIS-C (24).
Coronary aneurysm
Coronary aneurysm refers to the local or segmental abnormal expansion of the coronary artery, which is approximately 1.5–2 times larger than the adjacent normal blood vessel or the largest coronary artery. It is a single or multiple phymatoid change, mainly due to the destruction of the elastic layer of the vascular middle membrane, smooth muscle necrosis, vasodilation, and aneurysm formation. In a study on 9 children in New York, echocardiography showed coronary artery dilatation or aneurysm formation in 6 children. Whittaker et al. studied 58 hospitalized children; 55 underwent echocardiography for the assessment of coronary artery aneurysm and 8 had abnormally dilated coronary arteries (Z-score >2) including 7 with Z-scores greater than 2.5; giant coronary artery aneurysms (Z-score >10) were discovered in two patients (2). Toubiana et al. reported that 5 out of 21 children with MIS-C had moderate coronary artery dilatation in France (3). Zahra et al. described six cases (17%) of coronary artery dilatation, including five with left main artery dilatation and one with right coronary artery dilatation (50). Verdoni et al. reported that 2 of 10 children who were diagnosed with MIS-C in Italy during the epidemic had coronary aneurysms on echocardiography (4). Immune system dysregulation and inflammatory cells and cytokines infiltration in patients with MIS-C leads to vascular destruction, smooth muscle necrosis, and coronary artery dilatation or coronary aneurysm.
Cardiac dysfunction
Cardiac dysfunction refers to impaired ventricular filling and/or ejection fraction caused by various cardiac structural or functional diseases, with cardiac output unable to meet the body's metabolic needs. Based on a literature review, left ventricular systolic dysfunction accounted for a large proportion of cardiac injuries in patients with MIS-C. One study from the UK reported that 75% of children with MIS-C had cardiac dysfunction (1). Rauf et al (55). reported that a 5-year-old Indian boy had left ventricular hypofunction and moderate systolic dysfunction. Verdoni et al. reported that five of ten patients had an ejection fraction of >55% (4). Gema et al. retrospectively analyzed 12 children with SARS-CoV-2 infection and shock; 4 (33%) developed ventricular dysfunction (19). Zahra et al. described left ventricular dysfunction as the main cardiac feature of MIS-C, and persistent diastolic dysfunction as a prominent manifestation of this syndrome. In their study, echocardiography in 35 patients showed decreased left ventricular systolic function on admission, with ejection fraction <30% (10 cases), ejection fraction between 30%–50% (25 cases), left ventricular dysfunction (31 cases), and segmental decreased wall motor function (3 cases); however, right ventricular function was normal in all patients (50). Shubhi et al. studied 33 children with MIS-C; echocardiography showed ventricular ejection fraction <50% in 21 children (65.6%), suggestive of cardiac dysfunction (53).
Hydropericardium
The normal pericardium is a conical serosal sac composed of visceral and parietal layers. The potential cavity between the parietal layer and the visceral pericardium is the pericardial cavity. A small amount of pericardial fluid has a lubricant role, but if liquid in the pericardial cavity exceeds a certain limit, it is referred to as hydropericardium. In serious cases, this can limit cardiac dilation, reduce cardiac compliance, and hinder diastolic filling of the heart. In MIS-C patients, a few children with heart injury were found to have hydropericardium. Zahra et al. and Verdoni et al. also reported hydropericardium in some children with MIS-C (4, 50).
Guo et al. reported that MIS-C patients with basic cardiac disease are more prone to myocardial injury (56). In patients with cardiovascular diseases such as hypertension, coronary heart disease, and cardiomyopathy, viral diseases can further damage myocardial cells through multiple mechanisms and heart damage is more likely, with increased risk of death. Another study by Shao et al. also reported heart injuries in patients with MIS-C; 30% had a history of coronary heart disease and 60% had hypertension, with raised inflammatory markers such as CRP and leukocytes in patients with myocardial injuries (57). The activation and enhanced release of these inflammatory markers can lead to apoptosis or necrosis of myocardial cells and cause cardiac injury.
In conclusion, the myocardial injury caused by MIS-C is diverse. The most prominent manifestations are increased troponin and BNP levels, ECG changes, and echocardiography findings of coronary artery dilation or aneurysm formation. Some serious cases may include ventricular dysfunction, myocarditis, and heart failure. MIS-C can cause acute thrombotic events or malignant arrhythmias. It can also lead to cardiac arrest and sudden cardiac death in asymptomatic patients or those without congenital cardiovascular disease.
Treatment
Antiviral and immunoregulatory therapies can be used for myocardial injury treatment. One can also use cytokines involved in the pathogenesis of biological therapies. Immunoregulatory therapies include intravenous immunoglobulin (IVIG) and corticosteroids. Biological agents include IL-1, IL-6 and TNF-α receptor antagonists. Based on the clinical symptoms and signs of myocardial injury, symptomatic supportive treatment should be administered. Belhadjer et al. (50) reported that early diagnosis and treatment with IVIG and corticosteroids improved the clinical path of multisystem diseases, which may prevent ventricular dysfunction and reduce heart failure severity. Therefore, almost all patients with MIS-C in their study used gamma globulin and corticosteroids in the early stages, which prevented serious cardiac injury, including coronary aneurysm formation and heart failure. For patients with a persistent inflammatory response, biological agents can be used to reduce cardiac injury. Patients with cardiac dysfunction can be treated with vasoactive agents, assisted mechanical ventilation, and veno-arterial extracorporeal membrane oxygenation (VA-ECMO). Few studies have shown good prognosis in patients with MIS-C who were treated with IVIG and corticosteroids together (Table 3). In addition, most children treated with biological agents (including IL-1, IL-6, and TNF-α inhibitors) improved clinically, and were discharged. Furthermore, for patients with shock and decreased circulatory function, symptomatic supportive treatments such as vasoactive agents, mechanical ventilation, and VA-ECMO can be used.
IVIG
IVIG has a strong effect on immune substitution and regulation (58), has anti-inflammatory properties, and can neutralize bacterial toxins (59). IVIG regulates innate and adaptive immunity through various mechanisms. The symptoms of patients with MIS-C who used IVIG can be relieved quickly, due to a significant decrease in cytokines, monocytes, macrophages, neutrophils, and activated T cells, and increased natural killer cells.
Corticosteroids
Corticosteroids affect the survival and activity of immune cells and the expression of inflammatory markers through many molecular mechanisms. Corticosteroids inhibit transcription of proinflammatory cells in the signaling pathway, resulting in reduced release of proinflammatory cytokines (IL-1, TNF-α, IL-6, IL-11, IL-16). It restrains some of the stimulators involved in neutrophils, antigen presenting cells, and lymphocyte activation and differentiation (60, 61). In addition, corticosteroids affect somatic and endothelial cells by downregulating the accumulation of adhesion molecules and neutrophils, and inhibiting platelet adhesion and T and B lymphocytes activity (16, 62, 63). Corticosteroid use is not recommended in the early stages of MIS-C as they might delay virus clearance and increase infection risk (59).
Biological agents
The biological response modifier drug is a new therapeutic agent composed of recombinant human monoclonal antibody or receptor antagonists, including IL-1 receptor antagonists, IL-6 receptor antagonists, and TNF-α receptor antagonists. It has been reported that the application of biological agents can inhibit cytokine storms in patients with MIS-C and improve clinical symptoms, signs, and inflammatory responses.
IL-1 receptor antagonist
Anti-IL-1 antagonist is the first-line biological agent for MIS-C treatment. IL-1 is a pleiotropic cytokine that is activated through the engagement of endothelial cells and other cells involved in the innate immune response. After activation, expression of IL-6, IL-8, TNF, IL-10, and vascular endothelial cell adhesion molecules increases through the hydroxybenzoyl mechanism (16). IL-1 is the main immunomodulator of inflammatory cytokine storms. IL-1 receptor antagonists can downregulate the inflammatory response secondary to IL-1, decrease adhesion molecules on endothelial cells, and inhibit vascular wall damage caused by neutrophil activation and degranulation (16). In a clinical study of Kawasaki disease, anakinra, an IL-1 receptor antagonist, was found to reduce coronary aneurysm development and myocarditis (64). In sample data from nine patients, anakinra was found to improve clinical manifestations and laboratory investigations effectively (65). In another study, it was found that the application of anakinra could effectively reduce patients' demand for assisted mechanical ventilation and decrease mortality in the intensive care unit (66).
IL-6 receptor antagonist
IL-6 is the main proinflammatory cytokine, leading to increased levels of cytokines such as IL-2, IL-8, IL-10, and TNF-α. Data suggest that the IL-6 pathway may play an important role in the inflammatory response of patients with COVID-19 (59). Therefore, it may be a potential target for immunotherapy in patients with MIS-C. Tocilizumab is an IL-6 receptor antagonist that downregulates IL-6's paracrine and autocrine functions and prevents inflammatory response events, including CD4 + T cells differentiation and T regulatory cell inhibition (67). Tocilizumab is approved for systemic juvenile idiopathic arthritis in children aged 2 years and older. This disease has a similar cytokine cascade response to MIS-C; therefore, the study of tocilizumab in the treatment of MIS-C patients is promising (68, 69). One study showed that during tocilizumab administration, 75% of patients with severe COVID-19 had fever cessation, lower oxygen requirements, and significantly improved computed tomography imaging changes (70). Early clinical reports showed that after the application of tocilizumab, 20 severely ill patients had fever subside within 1 min, and 95% of them recovered well enough to be discharged within 2 weeks. Guo et al. showed that tocilizumab treatment can improve the excessively activated inflammatory immune response in children and enhance the antiviral immune response regulated by B cells and CD8 + T cells (56). Luo et al. (71) suggested repeated treatment with a low-dose IL-6 receptor blocker to obtain the greatest effect. Zain et al. (72) reported that patients who received tocilizumab treatment had marked improvements in oxyhemoglobin saturation, lung imaging, ventilator support time, CRP, ferritin, and LDH, among other factors. In summary, IL-6 receptor antagonists are a promising target for MIS-C treatment, and further research should be conducted on their efficacy and safety.
TNF-α receptor antagonist
TNF-α is considered to be a key participant in the production of proinflammatory cytokines and the activation of platelets and is involved in the occurrence of many chronic autoinflammatory diseases. Since TNF-α plays an important role in the activation of the cytokine pathway, it is also a key biological target (73). Infliximab is a TNF-α antagonist that can be used to treat patients with MIS-C. As TNF-α antagonists are an effective treatment for Crohn disease, some studies have shown some success in treating patients with MIS-C and Crohn disease at the same time (74). There is still a lack of experience with the combined use of anti-IL-1 and anti-TNF-α. Future studies should focus on determining whether drug combinations maximize treatment efficacy while minimizing side effects.
Targeted blocking drugs for ACE2 receptor
When the virus invades, the S protein on the virus surface binds to ACE2 on the surface of the target cell. In view of this mechanism, it is possible to study targeted blocking drugs for the S protein ACE2 receptor. ACE2 derivatives (P4, P5, and P6) are now in commercial use as they are effective in blocking SARS-CoV invasion. However, the efficacy of these drugs is still unclear and many drugs are in different phases of clinical trials. In addition, we should also consider the physiological changes to RAS induced by these drugs, which may lead to secondary tissue injury. All these factors require further study.
Conclusion
The clinical manifestations of myocardial injury caused by MIS-C are diverse. This review article summarizes the clinical manifestations, laboratory investigations, and treatments for cardiac injury. This may help clinicians in early diagnosis and treatment to reduce complications, severity of heart injury, and mortality. Studies on the treatment of this disease is very limited. For this reason, the therapeutic effect of the treatments on cardiac injury cannot be assessed. In addition, the long-term consequences of cardiac damage due to MIS-C disease are unknown. Some of the treatments described in this article need further study.
Author contributions
SS: Writing – review & editing, Writing – original draft. WH: Writing – review & editing, Investigation. XC: Writing – review & editing, Writing – original draft, Validation. YR: Writing – review & editing, Investigation. YL: Writing – review & editing, Investigation. JS: Writing – review & editing, Investigation. TZ: Writing – review & editing, Investigation. HZ: Writing – review & editing, Conceptualization. MW: Writing – review & editing, Data curation. YW: Writing – review & editing, Resources. FZ: Writing – review & editing, Software. RJ: Writing – review & editing, Supervision. YL: Writing – review & editing, Supervision. HZ: Writing – review & editing, Supervision. GL: Writing – review & editing, Supervision.
Funding
The author(s) declare that no financial support was received for the research, authorship, and/or publication of this article.
Conflict of Interest
The authors declare that the research was conducted in the absence of any commercial or financial relationships that could be construed as a potential conflict of interest.
Publisher's note
All claims expressed in this article are solely those of the authors and do not necessarily represent those of their affiliated organizations, or those of the publisher, the editors and the reviewers. Any product that may be evaluated in this article, or claim that may be made by its manufacturer, is not guaranteed or endorsed by the publisher.
References
1. Riphagen S, Gomez X, Gonzalez-Martinez C, Wilkinson N, Theocharis P. Hyperinflammatory shock in children during COVID-19 pandemic. Lancet. (2020) 395:1607–8. doi: 10.1016/S0140-6736(20)31094-1
2. Whittaker E, Bamford A, Kenny J, Kaforou M, Jones CE, Shah P, et al. Clinical characteristics of 58 children with a pediatric inflammatory multisystem syndrome temporally associated with SARS-CoV-2. JAMA. (2020) 324:259–69. doi: 10.1001/jama.2020.10369
3. Toubiana J, Poirault C, Corsia A, Bajolle F, Fourgeaud J, Angoulvant F, et al. Kawasaki-like multisystem inflammatory syndrome in children during the COVID-19 pandemic in Paris, France: prospective observational study. BMJ. (2020) 369:m2094. doi: 10.1136/bmj.m2094
4. Verdoni L, Mazza A, Gervasoni A, Martelli L, Ruggeri M, Ciuffreda M, et al. An outbreak of severe Kawasaki-like disease at the Italian epicentre of the SARS-CoV-2 epidemic: an observational cohort study. Lancet. (2020) 395:1771–8. doi: 10.1016/S0140-6736(20)31103-X
5. Cheung EW, Zachariah P, Gorelik M, Boneparth A, Kernie SG, Orange JS, et al. Multisystem inflammatory syndrome related to COVID-19 in previously healthy children and adolescents in New York City. JAMA. (2020) 324:294–6. doi: 10.1001/jama.2020.10374
6. Woo PC, Huang Y, Lau SK, Yuen KY. Coronavirus genomics and bioinformatics analysis. Viruses. (2010) 2:1804–20. doi: 10.3390/v2081803
7. Yan R, Zhang Y, Li Y, Xia L, Guo Y, Zhou Q. Structural basis for the recognition of SARS-CoV-2 by full-length human ACE2. Science. (2020) 367:1444–8. doi: 10.1126/science.abb2762
8. Belouzard S, Chu VC, Whittaker GR. Activation of the SARS coronavirus spike protein via sequential proteolytic cleavage at two distinct sites. Proc Natl Acad Sci U S A. (2009) 106:5871–6. doi: 10.1073/pnas.0809524106
9. Zhang H, Penninger JM, Li Y, Zhong N, Slutsky AS. Angiotensin-converting enzyme 2 (ACE2) as a SARS-CoV-2 receptor: molecular mechanisms and potential therapeutic target. Intensive Care Med. (2020) 46:586–90. doi: 10.1007/s00134-020-05985-9
10. Donoghue M, Hsieh F, Baronas E, Godbout K, Gosselin M, Stagliano N, et al. A novel angiotensin-converting enzyme-related carboxypeptidase (ACE2) converts angiotensin I to angiotensin 1-9. Circ Res. (2000) 87:E1–9. doi: 10.1161/01.RES.87.5.e1
11. Zhang H, Wada J, Hida K, Tsuchiyama Y, Hiragushi K, Shikata K, et al. Collectrin, a collecting duct-specific transmembrane glycoprotein, is a novel homolog of ACE2 and is developmentally regulated in embryonic kidneys. J Biol Chem. (2001) 276:17132–9. doi: 10.1074/jbc.M006723200
12. Hamming I, Timens W, Bulthuis ML, Lely AT, Navis G, van Goor H. Tissue distribution of ACE2 protein, the functional receptor for SARS coronavirus. A first step in understanding SARS pathogenesis. J Pathol. (2004) 203:631–7. doi: 10.1002/path.1570
13. Li W, Moore MJ, Vasilieva N, Sui J, Wong SK, Berne MA, et al. Angiotensin-converting enzyme 2 is a functional receptor for the SARS coronavirus. Nature. (2003) 426:450–4. doi: 10.1038/nature02145
14. Walls AC, Park YJ, Tortorici MA, Wall A, McGuire AT, Veesler D. Structure, function, and antigenicity of the SARS-CoV-2 spike glycoprotein. Cell. (2020) 181:281–92.e286. doi: 10.1016/j.cell.2020.02.058
15. South AM, Diz DI, Chappell MC. COVID-19, ACE2, and the cardiovascular consequences. Am J Physiol Heart Circ Physiol. (2020) 318:H1084–90. doi: 10.1152/ajpheart.00217.2020
16. McMurray JC, May JW, Cunningham MW, Jones OY. Multisystem inflammatory syndrome in children (MIS-C), a post-viral myocarditis and systemic vasculitis-A critical review of its pathogenesis and treatment. Front Pediatr. (2020) 8:626182. doi: 10.3389/fped.2020.626182
17. Dhar D, Dey T, Samim MM, Padmanabha H, Chatterjee A, Naznin P, et al. Systemic inflammatory syndrome in COVID-19-SISCoV study: systematic review and meta-analysis. Pediatr Res. (2022) 91:1334–49. doi: 10.1038/s41390-021-01545-z
18. Brodsky NN, Ramaswamy A, Lucas CL. The mystery of MIS-C post-SARS-CoV-2 infection. Trends Microbiol. (2020) 28:956–8. doi: 10.1016/j.tim.2020.10.004
19. Caro-Patón GL, de Azagra-Garde AM, García-Salido A, Cabrero-Hernández M, Tamariz A, Nieto-Moro M. Shock and myocardial injury in children with multisystem inflammatory syndrome associated with SARS-CoV-2 infection: what we know. Case series and review of the literature. J Intensive Care Med. (2021) 36:392–403. doi: 10.1177/0885066620969350
20. Kabeerdoss J, Pilania RK, Karkhele R, Kumar TS, Danda D, Singh S. Severe COVID-19, multisystem inflammatory syndrome in children, and Kawasaki disease: immunological mechanisms, clinical manifestations and management. Rheumatol Int. (2021) 41:19–32. doi: 10.1007/s00296-020-04749-4
21. Feldstein LR, Rose EB, Horwitz SM, Collins JP, Newhams MM, Son MBF, et al. Multisystem inflammatory syndrome in U.S. children and adolescents. N Engl J Med. (2020) 383:334–46. doi: 10.1056/NEJMoa2021680
22. Jiang L, Tang K, Levin M, Irfan O, Morris SK, Wilson K, et al. COVID-19 and multisystem inflammatory syndrome in children and adolescents. Lancet Infect Dis. (2020) 20:e276–88. doi: 10.1016/S1473-3099(20)30651-4
23. Belot A, Antona D, Renolleau S, Javouhey E, Hentgen V, Angoulvant F, et al. SARS-CoV-2-related paediatric inflammatory multisystem syndrome, an epidemiological study, France, 1 March to 17 May 2020. Euro Surveill. (2020) 25(2):2001010. doi: 10.2807/1560-7917.ES.2020.25.22.2001010
24. Gruber CN, Patel RS, Trachtman R, Lepow L, Amanat F, Krammer F, et al. Mapping systemic inflammation and antibody responses in multisystem inflammatory syndrome in children (MIS-C). Cell. (2020) 183:982–95.e914. doi: 10.1016/j.cell.2020.09.034
25. Gruber C, Patel R, Trachman R, Lepow L, Amanat F, Krammer F, et al. Mapping systemic inflammation and antibody responses in multisystem inflammatory syndrome in children (MIS-C). medRxiv [preprint]. (2020).
26. Oudit GY, Kassiri Z, Jiang C, Liu PP, Poutanen SM, Penninger JM, et al. SARS-coronavirus modulation of myocardial ACE2 expression and inflammation in patients with SARS. Eur J Clin Investig. (2009) 39:618–25. doi: 10.1111/j.1365-2362.2009.02153.x
27. Zhu N, Zhang D, Wang W, Li X, Yang B, Song J, et al. A novel coronavirus from patients with pneumonia in China, 2019. N Engl J Med. (2020) 382:727–33. doi: 10.1056/NEJMoa2001017
28. Xu X, Chen P, Wang J, Feng J, Zhou H, Li X, et al. Evolution of the novel coronavirus from the ongoing Wuhan outbreak and modeling of its spike protein for risk of human transmission. Sci China Life Sci. (2020) 63:457–60. doi: 10.1007/s11427-020-1637-5
29. Siripanthong B, Nazarian S, Muser D, Deo R, Santangeli P, Khanji MY, et al. Recognizing COVID-19-related myocarditis: the possible pathophysiology and proposed guideline for diagnosis and management. Heart Rhythm. (2020) 17:1463–71. doi: 10.1016/j.hrthm.2020.05.001
30. Huang C, Wang Y, Li X, Ren L, Zhao J, Hu Y, et al. Clinical features of patients infected with 2019 novel coronavirus in Wuhan, China. Lancet. (2020) 395:497–506. doi: 10.1016/S0140-6736(20)30183-5
31. Hedrich CM. COVID-19—considerations for the paediatric rheumatologist. Clin Immunol. (2020) 214:108420. doi: 10.1016/j.clim.2020.108420
32. McGonagle D, Sharif K, O'Regan A, Bridgewood C. The role of cytokines including interleukin-6 in COVID-19 induced pneumonia and macrophage activation syndrome-like disease. Autoimmun Rev. (2020) 19:102537. doi: 10.1016/j.autrev.2020.102537
33. Atkin-Smith GK, Duan M, Chen W, Poon IKH. The induction and consequences of influenza A virus-induced cell death. Cell Death Dis. (2018) 9:1002. doi: 10.1038/s41419-018-1035-6
34. Zhang W, Zhao Y, Zhang F, Wang Q, Li T, Liu Z, et al. The use of anti-inflammatory drugs in the treatment of people with severe coronavirus disease 2019 (COVID-19): the perspectives of clinical immunologists from China. Clin Immunol. (2020) 214:108393. doi: 10.1016/j.clim.2020.108393
35. Li H, Liu L, Zhang D, Xu J, Dai H, Tang N, et al. SARS-CoV-2 and viral sepsis: observations and hypotheses. Lancet. (2020) 395:1517–20. doi: 10.1016/S0140-6736(20)30920-X
36. Liu J, Li S, Liu J, Liang B, Wang X, Wang H, et al. Longitudinal characteristics of lymphocyte responses and cytokine profiles in the peripheral blood of SARS-CoV-2 infected patients. EBioMedicine. (2020) 55:102763. doi: 10.1016/j.ebiom.2020.102763
37. Sardu C, Gambardella J, Morelli MB, Wang X, Marfella R, Santulli G. Hypertension, thrombosis, kidney failure, and diabetes: is COVID-19 an endothelial disease? A comprehensive evaluation of clinical and basic evidence. J Clin Med. (2020) 9:1417. doi: 10.3390/jcm9051417
38. Varga Z, Flammer AJ, Steiger P, Haberecker M, Andermatt R, Zinkernagel AS, et al. Endothelial cell infection and endotheliitis in COVID-19. Lancet. (2020) 395:1417–8. doi: 10.1016/S0140-6736(20)30937-5
39. Carter MJ, Fish M, Jennings A, Doores KJ, Wellman P, Seow J, et al. Peripheral immunophenotypes in children with multisystem inflammatory syndrome associated with SARS-CoV-2 infection. Nat Med. (2020) 26:1701–7. doi: 10.1038/s41591-020-1054-6
40. Consiglio CR, Cotugno N, Sardh F, Pou C, Amodio D, Rodriguez L, et al. The immunology of multisystem inflammatory syndrome in children with COVID-19. Cell. (2020) 183:968–81.e967. doi: 10.1016/j.cell.2020.09.016
41. Vella LA, Giles JR, Baxter AE, Oldridge DA, Diorio C, Kuri-Cervantes L, et al. Deep immune profiling of MIS-C demonstrates marked but transient immune activation compared to adult and pediatric COVID-19. Sci Immunol. (2021) 6:eabf7570. doi: 10.1126/sciimmunol.abf7570
42. Cao X. COVID-19: immunopathology and its implications for therapy. Nat Rev Immunol. (2020) 20:269–70. doi: 10.1038/s41577-020-0308-3
43. Wu C, Chen X, Cai Y, Xia J, Zhou X, Xu S, et al. Risk factors associated with acute respiratory distress syndrome and death in patients with coronavirus disease 2019 pneumonia in Wuhan, China. JAMA Intern Med. (2020) 180:934–43. doi: 10.1001/jamainternmed.2020.0994
44. Li H, Xiang X, Ren H, Xu L, Zhao L, Chen X, et al. Serum amyloid A is a biomarker of severe coronavirus disease and poor prognosis. J Infect. (2020) 80:646–55. doi: 10.1016/j.jinf.2020.03.035
45. Jamilloux Y, Henry T, Belot A, Viel S, Fauter M, El Jammal T, et al. Should we stimulate or suppress immune responses in COVID-19? Cytokine and anti-cytokine interventions. Autoimmun Rev. (2020) 19:102567. doi: 10.1016/j.autrev.2020.102567
46. Channappanavar R, Fehr AR, Vijay R, Mack M, Zhao J, Meyerholz DK, et al. Dysregulated type I interferon and inflammatory monocyte-macrophage responses cause lethal pneumonia in SARS-CoV-infected mice. Cell Host Microbe. (2016) 19:181–93. doi: 10.1016/j.chom.2016.01.007
47. Law HK, Cheung CY, Ng HY, Sia SF, Chan YO, Luk W, et al. Chemokine up-regulation in SARS-coronavirus-infected, monocyte-derived human dendritic cells. Blood. (2005) 106:2366–74. doi: 10.1182/blood-2004-10-4166
48. Lau SKP, Lau CCY, Chan KH, Li CPY, Chen H, Jin DY, et al. Delayed induction of proinflammatory cytokines and suppression of innate antiviral response by the novel Middle East respiratory syndrome coronavirus: implications for pathogenesis and treatment. J Gen Virol. (2013) 94:2679–90. doi: 10.1099/vir.0.055533-0
49. Cheng MH, Zhang S, Porritt RA, Arditi M, Bahar I. An insertion unique to SARS-CoV-2 exhibits superantigenic character strengthened by recent mutations. bioRxiv [preprint]. (2020).
50. Belhadjer Z, Méot M, Bajolle F, Khraiche D, Legendre A, Abakka S, et al. Acute heart failure in multisystem inflammatory syndrome in children in the context of global SARS-CoV-2 pandemic. Circulation. (2020) 142:429–36. doi: 10.1161/CIRCULATIONAHA.120.048360
51. Dufort EM, Koumans EH, Chow EJ, Rosenthal EM, Muse A, Rowlands J, et al. Multisystem inflammatory syndrome in children in New York state. N Engl J Med. (2020) 383:347–58. doi: 10.1056/NEJMoa2021756
52. Dolinger MT, Person H, Smith R, Jarchin L, Pittman N, Dubinsky MC, et al. Pediatric crohn disease and multisystem inflammatory syndrome in children (MIS-C) and COVID-19 treated with infliximab. J Pediatr Gastroenterol Nutr. (2020) 71:153–5. doi: 10.1097/MPG.0000000000002809
53. Kaushik S, Aydin SI, Derespina KR, Bansal PB, Kowalsky S, Trachtman R, et al. Multisystem inflammatory syndrome in children associated with severe acute respiratory syndrome coronavirus 2 infection (MIS-C): a multi-institutional study from New York City. J Pediatr. (2020) 224:24–9. doi: 10.1016/j.jpeds.2020.06.045
54. Waltuch T, Gill P, Zinns LE, Whitney R, Tokarski J, Tsung JW, et al. Features of COVID-19 post-infectious cytokine release syndrome in children presenting to the emergency department. Am J Emerg Med. (2020) 38:2246.e2243–46. doi: 10.1016/j.ajem.2020.05.058
55. Rauf A, Vijayan A, John ST, Krishnan R, Latheef A. Multisystem inflammatory syndrome with features of atypical Kawasaki disease during COVID-19 pandemic. Indian J Pediatr. (2020) 87:745–7. doi: 10.1007/s12098-020-03357-1
56. Guo T, Fan Y, Chen M, Wu X, Zhang L, He T, et al. Cardiovascular implications of fatal outcomes of patients with coronavirus disease 2019 (COVID-19). JAMA Cardiol. (2020) 5:811–8. doi: 10.1001/jamacardio.2020.1017
57. Shi S, Qin M, Shen B, Cai Y, Liu T, Yang F, et al. Association of cardiac injury with mortality in hospitalized patients with COVID-19 in Wuhan, China. JAMA Cardiol. (2020) 5:802–10. doi: 10.1001/jamacardio.2020.0950
58. Lee PY, Day-Lewis M, Henderson LA, Friedman KG, Lo J, Roberts JE, et al. Distinct clinical and immunological features of SARS-CoV-2-induced multisystem inflammatory syndrome in children. J Clin Invest. (2020) 130:5942–50. doi: 10.1172/JCI141113
59. Jolles S, Sewell WA, Misbah SA. Clinical uses of intravenous immunoglobulin. Clin Exp Immunol. (2005) 142:1–11. doi: 10.1111/j.1365-2249.2005.02834.x
60. Song Y, Zhang M, Yin L, Wang K, Zhou Y, Zhou M, et al. COVID-19 treatment: close to a cure? A rapid review of pharmacotherapies for the novel coronavirus (SARS-CoV-2). Int J Antimicrob Agents. (2020) 56:106080. doi: 10.1016/j.ijantimicag.2020.106080
61. Williams DM. Clinical pharmacology of corticosteroids. Respir Care. (2018) 63:655–70. doi: 10.4187/respcare.06314
62. D'Acquisto F, May MJ, Ghosh S. Inhibition of nuclear factor kappa B (NF-B): an emerging theme in anti-inflammatory therapies. Mol Interv. (2002) 2:22–35. doi: 10.1124/mi.2.1.22
63. Ferrara G, Petrillo MG, Giani T, Marrani E, Filippeschi C, Oranges T, et al. Clinical use and molecular action of corticosteroids in the pediatric age. Int J Mol Sci. (2019) 20:444. doi: 10.3390/ijms20020444
64. Liverani E, Banerjee S, Roberts W, Naseem KM, Perretti M. Prednisolone exerts exquisite inhibitory properties on platelet functions. Biochem Pharmacol. (2012) 83:1364–73. doi: 10.1016/j.bcp.2012.02.006
65. Gorelik M, Lee Y, Abe M, Andrews T, Davis L, Patterson J, et al. IL-1 receptor antagonist, anakinra, prevents myocardial dysfunction in a mouse model of Kawasaki disease vasculitis and myocarditis. Clin Exp Immunol. (2019) 198:101–10. doi: 10.1111/cei.13314
66. Aouba A, Baldolli A, Geffray L, Verdon R, Bergot E, Martin-Silva N, et al. Targeting the inflammatory cascade with anakinra in moderate to severe COVID-19 pneumonia: case series. Ann Rheum Dis. (2020) 79:1381–2. doi: 10.1136/annrheumdis-2020-217706
67. Huet T, Beaussier H, Voisin O, Jouveshomme S, Dauriat G, Lazareth I, et al. Anakinra for severe forms of COVID-19: a cohort study. Lancet Rheumatol. (2020) 2:e393–400. doi: 10.1016/S2665-9913(20)30164-8
68. Sheppard M, Laskou F, Stapleton PP, Hadavi S, Dasgupta B. Tocilizumab (Actemra). Hum Vaccin Immunother. (2017) 13:1972–88. doi: 10.1080/21645515.2017.1316909
69. Ye Q, Wang B, Mao J. The pathogenesis and treatment of the 'Cytokine storm’ in COVID-19. J Infect. (2020) 80:607–13. doi: 10.1016/j.jinf.2020.03.037
70. Lee JJY, Schneider R. Systemic juvenile idiopathic arthritis. Pediatr Clin N Am. (2018) 65:691–709. doi: 10.1016/j.pcl.2018.04.005
71. Xu X, Han M, Li T, Sun W, Wang D, Fu B, et al. Effective treatment of severe COVID-19 patients with tocilizumab. Proc Natl Acad Sci U S A. (2020) 117:10970–5. doi: 10.1073/pnas.2005615117
72. Luo P, Liu Y, Qiu L, Liu X, Liu D, Li J. Tocilizumab treatment in COVID-19: a single center experience. J Med Virol. (2020) 92:814–8. doi: 10.1002/jmv.25801
73. Zain Mushtaq M, Bin Zafar Mahmood S, Jamil B, Aziz A, Ali SA. Outcome of COVID-19 patients with use of tocilizumab: a single center experience. Int Immunopharmacol. (2020) 88:106926. doi: 10.1016/j.intimp.2020.106926
Keywords: severe acute respiratory syndrome coronavirus 2, children, multisystem inflammatory syndrome, cardiac injury, coronavirus disease 2019
Citation: Su S, Hu W, Chen X, Ren Y, Lu Y, Shi J, Zhang T, Zhang H, Wang M, Wang Y, Zhao F, Jin R, Liu Y, Zhang H and Liu G (2024) Cardiac injury progression in children with multisystem inflammatory syndrome associated with SARS-CoV-2 infection: a review. Front. Pediatr. 12:1348016. doi: 10.3389/fped.2024.1348016
Received: 4 December 2023; Accepted: 22 February 2024;
Published: 6 March 2024.
Edited by:
Nazmi Narin, Izmir Katip Celebi University, TürkiyeReviewed by:
Süleyman Sunkak, Kayseri Education and Research Hospital, TürkiyeEngin Gerçeker, Izmir Tepecik Training and Research Hospital, Türkiye
© 2024 Su, Hu, Chen, Ren, Lu, Shi, Zhang, Zhang, Wang, Wang, Zhao, Jin, Liu, Zhang and Liu. This is an open-access article distributed under the terms of the Creative Commons Attribution License (CC BY). The use, distribution or reproduction in other forums is permitted, provided the original author(s) and the copyright owner(s) are credited and that the original publication in this journal is cited, in accordance with accepted academic practice. No use, distribution or reproduction is permitted which does not comply with these terms.
*Correspondence: Guohua Liu liuguohua0204@163.com
†These authors have contributed equally to this work and share first authorship
Abbreviations BNP, brain natriuretic peptide; CDC, Centers for Disease Control and Prevention; COVID-19, coronavirus disease 2019; CRP, C-reactive protein; CTD, carboxy-terminal domain; ECG, electrocardiogram; ESR, erythrocyte sedimentation rate; HLA, human leukocyte antigen; IFN, interferon; IL, interleukin; IVIG, intravenous immunoglobulin; LDH, lactate dehydrogenase; MIS-C, multisystem inflammatory syndrome in children; NT-proBNP, N-terminal pro brain natriuretic peptide; PCR, polymerase chain reaction; PD, peptidase domain; RAS, renin-angiotensin system; RBD, receptor-binding domain; SARS-CoV, severe acute respiratory syndrome coronavirus; SARS-CoV-2, severe acute respiratory syndrome coronavirus 2; TNF, tumor necrosis factor; VA-ECMO, veno-arterial extracorporeal membrane oxygenation; WHO, World Health Organization.