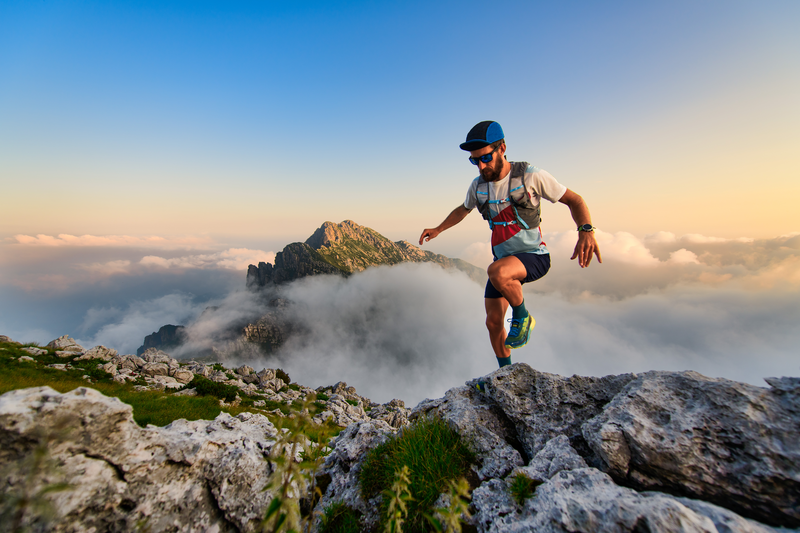
94% of researchers rate our articles as excellent or good
Learn more about the work of our research integrity team to safeguard the quality of each article we publish.
Find out more
REVIEW article
Front. Pediatr. , 24 November 2023
Sec. Pediatric Pulmonology
Volume 11 - 2023 | https://doi.org/10.3389/fped.2023.1306234
This article is part of the Research Topic Reviews in Pediatric Pulmonology View all 5 articles
Background: Mycoplasma pneumoniae pneumonia (MPP), attributable to Mycoplasma pneumoniae (MP), represents a predominant form of community-acquired pneumonia in pediatric populations, thereby posing a significant threat to pediatric health. Given the burgeoning volume of research literature associated with pediatric MPP in recent years, it becomes imperative to undertake a bibliometric analysis aimed at delineating the current research landscape and emerging trends, thereby furnishing a framework for subsequent investigations.
Methods: A comprehensive literature search targeting pediatric MPP was conducted in the Web of Science Core Collection. After the removal of duplicate entries through Endnote software, the remaining articles were subject to scientometric analysis via Citespace software, VOSviewer software and R language, focusing on variables such as publication volume, contributing nations, institutions and authors, references and keywords.
Results: A total of 1,729 articles pertinent to pediatric MPP were included in the analysis. China and the United States emerged as the nations with the highest publication output. Italian scholar Susanna Esposito and Japanese scholar Kazunobu Ouchi were the most influential authors in the domain of pediatric MPP. Highly-cited articles primarily focused on the epidemiological investigation of pediatric MPP, the clinical characteristics and treatment of macrolide-resistant MPP, and biomarkers for refractory Mycoplasma pneumoniae pneumonia (RMPP). From the corpus of 1,729 articles, 636 keywords were extracted and categorized into ten clusters: Cluster #0 centered on molecular-level typing of macrolide-resistant strains; Cluster #1 focused on lower respiratory tract co-infections; Clusters #2 and #6 emphasized other respiratory ailments caused by MP; Cluster #3 involved biomarkers and treatment of RMPP; Clusters #4 and #9 pertained to extrapulmonary complications of MPP, Clusters #5 and #7 addressed etiological diagnosis of MPP, and Cluster #8 explored pathogenic mechanisms.
Conclusions: The past few years have witnessed extensive attention directed towards pediatric MPP. Research in pediatric MPP principally revolves around diagnostic techniques for MP, macrolide resistance, complications of MPP, treatment and diagnosis of RMPP, and elucidation of pathogenic mechanisms. The present study provides pediatric clinicians and researchers with the research status and focal points in this field, thereby guiding the orientation of future research endeavors.
Mycoplasma pneumoniae (MP) is presently recognized as the smallest prokaryotic microorganism capable of independent survival without a host cell (1), and concurrently serves as the leading etiological agent responsible for pediatric community-acquired pneumonia (CAP) (2). Globally, epidemics of Mycoplasma pneumoniae pneumonia (MPP) materialize at intervals of 3–7 years, accounting for more than 40% of pediatric CAP cases during epidemic years (3). It was previously believed that MPP usually occured in children over five years old, and the symptoms were mild (4, 5). However, recent clinical observations have indicated a trend towards a younger age of onset for MPP (6), accompanied by a rise in cases of refractory pneumonia and multisystemic complications induced by MP, thereby posing a substantial threat to pediatric health (7).
In light of these factors, there has been heightened attention towards this malady from both pediatric clinicians and scientific researchers, culminating in the prolific dissemination of research findings in the form of academic articles. On one hand, this surge in published literature has significantly propelled advancements in the field of pediatric MPP and fostered collaborative endeavors. On the other hand, due to the voluminous, dispersed, and at times redundant nature of these publications, obtaining a comprehensive and systematic understanding of the current state of research on pediatric MPP remains challenging. Bibliometrics, an interdisciplinary field employing mathematical and statistical methodologies, serves to conduct quantitative analysis of the knowledge carriers within a specific academic domain (8). By executing a bibliometric analysis on the extant literature, one can obtain outstanding contributors and current research hotspots in this field, which is beneficial for researchers to identify partners and design research subject in the future. However, to the best of our knowledge, there has yet to be a bibliometric study specifically targeting pediatric MPP. Consequently, the objective of the present study is to deploy bibliometric techniques to integrate existing theoretical, clinical, and experimental findings, thereby manifesting the research advancements in pediatric MPP through knowledge mapping. The study further aims to analyze the prevailing research hotspots and cutting-edge theories within this domain, with the intention of furnishing a referential framework for future investigations.
The Web of Science is the most commonly used database for bibliometrics research. It contains academic journals, monographs, and conference proceedings in multidisciplinary fields (9). Consequently, this study sourced its corpus of literature from the Web of Science Core Collection (WoSCC). The retrieval strategy employed was articulated as follows: TS = (“Mycoplasma pneumoniae” or “Eaton Agent” or “M. pneumoniae” or “MP”) and TS = (Pneumonia* or “Pulmonary Inflammation*” or “Inflammation*, Pulmonary” or “Lung Inflammation*” or “Inflammation*, Lung”) AND TS = (Child* or Pediatric* or Paediatric* or Infant* or Newborn* or Neonat* or Preschool* or Pubert* or Adolescen* or Teen*). Among them, “TS” denotes “Topic Search”; asterisks serve as truncation symbols, capable of representing zero or more succeeding characters (e.g., Child* may signify Child, Children, or Childhood). The time-frame was delimited to include works published up to June 2, 2023. Language criteria were restricted to English, and document type was confined to either “article” or “review”. Duplicate entries were excised utilizing Endnote software (version X9.3.3), culminating in a dataset of 1,729 articles for ensuing analysis. The schematic representation of the literature retrieval procedure is delineated in Figure 1.
Subsequent to retrieving a total of 1,729 articles from the WoSCC, we downloaded the “Full Record and Cited References” and stored them as plain text files. Predominantly, bibliometric analyses were executed using Citespace software (version 6.2.R3), VOSviewer software (version 1.6.18) and R language's bibliometrix package, focusing on the parameters of publication volume, national contributions, institutional affiliations, authorship, reference co-citations, and keywords.
In compliance with our retrieval strategy and criteria for inclusion and exclusion, a corpus of 1,729 articles pertinent to pediatric MPP was amassed. Of these, 1,518 were categorized as original research articles, accounting for approximately 90% of the total, while an additional 211 were review articles. As depicted in Figure 2, the cumulative publication volume from 1999 through 2023 approximates an exponential growth pattern (R2 = 0.9529). Although year-to-year fluctuations in publication counts were observed, the overarching trend manifested a robust increase, notably in the years 2021 and 2022, where the growth was most conspicuous.
Utilizing Citespace software, networks delineating national and institutional collaborations were constructed respectively, as illustrated in Figure 3. Within this graphical representation, each node is emblematic of a nation/region or an institution. The magnitude of the node is indicative of its publication volume, while the color designates the year of publication. Nodes encircled by a purple perimeter possess elevated levels of betweenness centrality (centrality >0.1), signifying their pivotal role in catalyzing international collaborations or institutional communication. As discerned from Figure 3A and Table 1, a total of 86 nations/regions have engaged in pediatric MPP research. China has published 595 articles, constituting 34.4% of the total publication volume and thereby occupying the foremost position. Following China are the United States, Japan, Italy, and South Korea. Notably, the collaborative network among global nations/regions manifests as an intricate web of partnerships. The United States exhibits the highest level of betweenness centrality, indicating its significant contribution to facilitating international exchanges and cooperation.
Figure 3. National/regional and institutional cooperation network. (A) National/regional cooperation network. Scotland, England and Wales are collectively called UK. New Caledonia is included in France. (B) Institutional collaboration network.
Figure 3B and Table 2 display the relevant institutions engaged in pediatric MPP research. Soochow University and Capital Medical University emerged as the institution with the highest publication volume (n = 59). Most institutions tend to conduct research independently, and the only institutions with high centrality are the Centers for Disease Control & Prevention in the United States, Boston University and Research Libraries UK.
The h-index is a commonly used indicator to characterize the scientific output of authors in bibliometrics research (10). It represents that a given author has published h papers, and each paper has been cited at least h times (11). The h-index simultaneously measures the quantity and quality of the authors' publications (12), so it can be used to identify authors who have significant influence in the domain of pediatric MPP. We used R language's bibliometrix package to obtain the h-index for a total of 6,972 authors (Supplementary Table S1). Figure 4 and Table 3 display authors with high h-index. Susanna Esposito from the University of Milan, Italy, is the most influential author, having published 25 papers, with a total of 806 citations, and the highest h-index of 16. Subsequent to Susanna Esposito are Japanese scholar Kazunobu Ouchi, with 26 papers, a total of 809 citations, and the h-index of 15. Chinese scholar Wei Ji, Yong-Dong Yan and Italian scholar Nicola Principi tie for third place with the h-index of 13.
The foundation of a scientific field lies in its highly cited literature. In the realm of pediatric MPP, influential publications have substantially propelled the progression of research. Employing CiteSpace software for a bibliometric analysis of the cited literature, a co-citation network (Figure 5) was generated. Each node in this network symbolizes an individual reference, with the node size proportionate to its citation frequency. Since the total citations of an article is closely related to its publication time, we calculated the annual average citations for each reference (Supplementary Table S2) and listed the top ten articles in Table 4.
The most frequently cited article is a comprehensive review by Waites KB et al., published in Clinical Microbiology Reviews in 2017. This article offered an exhaustive summary of global epidemiology, pathogenic mechanisms, clinical manifestations, in vitro culture of MP, diagnostic techniques, antibiotic treatments, macrolide resistance, drug development against MP, and molecular typing (13). The second (14) and third (15) highly cited articles conducted multicenter, prospective clinical studies on pediatric MPP and pediatric CAP in hospitalized patients, respectively. Both studies showed that MP was a prevalent etiological agent of pediatric CAP; MPP was notably frequent in older children (≥5 years, especially between 10 and 17 years), presented usually with milder clinical symptoms; however, compared to pneumonias induced by other pathogens, MPP exhibited non-specific clinical manifestations and radiological findings. The fourth (16), ninth (17) and tenth (18) highly cited papers focused on macrolide-resistant Mycoplasma pneumoniae (MRMP). The fourth article reviewed the clinical features and treatment modalities of macrolide-resistant Mycoplasma pneumoniae pneumonia (MRMPP) in children. Based on a retrospective clinical study conducted in Taiwan, the authors of the ninth article believed that delayed treatment caused by macrolide resistance was associated with disease severity and multiple extrapulmonary complications. Similarly, through a meta-analysis, the authors of the tenth article found that patients infected with MRMP had more severe clinical manifestations and longer hospital stay than those infected with macrolide-sensitive Mycoplasma pneumoniae. The fifth referenced paper (19) delved into the treatment of MRMPP and refractory Mycoplasma pneumoniae pneumonia (RMPP) in children. The sixth (20) and seventh (21) cited article conducted clinical retrospective analyzes on the epidemiology and clinical characteristics of pediatric MPP. The eighth cited article (22) comparatively analyzed clinical data of RMPP against general MPP and concluded that elevated levels of C-reactive protein (CRP), lactic dehydrogenase (LDH), and interleukin (IL)-6 could serve as predictive indicators for RMPP.
Keyword serves as a summarization of the research themes addressed in the academic articles. Utilizing Citespace software, keywords were extracted from a compendium of 1,729 scholarly papers, and synonymous terms were subsequently consolidated (Supplementary Table S3). A total of 636 keywords were generated. Figure 6A portrays the keyword co-occurrence network, where the node dimensions are indicative of the frequency with which each keyword appears; a higher frequency results in an enlarged node. Edges connecting these nodes represent co-occurrence within scholarly works of a thematic similarity. Table 5 delineates the 20 most frequently occurring keywords, including “MP”, “infection”, “children”, “CAP”, “respiratory infections”, and “MPP”, all of which are germane to the overarching subject matter of the present investigation. Moreover, the terms “diagnosis”, “PCR”, “real-time PCR”, “antibody”, “epidemiology”, “etiology”, “chlamydia pneumoniae”, “respiratory syncytial virus”, and “streptococcus pneumoniae” reflect an investigative focus on pathogenic diagnostics, epidemiology, and clinical characteristics. Keywords such as “macrolide resistance” and “strains” reflect the attention paid to the situation of MP resistance. Subsequent to this, a cluster analysis was employed to categorize these 636 keywords into ten clusters (Figure 6B and Table 6). Specifically, Cluster #0 is dedicated to molecular-level typing of macrolide-resistant strains; Cluster #1 investigates lower respiratory tract co-infections; Clusters #2 and #6 elucidate the interrelation of MP with other respiratory diseases; Cluster #3 delves into the biomarkers and treatment modalities of RMPP; Cluster #4 and #9 focus on extra-pulmonary complications in MPP; Cluster #5 and #7 are concerned with etiological diagnostics of MPP; and Cluster #8 pertains to pathogenic mechanisms.
Figure 6. Keyword analysis. (A) Keyword co-occurrence network; (B) keyword clustering map; (C) top 25 keywords with the strongest citation bursts.
Keyword burst refers to the rapid surge in the frequency of particular keywords within a specified timeframe (8). By implementing a keyword burst analysis, one can elucidate the evolutionary trajectory of research hotspots in pediatric MPP, thereby identifying emergent research frontiers and trending avenues of inquiry. Figure 6C showcases the top 25 keywords with the strongest citation bursts from the years 1999 to 2023. The evolution of focal points in pediatric MPP research can be principally bifurcated into two phases: The initial phase spanned from 1999 to 2009, characterized by a research emphasis predominantly on MP diagnostic methodologies (PCR, clinical samples, antibody, culture, enzyme immunoassay), central nervous system damage, therapeutic efficacy, and alternative respiratory pathogens (chlamydia pneumoniae, human metapneumovirus, legionella pneumophila). The subsequent phase, commencing in 2009, manifested new hotspots including resistance mutation sites in macrolide antibiotics (23s ribosomal RNA), molecular biology assays (real-time PCR, molecular analysis, tandem repeat analysis), clinical features, pathogenic mechanisms (cytokines), and treatments (methylprednisolone pulse therapy, requiring hospitalization). Among these, the terms “cytokines”, “tandem repeat analysis”, “methylprednisolone pulse therapy”, and “requiring hospitalization” have maintained their burst status through to 2023, signifying current research hotspots and frontiers. Figure 7 depicts the co-occurrence network of four leading-edge keywords. Those tightly associated with “cytokines” primarily include “inflammation”, “activation”, “risk factors”, and “biomarker”. The term “tandem repeat analysis” is closely linked with “23s ribosomal RNA”, “strains”, “macrolide resistance” and “molecular analysis”. “Methylprednisolone pulse therapy” frequently co-occurs with “surveillance”, “adults”, “clinical features”, “risk factors” and “RMPP”. Lastly, “requiring hospitalization” is commonly co-occurring with “respiratory infections”, “virus”, “23s ribosomal RNA” and “cards toxin”.
Figure 7. Co-occurrence map of 4 research frontier keywords. (A) “Cytokines” co-occurrence map; (B) “tandem repeat analysis” co-occurrence map; (C) “methylprednisolone pulse therapy” co-occurrence map; (D) “requiring hospitalization” co-occurrence map.
MPP constitutes a salient public health issue pertaining to global pediatric well-being, thus engendering an influx of research endeavors in recent years. In the current bibliometric study, we employed Citespace software, VOSviewer software and R language to methodically analyze pediatric MPP literature published in the Web of Science database, aiming to furnish researchers in the domain with a structured and visualizable knowledge framework.
Between 1999 and 2023, the publication volume concerning pediatric MPP has exhibited a steady ascendance, entering an accelerated growth phase post-2018, with an annual augmentation exceeding 100 publications. This trend underscores the burgeoning attention directed towards this disease. Concurrently, the emergence of Severe Acute Respiratory Syndrome Coronavirus 2 (SARS-CoV-2), causing Coronavirus Disease 2019 (COVID-19), has swiftly engulfed the global landscape since its initial reportage in December 2019, marking it as the most significant public health exigency of the century (23). In response, various national governments have instituted stringent containment measures, such as civilian movement restrictions, obligatory mask-wearing, and hand hygiene campaigns, thereby mitigating respiratory pathogen transmission pathways (24, 25) and inducing a palpable decrement in pediatric MPP incidence rates (26, 27). Nevertheless, our findings denoted that the years 2021 and 2022 were maximal in publication output, featuring 176 and 174 pediatric MPP-related documents respectively. The presumptive rationale may be that the pre-COVID-19 era coincided with an MPP epidemic, thereby inflating incidence rates (28, 29), which not only availed clinicians with substantial clinical data but also encouraged scholars to carry out more foundational research.
A total of 86 nations and regions around the world have engaged in pediatric MPP research. China and the United States emerge as the nations with the highest publication output, issuing 595 and 292 papers respectively. Cooperation between countries is relatively close, forming an intricate web of partnerships. On the contrary, as evinced by Figure 3B, the distribution of most institutions is scattered, indicating that cooperation between institutions needs to be strengthened. In terms of authorship, Italian scholar Susanna Esposito has the highest h-index, primarily focusing on clinical research of pediatric MPP to analyze clinical manifestations and explore the correlation between MP and asthma, upper respiratory tract infections, and extrapulmonary diseases (30–34). Japanese scholar Kazunobu Ouchi has published multiple high-quality original articles in the field of MRMP, and his h-index ranks second. Since 2004, Ouchi K's research team has been dedicated to conducting epidemiological investigations of MRMP infection, spotlighting molecular typing of drug-resistant strains (35–37). At the same time, he also evaluated the clinical efficacy of macrolide antibiotics, tosufloxacin, and minocycline against MRMPP in children (38, 39).
Through the statistical analysis of the references by the Citespace software, we discerned that among the ten articles with the highest average citations in this sphere, including six original research articles and four review papers. It is noteworthy that these original articles all pertain to clinical studies, signifying an exigency for substantial attention toward foundational research in the domain of pediatric MPP in the future. The principal foci of these highly-cited articles predominantly encompass the clinical characteristics of pediatric MPP, treatment modalities of MRMP, and biomarkers of RMPP.
Through the analysis of literature co-citation, as well as co-occurrence, cluster, and burst analysis of keywords, several salient issues in the pediatric MPP domain can be summarized:
Accurate and expeditious diagnosis of MP infection is pivotal for the initiation of appropriate antibiotic therapy (40). Current diagnostic methodologies for MP include culture, serological test, and nucleic acid amplification techniques [primarily polymerase chain reaction (PCR)], each beset with its own set of limitations (41). Traditional culture method has historically been the “gold standard” for MP diagnosis, yet this approach is hampered by the slow in vitro growth rate of MP and stringent environmental requirements, leading to an elevated risk of false-negative outcome (42).
Serological test, particularly complement fixation test and enzyme immunoassay, serve as pivotal tools in clinical practice for MP diagnosis (43). The surface of MP contains abundant lipid and protein antigens, which can trigger antibody responses and produce specific anti-MP immunoglobulins M (IgM) and G (IgG), becoming the main targets for serological test (44). IgM antibodies typically manifest within seven to ten days post-infection and elevated levels may persist for several months. It should be noted that infants, due to their underdeveloped immune systems, are often incapable of mounting a robust humoral immune response against MP, thus making the detection of IgM challenging (13). IgG antibodies emerge later, approximately three weeks following MP infection (45). Regardless of whether IgM or IgG is considered, relying solely on a single testing event for diagnosis is deemed unreliable; a fourfold or greater elevation of antibody levels in the convalescent phase compared to the acute phase offers diagnostic validity (46, 47).
Given the expediency, high sensitivity, and specificity of PCR techniques, they have been widely adopted for early identification of various pathogens across multiple clinical sample types, including sputum, blood, and nasopharyngeal swabs (48). Advances in molecular diagnostics have led to the diversification of PCR into conventional PCR, real-time PCR, nested PCR, and reverse transcriptase PCR among others (49). The most prevalent assay targets the P1 gene of MP utilizing real-time PCR (46). A meta-analysis by Zhang L et al. substantiated that real-time PCR offered superior performance in identifying MP infection (50). Thus, “real-time PCR” emerged as a focal term during the period of 2010–2014. Albeit numerous clinical studies affirmed higher sensitivity of PCR compared to serological test for early diagnosis in pediatric MP infections (51, 52), challenges persist due to poor cooperation among children in obtaining high-quality samples, in addition to the potential influence of PCR inhibitors and contamination, leading to false-negative or false-positive result (53). Considering the strengths and weaknesses of both serology and PCR, a combined approach was recommended for reliable diagnosis of early-stage MP infections (50).
Macrolides, represented by azithromycin and erythromycin, are the first-line antibiotics for the treatment of pediatric MPP (19). With the ubiquitous employment of macrolide antibiotics, resistance in MP has increasingly attracted attention, especially in East Asian countries such as Japan, China, and South Korea, where the incidence of MRMP in children exceeds 70% (35, 54, 55). The target of macrolide antibiotics is the 23S rRNA component of the MP ribosome. The ribosome serves as the nexus for protein synthesis, with 23S rRNA being an integral part of its 50S large subunit, which is further divided into six structural domains. Domain V is intricately associated with peptidyl transferase activity (56). Consequently, when mutations occur at specific sites on the domain V of the 23S rRNA in the bacterial strain, the affinity between macrolide antibiotics and MP is compromised. This impediment renders the antibiotic ineffective in inhibiting protein synthesis, thereby leading to therapeutic failure (46). Current research has identified the mutated positions as 2,062, 2,063, 2,064, 2,067, 2,611, and 2,617 (57–59). Mutations at positions 2,063 and 2,064 are the most prevalent, serving as hallmarks of macrolide resistance in MP and constituting a focal point of contemporary research (60).
Our cluster analysis of keywords indicates that research closely associated with macrolide resistance is principally oriented at the molecular typing of MP (strains; tandem repeat analysis; pediatric patients; real-time PCR). Molecular typing of MP strains aids researchers in epidemiological surveillance and in studying disease outcomes. Traditional typing method primarily targets the P1 gene through PCR-restriction fragment length polymorphism (RFLP), categorizing MP strains into types Ⅰ and Ⅱ (61). In 2009, Dégrange et al. were the first to apply multiple-locus variable-number tandem-repeat analysis (MLVA) to MP strain typing (62). MLVA includes five loci (Mpn1, Mpn13, Mpn14, Mpn15, and Mpn16); however, owing to the instability of Mpn1, contemporary analyses frequently utilize a four-loci approach (excluding Mpn1) for MP identification (63). MLVA typing exhibits higher discriminatory power compared to traditional P1 gene typing (61). Numerous studies have been explored the association between MP strain typing and macrolide resistance. Ho PL et al. found that in the Hong Kong region of China, strains MLVA 4-5-7-2 and MLVA 3-5-6-2 were predominant and were strongly associated with macrolide resistance, comprised 89.6% of cases in MRMPP patients (64). A meta-analysis by Wang et al. reached a similar conclusion, identified MLVA 4-5-7-2 as the principal type in MRMP (60). However, research by Rodman Berlot et al. failed to establish a correlation between MLVA genotypes and resistance (65), suggesting that geographic variations may contribute to disparate findings.
Given the escalating global crisis of macrolide resistance, the quest for alternative antibiotics for treating MRMP is gaining momentum. Lung DC et al. published practice recommendations for management of CAP in children, recommended the use of doxycycline for MRMP in children >8 years of age; for those ≤8 years, a cautious risk-benefit analysis should be employed, potentially resorted to doxycycline or fluoroquinolone (66). However, tetracyclines may induce enamel hypoplasia and permanent tooth discoloration, while fluoroquinolones carry the potential risk of musculoskeletal toxicity (67). Consequently, these drug classes are seldom utilized in pediatric clinical practice. In 2010, tosufloxacin was approved in Japan for treating pediatric MP infections (68). Oishi T et al. conducted resistance testing on clinical isolates of MP and found no resistance to tosufloxacin (36). Regrettably, a subsequent clinical study by the same team revealed that tosufloxacin demonstrated no significant therapeutic efficacy in treating pediatric MRMPP (69). Hence, the search for more efficacious and safer alternative treatments for MRMP remains an ongoing imperative for researchers globally.
Clusters #2 and #6 focus on the nexus between MP and other respiratory diseases, encompassing key terms such as asthma, pertussis-like syndrome, and pharyngitis. MP infection triggers inflammatory responses in airways and augments airway resistance (70), both of which play pivotal roles in the pathogenesis of asthma (71). MP has been implicated as both an inciting and exacerbating factor in pediatric asthma, and has been confirmed by multiple studies (72–76). For instance, Biscardi S et al. discovered that in children experiencing their initial episodes of asthma, 50% exhibited acute MP infection, with MP-induced acute exacerbations of asthma constituting approximately 20% (76). A meta-analysis demonstrated a statistically significant positive association between MP infection and any type of childhood asthma. Meanwhile, some studies have also corroborated the efficacy of macrolide antibiotics in treating asthma, leading to improved pulmonary function, symptomatic relief, and a reduction in the exacerbation rate in severe neutrophilic asthma cases (77–79). However, vigilance is required concerning the risk of antibiotic resistance and the adverse effects of long-term usage; insufficient evidence exists to substantiate the use of macrolide medications in chronic or acute asthma settings (80).
Pertussis-like syndrome constitutes a clinical syndrome elicited by pathogens other than Bordetella pertussis, and its clinical manifestations resemble those of pertussis, commonly manifesting as paroxysmal spasmodic coughing, crowing reverberations upon cough cessation, and post-cough emesis. Infants may exhibit cyanosis, apnea, and convulsions following intense coughing episodes (81). The disease often persists for several months, with pharmacological interventions generally exhibiting suboptimal efficacy (82). MP constitutes a significant etiological factor in pediatric pertussis-like syndrome (83). Upon human entry, MP adheres prolifically to the surface of airway epithelial cells and secretes the community-acquired respiratory distress syndrome toxin (CARDS TX), which possesses ADP-ribosyltransferase (ADPRT) and vacuolating cytotoxin activities. CARDS TX is highly analogous to the S1 subunit of the pertussis toxin, which can lead to ciliary stasis, lymphocytic infiltration and increased tissue permeability, thus escalating the secretion of airway inflammatory mediators and inhibiting the clearance of mucosal secretions (84). The resultant residual secretions persistently stimulate peripheral airway nerves, thereby inducing enduring paroxysmal coughing, a mechanism akin to that of pertussis (85).
While MP is not a principal causative agent for acute pharyngitis (86, 87), a prospective cohort study indicated its association with frequent recurrences of pediatric pharyngitis (32).
MP manifests its pathological influence not only within the respiratory system but also across a multitude of organs, encompassing cutaneous, hematologic, cardiovascular, musculoskeletal, and neurological systems (88). The mechanisms underpinning these extrapulmonary manifestations can be categorized into three classes: (i) Direct: MP disseminates via the bloodstream to distal organs, instigating localized inflammatory processes. (ii) Indirect: Pathological consequences arise from immunological dysregulation incited by MP, including autoimmunity, hypersensitivity, and formation of immune complexes. (iii) Vascular occlusive: Extrathoracic pathologies ensue due to vasculitis, thrombogenesis, or a systemic hypercoagulable state induced by MP (89). Our findings indicate that neurological and dermatological connective tissue abnormalities provoked by MP attract considerable attention.
MP-associated neurological impairments commonly present as encephalitis, acute disseminated encephalomyelitis, and stroke. Based on the timing of neurological symptomatology, one can distinguish between early-onset and late-onset conditions (90). Neurological damage occurring within seven days of respiratory infection is classified as early-onset, and higher detection rates of MP in the cerebrospinal fluid of these patients have been reported (91). Narita M et al. observed a significant elevation in the levels of inflammatory markers in the cerebrospinal fluid of pediatric patients with early-onset encephalitis and aseptic meningitis (92). Consequently, some scholars posited that MP-related early-onset neurological diseases represent a form of direct extrapulmonary injury (90). The neurological symptoms following ≥8 days of respiratory infection is termed as late-onset condition, which is indirect extrapulmonary lesions with autoimmune dysregulation as the main pathological mechanism (90). Galactocerebroside C (Gal-C), a glycolipid localized within myelin of the nervous system, can cross-react with P1 adhesin and glycolipids of MP to form a Gal-C-like structure, thereby inducing cross-reactive antibody production (93).
Keywords associated with skin and connective tissue lesions induced by MP include toxic epidermal necrolysis (TEN), erythema multiforme (EM), Stevens–Johnson Syndrome (SJS), and mucositis. SJS and TEN are life-threatening dermatologic conditions characterized by extensive blistering, necrosis and desquamation of the skin (94). According to the affected body surface area (BSA), classifications include SJS (BSA <10%), SJS/TEN overlap (10% ≤BSA ≤30%), and TEN (BSA >30%) (95). SJS and TEN are predominantly triggered by adverse drug reactions or infections, with MP being the most commonly implicated infectious agent (94). EM is an acute, self-limiting dermatological disorder characterized by symmetrical targetoid erythematous lesions on the extremities, with occasional papules (96, 97). According to whether the mucosa is involved, EM can be subdivided into simple skin type and skin combined with mucosal type (98). Instances also exist where MP infection manifests solely as mucosal lesions without cutaneous involvement, previously defined variously as “atypical SJS”, “incomplete SJS”, or “EM without skin lesions” (96). However, these definitions often lead to confusion. Therefore, the American Academy of Dermatology introduced a new term in 2015, “Mycoplasma-induced rash and mucositis (MIRM)”, to encapsulate this condition, characterized by pronounced mucositis, absence of cutaneous lesions or manifesting only sparse vesiculobullous and/or targetoid eruptions, with a mild course and negligible mortality (99). For MP-associated SJS, TEN, and EM, the prevailing hypothesis posits that MP disseminates via the bloodstream to the skin, where it incites cytokine release and subsequent inflammatory blistering (89). The etiology of MIRM diverges; following MP infection, polyclonal B-cell proliferation ensues, leading to the production of specific antibodies and deposition of immune complexes in the skin, which subsequently activate cytotoxic T cells, resulting in mucosal injury (100).
If the patients with MPP still have persistent fever, unalleviated clinical symptoms and deteriorating radiographic findings after rational use of macrolide antibiotics for more than seven days, it is necessary to diagnose RMPP (101). Numerous factors contribute to the progression of MPP to RMPP, including an exaggerated host immune response, macrolide resistance, and polymicrobial infection (102). Early identification and timely therapeutic intervention are imperative for ameliorating the clinical course and prognosis of RMPP. Consequently, the identification of clinical prognostic indicators for RMPP remains a focal point of research. LDH is ubiquitously present in human tissues and serves as a frequently reported assessment metric (Cluster #3). Elevated LDH levels, released into the bloodstream due to increased cell membrane permeability during inflammation or tissue damage, offer significant diagnostic utility (103). Abundant studies corroborated that serum LDH levels were markedly elevated in RMPP and could serve as an early identification metric. However, consensus has yet to be reached regarding the predictive value of LDH in RMPP, and research findings presented some discrepancies. Zhang Y et al. conducted a retrospective analysis and ascertained that LDH ≥417 IU/L could serve as a predictive indicator for RMPP (22). Liu TY et al. determined an LDH threshold of 408 IU/L based on receiver operating characteristic curve analysis (104). Similarly, Lu A and colleagues identified an LDH level of ≥379 IU/L as indicative of a potential progression from MPP to RMPP (105). Inamura N et al. reported an LDH threshold of 410 IU/L (106). Collectively, these findings suggested an approximate LDH predictive value for RMPP around 400 IU/L. Further large-scale clinical studies are necessitated for the formulation of more precise and authoritative conclusions to guide clinical management. Additionally, other biomarkers such as CRP, erythrocyte sedimentation rate, percentage of neutrophils, 35 α-hydroxybutyrate dehydrogenase, D-dimer, procalcitonin, IL-6, IL-10, IL-18, interferon gamma (IFN-γ), and tumor necrosis factor alpha (TNF-α) have also been reported as early prognostic indicators for RMPP (22, 67, 103, 105, 107).
Regarding the therapeutic approaches for RMPP, key terms include “methylprednisolone pulse therapy” and “bronchoalveolar lavage fluid”. Severe pulmonary injury associated with RMPP is primarily attributed to an excessive host immune response rather than direct damage inflicted by MP (19). Glucocorticoids exhibit potent immunomodulatory properties (108). For RMPP, particularly severe cases, the combination of macrolide antibiotics and methylprednisolone pulse therapy is frequently utilized (109). However, a standardized glucocorticoid treatment regimen for pediatric MPP has yet to be established, and the dosage of methylprednisolone varies widely, ranging from 1 mg/kg/day to 30 mg/kg/day (102, 110). Both research groups led by You SY and Tamura A recommended a regimen of intravenous methylprednisolone at 30 mg/kg/day for three days for the treatment of severe RMPP. At the same time, they also emphasized that specific treatment dosages and duration should be adjusted according to the severity of the clinical presentation to prevent medication misuse (109, 111). The advent of bronchoscopic techniques marked a new phase in the diagnosis and treatment of RMPP. On one hand, bronchoalveolar lavage fluid serves as a lower respiratory tract sample, mitigating the interference from upper respiratory tract colonizing microbes, thereby offering compelling evidence for etiological diagnosis (112). On the other hand, therapeutic bronchoscopy can effectively remove mucus plugs, enhance pulmonary ventilation, and expedite the resolution of pulmonary inflammation (113). However, bronchoscopy is an invasive procedure; pediatricians should exercise caution in weighing the risks and benefits, generally reserving its use for cases with severe airway obstruction, such as those complicated by atelectasis or plastic bronchitis (102).
The pathogenicity of MP predominantly encompasses four aspects: direct adhesion-induced injury, exotoxin release, inflammatory damage, and immune evasion (114). In this investigation, salient keywords associated with pathogenic mechanisms include “cytokines” and “CARDS TX”, underscoring that inflammatory damage and exotoxin-mediated mechanisms are the focal points of foundational research. The MP surface harbors over 50 types of lipoproteins, the majority of which are implicated in inflammatory responses (114). These lipoproteins can be recognized by toll-like receptors (TLR1, TLR2, TLR4, and TLR6), thereby initiating the nuclear factor kappa B (NF-κB) signaling pathway and subsequently releasing a myriad of proinflammatory cytokines such as IL-6, TNF-α, and IL-1β (115, 116). These cytokines act as stimulants for human immune cells, eliciting neutrophil, lymphocyte, and macrophage infiltration into the bronchioles and alveoli, ultimately culminating in an inflammatory cascade (117). CARDS TX was initially reported in 2005 (118) and, as the sole identified exotoxin of MP, is garnering increasing attention for its role in the pathogenicity of MP (119). (i) CARDS TX can bind to human surfactant protein A, thereby promoting adhesion of MP to airway epithelial cells (120). (ii) CARDS TX, facilitated by clathrin-mediated endocytosis, gains intracellular entry and engenders the formation of perinuclear vesicles. These vesicles continually amalgamate and exert pressure on the nucleus, ultimately inducing cell death (121). The vesiculating toxicity of CARDS TX is closely aligned with the histopathological alterations in MPP, including vacuolar degeneration of ciliated cells and airway epithelial cells, leading to ciliary dysfunction and epithelial cells necrosis (119). (iii) The ADPRT activity of CARDS TX plays a pivotal role in the post-translational modification and activation of NLRP3 inflammasomes, thereby catalyzing the maturation and release of IL-1β, and triggering systemic inflammation (122). (iv) CARDS TX can induce T-cell differentiation into Th1 cells, generating IFN-γ, which in turn polarizes macrophages towards the M1 phenotype, secreting an array of chemokines. These chemokines further recruit more Th1 cells to the infection site, establishing a type Ⅰ immune response positive feedback loop and exacerbating pulmonary immune injury (123). (v) CARDS TX is implicated in the exacerbation of asthma caused by MP. Utilizing recombinant CARDS TX (rCARDS TX) to establish a murine model of airway inflammation, rCARDS TX elevated total and CARDS TX-specific serum IgE levels, triggered mast cell degranulation, and induced airway hyper-responsiveness (84, 124).
To the best of our knowledge, this constitutes the inaugural work employing bibliometric methodologies to delineate research trends in pediatric MPP. Our analysis elucidates key influential nations, institutions, and authors within this domain, as well as highlights research foci. Concurrently, we identify several pressing issues warranting immediate resolution within pediatric MPP research. For instance, there currently exists no therapeutic agents that can serve as impeccable substitutes for macrolide antibiotics in the pediatric population; the relative paucity of foundational research; and with regard to the diagnosis and treatment of RMPP in children, certain aspects have yet to coalesce into unified and unequivocal standards. Certainly, some limitations are inherent to our study. Initially, this study solely incorporates English-language literature indexed in WoSCC, which may engender omissions. However, this constraint is attributed to limitations inherent in alternative databases and bibliometric analysis software. For example, citation analysis is not feasible in the PubMed database; likewise, multi-language analysis is not supported by the Citespace software. Secondly, owing to the dynamic updates of the WoSCC database, our conclusions retain their applicability solely to the current juncture. Finally, in the analysis of countries, the impact of population size on the number of publications was not taken into account. In general, countries with larger populations have more research institutions and researchers, and naturally more publications. In future research, the interference of the above factors should be eliminated as much as possible to draw more accurate conclusions.
In recent years, there has been a precipitous uptick in the volume of publications on pediatric MPP, indicative of heightened academic and clinical interest in this malady. China and the United States emerge as the nations with the highest publication output. Italian scholar Susanna Esposito and Japanese scholar Kazunobu Ouchi are the most influential authors. Diagnostic technologies for MP, macrolide resistance, complications associated with MPP, clinical management and diagnosis of RMPP, and underlying pathogenic mechanisms constitute the current research hotspots. Our research offers a comprehensive overview of prevailing trends in pediatric MPP studies, thereby potentially expediting the resolution of intricate challenges in clinical diagnostics and treatment.
ZS: Writing – original draft. GJ: Methodology. GL: Software. Writing – original draft. CH: Supervision. BZ: Funding acquisition, Project administration, Supervision. XW: Resources, Supervision.
The author(s) declare financial support was received for the research, authorship, and/or publication of this article.
This research was supported by Shandong Province Integrated Traditional Chinese and Western Medicine Professional Disease Prevention and Control Project (No. YXH2019ZXY010).
The authors declare that the research was conducted in the absence of any commercial or financial relationships that could be construed as a potential conflict of interest.
All claims expressed in this article are solely those of the authors and do not necessarily represent those of their affiliated organizations, or those of the publisher, the editors and the reviewers. Any product that may be evaluated in this article, or claim that may be made by its manufacturer, is not guaranteed or endorsed by the publisher.
The Supplementary Material for this article can be found online at: https://www.frontiersin.org/articles/10.3389/fped.2023.1306234/full#supplementary-material
Supplementary Table S1
The h-index of 6,972 authors.
Supplementary Table S2
The annual average citations for references.
Supplementary Table S3
Combination and replacement of keywords.
1. Kumar S, Kumar S. Mycoplasma pneumoniae: among the smallest bacterial pathogens with great clinical significance in children. Indian J Med Microbiol. (2023) 46:100480. doi: 10.1016/j.ijmmb.2023.100480
2. Kumar S. Mycoplasma pneumoniae: a significant but underrated pathogen in paediatric community-acquired lower respiratory tract infections. Indian J Med Res. (2018) 147(1):23–31. doi: 10.4103/ijmr.IJMR_1582_16
3. Jacobs E, Ehrhardt I, Dumke R. New insights in the outbreak pattern of Mycoplasma pneumoniae. Int J Med Microbiol. (2015) 305(7):705–8. doi: 10.1016/j.ijmm.2015.08.021
4. Liu JR, He RX, Zhang XY, Zhao F, Liu LY, Wang H, et al. Clinical features and “early” corticosteroid treatment outcome of pediatric Mycoplasma pneumoniae pneumonia. Front Cell Infect Microbiol. (2023) 13:10. doi: 10.3389/fcimb.2023.1135228
5. Atkinson TP, Waites KB. Mycoplasma pneumoniae infections in childhood. Pediatr Infect Dis J. (2014) 33(1):92–4. doi: 10.1097/inf.0000000000000171
6. Gadsby NJ, Reynolds AJ, McMenamin J, Gunson RN, McDonagh S, Molyneaux PJ, et al. Increased reports of Mycoplasma pneumoniae from laboratories in Scotland in 2010 and 2011—impact of the epidemic in infants. Euro Surveill. (2012) 17(10):15–8. doi: 10.2807/ese.17.10.20110-en
7. Poddighe D, Demirkaya E, Sazonov V, Romano M. Mycoplasma pneumoniae infections and primary immune deficiencies. Int J Clin Pract. (2022) 2022:6. doi: 10.1155/2022/6343818
8. Lu C, Qi H, Xu H, Hao Y, Yang Z, Yu W, et al. Global research trends of steroid-induced osteonecrosis of the femoral head: a 30-year bibliometric analysis. Front Endocrinol (Lausanne). (2022) 13:1027603. doi: 10.3389/fendo.2022.1027603
9. Luo F, Li Y, Zhang Y, Song Y, Diao J. Bibliometric analysis of IgA vasculitis nephritis in children from 2000 to 2022. Front Public Health. (2022) 10:1020231. doi: 10.3389/fpubh.2022.1020231
10. Hirsch JE. An index to quantify an individual’s scientific research output. Proc Natl Acad Sci U S A. (2005) 102(46):16569–72. doi: 10.1073/pnas.0507655102
11. Koo M. Systemic lupus erythematosus research: a bibliometric analysis over a 50-year period. Int J Environ Res Public Health. (2021) 18(13):7095. doi: 10.3390/ijerph18137095
12. Wu H, Cheng K, Tong L, Wang Y, Yang W, Sun Z. Knowledge structure and emerging trends on osteonecrosis of the femoral head: a bibliometric and visualized study. J Orthop Surg Res. (2022) 17(1):194. doi: 10.1186/s13018-022-03068-7
13. Waites KB, Xiao L, Liu Y, Balish MF, Atkinson TP. Mycoplasma pneumoniae from the respiratory tract and beyond. Clin Microbiol Rev. (2017) 30(3):747–809. doi: 10.1128/cmr.00114-16
14. Kutty PK, Jain S, Taylor TH, Bramley AM, Diaz MH, Ampofo K, et al. Mycoplasma pneumoniae among children hospitalized with community-acquired pneumonia. Clin Infect Dis. (2019) 68(1):5–12. doi: 10.1093/cid/ciy419
15. Jain S, Williams DJ, Arnold SR, Ampofo K, Bramley AM, Reed C, et al. Community-acquired pneumonia requiring hospitalization among US children. N Engl J Med. (2015) 372(9):835–45. doi: 10.1056/NEJMoa1405870
16. Lee H, Yun KW, Lee HJ, Choi EH. Antimicrobial therapy of macrolide-resistant Mycoplasma pneumoniae pneumonia in children. Expert Rev Anti-Infect Ther. (2018) 16(1):23–34. doi: 10.1080/14787210.2018.1414599
17. Yang TI, Chang TH, Lu CY, Chen JM, Lee PI, Huang LM, et al. Mycoplasma pneumoniae in pediatric patients: do macrolide-resistance and/or delayed treatment matter? J Microbiol Immunol Infect. (2019) 52(2):329–35. doi: 10.1016/j.jmii.2018.09.009
18. Chen YC, Hsu WY, Chang TH. Macrolide-resistant Mycoplasma pneumoniae infections in pediatric community-acquired pneumonia. Emerg Infect Dis. (2020) 26(7):1382–91. doi: 10.3201/eid2607.200017
19. Tsai TA, Tsai CK, Kuo KC, Yu HR. Rational stepwise approach for Mycoplasma pneumoniae pneumonia in children. J Microbiol Immunol Infect. (2021) 54(4):557–65. doi: 10.1016/j.jmii.2020.10.002
20. Gao LW, Yin J, Hu YH, Liu XY, Feng XL, He JX, et al. The epidemiology of paediatric Mycoplasma pneumoniae pneumonia in north China: 2006–2016. Epidemiol Infect. (2019) 147:5. doi: 10.1017/s0950268819000839
21. Sondergaard MJ, Friis MB, Hansen DS, Jorgensen IM. Clinical manifestations in infants and children with Mycoplasma pneumoniae infection. PLoS One. (2018) 13(4):10. doi: 10.1371/journal.pone.0195288
22. Zhang YY, Zhou YL, Li SX, Yang DH, Wu XL, Chen ZM. The clinical characteristics and predictors of refractory Mycoplasma pneumoniae pneumonia in children. PLoS One. (2016) 11(5):10. doi: 10.1371/journal.pone.0156465
23. Yuan Y, Jiao B, Qu L, Yang D, Liu R. The development of COVID-19 treatment. Front Immunol. (2023) 14:1125246. doi: 10.3389/fimmu.2023.1125246
24. To KK, Sridhar S, Chiu KH, Hung DL, Li X, Hung IF, et al. Lessons learned 1 year after sars-cov-2 emergence leading to COVID-19 pandemic. Emerg Microbes Infect. (2021) 10(1):507–35. doi: 10.1080/22221751.2021.1898291
25. Zhang J, Yang T, Zou MJ, Wang LL, Sai L. The epidemiological features of respiratory tract infection using the multiplex panels detection during COVID-19 pandemic in Shandong province, China. Sci Rep. (2023) 13(1):8. doi: 10.1038/s41598-023-33627-9
26. Wang HP, Zheng YJ, de Jonge MI, Wang RJ, Verhagen LM, Chen YS, et al. Lockdown measures during the COVID-19 pandemic strongly impacted the circulation of respiratory pathogens in southern China. Sci Rep. (2022) 12(1):8. doi: 10.1038/s41598-022-21430-x
27. Xu MH, Liu PC, Su LY, Cao LF, Zhong HQ, Lu LJ, et al. Comparison of respiratory pathogens in children with lower respiratory tract infections before and during the COVID-19 pandemic in Shanghai, China. Front Pediatr. (2022) 10:8. doi: 10.3389/fped.2022.881224
28. Cheng Y, Cheng YJ, Dai SZ, Hou DQ, Ge ML, Zhang Y, et al. The prevalence of Mycoplasma pneumoniae among children in Beijing before and during the COVID-19 pandemic. Front Cell Infect Microbiol. (2022) 12:6. doi: 10.3389/fcimb.2022.854505
29. Roh EJ, Lee MH, Lee JY, Kim HB, Ahn YM, Kim JK, et al. Analysis of national surveillance of respiratory pathogens for community-acquired pneumonia in children and adolescents. BMC Infect Dis. (2022) 22(1):13. doi: 10.1186/s12879-022-07263-z
30. Esposito S, Blasi F, Bellini F, Allegra L, Principi N, Mowgli Study G. Mycoplasma pneumoniae and chlamydia pneumoniae infections in children with pneumonia. Eur Resp J. (2001) 17(2):241–5. doi: 10.1183/09031936.01.17202410
31. Esposito S, Blasi F, Arosio C, Fioravanti L, Fagetti L, Droghetti R, et al. Importance of acute Mycoplasma pneumoniae and chlamydia pneumoniae infections in children with wheezing. Eur Resp J. (2000) 16(6):1142–6. doi: 10.1034/j.1399-3003.2000.16f21.x
32. Esposito S, Cavagna R, Bosis S, Droghetti R, Faelli N, Principi N. Emerging role of Mycoplasma pneumoniae in children with acute pharyngitis. Eur J Clin Microbiol Infect Dis. (2002) 21(8):607–10. doi: 10.1007/s10096-002-0780-7
33. D'Alonzo R, Mencaroni E, Di Genova L, Laino D, Principi N, Esposito S. Pathogenesis and treatment of neurologic diseases associated with Mycoplasma pneumoniae infection. Front Microbiol. (2018) 9:9. doi: 10.3389/fmicb.2018.02751
34. Esposito S, Tagliabue C, Bosis S, Principi N. Levofloxacin for the treatment of Mycoplasma pneumoniae-associated meningoencephalitis in childhood. Int J Antimicrob Agents. (2011) 37(5):472–5. doi: 10.1016/j.ijantimicag.2011.01.008
35. Tanaka T, Oishi T, Miyata I, Wakabayashi S, Kono M, Ono S, et al. Macrolide-resistant Mycoplasma pneumoniae infection, Japan, 2008–2015. Emerg Infect Dis. (2017) 23(10):1703–6. doi: 10.3201/eid2310.170106
36. Oishi T, Yoshioka D, Nakano T, Ouchi K. Recent trend of antimicrobial susceptibility among Mycoplasma pneumoniae isolated from Japanese children. Microorganisms. (2022) 10(12):8. doi: 10.3390/microorganisms10122428
37. Matsuoka M, Narita M, Okazaki N, Ohya H, Yamazaki T, Ouchi K, et al. Characterization and molecular analysis of macrolide-resistant Mycoplasma pneumoniae clinical isolates obtained in Japan. Antimicrob Agents Chemother. (2004) 48(12):4624–30. doi: 10.1128/aac.48.12.4624-4630.2004
38. Kawai Y, Miyashita N, Kubo M, Akaike H, Kato A, Nishizawa Y, et al. Therapeutic efficacy of macrolides, minocycline, and tosufloxacin against macrolide-resistant Mycoplasma pneumoniae pneumonia in pediatric patients. Antimicrob Agents Chemother. (2013) 57(5):2252–8. doi: 10.1128/aac.00048-13
39. Kawai Y, Miyashita N, Yamaguchi T, Saitoh A, Kondoh E, Fujimoto H, et al. Clinical efficacy of macrolide antibiotics against genetically determined macrolide-resistant Mycoplasma pneumoniae pneumonia in paediatric patients. Respirology. (2012) 17(2):354–62. doi: 10.1111/j.1440-1843.2011.02102.x
40. Jiang XW, Huang TS, Xie L, Chen SZ, Wang SD, Huang ZW, et al. Development of a diagnostic assay by three-tube multiplex real-time PCR for simultaneous detection of nine microorganisms causing acute respiratory infections. Sci Rep. (2022) 12(1):17. doi: 10.1038/s41598-022-15543-6
41. Tuo W, Guo X, Wu M, Xie S, Shen X, Wang J, et al. Application value of antibody titres and RNA detection in the early prediction of Mycoplasma pneumoniae pneumonia in children: a retrospective study. BMC Infect Dis. (2023) 23(1):10. doi: 10.1186/s12879-023-08161-8
42. Montagnani F, Rossetti B, Vannoni A, Cusi MG, De Luca A. Laboratory diagnosis of Mycoplasma pneumoniae infections: data analysis from clinical practice. New Microbiol. (2018) 41(3):203–7. PMID: 29874388
43. Loens K, Goossens H, Ieven M. Acute respiratory infection due to Mycoplasma pneumoniae: current status of diagnostic methods. Eur J Clin Microbiol Infect Dis. (2010) 29(9):1055–69. doi: 10.1007/s10096-010-0975-2
44. Atkinson TP, Balish MF, Waites KB. Epidemiology, clinical manifestations, pathogenesis and laboratory detection of Mycoplasma pneumoniae infections. FEMS Microbiol Rev. (2008) 32(6):956–73. doi: 10.1111/j.1574-6976.2008.00129.x
45. Ferwerda A, Moll HA, de Groot R. Respiratory tract infections by Mycoplasma pneumoniae in children: a review of diagnostic and therapeutic measures. Eur J Pediatr. (2001) 160(8):483–91. doi: 10.1007/s004310100775
46. Leng MD, Yang JM, Zhou JJ. The molecular characteristics, diagnosis, and treatment of macrolide-resistant Mycoplasma pneumoniae in children. Front Pediatr. (2023) 11:9. doi: 10.3389/fped.2023.1115009
47. Ozaki T, Nishimura N, Ahn J, Watanabe N, Muto T, Saito A, et al. Utility of a rapid diagnosis kit for Mycoplasma pneumoniae pneumonia in children, and the antimicrobial susceptibility of the isolates. J Infect Chemother. (2007) 13(4):204–7. doi: 10.1007/s10156-007-0519-6
48. Saranglao A, Smith PR. Diagnostic tests for CAP: current approaches and future perspectives. Expert Rev Mol Diagn. (2002) 2(4):329–36. doi: 10.1586/14737159.2.4.329
49. Loens K, Ieven M. Mycoplasma pneumoniae: current knowledge on nucleic acid amplification techniques and serological diagnostics. Front Microbiol. (2016) 7:8. doi: 10.3389/fmicb.2016.00448
50. Zhang L, Zong ZY, Liu YB, Ye H, Lv XJ. PCR versus serology for diagnosing Mycoplasma pneumoniae infection: a systematic review & meta-analysis. Indian J Med Res. (2011) 134(3):270–80. PMID: 21985809; PMCID: PMC3193707
51. Liu FC, Chen PY, Huang F, Tsai CR, Lee CY, Wang LC. Rapid diagnosis of Mycoplasma pneumoniae infection in children by polymerase chain reaction. J Microbiol Immunol Infect. (2007) 40(6):507–12. PMID: 18087631
52. Nilsson AC, Björkman P, Persson K. Polymerase chain reaction is superior to serology for the diagnosis of acute Mycoplasma pneumoniae infection and reveals a high rate of persistent infection. BMC Microbiol. (2008) 8:93. doi: 10.1186/1471-2180-8-93
53. Loens K, Ursi D, Goossens H, Ieven M. Molecular diagnosis of Mycoplasma pneumoniae respiratory tract infections. J Clin Microbiol. (2003) 41(11):4915–23. doi: 10.1128/jcm.41.11.4915-4923.2003
54. Sung M, Roh EJ, Lee ES, Lee JY, Kim HB, Ahn Y, et al. Assessment of variables associated with prolonged admission duration in children with Mycoplasma pneumoniae pneumonia. Clin Respir J. (2022) 16(11):756–67. doi: 10.1111/crj.13549
55. Hsiung JCC, Ma HY, Lu CY, Yen TY, Chi H, Liau YJ, et al. Children with Mycoplasma pneumoniae infection in Taiwan: changes in molecular characteristics and clinical outcomes. J Formos Med Assoc. (2022) 121(11):2273–80. doi: 10.1016/j.jfma.2022.05.001
56. Vázquez-Laslop N, Mankin AS. How macrolide antibiotics work. Trends Biochem Sci. (2018) 43(9):668–84. doi: 10.1016/j.tibs.2018.06.011
57. Okazaki N, Narita M, Yamada S, Izumikawa K, Umetsu M, Kenri T, et al. Characteristics of macrolide-resistant Mycoplasma pneumoniae strains isolated from patients and induced with erythromycin in vitro. Microbiol Immunol. (2001) 45(8):617–20. doi: 10.1111/j.1348-0421.2001.tb01293.x
58. Pereyre S, Guyot C, Renaudin H, Charron A, Bébéar C, Bébéar CM. In vitro selection and characterization of resistance to macrolides and related antibiotics in Mycoplasma pneumoniae. Antimicrob Agents Chemother. (2004) 48(2):460–5. doi: 10.1128/aac.48.2.460-465.2004
59. Cardinale F, Chironna M, Dumke R, Binetti A, Daleno C, Sallustio A, et al. Macrolide-resistant Mycoplasma pneumoniae in paediatric pneumonia. Eur Respir J. (2011) 37(6):1522–4. doi: 10.1183/09031936.00172510
60. Wang GT, Wu P, Tang R, Zhang WD. Global prevalence of resistance to macrolides in Mycoplasma pneumoniae: a systematic review and meta-analysis. J Antimicrob Chemother. (2022) 77(9):2353–63. doi: 10.1093/jac/dkac170
61. Wang N, Zhang H, Yin YH, Xu XG, Xiao L, Liu Y. Antimicrobial susceptibility profiles and genetic characteristics of Mycoplasma pneumoniae in Shanghai, China, from 2017 to 2019. Infect Drug Resistance. (2022) 15:4443–52. doi: 10.2147/idr.S370126
62. Dégrange S, Cazanave C, Charron A, Renaudin H, Bébéar C, Bébéar CM. Development of multiple-locus variable-number tandem-repeat analysis for molecular typing of Mycoplasma pneumoniae. J Clin Microbiol. (2009) 47(4):914–23. doi: 10.1128/jcm.01935-08
63. Sun HM, Xue GH, Yan C, Li SL, Cao L, Yuan Y, et al. Multiple-locus variable-number tandem-repeat analysis of Mycoplasma pneumoniae clinical specimens and proposal for amendment of MLVA nomenclature. PLoS One. (2013) 8(5):7. doi: 10.1371/journal.pone.0064607
64. Ho PL, Law PY, Chan BW, Wong CW, To KK, Chiu SS, et al. Emergence of macrolide-resistant Mycoplasma pneumoniae in Hong Kong is linked to increasing macrolide resistance in multilocus variable-number tandem-repeat analysis type 4-5-7-2. J Clin Microbiol. (2015) 53(11):3560–4. doi: 10.1128/jcm.01983-15
65. Berlot JR, Mrvic T, Kese D. Mycoplasma pneumoniae multilocus variable-number tandem-repeat analysis genotypes are associated with inflammatory biomarker levels in children with lower respiratory tract infections. Eur J Clin Microbiol Infect Dis. (2022) 41(8):1099–105. doi: 10.1007/s10096-022-04467-8
66. Lung DC, Lam DSY, Chan E, Chan KC, Chiu SSS, Ho PL, et al. Practice recommendations for management of community acquired pneumonia in children. Hong Kong J Paediatr. (2016) 21(3):178–93.
67. Xie QY, Zhang XT, Cui W, Pang YJ. Construction of a nomogram for identifying refractory Mycoplasma pneumoniae pneumonia among macrolide-unresponsive Mycoplasma pneumoniae pneumonia in children. J Inflamm Res. (2022) 15:6495–504. doi: 10.2147/jir.S387809
68. Oishi T, Ouchi K. Recent trends in the epidemiology, diagnosis, and treatment of macrolide-resistant Mycoplasma pneumoniae. J Clin Med. (2022) 11(7):8. doi: 10.3390/jcm11071782
69. Okada T, Morozumi M, Tajima T, Hasegawa M, Sakata H, Ohnari S, et al. Rapid effectiveness of minocycline or doxycycline against macrolide-resistant Mycoplasma pneumoniae infection in a 2011 outbreak among Japanese children. Clin Infect Dis. (2012) 55(12):1642–9. doi: 10.1093/cid/cis784
70. Hardy RD, Jafri HS, Olsen K, Wordemann M, Hatfield J, Rogers BB, et al. Elevated cytokine and chemokine levels and prolonged pulmonary airflow resistance in a murine Mycoplasma pneumoniae pneumonia model: a microbiologic, histologic, immunologic, and respiratory plethysmographic profile. Infect Immun. (2001) 69(6):3869–76. doi: 10.1128/iai.69.6.3869-3876.2001
71. Mims JW. Asthma: definitions and pathophysiology. Int Forum Allergy Rhinol. (2015) 5(Suppl 1):S2–6. doi: 10.1002/alr.21609
72. Atkinson TP, Duffy LB, Pendley D, Dai YL, Cassell GH. Deficient immune response to Mycoplasma pneumoniae in childhood asthma. Allergy Asthma Proc. (2009) 30(2):158–65. doi: 10.2500/aap.2009.30.3207
73. Yeh JJ, Wang YC, Hsu WH, Kao CH. Incident asthma and Mycoplasma pneumoniae: a nationwide cohort study. J Allergy Clin Immunol. (2016) 137(4):1017–U566. doi: 10.1016/j.jaci.2015.09.032
74. Wood PR, Kampschmidt JC, Dube PH, Cagle MP, Chaparro P, Ketchum NS, et al. Mycoplasma pneumoniae and health outcomes in children with asthma. Ann Allergy Asthma Immunol. (2017) 119(2):146. doi: 10.1016/j.anai.2017.05.022
75. Wood PR, Hill VL, Burks ML, Peters JI, Singh H, Kannan TR, et al. Mycoplasma pneumoniae in children with acute and refractory asthma. Ann Allergy Asthma Immunol. (2013) 110(5):328. doi: 10.1016/j.anai.2013.01.022
76. Biscardi S, Lorrot M, Marc E, Moulin F, Boutonnat-Faucher B, Heilbronner C, et al. Mycoplasma pneumoniae and asthma in children. Clin Infect Dis. (2004) 38(10):1341–6. doi: 10.1086/392498
77. Simpson JL, Powell H, Boyle MJ, Scott RJ, Gibson PG. Clarithromycin targets neutrophilic airway inflammation in refractory asthma. Am J Respir Crit Care Med. (2008) 177(2):148–55. doi: 10.1164/rccm.200707-1134OC
78. Fonseca-Aten M, Okada PJ, Bowlware KL, Chavez-Bueno S, Mejias A, Rios AM, et al. Effect of clarithromycin on cytokines and chemokines in children with an acute exacerbation of recurrent wheezing: a double-blind, randomized, placebo-controlled trial. Ann Allergy Asthma Immunol. (2006) 97(4):457–63. doi: 10.1016/s1081-1206(10)60935-0
79. Koutsoubari I, Papaevangelou V, Konstantinou GN, Makrinioti H, Xepapadaki P, Kafetzis D, et al. Effect of clarithromycin on acute asthma exacerbations in children: an open randomized study. Pediatr Allergy Immunol. (2012) 23(4):385–90. doi: 10.1111/j.1399-3038.2012.01280.x
80. Wong EH, Porter JD, Edwards MR, Johnston SL. The role of macrolides in asthma: current evidence and future directions. Lancet Respir Med. (2014) 2(8):657–70. doi: 10.1016/s2213-2600(14)70107-9
81. Liu Y, Ai T, Fan Y, Xie C, Lou R, Zeng X, et al. Etiological distribution of pertussis-like syndrome in 756 children in Chengdu. Transl Pediatr. (2021) 10(4):984–9. doi: 10.21037/tp-21-140
82. Xiong Q, Hao S, Shen L, Liu J, Chen T, Zhang G, et al. Pertussis-like syndrome often not associated with Bordetella pertussis: 5-year study in a large children’s hospital. Infectious Diseases (Lond). (2020) 52(10):736–42. doi: 10.1080/23744235.2020.1784995
83. Gu W, Wang K, Zhang X, Hao C, Lu Y, Wu M, et al. Pathogen analysis of pertussis-like syndrome in children. BMC Infect Dis. (2020) 20(1):353. doi: 10.1186/s12879-020-05074-8
84. Hardy RD, Coalson JJ, Peters J, Chaparro A, Techasaensiri C, Cantwell AM, et al. Analysis of pulmonary inflammation and function in the mouse and baboon after exposure to Mycoplasma pneumoniae cards toxin. PLoS One. (2009) 4(10):e7562. doi: 10.1371/journal.pone.0007562
85. Kilgore PE, Salim AM, Zervos MJ, Schmitt HJ. Pertussis: microbiology, disease, treatment, and prevention. Clin Microbiol Rev. (2016) 29(3):449–86. doi: 10.1128/cmr.00083-15
86. Gerber MA, Randolph MF, Chanatry J, Mayo DR, Schachter J, Tilton RC. Role of Chlamydia trachomatis and Mycoplasma pneumoniae in acute pharyngitis in children. Diagn Microbiol Infect Dis. (1987) 6(3):263–5. doi: 10.1016/0732-8893(87)90021-6
87. Fernández de Sevilla M, Alayeto J, Fernández Y, Muñoz-Almagro C, Luaces C, García-García JJ. Low prevalence of Mycoplasma pneumoniae infection in children with acute pharyngitis. Enferm Infecc Microbiol Clin. (2009) 27(7):403–5. doi: 10.1016/j.eimc.2008.06.009
88. Narita M. Pathogenesis of extrapulmonary manifestations of Mycoplasma pneumoniae infection with special reference to pneumonia. J Infect Chemother. (2010) 16(3):162–9. doi: 10.1007/s10156-010-0044-x
89. Narita M. Classification of extrapulmonary manifestations due to Mycoplasma pneumoniae infection on the basis of possible pathogenesis. Front Microbiol. (2016) 7:23. doi: 10.3389/fmicb.2016.00023
90. Narita M. Pathogenesis of neurologic manifestations of Mycoplasma pneumoniae infection. Pediatr Neurol. (2009) 41(3):159–66. doi: 10.1016/j.pediatrneurol.2009.04.012
91. Narita M, Itakura O, Matsuzono Y, Togashi T. Analysis of mycoplasmal central nervous system involvement by polymerase chain reaction. Pediatr Infect Dis J. (1995) 14(3):236–7. doi: 10.1097/00006454-199503000-00013
92. Narita M, Tanaka H, Togashi T, Abe S. Cytokines involved in CNS manifestations caused by Mycoplasma pneumoniae. Pediatr Neurol. (2005) 33(2):105–9. doi: 10.1016/j.pediatrneurol.2005.03.003
93. Kuwahara M, Samukawa M, Ikeda T, Morikawa M, Ueno R, Hamada Y, et al. Characterization of the neurological diseases associated with Mycoplasma pneumoniae infection and anti-glycolipid antibodies. J Neurol. (2017) 264(3):467–75. doi: 10.1007/s00415-016-8371-1
94. Chi MH, Chung WH, Hui RCY, Chen CB, Lu CW, Chiu TM, et al. Clinical features and outcomes in children with Stevens–Johnson syndrome and toxic epidermal necrolysis. J Dermatol. (2022) 49(9):895–902. doi: 10.1111/1346-8138.16476
95. Frantz R, Huang S, Are A, Motaparthi K. Stevens–Johnson syndrome and toxic epidermal necrolysis: a review of diagnosis and management. Medicina (Kaunas). (2021) 57(9):895. doi: 10.3390/medicina57090895
96. De Luigi G, Meoli M, Zgraggen L, Kottanattu L, Simonetti GD, Terrani I, et al. Mucosal respiratory syndrome: a systematic literature review. Dermatology. (2022) 238(1):53–9. doi: 10.1159/000514815
97. Trayes KP, Love G, Studdiford JS. Erythema multiforme: recognition and management. Am Fam Physician. (2019) 100(2):82–8. PMID: 31305041
98. Lerch M, Mainetti C, Beretta-Piccoli BT, Harr T. Current perspectives on Erythema multiforme. Clin Rev Allergy Immunol. (2018) 54(1):177–84. doi: 10.1007/s12016-017-8667-7
99. Canavan TN, Mathes EF, Frieden I, Shinkai K. Mycoplasma pneumoniae-induced rash and mucositis as a syndrome distinct from Stevens–Johnson syndrome and Erythema multiforme: a systematic review. J Am Acad Dermatol. (2015) 72(2):239–45. doi: 10.1016/j.jaad.2014.06.026
100. Chen N, Li M. Case report and literature review: clinical characteristics of 10 children with Mycoplasma pneumoniae-induced rash and mucositis. Front Pediatr. (2022) 10:9. doi: 10.3389/fped.2022.823376
101. Fu Y, Zhang TQ, Dong CJ, Xu YS, Dong HQ, Ning J. Clinical characteristics of 14 pediatric Mycoplasma pneumoniae pneumonia associated thrombosis: a retrospective study. BMC Cardiovasc Disord. (2023) 23(1):9. doi: 10.1186/s12872-022-03030-9
102. Tong L, Huang SM, Zheng C, Zhang YY, Chen ZM. Refractory Mycoplasma pneumoniae pneumonia in children: early recognition and management. J Clin Med. (2022) 11(10):13. doi: 10.3390/jcm11102824
103. Shen FF, Dong CJ, Zhang TQ, Yu CJ, Jiang K, Xu YS, et al. Development of a nomogram for predicting refractory Mycoplasma pneumoniae pneumonia in children. Front Pediatr. (2022) 10:9. doi: 10.3389/fped.2022.813614
104. Liu TY, Lee WJ, Tsai CM, Kuo KC, Lee CH, Hsieh KS, et al. Serum lactate dehydrogenase isoenzymes 4 plus 5 is a better biomarker than total lactate dehydrogenase for refractory Mycoplasma pneumoniae pneumonia in children. Pediatr Neonatol. (2018) 59(5):501–6. doi: 10.1016/j.pedneo.2017.12.008
105. Lu AZ, Wang CK, Zhang XB, Wang LB, Qian LL. Lactate dehydrogenase as a biomarker for prediction of refractory Mycoplasma pneumoniae pneumonia in children. Respir Care. (2015) 60(10):1469–75. doi: 10.4187/respcare.03920
106. Inamura N, Miyashita N, Hasegawa S, Kato A, Fukuda Y, Saitoh A, et al. Management of refractory Mycoplasma pneumoniae pneumonia: utility of measuring serum lactate dehydrogenase level. J Infect Chemother. (2014) 20(3–4):270–3. doi: 10.1016/j.jiac.2014.01.001
107. Zhang YY, Mei SF, Zhou YL, Huang MX, Dong GJ, Chen ZM. Cytokines as the good predictors of refractory Mycoplasma pneumoniae pneumonia in school-aged children. Sci Rep. (2016) 6:6. doi: 10.1038/srep37037
108. Deng F, Cao HL, Liang XH, Li QB, Yang Y, Zhao ZH, et al. Analysis of cytokine levels, cytological findings, and mp-DNA level in bronchoalveolar lavage fluid of children with Mycoplasma pneumoniae pneumonia. Immun Inflamm Dis. (2023) 11(5):19. doi: 10.1002/iid3.849
109. You SY, Jwa HJ, Yang EA, Kil HR, Lee JH. Effects of methylprednisolone pulse therapy on refractory Mycoplasma pneumoniae pneumonia in children. Allergy Asthma Immunol Res. (2014) 6(1):22–6. doi: 10.4168/aair.2014.6.1.22
110. Sun LL, Ye C, Zhou YL, Zuo SR, Deng ZZ, Wang CJ. Meta-analysis of the clinical efficacy and safety of high- and low-dose methylprednisolone in the treatment of children with severe Mycoplasma pneumoniae pneumonia. Pediatr Infect Dis J. (2020) 39(3):177–83. doi: 10.1097/inf.0000000000002529
111. Tamura A, Matsubara K, Tanaka T, Nigami H, Yura K, Fukaya T. Methylprednisolone pulse therapy for refractory Mycoplasma pneumoniae pneumonia in children. J Infect. (2008) 57(3):223–8. doi: 10.1016/j.jinf.2008.06.012
112. Wang LJ, Xie QL, Xu SS, Li HJ, Zhang LY, Ai JW, et al. The role of flexible bronchoscopy in children with Mycoplasma pneumoniae pneumonia. Pediatr Res. (2023) 93(1):198–206. doi: 10.1038/s41390-021-01874-z
113. Lu JM, Zhang JQ, Wang GF, Zhang XB, Li ZP. Effects of bronchoalveolar lavage on Mycoplasma pneumoniae pneumonia: a propensity score matched-cohort study. Front Pediatr. (2023) 10:8. doi: 10.3389/fped.2022.1066640
114. Jiang ZL, Li SH, Zhu CM, Zhou RJ, Leung PHM. Mycoplasma pneumoniae infections: pathogenesis and vaccine development. Pathogens. (2021) 10(2):18. doi: 10.3390/pathogens10020119
115. Naghib M, Hatam-Jahromi M, Niktab M, Ahmadi R, Kariminik A. Mycoplasma pneumoniae and toll-like receptors: a mutual avenue. Allergol Immunopath. (2018) 46(5):508–13. doi: 10.1016/j.aller.2017.09.021
116. Shimizu T, Kida Y, Kuwano K. A dipalmitoylated lipoprotein from Mycoplasma pneumoniae activates NF-kappa B through TLR1, TLR2, and TLR6. J Immunol. (2005) 175(7):4641–6. doi: 10.4049/jimmunol.175.7.4641
117. Chaudhry R, Ghosh A, Chandolia A. Pathogenesis of Mycoplasma pneumoniae: an update. Indian J Med Microbiol. (2016) 34(1):7–16. doi: 10.4103/0255-0857.174112
118. Kannan TR, Provenzano D, Wright JR, Baseman JB. Identification and characterization of human surfactant protein a binding protein of Mycoplasma pneumoniae. Infect Immun. (2005) 73(5):2828–34. doi: 10.1128/iai.73.5.2828-2834.2005
119. Su XL, You XX, Luo HD, Liang KY, Chen L, Tian W, et al. Community-acquired respiratory distress syndrome toxin: unique exotoxin for M. Pneumoniae. Front Microbiol. (2021) 12:13. doi: 10.3389/fmicb.2021.766591
120. Becker A, Kannan TR, Taylor AB, Pakhomova ON, Zhang Y, Somarajan SR, et al. Structure of cards toxin, a unique ADP-ribosylating and vacuolating cytotoxin from Mycoplasma Pneumoniae. Proc Natl Acad Sci U S A. (2015) 112(16):5165–70. doi: 10.1073/pnas.1420308112
121. Krishnan M, Kannan TR, Baseman JB. Mycoplasma pneumoniae cards toxin is internalized via clathrin-mediated endocytosis. PLoS One. (2013) 8(5):e62706. doi: 10.1371/journal.pone.0062706
122. Bose S, Segovia JA, Somarajan SR, Chang TH, Kannan TR, Baseman JB. Adp-ribosylation of NLRP3 by Mycoplasma pneumoniae cards toxin regulates inflammasome activity. mBio. (2014) 5(6):e02186-14. doi: 10.1128/mBio.02186-14
123. Wang T, Sun HM, Lu ZT, Jiang WJ, Dai G, Huang L, et al. The cards toxin of Mycoplasma pneumoniae induces a positive feedback loop of type 1 immune response. Front Immunol. (2022) 13:14. doi: 10.3389/fimmu.2022.1054788
Keywords: Mycoplasma pneumoniae pneumonia (MPP), children, bibliometric analysis, research trends, hotspots
Citation: Song Z, Jia G, Luo G, Han C, Zhang B and Wang X (2023) Global research trends of Mycoplasma pneumoniae pneumonia in children: a bibliometric analysis. Front. Pediatr. 11:1306234. doi: 10.3389/fped.2023.1306234
Received: 3 October 2023; Accepted: 13 November 2023;
Published: 24 November 2023.
Edited by:
Stijn Verhulst, University of Antwerp, BelgiumReviewed by:
Ine Ilona Decuyper, Institute of Tropical Medicine Antwerp, Belgium© 2023 Song, Jia, Luo, Han, Zhang and Wang. This is an open-access article distributed under the terms of the Creative Commons Attribution License (CC BY). The use, distribution or reproduction in other forums is permitted, provided the original author(s) and the copyright owner(s) are credited and that the original publication in this journal is cited, in accordance with accepted academic practice. No use, distribution or reproduction is permitted which does not comply with these terms.
*Correspondence: Baoqing Zhang NzEwMDA5MjNAc2R1dGNtLmVkdS5jbg== Xiao Wang MjAxNzEwMTA2OUBzZHV0Y20uZWR1LmNu
Disclaimer: All claims expressed in this article are solely those of the authors and do not necessarily represent those of their affiliated organizations, or those of the publisher, the editors and the reviewers. Any product that may be evaluated in this article or claim that may be made by its manufacturer is not guaranteed or endorsed by the publisher.
Research integrity at Frontiers
Learn more about the work of our research integrity team to safeguard the quality of each article we publish.