- Department of Orthopedic Surgery, Shanghai Children’s Medical Center Affiliated to Shanghai Jiaotong University School of Medicine, Shanghai, China
Objectives: Recurrent patellar dislocation (RPD) greatly affects active young individuals, necessitating the identification of risk factors for a better understanding of its cause. Previous research has connected RPD to lower limb alignment (LEA) abnormalities, such as increased femoral anteversion, tibial external rotation, knee valgus, and flexion. This study aims to use EOS technology to detect RPD-related LEA anomalies, enabling three-dimensional assessment under load conditions.
Methods: A total of 100 limbs (50 in the RPD group, 50 in the control group) were retrospectively analyzed. In the RPD group, we included limbs with recurrent patellar dislocation, characterized by dislocations occurs at least two times, while healthy limbs served as the control group. We used EOS technology, including 2D and 3D imaging, to measure and compare the following parameters between the two groups in a standing position: Femoral neck shaft angle (NSA), Mechanical femoral tibial angle (MFTA), Mechanical lateral distal femoral angle (mLDFA), Medial proximal tibial angle (MPTA), Anatomical femoral anteversion (AFA), External tibial torsion (ETT), and Femorotibial rotation (FTR).
Results: The significant differences between the two groups were shown in NSA 3/2D, MFTA 3/2D, mLDFA 3/2D, MPTA 3D, AFA, FTR. No significant difference was shown in MPTA 2D, ETT between the RPD group and the control group. Further binary logistic regression analysis. Further binary logistic regression analysis was conducted on the risk factors affecting RPD mentioned above. and found four risk factors for binary logistic regression analysis: mLDFA (3D), AFA, NSA(3D), and FTR.
Conclusions: EOS imaging identified abnormal LEA parameters, including NSA, MFTA, mLDFA, MPTA, AFA, and FTR, as risk factors for RPD. Children with these risk factors should receive moderate knee joint protection.
1. Introduction
Patellar dislocation (PD) is a common unstable knee disease that affects young individuals (1, 2). It occurs when the patella moves sideways out of trochlea groove of the femoral condyle. The annual incidence rate of pediatric PD has been reported to range from 2/100,000 to 107/100,000, accounting for 0.4% of pediatric emergency hospitalizations in surgical wards (3, 4). This greatly reduces patients’ physical activity and lead to long-term complications in adulthood, such as pain, chronic instability, cartilage deterioration and early-onset osteoarthritis (5, 6). Importantly, patients with prior PD have a 22.7–86.2% risk of experiencing a recurrent patellar dislocation (RPD) (7–9). RPD is an important cause of disability in young and active people. Therefore, correct identification of the risk factors for RPD is crucial for the study of the pathophysiology and etiology of RPD (10, 11).
When the knee joint moves back and forth between flexion and extension, the trajectory of the patella depends on the complex three-dimensional motion sequence between the femur, tibia, and patella. The anatomical basis of these movements is the interaction between the joint surface, bone structure, meniscus, ligaments, and muscles. While the initial onset of patellar dislocation is often attributed to accidental injury, the recurrence of this condition is multifactorial, with contributions from various mechanical factors. These factors include lower extremity alignment (LEA), dysfunction of the medial patellofemoral ligament or internal oblique muscle, excessive tension of the lateral retinaculum, systemic joint relaxation (12–18), as well as the alignment of the knee extension device, namely the Q angle (19, 20), and the shape and depth of the femoral sulcus. Among these factors, LEA is extremely important in the pathophysiology and etiology of RPD (21–24). During complete knee flexion, as the knee transitions from an extended state to a flexed state, the patella moves distally relative to the femur, ultimately aligning with the femoral groove. This movement is guided by the complex rotation of the tibia relative to the femur, which in turn is controlled by the consistency of the femur tibia joint surface and the forces of the muscles and ligaments, with significant individual differences. Previous studies have shown that LEA abnormalities, such as increased femoral anteversion angle, increased tibial external rotation, increased tibial external rotation, knee valgus, and knee reflection, are associated with RPD (21–30).
Several studies have shown that the kinematics of the patellofemoral joint observed in the non-weight-bearing posture are significantly different from the kinematics of the patellofemoral joint observed in the weight-bearing posture (31–33). Compared to non-weight-bearing measurements, weight-bearing conditions can alter the measurement of relative force lines due to changes in gravity, muscle strength, and knee joint geometry. Alfredsson et al. reported a radiographic system for examining and evaluating weight-bearing knee joints, including a model for measuring femoral and tibial rotation, patellar translation, and Q-angle (34–37). These previous studies on LEA for RPD were based on two-dimensional (2D) measurements, such as transverse images from plain films or computed tomography (CT) scans. Due to the influence of radiation source position and limb positioning on 2D measurements, there may be limitations in accurately evaluating these force factors. Therefore, the lower limb function in three-dimensional (3D) evaluation should lead to more clinically relevant conclusions.
We urgently needed a new option that can three-dimensionally evaluate lower extremity alignment under weight-bearing conditions. Fortunately, in 2019, our hospital obtained the EOS-3D imaging system (EOS Imaging, Paris, France), which has been applied in many centers around the world. The system can scan the coronal plane and sagittal plane at the same time, and provide the three-dimensional shape of both lower limbs in an upright state under load (38). The two advantages of this system are that it exposes patients to lower radiation doses than traditional radiography (39–41). In addition, traditional radiography uses point source geometry, which can lead to spatial distortion. The EOS system uses a collimator to generate parallel beams, thus minimizing the aforementioned spatial distortion (38).
The purpose of this study was to use EOS technology to identify LEA anomalies related to RPD, which allows for a three-dimensional evaluation of LEA under load conditions.
2. Materials and methods
2.1. Patient selection
2.1.1. Inclusion criteria
(1) Patients with recurrent patellar instability, having experienced at least two patellar dislocations, despite non-operative treatment. (2) History of two or more positive imaging signs, including patellar dislocation, fracture of the medial patellar medial facet or the lateral femoral condyle, or injuries to the vastus medialis obliquus and the medial patellofemoral ligament on radiographs or magnetic resonance imaging (15, 42). (3) Both lower limbs were analysed to determine whether both knees showed symptoms of RPD. RPD diagnosis is performed by Dr. Haiqing Cai, a paediatric knee disease specialist with 25 years of experience.
2.1.2. Exclusion criteria
(1) Patients with a single episode of patella dislocation. (2) Patients with a history of prior knee surgery. (3) Patients with a history of other knee injuries or dislocations due to direct trauma. (4) Patients with other types of patella dislocation, including habitual or fixed dislocation. After capturing EOS images, we conducted visual assessments to detect limb motion, excluding any images displaying S-shaped curves or bone cortex discontinuity as they were deemed unacceptable.
2.1.3. Control group
Healthy young volunteers without knee complaints or a history of knee injuries were chosen. We reviewed the outpatient medical history system, identified patients who had undergone systemic EOS, and selected individuals who did not exhibit unequal lower limb lengths, abnormal power lines, or discomfort-related complaints for the control group. We tried to select patients around 14 years old who could match the age range of RPD patients. Because these patients just underwent regular EOS during routine follow-up, we only needed to retrieve the data from the database, so there was no need to ask these patients to take EOS again.
2.2. EOS examination and analysis
2.2.1. The EOS imaging system
Anteroposterior and lateral images (tube voltage: 80 kV; tube current: 200 mA) were acquired simultaneously using the EOS imaging system with the patient in an upright posture to bear the physiological load. The patients applied the load evenly to both lower extremities, with the patella facing forward, and then rotated 15° in both directions to avoid superimposition of the anatomical references on the lateral radiographs for a total of two EOS images. Each 3D model was reconstructed from these biplanar x-ray images using a sterEOS workstation (EOS Imaging Inc., Paris, France). The 3D models of the lower extremities were obtained using semi-automatic adjustments of the anatomical reference points over the bone contours followed by fine manual manipulation (Figure 1) (43–46).
2.2.2. The following variables were measured and analyzed
(1) NSA: Femoral neck shaft angle (NSA)
(2) Mechanical femoral tibial angle (MFTA)
The frontal femoral plane represents the angle between the femoral mechanical axis and the tibial mechanical axis, with a positive angle for valgus and a negative angle for varus alignments.
(3) Mechanical lateral distal femoral angle (mLDFA)
The frontal femoral planet represents the lateral angle between the femoral mechanical axis and the axis across the most distal point of the medial and lateral condyles and is always expressed as a positive angle.
(4) Medial proximal tibial angle (MPTA)
The frontal femoral plane is the medial angle between the tibial mechanical axis and the line connecting the centers of the medial and lateral tibia plateaus.
(5) Anatomical femoral anteversion (AFA)
This represents the angle between the femoral neck axis and the bicondylar axis (the axis between the centers of the spheres fitted to the medial and lateral femoral condyles). It is measured by projecting it onto a plane perpendicular to the mechanical axis of the femur. A negative value indicates femoral retroversion.
(6) External tibial torsion (ETT)
This represents the angle between the line tangential to the posterior portion of the tibial plateau and the bimalleolar axis. It is measured by projecting it onto a plane orthogonal to the mechanical axis of the tibia with a positive angle for external rotation (when the malleoli are turned externally in relation to the tibial plateau) and a negative angle for internal rotation (when the malleoli are turned internally in relation to the tibial plateau).
(7) Femorotibial rotation (FTR)
It is the angle between the posterior bicondylar axis and the axis in contact with the posterior part of the tibial plateau with a positive angle for external rotation of the tibial plateau relative to the femoral condyles and a negative angle when it is internally rotated.
2.2.3. The reference axes were defined as follows
(1) Femoral mechanical axis: the line connecting the center of the femoral head and the center of the trochlea.
(2) Femoral anatomical axis: the line drawn down the center of the femur's diaphysis.
(3) Tibial mechanical axis (correspond with tibial anatomical axis): the line connecting the center of the tibial spines and the center of the distal articular surface of the tibia.
2.3. Statistical analysis
The Student's t-test without correspondence was used to compare the mean values between the two groups. For these parameters, which had significant and clinically important differences between both groups according to the Student's t-test, the relationships between each parameter of the LEA and RPD were assessed using binary logistic regression. All analyses were performed using SPSS version 24.0 (IBM Corp., Armonk, NY), and statistical significance was set at an alpha level of 5%.
3. Results
3.1. Cohort characteristics
We gathered data from a cohort of 31 patients with recurrent patellar dislocation (RPD), comprising 19 with bilateral involvement and 12 with unilateral symptoms. This allowed us to collect a total of 50 affected limbs and 12 healthy limbs from the RPD group. Additionally, we included 19 patients in the control group, totaling 38 healthy lower limbs. In summary, our study involved a collection of 50 affected limbs and 50 healthy limbs for analysis. Among the limbs affected by RPD, 10 belonged to male patients and 40 belonged to female patients, with an average age of 13.6 ± 1.73793 years. There were 22 on the right side and 28 on the left side. Among the healthy limbs, 20 belonged to males and 30 belonged to females, with an average age of 12.8 ± 2.28571 years. There were 28 on the right and 22 on the left (Table 1).
3.2. Lower extremity alignment (LEA)
The mean values and standard deviations of the alignment parameters are described in Table 1. Significant and clinically important differences between the two groups were shown in NSA 3/2D, MFTA 3/2D, mLDFA 3/2D, MPTA 3D, AFA, and FTR. There was no significant difference in MPTA 2D and ETT between the RPD group and the control group, respectively (Table 1).
3.3. Logistic regression analysis
Further binary logistic regression analysis was conducted on the risk factors affecting RPD mentioned above. Because there may be a linear relationship between MFTA, mLDFA, or MPTA, it was found that the P value of the Hosmer and Lemeshow test of mLDFA was higher (0.892) when one of the three was selected and included in the regression analysis model. When MFTA was selected, the P value was (0.866). When MPTA was selected, the P value was only (0.356). Therefore, we selected four risk factors for binary logistic regression analysis: 3D mLDFA, AFA, 3D NSA, and FTR (Table 2).
4. Discussion
Recurrent knee pain conditions in children and adolescents, such as patellofemoral pain syndrome (PFPS) (47, 48) and RPD, significantly restrict young individuals, especially young athletes, from returning to regular sports activities. Utilizing imaging techniques to identify patients at a higher mechanical risk for these conditions is crucial. EOS technology offers a low-radiation method for measuring the three-dimensional lower limb force lines while patients bear weight. This approach provides unique advantages over traditional x-rays, CT scans, or MRI.
4.1. Coronal plane malformation
Our study demonstrated that coronal plane deformities of the lower limbs in RPD patients, including smaller NSA, smaller mLDFA, and larger MPTA, lead to the exacerbation of genu valgus, potentially affecting the Q angle-a potential risk factor for habitual patellar dislocation.
4.2. Horizontal rotation deformities
Previous studies have shown that the measurement results of femoral and tibial torsion obtained through EOS imaging are comparable to those obtained from 2D CT scans (49). It has good consistency in the results of measuring femur, tibia, and femoral tibial torsion based on 3D CT reconstruction technology. In Yan's study, there was no trend of increasing (or decreasing) variability between the two methods, as the mean values of CT and EOS measurements increased. This indicates good consistency between the two methods in measuring femur, tibia, and femur tibia torsion (50).
4.2.1. Anatomical femoral anteversion (AFA)
As we know, the strategies for treating RPD vary from case to case (51–53). Recently, excessive femoral anatomical anteversion angle (AFA) has been found in RPD patients (54–56), and recurrence of patellar dislocation has been found by causing distal femoral internal rotation and incorrect coupling between the patella and the femoral trochlear (54, 57, 58). To address this risk factor, some studies have suggested performing distal femoral circumcision (DDFO) for these patients (59–63). However, there is significant controversy regarding the AFA threshold for DDFO. Nelitz et al. (63) proposed a threshold of AFA >25°, while Weber et al. (64) proposed a lower threshold, suggesting AFA >20°. In addition, it is recommended to combine other factors (such as the “J” sign) with AFA when determining DDFO (65, 66).
However, measuring AFA often requires a CT examination of the lower limbs and a large amount of radiation is often avoided by doctors and patients, which cannot be widely used in clinical practice. At the same time, conducting lower limb CT scans on normal individuals is unethical, which may result in insufficient research data for the relevant control population (63–65). However, EOS technology provides a new method for measuring AFA under the premise of a small amount of radiation. The application of this method can obtain AFA data in the physical examinations of healthy individuals, thus providing assistance for more in-depth research.
This study applied the latest EOS technology to obtain the AFA when the patient was standing. A similar trend was observed in the femur as in previous studies (with an increase in AFA in RPD compared to normal) (Figure 2 and Table 3) (14, 21, 24, 29, 70). Through statistical analysis, it was found that an abnormal increase in AFA may be one of the risk factors for RPD.
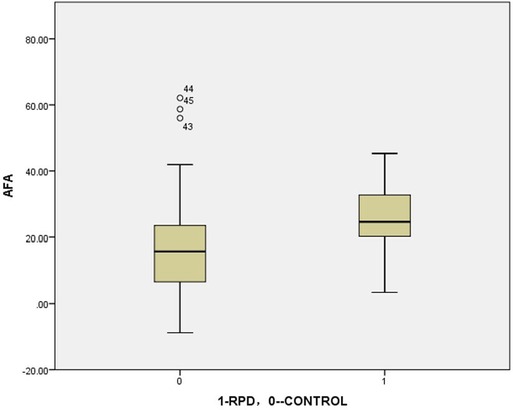
Figure 2. The boxplot of anatomical femoral anteversion: RVD VS CONTROL (analyzed by independent-samples t-test)= 23.0°VS 17.5°, P = 0.002.
Because all RPD patients in this study had not undergone DDFO surgery, it is not possible to further determine the threshold of DDFO, which requires further research in the future.
4.2.2. External tibial torsion (ETT)
In this study, we did not find any abnormal rotation of the tibia in RPD patients relative to the control group (Figure 3), which is consistent with previous studies (Table 3) (21, 22, 24, 29). However, this is different from the research by Takai and Takagi, whose data showed a decrease in tibial torsion in patients with RPD (29, 70).
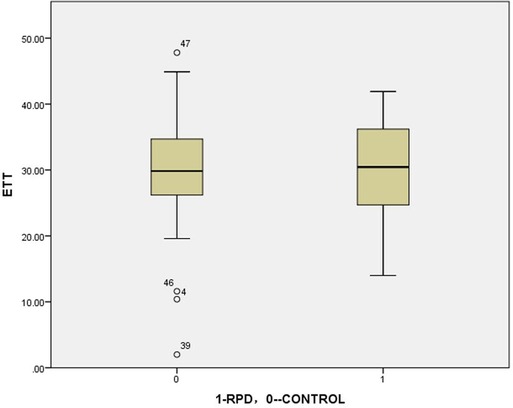
Figure 3. The boxplot of external tibial torsion: RPD VS CONTROL (analyzed by independent-samples t-test)= 30.3°VS 29.8°, P = 0.704.
There is currently little literature on using EOS to measure tibial torsion deformity in patients with RPD. Therefore, due to the lack of relevant comparative data, we can only temporarily understand it as an error caused by different measurement methods. However, from the perspective of RPD pathology, so-called miserable malalignment syndrome may exist, including excessive femoral anteversion, with, or without increased external tibial torsion (71). The position of the tibial tuberosity relative to the femoral trochlea further complicates the process of patella entry into the trochlea (14). The increase in the external rotation of the tibia seems to be more likely to cause external displacement of the tibial tubercle, thereby affecting the trajectory of the patella. This also supports the fact that patients with RPD are more likely to have an increase in tibial external rotation torsion rather than a decrease in tibial external rotation torsion.
4.2.3. Femorotibial rotation (FTR)
This study showed that, under weight-bearing conditions, in the RPD group, the tibia rotated more outward at the knee joint relative to the femur compared to the control group (Figure 4). This is generally consistent with previous research results (Table 3) (14, 21, 22, 24, 29, 70).
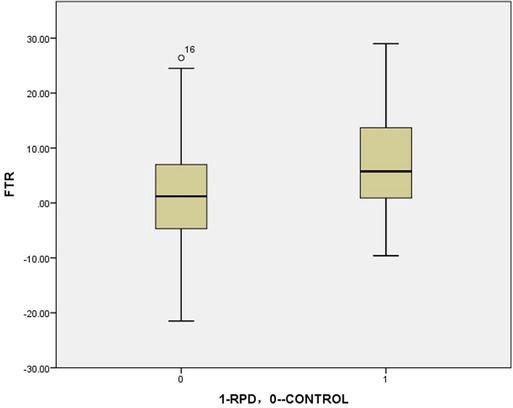
Figure 4. The boxplot of femorotibial rotation: RPD VS CONTROL (analyzed by independent-samples t-test) = 7.3°VS 2.0°, P = 0.004.
As mentioned above, the position of the tibial tuberosity relative to the femoral trochlea further complicates the process of patella entry into the trochlea (14). Therefore, the lateral rotation of the tibia relative to the femur during the weight-bearing position also affects the position of the tibial tubercle and may result in misclassified malalignment syndrome. Therefore, an excessive increase in FTR may also be one of the risk factors for RPD.
4.3. Limitations
This study has several limitations. Firstly, we did not investigate other potential risk factors for RPD, such as foot anomalies. Future research should consider examining a combination of these risk factors. Secondly, due to the higher susceptibility of female patients to RPD, it is essential to increase the number of female patients in control groups for upcoming studies. Additionally, using unaffected limbs from some RPD patients as controls in the control group might potentially impact the final data analysis. Therefore, future research should aim to expand the collection of control group data to reduce this potential source of bias.
5. Conclusion
EOS is suitable for LEA screenings in adolescents and children. The 3D-EOS imaging system identified abnormal LEA parameters, including NSA, MFTA, mLDFA, MPTA, AFA, and FTR, as risk factors for RPD. Providing moderate knee joint protection for children with these identified risk factors is advisable to reduce the likelihood of RPD.
Data availability statement
The original contributions presented in the study are included in the article/Supplementary Material, further inquiries can be directed to the corresponding authors.
Author contributions
MM: Software, Writing—original draft, Data curation, Investigation. HaiC: Conceptualization, Methodology, Resources, Writing—original draft, Writing—review & editing. LZ: Writing—review & editing, Validation. HaoC: Validation, Writing—review & editing, Conceptualization, Formal Analysis, Methodology, Resources, Software, Supervision, Writing—original draft.
Funding
The author(s) declare financial support was received for the research, authorship, and/or publication of this article. This research is founded by Shanghai Committee of Science and Technology (Grant No. 20Y11913200). This research received no specific grant from any funding agency in the commercial sector.
Conflict of interest
The authors declare that the research was conducted in the absence of any commercial or financial relationships that could be construed as a potential conflict of interest.
Publisher's note
All claims expressed in this article are solely those of the authors and do not necessarily represent those of their affiliated organizations, or those of the publisher, the editors and the reviewers. Any product that may be evaluated in this article, or claim that may be made by its manufacturer, is not guaranteed or endorsed by the publisher.
References
1. Chotel F, Berard J, Raux S. Patellar instability in children and adolescents. Orthop Traumatol Surg Res. (2014) 100(1 Suppl):S125–37. doi: 10.1016/j.otsr.2013.06.014
2. Clark D, Metcalfe A, Wogan C, Mandalia V, Eldridge J. Adolescent patellar instability: current concepts review. Bone Joint J. (2017) 99-B(2):159–70. doi: 10.1302/0301-620X.99B2.BJJ-2016-0256.R1
3. Nietosvaara Y, Aalto K, Kallio PE. Acute patellar dislocation in children: incidence and associated osteochondral fractures. J Pediatr Orthop. (1994) 14(4):513–5. doi: 10.1097/01241398-199407000-00018
4. Miao M, Cai H, Wang Z, Hu L, Bian J, Cai H. Suprapatellar pouch effusion is associated with an increased risk of neglected osteochondral fractures in primary acute traumatic patellar dislocation: a consecutive series of 113 children. J Orthop Surg Res. (2023) 18(1):627. doi: 10.1186/s13018-023-04130-8
5. Fithian DC, Paxton EW, Stone ML, Silva P, Davis DK, Elias DA, et al. Epidemiology and natural history of acute patellar dislocation. Am J Sports Med. (2004) 32(5):1114–21. doi: 10.1177/0363546503260788
6. Hawkins RJ, Bell RH, Anisette G. Acute patellar dislocations. The natural history. Am J Sports Med. (1986) 14(2):117–20. doi: 10.1177/036354658601400204
7. Lewallen LW, McIntosh AL, Dahm DL. Predictors of recurrent instability after acute patellofemoral dislocation in pediatric and adolescent patients. Am J Sports Med. (2013) 41(3):575–81. doi: 10.1177/0363546512472873
8. Lewallen L, McIntosh A, Dahm D. First-time patellofemoral dislocation: risk factors for recurrent instability. J Knee Surg. (2015) 28(4):303–9. doi: 10.1055/s-0034-1398373
9. Jaquith BP, Parikh SN. Predictors of recurrent patellar instability in children and adolescents after first-time dislocation. J Pediatr Orthop. (2017) 37(7):484–90. doi: 10.1097/BPO.0000000000000674
10. Boden BP, Pearsall AW, Garrett WE, Feagin JA. Patellofemoral instability: evaluation and management. J Am Acad Orthop Surg. (1997) 5(1):47–57. doi: 10.5435/00124635-199701000-00006
11. Danielsen O, Poulsen TA, Eysturoy NH, Mortensen ES, Hölmich P, Barfod KW. Trochlea dysplasia, increased TT-TG distance and patella alta are risk factors for developing first-time and recurrent patella dislocation: a systematic review. Knee Surg Sports Traumatol Arthrosc. (2023) 31(9):3806–46. doi: 10.1007/s00167-022-07255-1
12. Seitlinger G, Ladenhauf HN, Wierer G. What is the chance that a patella dislocation will happen a second time: update on the natural history of a first time patella dislocation in the adolescent. Curr Opin Pediatr. (2018) 30(1):65–70. doi: 10.1097/MOP.0000000000000568
13. Colvin AC, West RV. Patellar instability. J Bone Joint Surg Am. (2008) 90(12):2751–62. doi: 10.2106/JBJS.H.00211
14. Dejour H, Walch G, Nove-Josserand L, Guier C. Factors of patellar instability: an anatomic radiographic study. Knee Surg Sports Traumatol Arthrosc. (1994) 2(1):19–26. doi: 10.1007/BF01552649
15. Diederichs G, Issever AS, Scheffler S. MR Imaging of patellar instability: injury patterns and assessment of risk factors. Radiographics. (2010) 30(4):961–81. doi: 10.1148/rg.304095755
16. Redziniak DE, Diduch DR, Mihalko WM, Fulkerson JP, Novicoff WM, Sheibani-Rad S. Patellar instability. J Bone Joint Surg Am. (2009) 91(9):2264–75.19724006
17. Schiphouwer L, Rood A, Tigchelaar S, Koëter S. Complications of medial patellofemoral ligament reconstruction using two transverse patellar tunnels. Knee Surg Sports Traumatol Arthrosc. (2017) 25(1):245–50. doi: 10.1007/s00167-016-4245-4
18. Yamada Y, Toritsuka Y, Nakamura N, Horibe S, Sugamoto K, Yoshikawa H, et al. Correlation of 3D shift and 3D tilt of the patella in patients with recurrent dislocation of the patella and healthy volunteers: an in vivo analysis based on 3-dimensional computer models. Am J Sports Med. (2017) 45(13):3111–8. doi: 10.1177/0363546517720193
19. Brattstroem H. Shape of the intercondylar groove normally and in recurrent dislocation of patella. A clinical and x-ray-anatomical investigation. Acta Orthop Scand Suppl. (1964) 68(SUPPL 68):1–148. doi: 10.3109/ort.1964.35.suppl-68.01
20. Paulos L, Rusche K, Johnson C, Noyes FR. Patellar malalignment: a treatment rationale. Phys Ther. (1980) 60(12):1624–32. doi: 10.1093/ptj/60.12.1624
21. Diederichs G, Köhlitz T, Kornaropoulos E, Heller MO, Vollnberg B, Scheffler S. Magnetic resonance imaging analysis of rotational alignment in patients with patellar dislocations. Am J Sports Med. (2013) 41(1):51–7. doi: 10.1177/0363546512464691
22. Erkocak OF, Altan E, Altintas M, Turkmen F, Aydin BK, Bayar A. Lower extremity rotational deformities and patellofemoral alignment parameters in patients with anterior knee pain. Knee Surg Sports Traumatol Arthrosc. (2016) 24(9):3011–20. doi: 10.1007/s00167-015-3611-y
23. Parikh S, Noyes FR. Patellofemoral disorders: role of computed tomography and magnetic resonance imaging in defining abnormal rotational lower limb alignment. Sports Health. (2011) 3(2):158–69. doi: 10.1177/1941738111399372
24. Prakash J, Seon J-K, Woo S-H, Jin C, Song E-K. Comparison of radiological parameters between normal and patellar dislocation groups in Korean population: a rotational profile CT-based study. Knee Surg Relat Res. (2016) 28(4):302–11. doi: 10.5792/ksrr.16.010
25. Beaconsfield T, Pintore E, Maffulli N, Petri GJ. Radiological measurements in patellofemoral disorders. A review. Clin Orthop Relat Res. (1994) 308:18–28. doi: 10.1097/00003086-199411000-00004
26. Gillespie D, Mandziak D, Howie C. Influence of posterior lateral femoral condyle geometry on patellar dislocation. Arch Orthop Trauma Surg. (2015) 135(11):1503–9. doi: 10.1007/s00402-015-2310-y
27. Hermans K, Claes S, Bellemans J. Valgus instability as a cause for recurrent lateral patellar dislocation: a new mechanism for patellofemoral instability? Acta Orthop Belg. (2013) 79(5):495–501.24350508
28. Kearney SP, Mosca VS. Selective hemiepiphyseodesis for patellar instability with associated genu valgum. J Orthop. (2015) 12(1):17–22. doi: 10.1016/j.jor.2015.01.005
29. Takai S, Sakakida K, Yamashita F, Suzu F, Izuta F. Rotational alignment of the lower limb in osteoarthritis of the knee. Int Orthop. (1985) 9(3):209–15. doi: 10.1007/BF00268173
30. Tamari K, Tinley P, Briffa K, Breidahl W. Validity and reliability of existing and modified clinical methods of measuring femoral and tibiofibular torsion in healthy subjects: use of different reference axes may improve reliability. Clin Anat. (2005) 18(1):46–55. doi: 10.1002/ca.20050
31. Powers CM, Ward SR, Fredericson M, Guillet M, Shellock FG. Patellofemoral kinematics during weight-bearing and non-weight-bearing knee extension in persons with lateral subluxation of the patella: a preliminary study. J Orthop Sports Phys Ther. (2003) 33(11):677–85. doi: 10.2519/jospt.2003.33.11.677
32. Uemura K, Takao M, Hamada H, Sakai T, Sugano N. Change in axial rotation of the femur in the resting supine position following total hip arthroplasty. Artif Organs. (2018) 42(3):290–6. doi: 10.1111/aor.12998
33. Uemura K, Takao M, Otake Y, Koyama K, Yokota F, Hamada H, et al. Can anatomic measurements of stem anteversion angle be considered as the functional anteversion angle? J Arthroplasty. (2018) 33(2):595–600. doi: 10.1016/j.arth.2017.09.027
34. Sanfridsson J, Ryd L, Eklund K, Kouvaras Y, Jonsson K. Angular configuration of the knee. Comparison of conventional measurements and the QUESTOR precision radiography system. Acta Radiol. (1996) 37(5):633–8. doi: 10.3109/02841859609177689
35. Sanfridsson J, Ryd L, Svahn G, Fridén T, Jonsson K. Radiographic measurement of femorotibial rotation in weight-bearing. The influence of flexion and extension in the knee on the extensor mechanism and angles of the lower extremity in a healthy population. Acta Radiol. (2001) 42(2):207–17. doi: 10.1080/028418501127346512
36. Sanfridsson J, Svahn G, Jonsson K, Ryd L. Computed radiography for characterisation of the weight-bearing knee. Acta Radiol. (1997):38(4 Pt 1):514–9. doi: 10.1080/02841859709174378
37. Sanfridsson J, Svahn G, Ryd L, Ahl TL, Sundén P, Jonsson K. Assessment of image post-processing and of measuring assistance tools in computed radiography. Evaluation of the weight-bearing knee. Acta Radiol. (1998) 39(6):642–8. doi: 10.3109/02841859809175490
38. Melhem E, Assi A, El Rachkidi R, Ghanem I. EOS((R)) biplanar x-ray imaging: concept, developments, benefits, and limitations. J Child Orthop. (2016) 10(1):1–14. doi: 10.1007/s11832-016-0713-0
39. McKenna C, Wade R, Faria R, Yang H, Stirk L, Gummerson N, et al. EOS 2D/3D x-ray imaging system: a systematic review and economic evaluation. Health Technol Assess. (2012) 16(14):1–188. doi: 10.3310/hta16140
40. Goodbody C, Kedem P, Thompson M, Do HT, Mintz DN, Widmann RF, et al. Reliability and reproducibility of subject positioning with EOS low-dose biplanar x-ray. HSS J. (2017) 13(3):263–6. doi: 10.1007/s11420-017-9548-6
41. Huang J, Tian F, Zhang Z, Shi W, Lin J, Chen L, et al. Reliability and concurrent validity of angle measurements in lower limb: eOS 3D goniometer versus 2D manual goniometer. J Orthop Translat. (2020) 24:96–102. doi: 10.1016/j.jot.2020.05.002
42. Elias DA, White LM. Imaging of patellofemoral disorders. Clin Radiol. (2004) 59(7):543–57. doi: 10.1016/j.crad.2004.01.004
43. Gaumétou E, Quijano S, Ilharreborde B, Presedo A, Thoreux P, Mazda K, et al. EOS Analysis of lower extremity segmental torsion in children and young adults. Orthop Traumatol Surg Res. (2014) 100(1):147–51. doi: 10.1016/j.otsr.2013.09.010
44. Illes T, Somoskeoy S. The EOS imaging system and its uses in daily orthopaedic practice. Int Orthop. (2012) 36(7):1325–31. doi: 10.1007/s00264-012-1512-y
45. Chen C, Min L, Sun M, Weng W, Wang W. Abnormal femur rotation in patients with recurrent patellar dislocation: a study on upright standing three dimensionally reconstructed EOS images. Knee. (2021) 32::131–9. doi: 10.1016/j.knee.2021.08.011
46. Cho BW, Lee TH, Kim S, Choi CH, Jung M, Lee KY, et al. Evaluation of the reliability of lower extremity alignment measurements using EOS imaging system while standing in an even weight-bearing posture. Sci Rep. (2021) 11(1):22039. doi: 10.1038/s41598-021-01646-z
47. Caton JH, Dejour D. Tibial tubercle osteotomy in patello-femoral instability and in patellar height abnormality. Int Orthop. (2010) 34(2):305–9. doi: 10.1007/s00264-009-0929-4
48. Dejour D, Byn P, Ntagiopoulos PG. The Lyon’s sulcus-deepening trochleoplasty in previous unsuccessful patellofemoral surgery. Int Orthop. (2013) 37(3):433–9. doi: 10.1007/s00264-012-1746-8
49. Buck FM, Guggenberger R, Koch PP, Pfirrmann CW. Femoral and tibial torsion measurements with 3D models based on low-dose biplanar radiographs in comparison with standard CT measurements. AJR. American Journal of Roentgenology. (2012) 199(5):W607–12. doi: 10.2214/AJR.11.8295
50. Yan W, Xu X, Xu Q, Yan W, Sun Z, Jiang Q, et al.. Femoral and tibial torsion measurements based on EOS imaging compared to 3D CT reconstruction measurements. Ann Transl Med. (2019) 7(18):460. doi: 10.21037/atm.2019.08.49
51. Laidlaw MS, Feeley SM, Ruland JR, Diduch DR. Sulcus-deepening trochleoplasty and medial patellofemoral ligament reconstruction for recurrent patellar instability. Arthrosc Tech. (2018) 7(2):e113–23. doi: 10.1016/j.eats.2017.08.061
52. Pavone V, Vescio A, Panvini FMC, Lucenti L, Caldaci A, Sapienza M, et al. Patellofemoral pain syndrome in young female athletes: a case-control study. Adv Orthop. (2022) 2022:1907975. doi: 10.1155/2022/1907975
53. Alonazi A, Hasan S, Anwer S, Jamal A, Parvez S, Alfaiz FAS, et al. Efficacy of electromyographic-biofeedback supplementation training with patellar taping on quadriceps strengthening in patellofemoral pain syndrome among young adult male athletes. Int J Environ Res Public Health. (2021) 18(9):4514. doi: 10.3390/ijerph18094514
54. Franciozi CE, Ambra LF, Albertoni LJ, Debieux P, Rezende FC, Oliveira MA, et al. Increased femoral anteversion influence over surgically treated recurrent patellar instability patients. Arthroscopy. (2017) 33(3):633–40. doi: 10.1016/j.arthro.2016.09.015
55. Kim TH, Sobti A, Lee SH, Lee JS, Oh KJ. The effects of weight-bearing conditions on patellofemoral indices in individuals without and with patellofemoral pain syndrome. Skeletal Radiol. (2014) 43(2):157–64. doi: 10.1007/s00256-013-1756-7
56. Laprade J, Culham E. Radiographic measures in subjects who are asymptomatic and subjects with patellofemoral pain syndrome. Clin Orthop Relat Res. (2003) 414:172–82. doi: 10.1097/01.blo.0000079269.91782.f5
57. Fitzpatrick CK, Steensen RN, Tumuluri A, Trinh T, Bentley J, Rullkoetter PJ. Computational analysis of factors contributing to patellar dislocation. J Orthop Res. (2016) 34(3):444–53. doi: 10.1002/jor.23041
58. Zhang Z, Zhang H, Song G, Zheng T, Ni Q, Feng H. Increased femoral anteversion is associated with inferior clinical outcomes after MPFL reconstruction and combined tibial tubercle osteotomy for the treatment of recurrent patellar instability. Knee Surg Sports Traumatol Arthrosc. (2020) 28(7):2261–9. doi: 10.1007/s00167-019-05818-3
59. Frings J, Krause M, Akoto R, Wohlmuth P, Frosch K-H. Combined distal femoral osteotomy (DFO) in genu valgum leads to reliable patellar stabilization and an improvement in knee function. Knee Surg Sports Traumatol Arthrosc. (2018) 26(12):3572–81. doi: 10.1007/s00167-018-5000-9
60. Imhoff FB, Beitzel K, Zakko P, Obopilwe E, Voss A, Scheiderer B, et al. Derotational osteotomy of the distal femur for the treatment of patellofemoral instability simultaneously leads to the correction of frontal alignment: a laboratory cadaveric study. Orthop J Sports Med. (2018) 6(6). doi: 10.1177/2325967118775664
61. Imhoff FB, Cotic M, Liska F, Dyrna FGE, Beitzel K, Imhoff AB, et al. Derotational osteotomy at the distal femur is effective to treat patients with patellar instability. Knee Surg Sports Traumatol Arthrosc. (2019) 27(2):652–8. doi: 10.1007/s00167-018-5212-z
62. Nelitz M. Femoral derotational osteotomies. Curr Rev Musculoskelet Med. (2018) 11(2):272–9. doi: 10.1007/s12178-018-9483-2
63. Nelitz M, Dreyhaupt J, Williams SRM, Dornacher D. Combined supracondylar femoral derotation osteotomy and patellofemoral ligament reconstruction for recurrent patellar dislocation and severe femoral anteversion syndrome: surgical technique and clinical outcome. Int Orthop. (2015) 39(12):2355–62. doi: 10.1007/s00264-015-2859-7
64. Weber AE, Nathani A, Dines JS, Allen AA, Shubin-Stein BE, Arendt EA, et al. An algorithmic approach to the management of recurrent lateral patellar dislocation. J Bone Joint Surg Am. (2016) 98(5):417–27. doi: 10.2106/JBJS.O.00354
65. Zhang Z, Zhang H, Song G, Zheng T, Feng H. A pre-operative grade 3 J-sign adversely affects short-term clinical outcome and is more likely to yield MPFL residual graft laxity in recurrent patellar dislocation. Knee Surg Sports Traumatol Arthrosc. (2020) 28(7):2147–56. doi: 10.1007/s00167-019-05736-4
66. Zhang Z, Zhang H, Song G, Wang X, Zhang J, Zheng T, et al. A high-grade J sign is more likely to yield higher postoperative patellar laxity and residual maltracking in patients with recurrent patellar dislocation treated with derotational distal femoral osteotomy. Am J Sports Med. (2020) 48(1):117–27. doi: 10.1177/0363546519884669
67. Fabry G, MacEwen GD, Shands AR Jr. Torsion of the femur. A follow-up study in normal and abnormal conditions. J Bone Joint Surg Am. (1973) 55(8):1726–38. doi: 10.2106/00004623-197355080-00017
68. Schröter S, Nakayama H, Ihle C, Ateschrang A, Maiotti M, Harrer J, et al. Torsional osteotomy. J Knee Surg. (2020) 33(5):486–95. doi: 10.1055/s-0039-1678677
69. Strecker W, Dickschas J. [Torsional osteotomy: operative treatment of patellofemoral maltracking]. Oper Orthop Traumatol. (2015) 27(6):505–24. doi: 10.1007/s00064-015-0430-8
70. Takagi S, Sato T, Watanabe S, Tanifuji O, Mochizuki T, Omori G, et al. Alignment in the transverse plane, but not sagittal or coronal plane, affects the risk of recurrent patella dislocation. Knee Surg Sports Traumatol Arthrosc. (2018) 26(10):2891–8. doi: 10.1007/s00167-017-4806-1
Keywords: EOS imaging system, 3D analysis, recurrent patellar dislocation, lower extremity alignment, standing position
Citation: Miao M, Cai H, Zhang L and Cai H (2023) Analysis of lower extremity alignment (LEA) in children with recurrent patellar dislocation by EOS system. Front. Pediatr. 11:1291739. doi: 10.3389/fped.2023.1291739
Received: 10 September 2023; Accepted: 5 October 2023;
Published: 25 October 2023.
Edited by:
Xin Tang, Huazhong University of Science and Technology, ChinaReviewed by:
Marco Sapienza, University of Catania, ItalyAnirejuoritse Bafor, Nationwide Children’s Hospital, United States
© 2023 Miao, Cai, Zhang and Cai. This is an open-access article distributed under the terms of the Creative Commons Attribution License (CC BY). The use, distribution or reproduction in other forums is permitted, provided the original author(s) and the copyright owner(s) are credited and that the original publication in this journal is cited, in accordance with accepted academic practice. No use, distribution or reproduction is permitted which does not comply with these terms.
*Correspondence: Haoqi Cai hiden1983@hotmail.com
†These authors share first authorship