- 1Department of NICU, The Children’s Hospital, Zhejiang University School of Medicine, National Clinical Research Center for Child Health, Hangzhou, China
- 2Department of National Clinical Research Center, The Children’s Hospital, Zhejiang University School of Medicine, National Clinical Research Center for Child Health, Hangzhou, China
Purpose: To investigate the dynamic changes in the intestinal microbiota in preterm infants with necrotizing enterocolitis (NEC) before and after treatment via a prospective case-control study.
Methods: Preterm infants with NEC and preterm infants with similar age and weight (control group) were enrolled in this study. They were divided into NEC_Onset (diagnosis time), NEC_Refeed (refeed time), NEC_FullEn (full enteral nutrition time), Control_Onset, and Control_FullEn groups according to the time of the fecal material collected. Except for basic clinical information, fecal specimens of the infants were obtained as well at indicated times for 16S rRNA gene sequencing. All infants were followed up after discharge from the NICU, and the growth data of the corrected age of 12 months were acquired from the electronic outpatient system and telephonic interviews.
Results: A total of 13 infants with NEC and 15 control infants were enrolled. A gut microbiota analysis showed that the Shannon and Simpson indices were lower in the NEC_FullEn group than in the Control_FullEn group (p < .05). Methylobacterium, Clostridium_butyricum, and Acidobacteria were more abundant in infants with NEC during diagnosis. Methylobacterium and Acidobacteria were remained plentiful in the NEC group until the end of treatment. These bacteria species were significantly positively correlated with CRP and negatively correlated with platelet count. The rate of delayed growth was higher in the NEC group than in the control group (25% vs. 7.1%) at 12 months of corrected age, but there was no significant difference. In addition, the pathways of synthesis and degradation of ketone bodies were more active in the NEC subgroups, including both the NEC_Onset group and the NEC_FullEn group. The pathway of sphingolipid metabolism was more active in the Control_FullEn group.
Conclusion: Even after reaching the full enteral nutrition period, alpha diversity in infants with NEC who underwent surgery was lower than that in the control group infants. It may take more time to reestablish the normal gut flora of NEC infants after surgery. The pathways of the synthesis and degradation of ketone bodies and sphingolipid metabolism might be related to the pathogenesis of NEC and physical development after the occurrence of NEC.
Introduction
Neonatal necrotizing enterocolitis (NEC) is a life-threatening disease, the typical characteristic of which is intestinal inflammatory necrosis. Most NEC cases are preterm infants (1, 2). The occurring frequency of NEC is close to 7% in very-low-birth-weight infants, and their mortality rates reach one-third. Surgical intervention is done in approximately 20%–40% of NEC infants (3). Also, NEC infants undergoing surgery may experience worse outcomes such as short bowel syndrome and neurodevelopmental dysplasia (4, 5).
The exact pathogenesis of NEC remains undetermined. However, it may involve unusual intestinal bacterial colonization as well as overinflammatory response to the intestinal microbiota (6, 7). Different from the conventional culture-based technique, the gene sequencing of the 16S bacterial ribosomal RNA (16S rRNA) can provide a more detailed spectrum of the human intestinal bacteria (8). Previous studies compared the bacterial abundance between healthy preterm infants and NEC patients. However, most of these studies focused only on the microbiota changes that occurred during the period in which NEC also occurred; in addition, these data did not lead to a unified conclusion (9–12). Some studies focused on the gut microbiota (GM) for surgical NEC. Stewart et al. (13) showed that preterm infants with NEC had a lower diversity and a higher phylum-level Proteobacteria compared with spontaneous isolated perforation using formalin-fixed paraffin-embedded tissue. Correa et al. (14) reported that surgical NEC was associated with a distinct microbiome, which was characterized by low diversity, a higher abundance of Staphylococcus, and Clostridium_sensu_stricto when compared with infants who received intestinal surgery without NEC. Different from these two researchers, Brower-Sinning et al. (15) found that no significant difference was observed in the mean Shannon diversity index (SDI) of NEC and non-NEC groups. They also concluded that NEC and non-NEC subjects had high interindividual variability and an abundance of opportunistic pathogens.
Different from previous studies, we designed this prospective case-control study to examine intestinal microbiota alteration in NEC between the time of diagnosis and the end of treatment. Thus, the purpose of this study is not only to investigate the abundance, composition, and distribution of the intestinal microbiota in NEC infants, but also to observe the alterations in the intestinal bacterial spectrum during treatment to provide scientific data for clinical pediatricians for NEC treatment.
Methods
Study design and patients
It was a prospective monocentric case-control cohort study conducted from June 2018 to June 2020. This project was authorized by the Ethics Committee of the Children's Hospital of Zhejiang University School of Medicine (No.2021-IRB-210).
The inclusion criteria of the NEC group were as follows:
1. The gestational age (GA) of infants was required to be ≤34 weeks and the birth weight (BW) of infants should be ≤2000 g.
2. Infants who were diagnosed with NEC at Bell's stage II or III based on the modified Bell's staging (16).
3. Infants who received surgical intervention in the form of either enterostomy or anastomosis.
NICU physicians invited neonatal surgeons for consultations and then made a collective decision on surgical intervention. The surgical indication of NEC was the presence of pneumoperitoneum (Bell stage IIIa) and persistent ileus or abdominal distension (persistent Bell stage IIb > 24 h), coupled with deteriorating clinical and biochemical statuses (e.g., either shock or a decrease in platelet/neutrophil count, or both, or persistent metabolic acidosis) (3).
The exclusion criteria of the NEC group were as follows:
1. Infants who had serious malformations, including congenital intestinal malformations.
2. Infants who did not have complete clinical data and/or failed to collect or store samples by following the necessary procedures.
The inclusion criteria of the control group were as follows:
Infants whose GA and BW were ≤34 weeks and ≤2000 g, without either NEC or congenital intestinal malformations, were included as controls.
Groups and fecal sample gathering
Fecal samples were gathered at three time points for the NEC group: NEC diagnosis or surgery at acute phase, 24 h within refeed after surgery, and the day of achieving full enteral nutrition. Thus, NEC groups were divided into three subgroups based on the fecal sample collection, the NEC_Onset group, the NEC_Refeed group, and the NEC_FullEn group, respectively.
Because NEC usually occurred 2 weeks after birth, the first time that the sample was collected for the control group was during this period. The second-time sample collection for this group was on the day of achieving full enteral nutrition. Thus, the controls were divided into the Control_Onset and the Control_FullEn groups as well.
The fecal samples were obtained by using disposable sterilized spatulas from the diaper during diaper exchange or from the ostomy bag of the infants who received ostomy. The collected samples were then immediately stored in a −80°C freezer until they were sent to Novogene Co. Ltd. (Headquarters), Beijing, China, for 16S rRNA analysis.
Data collection and definition
The data pertaining to hospitalization (including demographics, management or treatment, and laboratory examination) were collected from the patient charts or electronic systems, and all infants were followed up in the preterm infant outpatient department and by telephonic interviews after discharge. Growth parameters such as weight, height, and head circumference (HC) were obtained from clinical medical records or from their parents.
Growth delay was defined as weight, height, or HC less than 10 percentile at 12 months of corrected age, using WHO Child Growth Standards (2006) (17). Those infants who underwent a surgical treatment and achieved full enteral nutrition after treatment were said to have received successful clinical cure.
Microbiota analysis
Genomic DNA was isolated by using CTAB/SDS technology, purified on a 1% agarose gel, and adjusted to a concentration of 1 ng/μl by using sterile water. The 16S rRNAs were amplified with V4 region primers (515F and 806R) with barcode. PCR was reacted in a 30 μl mixture consisting of a 15 μl Phusion R High-Fidelity PCR Master Mix (New England Biolabs, Ipswich, MA, USA), 0.2 μM of 515F and 806R primers, and approximately 10 ng of reversed DNA. Thermal cycling was done for 1 min at 98°C, repeated 30 cycles at 98°C for 10 s, at 50°C for 30 s, and at 72°C for 30 s, and finally heated at 72°C for 5 min to terminate the reaction. The PCR-reacted mixture was then diluted with a 30 μl loading buffer that contained SYB green and isolated on a 2% agarose gel by electrophoresis. The PCR band (PCR products) was cut off and mixed with an equal volume of gel extraction buffer and purified with a PCR purification kit, GeneJETTM Gel Extraction Kit (Thermo Scientific, Waltham, MA, USA). Next, Ion Plus Fragment Library Kit 48 rxns (Thermo Scientific) was employed to prepare the sequencing libraries, the quality of which was analyzed on a Qubit@2.0 Fluorometer (Thermo Scientific). Lastly, the libraries were sequenced on the Ion S5TM XL System to generate single-end reads with 400–600 bp.
For amplicon sequences, raw data were processed using Qiime2 pipeline (2020 version) (18). FastQC was initially applied for quality evaluation. Dada2 (19) was used to denoise sequences and generate ASVs (amplicon sequence variants). ASVs were then mapped by using the Silva 132 database with 99% identity for taxonomy annotation. PICRUSt2 (2.2.0_b) (20) was further applied for predicting metagenome functions.
Statistical analysis
All clinical data analyses were performed with SPSS software (IBM-SPSS, Chicago, IL, USA; version 16.0). Based on the distribution pattern, skewed or otherwise, continuous variable data were summarized as the average ± standard deviation (SD). Comparisons of continuous variables were done with Student’s t-test for the NEC and control groups. The repeated measures ANOVA was applied for making comparisons within the NEC subgroups. Pearson's chi-square test was employed for comparing categorical variables. A value of p < .05 was considered statistically significant.
A statistical analysis of the intestinal microbiota was performed using R software 3.6.3. The Shapiro–Wilk test was used to test for normality. Variables that were normally distributed were examined using ANOVA and Student’s t-test, while those that were not normally distributed were assessed using the Kruskal–Wallis and Mann–Whitney U tests.
The differences in the intestinal microbiota between the two groups were examined by using either the Student’s t-test or the Mann–Whitney U test. ANOVA or the Kruskal–Wallis test was applied for making three group comparisons. The alpha diversity indices (the Shannon Diversity index and Simpson index) were calculated to measure the diversity of the GM using Vegan packages. Spearman’s rank correlation coefficient analysis was employed to evaluate the correlations between the GM and the clinical markers, and it was visualized using a heatmap.
Results
Study design and clinical characteristics
In this study, 13 infants with NEC (NEC group) and 15 similar GA and BW infants (control group) were enrolled for analysis. All 13 infants received surgical intervention, of which 11 underwent enterostomy and the remaining 2 anastomosis. A total of 39 and 30 fecal samples were collected from the NEC and the control groups, respectively, for microbiota analysis. The whole process is represented as a flow diagram (Figure 1).
The mean GA of the NEC group was 30.5 ± 2.4 weeks and that of the control group was 30.6 ± 2.2 weeks, separately. Mean BWs were 1428 ± 327 g and 1256 ± 208 g, respectively, in these two groups. The age of admission in the NEC group was much higher than in the control group. Also, antibiotic use was significantly higher in the NEC group (100.0% vs. 26.7%, p < .001). However, no significant differences in terms of the mean BW, mean GA, gender ratio, delivery mode, or type of feed were observed in these two groups (Table 1).
With regard to the time of fecal collection, there were four infants in the NEC group whose feces were gathered during surgery the first time. The mean interval between NEC diagnosis and surgery was 2(1,4) days. The age of onset for fecal collection was similar in both groups. However, as the time for achieving full enteral nutrition in the NEC group was significantly longer than that in the control group (p = .001), the time for fecal collection in this group was also longer than that in the other group (p = .002).
Surgical findings for the NEC group
Surgical findings are described in Table 2. A perforated bowel was found in three patients and necrosis without perforation was found in the remaining ten. The most frequent site of NEC was located in the ileum. Two patients received enterostomy and the others received primary anastomosis. All underwent a pathological examination (Supplementary Figure S1).
Taking into account the interval between NEC diagnosis and surgery, all infants with NEC were divided into two subgroups, NEC_SI(NEC_short interval) group including patients 3, 6, 9, and 12 and NEC_LI(NEC_long interval) group including the remaining 9.
Clinical data between the NEC and the control groups
Two infants were lost to follow-up after discharge. One belonged to the NEC group and the other to the control group. In addition, one infant with NEC died after discharge before 12 months of corrected age and one developed short bowel syndrome. Therefore, after 12 months of physical development, there were 11 NEC infants and 14 control infants, respectively. The rate of growth delay at the corrected 12 months was higher in the NEC group than in the control (25.0% vs. 7.1%); however, no statistically significant difference was found between the groups (Table 1).
Laboratory tests among the groups
The laboratory tests were also compared among the different subgroups. C-reactive protein (CRP) was obviously higher in the NEC_Onset group than in the Control_Onset group, and the platelet count was significantly lower in the NEC_Onset group (p < .001, p < .001). After reaching the full enteral nutrition period, CRP and the platelet count showed no remarkable differences between the NEC_FullEn and the Control_FullEn subgroups (p > .05). However, CRP and platelet showed significant differences among the three subgroups of NEC.
In addition, the total protein and albumin levels were slightly lower in the NEC_Onset group than in the other groups. Much increased aspartate aminotransferase (AST), alanine aminotransferase (ALT), and direct bilirubin (DB) levels were found in the NEC_FullEn group than in the Control_FullEn group (p < .05) (Table 3).
The GM feature of the NEC group
The species cumulative curve showed an asymptote after a sharp rise and tended to be flat, indicating that the sequencing results were adequate to reflect the diversity in the sample (Supplementary Figure S2). Furthermore, the samples were examined by using principal coordinate analysis (PCoA), which is based on the Jaccard index, to determine the microbiota structure. The NEC_Onset group and the control_Onset group showed different types of clustering (Figure 3E), but the distribution of each sample was relatively scattered. When all infants achieved full enteral nutrition, the two groups also showed separation, while the control group was more dispersed (Figure 4E).
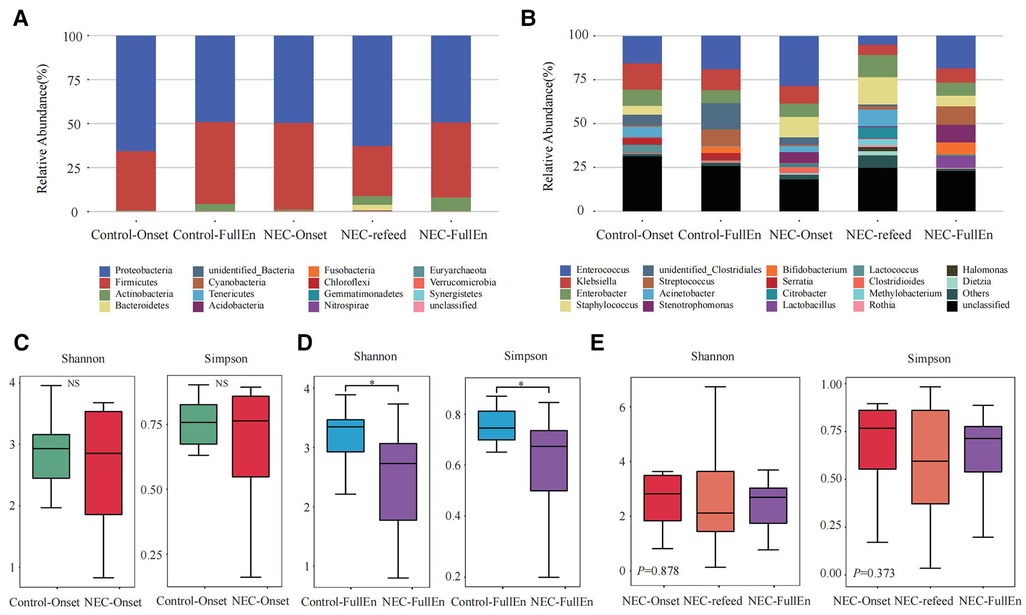
Figure 2. Taxonomic composition analysis and alpha diversity analysis. (A,B) Taxonomic composition analysis. (A) The top 10 at the phylum level between each group. (B) The top 20 at the genus level between each group. (C–E) Alpha diversity analysis. (C) Alpha diversity analysis between the NEC_Onset group and the Control_Onset group. (D) Alpha diversity analysis between the NEC_FullEn group and the Control_FullEn group. (E) Alpha diversity analysis between the three subgroups.
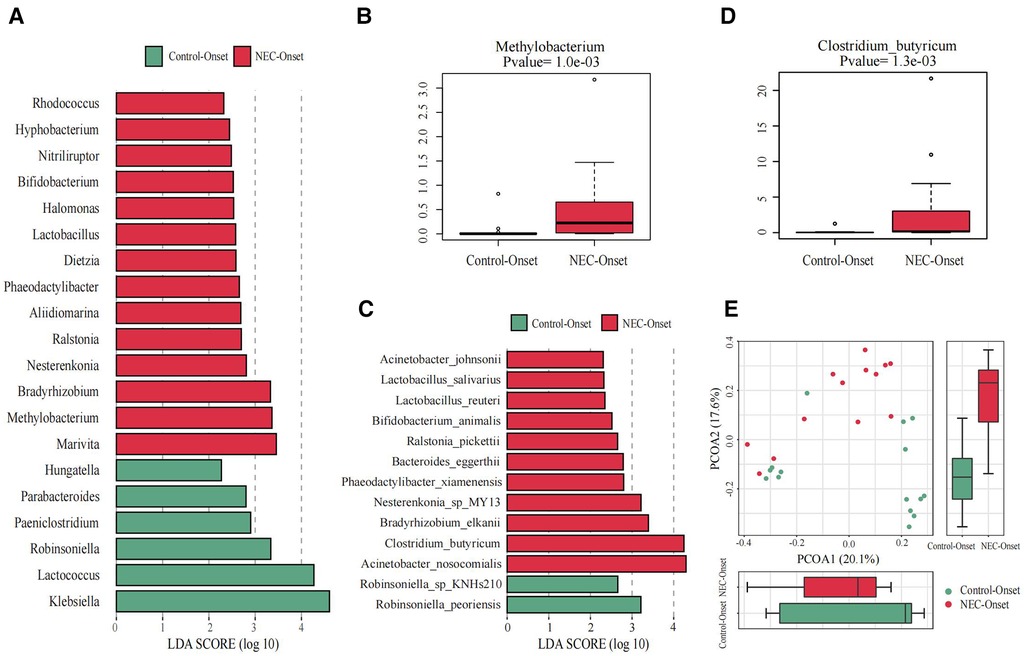
Figure 3. Significant differences in the microbiota and correlation. (A) Gut microbiota analysis at the genus level using the LDA score for NEC group infants and control group infants. (B) Bar chart of Methylobacterium between the NEC_Onset group and the Control_Onset group. (C) Gut microbiota analysis at the species level using the LDA score for NEC group infants and control infants. (D) Bar chart of Clostridium_butyricum between the NEC_Onset group and the Control_Onset group. (E) Principal coordinate analysis (PCoA) of the microbial communities of the two groups. The microbiota cluster in the infants of the NEC_Onset group (red circle) and the Control_Onset group (green circle) was examined by using PCoA. NEC, necrotizing enterocolitis; LDA, linear discriminant analysis.
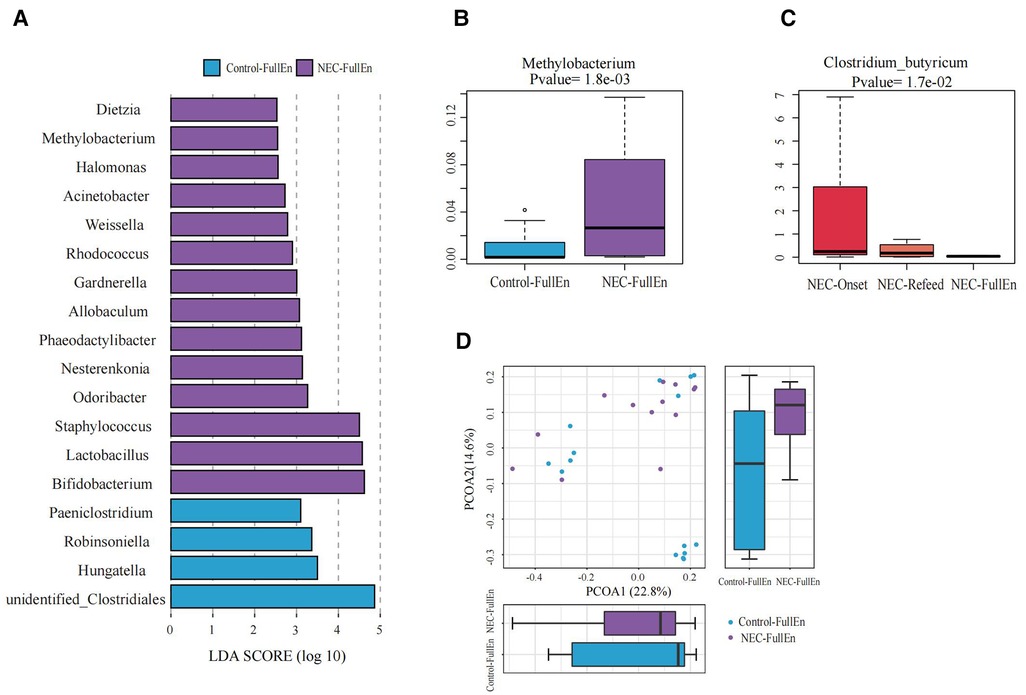
Figure 4. Significant differences in the microbiota and correlation. (A) Gut microbiota analysis at the genus level using the LDA score between two groups after they achieved full enteral nutrition. (B) Bar chart of Methylobacterium between the NEC_FullEn group and the Control_FullEN group. (C) Bar chart of Clostridium_butyricum among the three subgroups of NEC. (D) Principal coordinate analysis (PCoA) of the microbial communities of the two groups after achieving full enteral nutrition. The microbiota cluster in the infants of the NEC_FullEn group (purple circle) and the Control_FullEn group (blue circle) was examined by using PCoA. NEC, necrotizing enterocolitis; LDA, linear discriminant analysis.
In the examination of the overall GM spectrum, the Firmicutes and Proteobacteria were found to be the most abundant flora at the phylum level among all subgroups, which constituted more than 92% of the total bacteria species among the groups. In addition, at the genus level, Enterococcus, Klebsiella, Enterobacter, and Staphylococcus were the most abundant flora in each group (Figures 2A,B).
An analysis of the alpha diversity index indicated that the Shannon and Simpson indices of the NEC_Onset group were slightly lower than those of the Control_Onset group; however, there was no obvious difference between the groups (Figure 2C). These two indices in the NEC_FullEn group were much lower than those in the Control_FullEn group when the infants achieved full enteral nutrition (p < .05) (Figure 2D). The alpha diversity index was also compared between the three subgroups of NEC infants. The Shannon and Simpson indices first showed a declining trend and then a rising trend after NEC treatment. Nevertheless, statistical analysis showed that the alpha diversity index was not significantly different over time (p = .878, p = .373) (Figure 2E).
Differential GM and correlations with clinical markers
The differences at each taxonomic level were observed through LEfSe analysis as well. Firmicutes and Proteobacteria were the most abundant microbiota at the phylum level in all subgroups. There were no differences in the amount of Firmicutes and Proteobacteria between the NEC_Onset and the Control_Onset groups, as well as the NEC_FullEn and the Control_FullEn groups. However, the relative amounts of Acidobacteria at the phylum level were remarkably higher in the NEC_Onset group than in the Control_Onset group (p = .0015). Notably, after treatment, there was an abundance of Acidobacteria in the NEC_FullEn group (p = .00036). Although there was a gradual declining trend of Acidobacteria among the NEC subgroups during therapy, no statistical significance was found (p = .178). In addition, Methylobacterium at the genus level and Clostridium_butyricum at the species level were also significantly abundant in the NEC_Onset group (Figures 3A–D). Even after treatment, the amount of Methylobacterium was still higher in the NEC_FullEn group (Figures 4A,B). In addition, there was a gradual decrease in the amount of Clostridium_butyricum among the three subgroups of infants with NEC (Figure 4C).
Inflammatory reaction always co-occurs with NEC. After a laboratory examination, there is a change in inflammatory indicators during NEC diagnosis or treatment. So, in this study, we also examined the fecal species at different levels correlated with infants’ characteristics and tests. Our examination revealed that Methylobacterium and Clostridium_butyricum were significantly positively correlated with CRP and negatively correlated with platelet count (Figures 5A,B).
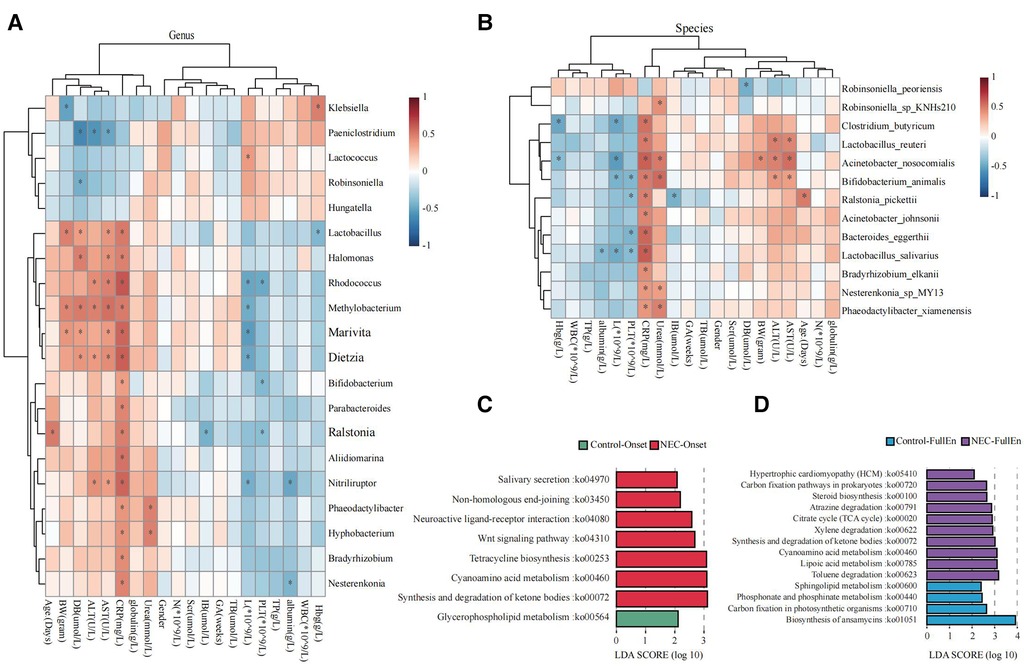
Figure 5. Microbial function prediction. (A) Correlation heatmap for bacteria at the genus level correlated with clinical characteristics and examinations. (B) Correlation heatmap for bacteria at the species level correlated with clinical characteristics and examinations. (C) LDA score for microbial pathways when NEC occurred. (D) LDA score for microbial pathways when all infants achieved full enteral nutrition. NEC, necrotizing enterocolitis; LDA, linear discriminant analysis.
Metabolic functions prediction by differential GM
Increased activities of the pathways related to ketone body synthesis and degradation, metabolism of cyanoamino acid, tetracycline biosynthesis, and Wnt signaling were found in the microbiota of the NEC_Onset group rather than that of the Control_Onset group (Figure 5C). The active difference in the ketone body synthesis and degradation pathway was the most significant factor between the two groups. Notably, this pathway was still more active in NEC infants than those in the control infants after treatment (Figure 5D). Interestingly, the pathway of sphingolipid metabolism was more active in the Control_FullEn group than in the NEC_FullEn group after treatment.
Discussion
NEC is a devastating disease that occurs predominantly in premature infants who survive in the preliminary days after birth (21). Although the precise etiology and pathogenesis of NEC is unclear to date, abnormal microbial colonization might be one of the important risk factors related to this disease (22). Some studies on the alteration of the intestinal microbiota prior to NEC symptoms after birth (10, 11) have shown that the intestinal diversity undergoes a change before clinical symptoms occur (23). Some specific intestinal bacteria have been described in different reports; however, there is no universal conclusion on NEC infants, and such conclusion may disclose only some patient variation but not the real disease etiology (24–26). Unlike these research studies, our longitudinal study collected a serial fecal sample from the time of NEC diagnosis to investigate the changes in the intestinal microbiota after NEC treatment. In addition, our study attempted to observe whether the GM had been restored when infants achieved full enteral nutrition as NEC was clinically cured.
Some previous studies (24, 27, 28) showed that the intestinal diversity decreased before NEC occurred. Some studies (29–31) did not even show any significant difference between the NEC and the control groups. Our results indicated that the Shannon and Simpson indices did not show any significant differences when NEC occurred; however, these two indices were lower in NEC infants after treatment. As the Shannon and Simpson indices are believed to reflect the abundance and evenness of the microbiota, the lower diversity may be associated with a decreased variety of bacteria species. Our results have demonstrated that the alteration of the GM diversity is closely correlated with the initiation and progression of NEC. Even at the time of achieving full enteral nutrition and when NEC infants were clinically cured, the GM alpha diversity had not yet recovered when compared with the control group. For preterm infants, more time is required for the establishment of intestinal flora because of many risk factors (32). Because infants with NEC underwent surgery, more time might be needed for the reestablishment and restoration of gut flora.
Given that the major gut bacteria in the preterm infants were Proteobacteria, Bacteroidetes, Firmicutes, and Actinobacteria at the phylum level were found to be similar to those in the previous report (26, 33). Through LEfSe analysis, our results indicated that Acidobacteria at the phylum level were significantly different between the NEC and the control groups. Even though there was a gradual decrease in this species after NEC therapy, it was still higher than that in control infants. Acidobacteria are the most distributed bacterial phyla, and they are the richest bacteria among a series of ecosystems, especially in soils. However, there are only limited studies on Acidobacteria in intestinal disease. Igarashi et al. (34) reported that the presence of the microbiota in gastric fluid could be described as Bacteroidetes > Proteobacteria, but Acidobacteria were non-existent in functional dyspepsia patients. This indicated an alteration of Acidobacteria in gastrointestinal disorders. Genome sequencing and metabolic analysis revealed some functionalities of Acidobacteria in preterm infants, such as carbohydrate metabolism, nitrogen metabolism, formation of exopolysaccharides, and transport (35). But it was unclear how Acidobacteria influenced the development of NEC.
In addition, our data revealed that the amount of Methylobacterium at the genus level was higher in NEC infants. Methylobacterium belongs to the family of Proteobacteria. Previous studies also reported similar results. Wang et al. indicated that an increased amount of γ-proteobacteria accompanied by a reduction of other bacterial species was found in patients with NEC (28). In a prospective case-control observation, Barbara et al. showed that before the occurrence of NEC, there was a relative enrichment of γ-proteobacteria in infants with very low BW (33). The findings of Lindberg et al. also indicated the association between NEC and gut Proteobacteria (36).
Clostridium_butyricum belongs to the family of anaerobic spore-forming bacillus, a gram-positive bacterium. It can produce a large amount of butyric acid. In recent years, some research studies indicated that the abnormality of Clostridium_butyricum was related to NEC onset (25, 37). Hosny et al. (38) reported that Clostridium_butyricum was significantly higher in excrement specimens collected from infants with NEC than in those taken from controls in the Neonatal Intensive Care Unit (NICU) from southern France. Dong et al. (39) also reported that 15 of 37 (40.50%) samples were contaminated with Clostridium_butyricum during an NEC outbreak at a hospital in China. They reasoned that the NEC outbreak was epidemiologically associated with the contamination of this bacterial species in the hospital. Our results on Clostridium_butyricum were consistent with those of previous studies and indicated that this species was related to NEC development.
Through Spearman correlation analysis, we observed a clear correlation between Methylobacterium, Clostridium_butyricum, Acidobacteria, increased CRP, and decreased platelets. CRP and platelet counts were the most commonly measured biomarkers of inflammation and were related to the severity of NEC. Severe thrombocytopenia (<100 × 109/L) was considered as a predictor of bowel gangrene, morbidity, and mortality (40, 41). Similar to thrombocytopenia, persistently elevated CRP in NEC infants made a case for surgical intervention (42). Therefore, in this respect, the abnormalities of these bacteria suggest a strong correlation with NEC. In addition, our data suggested that an excessive quantity of a single bacterium was not the cause of NEC, and that NEC might be caused by the combinative effect of multiple bacteria. In fact, a further study is necessary to know about this combined effect.
Through functional prediction analysis, we identified that the pathway of ketone body synthesis and degradation was more significantly active in infants with NEC, irrespective of whether NEC occurred before or after therapy. Ketone bodies consist of three different small molecules, acetoacetate, acetone, and β-hydroxybutyrate (β-HB), which are converted into main brain energy substrates during prolonged fasting or starvation. Recent studies indicate that ketone body signaling and ketone bodies themselves are associated with intestinal homeostasis and intestinal microbiota. β-HB, one of the ketone bodies, acts by inhibiting class I histone deacetylases to activate Notch signaling, thereby guiding the self-renewal and pedigree of intestinal stem cells (43). Other analyses suggest that ketone bodies selectively inhibit bifidobacterial growth, resulting in decreased intestinal Th17 cell levels (44). The Th17 cell plays a crucial role in autoimmune and abnormal inflammatory responses in the mucosal surfaces of the gastrointestinal tract (45). They are pro-inflammatory because they secrete cytokines that promote inflammation. On the other hand, under a stable state, the Th17 cells secrete both IL-22 and IL-17 and occupy mucosal barriers to regulate local homeostasis by promoting the function of the epithelial protective screen (46). Based on our data, we consider that the pathway of synthesis and degradation of ketone bodies might be associated with NEC onset and development. An in-depth exploration of how the intestinal microbiota participates in this pathway, such as combined intestinal metabolism (26), may help us understand the pathogenesis of NEC and devise ways to provide better treatment.
After discharge, NEC infants need to be followed up for physical development and neurodevelopmental outcomes (47). Our previous study (48) presented that physical development would be adversely affected in NEC infants, particularly in those who received surgical treatments. Similar results were also demonstrated in other research studies (49, 50). Our data also showed that growth delay occurred more frequently in the NEC group, although there was no significant difference with the control groups. Functional prediction analysis revealed that the pathway related to sphingolipid metabolism was more active in the control group when the infants achieved full enteral nutrition. Because of the multiple variations in the structural component, sphingolipids are extremely multiplexed lipids. They not only construct cellular membranes, as crucial signaling factors sphingolipids also participate in the diversity of cellular function regulations, such as cellular proliferation, growth and differentiation, as well as apoptotosis (51). Recent research studies indicated that intestinal sphingolipids played a crucial role in immunity and inflammatory disorders (52). They were also beneficial for maintaining gut homeostasis and symbiosis (53). Thus, combined with the results of our study and the research concerning the function of sphingolipids, we consider that the pathway of sphingolipid metabolism may act as functions related to physical development. Clearly, more further studies are needed to focus on this.
Among NEC patients, four infants had a relatively long interval between diagnosis and operation. It is valuable to explore and compare the GM features of NEC patients who had long or short intervals from diagnosis to surgery (NEC_LI vs. NEC_SI). The Shannon and Simpson indices indicated that microbial diversity was comparable between the two groups at three different time points (Supplementary Figure S3A). A further LEfSe analysis showed the differential bacteria at the genus level between two subgroups, especially at the refeed time point. Six different genera, including Sphingomonas, Gardnerella, and Bacteroides, were more abundant in the NEC_SI group (Supplementary Figure S3B). However, the small sample size of the two subgroups in this study requires further validation.
Limitation
The limitations of this study are its small sample size, single-center design, non-performance of fecal metabolome examination, and a non-analysis of fecal samples after discharge. Although in our study, the control group was age- and gender-matched, certain factors were difficult to match completely, such as antibiotic use and NICU duration. It is difficult to establish a normal control group in the NICU where preterm infants are admitted for treatment. Further multicenter studies and metabolome analysis might be required. Although the number of cases was limited, our study examined the changes in the intestinal microbiota of surgical NEC patients after treatment, providing some clues for further in-depth studies.
Conclusion
In summary, our data showed that even after achieving full enteral nutrition, the alpha diversity in infants with NEC who underwent surgery was still lower than that in the control group infants. Methylobacterium, Clostridium_butyricum, and Acidobacteria were more abundant in NEC infants at the time of diagnosis and until the end of treatment. NEC infants who opted for surgery for the reestablishment and restoration of gut flora may need some more time to realize these. In addition, the pathways of the ketone body synthesis and degradation were more active in NEC patients who opted for surgery. This indicates that ketone body synthesis and degradation may be related to the pathogenesis of NEC. The pathway of sphingolipid metabolism may influence the physical development of NEC infants.
Data availability statement
The original contributions presented in the study are included in the article/Supplementary Material, and further inquiries can be directed to the corresponding author/s.
Ethics statement
The studies involving human participants were reviewed and approved by the Ethics Committee of the Children's Hospital of Zhejiang University School of Medicine. Written informed consent from the participants’ legal guardian/next of kin was not required to participate in this study in accordance with national legislation and institutional requirements.
Author contributions
LZD and HJL initiated and designed the study, performed the data analysis, and undertook writing and revision. YN reviewed the study and revised the manuscript. CFX participated in the data analysis of the intestinal microbiota. JJC was involved in recruiting patients and collecting fecal samples. XLM and LPS were responsible for quality control. WS modified all figures. HJL and YN edited and prepared the final draft. All authors contributed to the article and approved the submitted version.
Funding
This project was supported by the Neonatal Research Foundation (Grant no. Z-2019-41-2101-04) and the Zhejiang Medical and Health Science and Technology Project (no. 2017KY432).
Acknowledgments
We thank the parents of the infants for agreeing to subject their babies to this study analysis and for participating in this project; we also thank our colleagues of the Neonatal Intensive Care Unit and the Neonatal Surgical Department for participating in the sample collection.
Conflict of interest
The authors declare that the research was conducted in the absence of any commercial or financial relationships that could be construed as a potential conflict of interest.
Publisher's note
All claims expressed in this article are solely those of the authors and do not necessarily represent those of their affiliated organizations, or those of the publisher, the editors and the reviewers. Any product that may be evaluated in this article, or claim that may be made by its manufacturer, is not guaranteed or endorsed by the publisher.
Supplementary material
The Supplementary Material for this article can be found online at: https://www.frontiersin.org/articles/10.3389/fped.2023.993759/full#supplementary-material.
References
1. Neu J, Walker WA. Necrotizing enterocolitis. N Engl J Med. (2011) 364:255–64. doi: 10.1056/NEJMra1005408
2. Frost BL, Modi BP, Jaksic T, Caplan MS. New medical and surgical insights into neonatal necrotizing enterocolitis: a review. JAMA Pediatr. (2017) 171:83–8. doi: 10.1001/jamapediatrics.2016.2708
3. Lin H, Mao S, Shi L, Tou J, Du L. Clinical characteristic comparison of low birth weight and very low birth weight preterm infants with neonatal necrotizing enterocolitis: a single tertiary center experience from eastern China. Pediatr Surg Int. (2018) 34:1201–7. doi: 10.1007/s00383-018-4339-9
4. Hong CR, Han SM, Jaksic T. Surgical considerations for neonates with necrotizing enterocolitis. Semin Fetal Neonatal Med. (2018) 23:420–5. doi: 10.1016/j.siny.2018.08.007
5. Moschino L, Duci M, Fascetti Leon F, Bonadies L, Priante E, Baraldi E, et al. Optimizing nutritional strategies to prevent necrotizing enterocolitis and growth failure after bowel resection. Nutrients. (2021) 13:340. doi: 10.3390/nu13020340
6. Nanthakumar N, Meng D, Goldstein AM, Zhu W, Lu L, Uauy R, et al. The mechanism of excessive intestinal inflammation in necrotizing enterocolitis: an immature innate immune response. PLoS ONE. (2011) 6:e17776. doi: 10.1371/journal.pone.0017776
7. Leis R, De Castro MJ, De Lamas C, Picáns R, Couce ML. Effects of prebiotic and probiotic supplementation on lactase deficiency and lactose intolerance: a systematic review of controlled trials. Nutrients. (2020) 12:1487. doi: 10.3390/nu12051487
8. Relman DA. The search for unrecognized pathogens. Science. (1999) 284:1308–10. doi: 10.1126/science.284.5418.1308
9. Heida FH, Van Zoonen A, Hulscher JBF, Te Kiefte BJC, Wessels R, Kooi EMW, et al. A necrotizing enterocolitis-associated gut microbiota is present in the meconium: results of a prospective study. Clin Infect Dis. (2016) 62:863–70. doi: 10.1093/cid/ciw016
10. Zhou Y, Shan G, Sodergren E, Weinstock G, Walker WA, Gregory KE. Longitudinal analysis of the premature infant intestinal microbiome prior to necrotizing enterocolitis: a case-control study. PLoS ONE. (2015) 10:e0118632. doi: 10.1371/journal.pone.0118632
11. Sim K, Shaw AG, Randell P, Cox MJ, Mcclure ZE, Li MS, et al. Dysbiosis anticipating necrotizing enterocolitis in very premature infants. Clin Infect Dis. (2015) 60:389–97. doi: 10.1093/cid/ciu822
12. Rozé JC, Ancel PY, Lepage P, Martin-Marchand L, Al Nabhani Z, Delannoy J, et al. Nutritional strategies and gut microbiota composition as risk factors for necrotizing enterocolitis in very-preterm infants. Am J Clin Nutr. (2017) 106:821–30. doi: 10.3945/ajcn.117.152967
13. Stewart CJ, Fatemizadeh R, Parsons P, Lamb CA, Shady DA, Petrosino JF, et al. Using formalin fixed paraffin embedded tissue to characterize the preterm gut microbiota in necrotising enterocolitis and spontaneous isolated perforation using marginal and diseased tissue. BMC Microbiol. (2019) 19:52. doi: 10.1186/s12866-019-1426-6
14. Romano-Keeler J, Shilts MH, Tovchigrechko A, Wang C, Brucker RM, Moore DJ, et al. Distinct mucosal microbial communities in infants with surgical necrotizing enterocolitis correlate with age and antibiotic exposure. PLoS ONE. (2018) 13:e0206366. doi: 10.1371/journal.pone.0206366
15. Brower-Sinning R, Zhong D, Good M, Firek B, Baker R, Sodhi CP, et al. Mucosa-associated bacterial diversity in necrotizing enterocolitis. PLoS ONE. (2014) 9:e105046. doi: 10.1371/journal.pone.0105046
16. Bell MJ, Ternberg JL, Feigin RD, Keating JP, Marshall R, Barton L, et al. Neonatal necrotizing enterocolitis. Therapeutic decisions based upon clinical staging. Ann Surg. (1978) 187:1–7. doi: 10.1097/00000658-197801000-00001
17. WHO Child Growth Standards: methods and development. https://www.who.int/tools/child-growth-standards
18. Bolyen E, Rideout JR, Dillon MR, Bokulich NA, Abnet CC, Al-Ghalith GA, et al. Reproducible, interactive, scalable and extensible microbiome data science using QIIME 2. Nat Biotechnol. (2019) 37:852–7. doi: 10.1038/s41587-019-0209-9
19. Callahan BJ, Mcmurdie PJ, Rosen MJ, Han AW, Johnson AJ, Holmes SP. DADA2: high-resolution sample inference from illumina amplicon data. Nat Methods. (2016) 13:581–3. doi: 10.1038/nmeth.3869
20. Douglas GM, Maffei VJ, Zaneveld JR, Yurgel SN, Brown JR, Taylor CM, et al. PICRUSt2 for prediction of metagenome functions. Nat Biotechnol. (2020) 38:685–8. doi: 10.1038/s41587-020-0548-6
21. Neu J. Necrotizing enterocolitis: the mystery goes on. Neonatology. (2014) 106:289–95. doi: 10.1159/000365130
22. Pammi M, Cope J, Tarr PI, Warner BB, Morrow AL, Mai V, et al. Intestinal dysbiosis in preterm infants preceding necrotizing enterocolitis: a systematic review and meta-analysis. Microbiome. (2017) 5:31. doi: 10.1186/s40168-017-0248-8
23. Kim CS, Claud EC. Necrotizing enterocolitis pathophysiology: how microbiome data alter our understanding. Clin Perinatol. (2019) 46:29–38. doi: 10.1016/j.clp.2018.10.003
24. Morrow AL, Lagomarcino AJ, Schibler KR, Taft DH, Yu Z, Wang B, et al. Early microbial and metabolomic signatures predict later onset of necrotizing enterocolitis in preterm infants. Microbiome. (2013) 1:13. doi: 10.1186/2049-2618-1-13
25. Cassir N, Benamar S, Khalil JB, Croce O, Saint-Faust M, Jacquot A, et al. Clostridium butyricum strains and dysbiosis linked to necrotizing enterocolitis in preterm neonates. Clin Infect Dis. (2015) 61:1107–15. doi: 10.1093/cid/civ468
26. Wandro S, Osborne S, Enriquez C, Bixby C, Arrieta A, Whiteson K. The microbiome and metabolome of preterm infant stool are personalized and not driven by health outcomes, including necrotizing enterocolitis and late-onset sepsis. mSphere. (2018) 3:e00104-18. doi: 10.1128/mSphere.00104-18
27. Mcmurtry VE, Gupta RW, Tran L, Blanchard EET, Penn D, Taylor CM, et al. Bacterial diversity and Clostridia abundance decrease with increasing severity of necrotizing enterocolitis. Microbiome. (2015) 3:11. doi: 10.1186/s40168-015-0075-8
28. Wang Y, Hoenig JD, Malin KJ, Qamar S, Petrof EO, Sun J, et al. 16S rRNA gene-based analysis of fecal microbiota from preterm infants with and without necrotizing enterocolitis. ISME J. (2009) 3:944–54. doi: 10.1038/ismej.2009.37
29. Mai V, Young CM, Ukhanova M, Wang X, Sun Y, Casella G, et al. Fecal microbiota in premature infants prior to necrotizing enterocolitis. PLoS ONE. (2011) 6:e20647. doi: 10.1371/journal.pone.0020647
30. Normann E, Fahlén A, Engstrand L, Lilja HE. Intestinal microbial profiles in extremely preterm infants with and without necrotizing enterocolitis. Acta Paediatr. (2013) 102:129–36. doi: 10.1111/apa.12059
31. Torrazza RM, Ukhanova M, Wang X, Sharma R, Hudak ML, Neu J, et al. Intestinal microbial ecology and environmental factors affecting necrotizing enterocolitis. PLoS ONE. (2013) 8:e83304. doi: 10.1371/journal.pone.0083304
32. Iizumi T, Battaglia T, Ruiz V, Perez GI P. Gut microbiome and antibiotics. Arch Med Res. (2017) 48:727–34. doi: 10.1016/j.arcmed.2017.11.004
33. Warner BB, Deych E, Zhou Y, Hall-Moore C, Weinstock GM, Sodergren E, et al. Gut bacteria dysbiosis and necrotising enterocolitis in very low birthweight infants: a prospective case-control study. Lancet. (2016) 387:1928–36. doi: 10.1016/s0140-6736(16)00081-7
34. Igarashi M, Nakae H, Matsuoka T, Takahashi S, Hisada T, Tomita J, et al. Alteration in the gastric microbiota and its restoration by probiotics in patients with functional dyspepsia. BMJ Open Gastroenterol. (2017) 4:e000144. doi: 10.1136/bmjgast-2017-000144
35. Kielak AM, Barreto CC, Kowalchuk GA, Van Veen JA, Kuramae EE. The ecology of Acidobacteria: moving beyond genes and genomes. Front Microbiol. (2016) 7:744. doi: 10.3389/fmicb.2016.00744
36. Lindberg TP, Caimano MJ, Hagadorn JI, Bennett EM, Maas K, Brownell EA, et al. Preterm infant gut microbial patterns related to the development of necrotizing enterocolitis. J Matern Fetal Neonatal Med. (2020) 33:349–58. doi: 10.1080/14767058.2018.1490719
37. Cassir N, Benamar S, La Scola B. Clostridium butyricum: from beneficial to a new emerging pathogen. Clin Microbiol Infect. (2016) 22:37–45. doi: 10.1016/j.cmi.2015.10.014
38. Hosny M, Bou Khalil JY, Caputo A, Abdallah RA, Levasseur A, Colson P, et al. Multidisciplinary evaluation of Clostridium butyricum clonality isolated from preterm neonates with necrotizing enterocolitis in South France between 2009 and 2017. Sci Rep. (2019) 9:2077. doi: 10.1038/s41598-019-38773-7
39. Dong Y, Li Y, Zhang D, Nguyen S, Maheshwari N, Hu Y, et al. Epidemiological and genetic characterization of Clostridium butyricum cultured from neonatal cases of necrotizing enterocolitis in China. Infect Control Hosp Epidemiol. (2020) 41:900–7. doi: 10.1017/ice.2019.289
40. Maheshwari A. Immunologic and hematological abnormalities in necrotizing enterocolitis. Clin Perinatol. (2015) 42:567–85. doi: 10.1016/j.clp.2015.04.014
41. Kenton AB, O'donovan D, Cass DL, Helmrath MA, Smith EO, Fernandes CJ, et al. Severe thrombocytopenia predicts outcome in neonates with necrotizing enterocolitis. J Perinatol. (2005) 25:14–20. doi: 10.1038/sj.jp.7211180
42. Pourcyrous M, Korones SB, Yang W, Boulden TF, Bada HS. C-reactive protein in the diagnosis, management, and prognosis of neonatal necrotizing enterocolitis. Pediatrics. (2005) 116:1064–9. doi: 10.1542/peds.2004-1806
43. Cheng CW, Biton M, Haber AL, Gunduz N, Eng G, Gaynor LT, et al. Ketone body signaling mediates intestinal stem cell homeostasis and adaptation to diet. Cell. (2019) 178:1115–31.e15. doi: 10.1016/j.cell.2019.07.048
44. Ang QY, Alexander M, Newman JC, Tian Y, Cai J, Upadhyay V, et al. Ketogenic diets alter the gut microbiome resulting in decreased intestinal Th17 cells. Cell. (2020) 181:1263–75.e16. doi: 10.1016/j.cell.2020.04.027
45. Steinmeyer S, Howsmon DP, Alaniz RC, Hahn J, Jayaraman A. Empirical modeling of T cell activation predicts interplay of host cytokines and bacterial indole. Biotechnol Bioeng. (2017) 114:2660–7. doi: 10.1002/bit.26371
46. Carr TM, Wheaton JD, Houtz GM, Ciofani M. JunB promotes Th17 cell identity and restrains alternative CD4(+) T-cell programs during inflammation. Nat Commun. (2017) 8:301. doi: 10.1038/s41467-017-00380-3
47. Bazacliu C, Neu J. Necrotizing enterocolitis: long term complications. Curr Pediatr Rev. (2019) 15:115–24. doi: 10.2174/1573396315666190312093119
48. Lin HJ, Shi LP, Du LZ. [Long-term prognosis of neonates with necrotizing enterocolitis]. Zhongguo Dang Dai Er Ke Za Zhi. (2018) 20:985–9. doi: 10.7499/j.issn.1008-8830.2018.12.002
49. Salhab WA, Perlman JM, Silver L, Sue Broyles R. Necrotizing enterocolitis and neurodevelopmental outcome in extremely low birth weight infants <1000 g. J Perinatol. (2004) 24:534–40. doi: 10.1038/sj.jp.7211165
50. Hintz SR, Kendrick DE, Stoll BJ, Vohr BR, Fanaroff AA, Donovan EF, et al. Neurodevelopmental and growth outcomes of extremely low birth weight infants after necrotizing enterocolitis. Pediatrics. (2005) 115:696–703. doi: 10.1542/peds.2004-0569
51. Burla B, Muralidharan S, Wenk MR, Torta F. Sphingolipid analysis in clinical research. Methods Mol Biol. (2018) 1730:135–62. doi: 10.1007/978-1-4939-7592-1_11
52. Abdel Hadi L, Di Vito C, Riboni L. Fostering inflammatory bowel disease: sphingolipid strategies to join forces. Mediators Inflamm. (2016) 2016:3827684. doi: 10.1155/2016/3827684
Keywords: gut microbiota, necrotizing enterocolitis, preterm, surgery, 16S rRNA sequencing
Citation: Lin H, Xu C, Chen J, Ma X, Shi L, Shi W, Du L and Ni Y (2023) Alteration of the gut microbiota after surgery in preterm infants with necrotizing enterocolitis. Front. Pediatr. 11:993759. doi: 10.3389/fped.2023.993759
Received: 14 July 2022; Accepted: 3 January 2023;
Published: 30 January 2023.
Edited by:
Narendra Aladangady, Homerton University Hospital NHS Foundation Trust, United KingdomReviewed by:
Lu-Quan Li, Chongqing Medical University, ChinaRoshonda B. Jones, Independent Researcher, Charlotte, NC, United States
© 2023 Lin, Xu, Chen, Ma, Shi, Shi, Du and Ni. This is an open-access article distributed under the terms of the Creative Commons Attribution License (CC BY). The use, distribution or reproduction in other forums is permitted, provided the original author(s) and the copyright owner(s) are credited and that the original publication in this journal is cited, in accordance with accepted academic practice. No use, distribution or reproduction is permitted which does not comply with these terms.
*Correspondence: Lizhong Du dulizhong@zju.edu.cn Yan Ni yanni617@zju.edu.cn
Specialty Section: This article was submitted to Neonatology, a section of the journal Frontiers in Pediatrics