- 1Gustavus Adolphus College, Saint Peter, MN, United States
- 2Department of Mathematics, Medgar Evers College, CUNY, Brooklyn, NY, United States
- 3Department of Neurosciences, University of New Mexico, Albuquerque, NM, United States
- 4Department of Pediatrics, University of New Mexico, Albuquerque, NM, United States
- 5Department of Pediatrics, University of Vermont, Burlington, VT, United States
- 6Department of Biology, Morgan State University, Baltimore, MD, United States
Cannabinoid use in all populations is increasing as legalization across the United States continues. Concerningly, there is a lack of caution provided by medical providers to pregnant individuals as to the impact the use of cannabinoids could have on the developing fetus. Research continues in both the preclinical and clinical areas, and is severely needed, as the potency of delta-9-tetrahydrocannabinol (THC), the primary psychoactive component of cannabis, has increased dramatically since the initial studies were completed. Thus far, clinical studies raise compelling evidence for short term memory deficits, impulse control issues, and attention deficiencies following prenatal cannabinoid exposure (PCE). These changes may be mediated through epigenetic modifications that not only impact the current offspring but could carry forward to future generations. While additional studies are needed, a pregnancy pause from cannabinoid products should be strongly recommended by providers to ensure the optimal health and well-being of our future generations.
Introduction
Cannabinoids and the extended endocannabinoid system (Endocannabinoidome)
The chemical nature of the Cannabis sativa plant (i.e., cannabis or marijuana) is complicated due to the presence of over 500 unique chemical compounds, more than 100 of which are a class of lipophilic chemicals known as phytocannabinoids or cannabinoids (CB) (1–3). Natural CB includes delta-9-tetrahydrocannabinol (THC), the primary psychoactive phytoCB (4), and cannabidiol (5) (CBD), the main non-psychoactive phytoCB in marijuana. Cannabinoids act on the G protein-coupled CB receptors, CB1R (6) and CB2R (7). Additionally, the endocannabinoid system (eCB) consists of the endogenous cannabinoids: N-arachidonoylethanolamine (anandamide; AEA) and 2-arachidonoylglycerol (2-AG), and their biosynthetic enzymes, N-acyl-phosphatidylethanolamine-hydrolyzing phospholipase D (NAPE-PLD) and sn-1-diacylglycerol lipases (DAGL), and their degradative enzymes, fatty acid amide hydrolase 1 (FAAH) and monoacylglycerol lipase (MGL) (8, 9). From 1970 to 2019, for both herbal cannabis and cannabis resin, the mean concentrations of THC increased. For this same period, there was no such increase in CBD concentration in herbal cannabis or cannabis resin (10). Between 2018 and 2019, the THC:CBD mean ratios (∼54:1 and ∼25:1, respectively) decreased by about 54%, indicating a preference for recreational cannabis use products containing a high-THC to low-CBD ratio (3). The near-ubiquitous neuromodulatory nature of the eCB is attributable to its homeostatic role in the modulation of neurodevelopment, neuroplasticity, several physiological and cognitive processes, and its responses to endogenous and exogenous perturbations (11–13). The homeostatic function of the eCB is to maintain the internal milieu (e.g., immunoregulation, mood regulation, and thermoregulation) and bioenergetic balance (i.e., energy flow through biological systems).
Moreover, the eCB effects nervous system development and function, fertility, pregnancy, and perinatal development. In addition, several components of the eCB have been identified in the embryo, follicular fluid, ovaries, the placenta, and the uterus (14, 15). The various constituents of the eCB system have both pro-homeostatic roles in the maintenance of health and well-being, and anti-homeostatic roles in the genesis of several diseases such as neurodegenerative diseases, cancer, and cardiovascular disease. Therefore, it is feasible that the eCB is a prime target in developing novel cannabimimetic therapeutic agents capable of modulating this system via inhibition or disinhibition of metabolic pathways or CB receptor agonism or antagonism (16). THC is now the most used psychoactive substance in the United States, with utilization continuing to rise as Americans support the legalization of recreational use nationwide (17). Concurrently, most adults perceive that THC is harmless during pregnancy (18, 19). Thus, it is imperative that additional research is conducted to determine the risks of THC use during pregnancy.
Prevalence of cannabinoid use
Has the prevalence of cannabinoid use increased with the legalization of cannabinoids for medicinal purposes?
Cannabinoids are the most widely used, federally illegal, recreational drug in the United States. The increased prevalence of cannabis use is partly due to its ever-increasing potency, availability, social acceptance, perceived safety, and access due to extensive legalization of cannabinoids for medical and recreational use in many states and worldwide (20, 21). Coinciding with the increased prevalence of cannabis containing higher concentrations of THC, the pattern of cannabinoid use is evolving towards almost daily use as compared to previous use patterns (22). While 55 million Americans report use of cannabis in the last year, which now surpasses the number of tobacco smokers, 1 in 16 high school seniors report daily use of cannabis products (23).
There exist several routes of exposure for cannabis including smoking the flowers and leaves, vaping concentrates, spraying a vaporized solution on the buccal mucosa, ingesting capsules, liquids, or foods, and applying topical lotions (24). The prevalence of cannabis vaping among adolescents has increased significantly, suggesting that the preference for cannabis products may be changing from smoking the dried herb to vaping cannabis oil (25, 26). Monitoring the Future is an ongoing survey funded by the National Institutes of Health that has measured drug and alcohol use since 1975. From 2017 to 2018, young adults were observed to double their past 30-day cannabis vaping use, which was among the largest 1-year increase in any substance use recorded in Monitoring the Future (27). Unfortunately, the following year showed a doubling of use for youth (27). A 2017 North Carolina Youth Tobacco Survey revealed that 1 in 10 high school students reported vaping cannabis (28). Recently, the FDA has made the public aware of reports numbering in the hundreds of vaping-associated severe lung illnesses and several deaths (29).
With the increased prevalence of vaping, there has been a corresponding increase in the vaping of new synthetic designer CB. Neocannabinoids (nCB) are the newest iteration of CB-like drugs that are intended to mimic THC but are chemical concoctions of various non-CB psychoactive stimulants that are not canonical CB receptor ligands. These chemicals can be sprayed on marijuana leaves and smoked to achieve a desired state of euphoria and are sold under a variety of names/street names that tout special effects (e.g., Black Magic, Crazy Clown, Paradise, Serenity, Spice). From 2009 to 2020, law enforcement agencies have witnessed a proliferation of hundreds of different designer nCB that are hyped and marketed as “legal” substitutes for marijuana and sold under the guise of “herbal incense” or “potpourri” (30, 31). Initially, these synthetic drugs were designed as pharmacological tools to interrogate the eCB and were anticipated to be innovative pharmacotherapeutic agents, now, these once promising nCB are highly abused for their extremely potent psychoactive properties (11, 32–34) With the proliferation of these new and potentially dangerous synthetic drugs flooding the market and the increasing quest of the user to obtain a greater “high,” there exists the distinct possibility that clinicians will soon witness an explosion in the prevalence of perinatal cannabinoid use.
Prevalence of perinatal cannabinoid Use
Has the proliferation of nCB contributed to the increased prevalence of perinatal cannabinoid use?
The FDA-approved THC-based medications further cloud the issue of whether individuals should use these drugs during pregnancy. The available evidence suggests adverse consequences of cannabinoid exposure on female reproductive health, pregnancy, and altered trajectories of fetal development and long-term health outcomes (see Figure 1) (20, 35–37). Additionally, 48%–60% of individuals continue consuming cannabinoids during pregnancy and lactation, which affects approximately 34% of all pregnancies (19, 22, 38) The self-disclosed prevalence of cannabinoid usage during pregnancy ranges from 2% to 5% in most studies but increases to 15%–28% among young, socioeconomically challenged, urban individuals(19). However, due to the longstanding status of cannabinoids as an illicit drug, there is a dearth of well-designed unequivocal studies that assess the effects of perinatal cannabinoid exposure on fetal and placental health outcomes (22, 39). The equivocal results of many studies of perinatal cannabinoid use, the increased potency of natural and synthetic cannabis products, the increased availability of cannabinoids due to ever-increasing legalization for medical and recreational uses, and the perceived innocuousness of cannabinoids have all contributed to an increased prevalence of pregnant individuals using cannabinoid products.
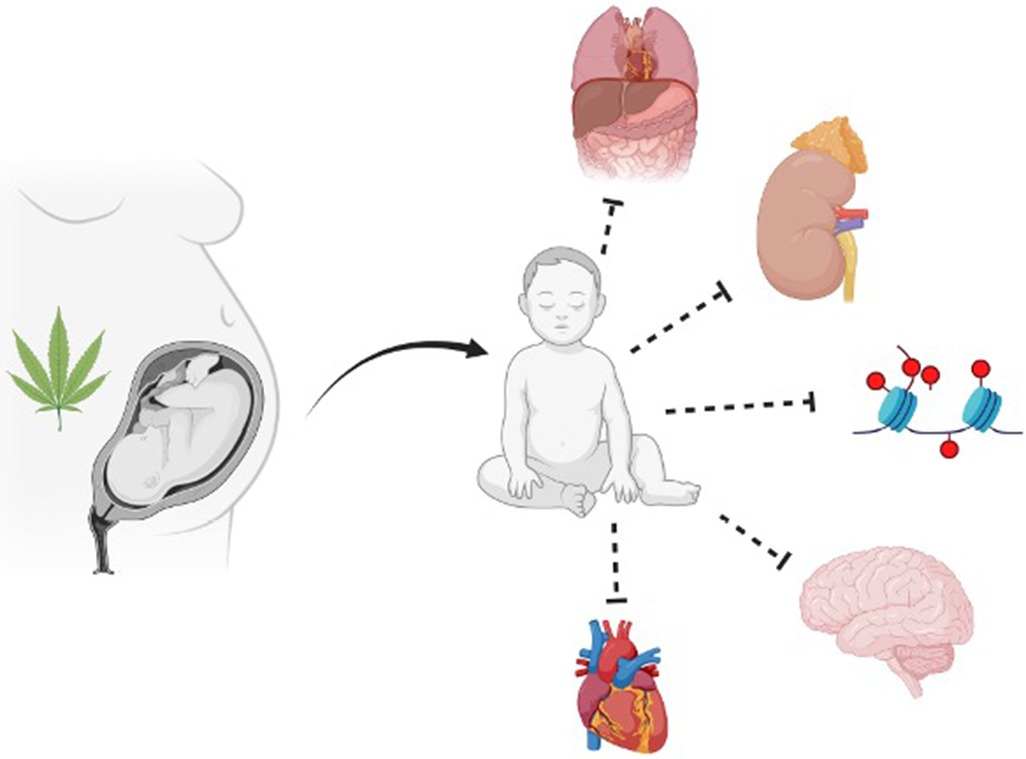
Figure 1. Summary of the broad impact prenatal cannabinoid exposure can have on fetal development. Multiple fetal systems can be impacted by prenatal cannabinoid exposure, including but not limited to abdominal wall, uronephrological, epigenetic, neurological, and cardiac. Figure created with BioRender.com.
During the COVID-19 pandemic, the prevalence of cannabinoid use increased among people of reproductive age, due, in part, to elevated anxiety and stress levels. Specifically, due to COVID-related stressors (e.g., loneliness-induced mental health crises, unemployment, the responsibility of homeschooling added to parental childcare, neglected prenatal care, and fear of being infected with the coronavirus), there was a significant increase in the rates of urinalysis-confirmed prenatal cannabis use among pregnant individuals in Northern California. The increased prevalence of prenatal cannabis use coincided with the dramatic increase in sale of cannabis in California at that time, as cannabis dispensaries were deemed to be essential businesses (40, 41), and identified three primary reasons why individuals use cannabinoids during pregnancy and lactation: (1) escapism or sensation seeking for pleasure, (2) management of chronic disease or amelioration of pregnancy-related symptoms, and (3) as a mechanism for coping with the vicissitudes of life. The authors concluded that the divergent reasons for pre-conceptional cannabinoid use and use during pregnancy and lactation highlights the participants' perceptions of the benefits and safety profile of cannabinoids and may be an effort to justify their usage as therapeutic to overcome the perceived stigma of perinatal drug use (41).
Epigenetics and exogenous cannabinoids
What effects do exogenous cannabinoids have on the developing fetus and through what mechanism are these effects potentially transmitted to subsequent generations?
The quote, “If DNA is thought of as the cells’ bioinformatic ‘hardware’ then the epigenome can be considered its programming ’software’” (42) is apropos. Epigenetics is the study of how heritable alterations of DNA can modify gene expression and alter the phenotype without altering the nucleotide sequence (43). Epigenetic modifications play a crucial role in guaranteeing that cells commit to a specific mitotically and meiotically inheritable phenotype and maintain the stability of the genome. The epigenetic mark-induced silencing of centromeres, telomeres, and transposons ensures that the spindle microtubules correctly connect to centromeres, decreases the incidence of recombination between repetitive sequences, and prevents translocation of transposons, which can result in mutations (43). These epigenetic modifications are thought to be the ideal candidates for the molecular mechanism by which environmental stimuli can be translated into heritable alterations of the DNA or chromatin structure. That is, epigenetic modifications are how extended perinatal (i.e., from early gestational through breastfeeding 20) cannabinoid exposure could lead to lasting effects, some of which can be transmitted to successive generations.
In a review by Basavarajappa et al., several studies highlight the importance of further evaluation of CB receptor regulation by epigenetic modifications (13, 44). Di Marzo et al. eloquently describes the discovery of an expanded eCB, the endocannabinoidome (eCBome), an elaborate signaling system that participates in neuronal development and synaptic plasticity in most brain areas (13, 45–47) and is subsequently involved in the onset, progression, and symptomology of major neuropsychiatric disorders, providing a potential target for the development of novel therapeutics against these disorders [see Figure 2 (11–13)]. The eCBome is comprised of several non-eCB long-chain fatty acid amides and esters including (a) AEA and 2-AG congeners, (b) N-acyl-amino acids, (c) N-acylated dopaminergic and serotonergic neurotransmitters, and (d) primary fatty acid amides. AEA and 2-AG congeners can produce their effects by sharing AEA and 2-AG biosynthetic or degradative enzymes, and sometimes their receptors (13). The eCB and the eCBome mediate, and are clinical targets used to treat, several neurodegenerative diseases, including Alzheimer's disease, Huntington's disease, Parkinson's disease, Amyotrophic lateral sclerosis, Multiple sclerosis, Epilepsy, Glioblastoma, Stroke, and Traumatic brain injury (13).
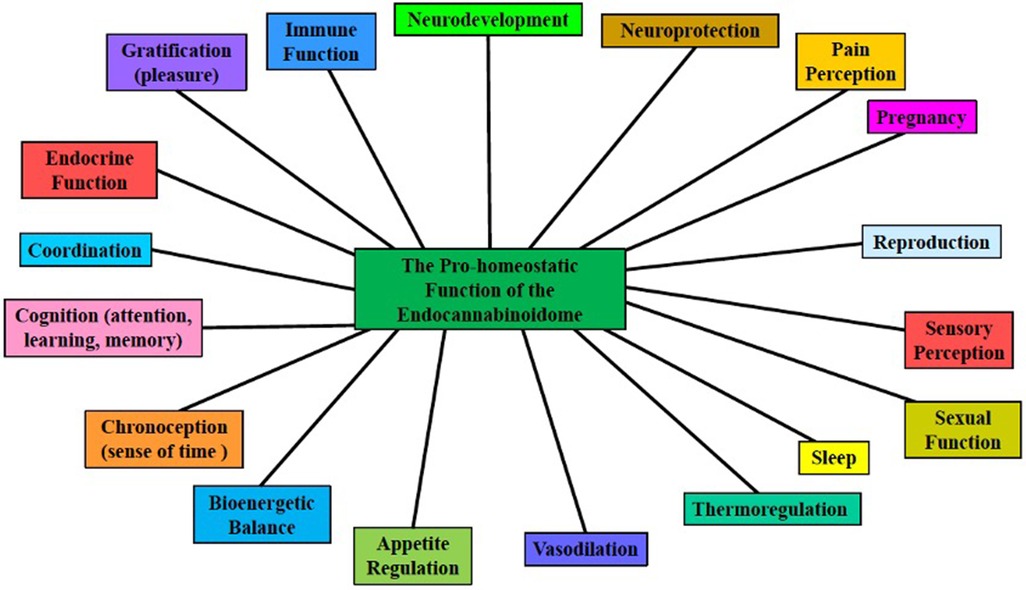
Figure 2. The pro-homeostatic function of the endocannabinoidome. The ubiquitous expanded endocannabinoid system (eCB, i.e., the endocannabinoidome, eCBome), which consists of many eCB-like lipid mediators, and their metabolic enzymes and molecular targets, is an overarching neuromodulatory system that plays crucial roles in neurodevelopment and neuroplasticity, in several physiological and cognitive processes, and responds to endogenous and exogenous perturbations [modified from Sun and Dey (11), Orsolini et al. (12), Di Marzo (13)].
The existence of the eCBome partially explains why some non-psychotropic CB, which modulate several eCBome proteins, are effective for the treatment of Multiple sclerosis and Epilepsy (13). Specifically, the FDA-approved, as a safe and effective tablet formulation of the THC-based medication, dronabinol (Marinol®) is an appetite stimulant for HIV/AIDS-induced anorexia and antiemetic for chemotherapy-induced nausea and vomiting, and nabilone (Cesamet®) is an antiemetic and a treatment for obstructive sleep apnea. Several other THC-based medications have been FDA-approved or are currently undergoing clinical trials. In several countries outside of the United States, nabiximols (Sativex®), an oral spray that is available for treating Multiple Sclerosis-induced spasticity and neuropathic pain, is a combination of THC and CBD. The FDA approved Epidiolex®, a CBD-based liquid formulation, for the treatment of Dravet Syndrome and Lennox-Gastaut Syndrome, which are two forms of rare, severe childhood epilepsy (29, 48).
The precise underlying molecular mechanisms that maintain the enduring effects of perinatal cannabis exposure remain to be fully elucidated. Evaluation of fetal brain tissue with prenatal cannabinoid exposure revealed a dose dependent decrease in dopamine receptor D2 mRNA levels in the nucleus accumbens region and has been hypothesized to contribute to adverse psychiatric outcomes following prenatal cannabinoid exposure (49). This finding was replicated in a preclinical study of rats, with the reduction of D2 mRNA levels persisting into adulthood (49). Epigenetic mechanisms are, indeed, the most logical candidates to explain protracted phenotypic alterations because the epigenome (i.e., the totality of all epigenetic marks/chromatin scars throughout the genome) can translate environmental exposures (e.g., perinatal drug exposure, diet, drugs, stressors, and toxins) into stable alterations of the genome by providing a means by which perinatal cannabis exposure can alter genes and their associated phenotypes [see Figure 3 (50)]. Lifestyle choices and environmental stressors can retune the neuroepigenetic machinery, which can impact an individual's susceptibility to drugs or mental illness by exacerbating perinatal cannabinoid-induced alterations in gene expression that undergird the transition from impulsive to compulsive drug taking (i.e., addiction).
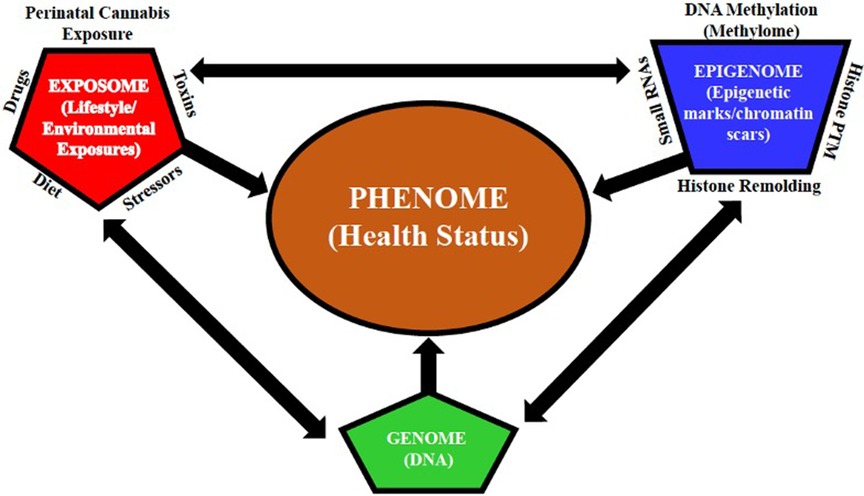
Figure 3. Multidimensional interactions between the genome, epigenome, and exposome determine the phenome. The multidimensional interactions between the genome (DNA), epigenome (i.e., the sum of all epigenetic marks/chromatin scars throughout the genome), and exposome (lifestyle/environmental exposures) determine the phenome (health status). Epigenetic alterations induced by cumulative lifestyle and environmental exposures (e.g., perinatal drug exposure, diet, drugs, stressors, and toxins), leading to alterations in the germline of the exposed individual, can be transmitted to the subsequent generations. The interaction between lifestyle and environmental exposures is represented by the exposome (alters the epigenome via DNA methylation (methylome), histone remodeling and post-translational modification (PTM), and gene expression regulators (small RNAs)), which, in turn, alters the genome, without altering the DNA sequence, and modulates gene expression, which, as a result, alters the phenome, which represents all phenotypic traits [modified from Paoloni-Giacobino (50)].
How do epigenetic modifications contribute to a maternally cannabinoid exposed individual's subsequent susceptibility to disease or mental illness?
The “thrifty phenotype” hypothesis of the etiology of non-insulin-dependent Type 2 diabetes can be applied to perinatal cannabinoid induced fetal/prenatal programming (i.e., the hypothesis that maternal cannabinoid use during fetal development plays a decisive role in determining health trajectories across the lifespan of the offspring), and this hypothesis ignited interest in the fetal origins of adult diseases, and, in 2003, the International Society for Developmental Origins of Health and Disease was founded (51). Fetal programming represents the impact of neurodevelopmental plasticity in response to lifestyle and environmental stressors during early life and the potential adverse outcomes later in life (52). Prenatal programming is regulated by fetal genetic information (i.e., the fetal genome), which is composed of both the maternal oocyte epigenome and paternal sperm epigenome both of which underwent epigenetic modifications in response to parental lifestyle/environmental factors. These epigenetic alterations can, not only, influence the health trajectory of the fetus but the phenotype of the fetus' offspring (i.e., gametic transmission) (53).
Why are preclinical studies paramount to our understanding of the effects of perinatal cannabinoid exposure in humans on neurodevelopment and fetal and adult outcomes in exposed offspring?
The use of non-human animal, specifically rodent, models in research is paramount to our elucidation of the effects of perinatal exposure on human development, behavior, and health, minimizing confounding variables, and establishing cause and effect relationships between the exposure and potential epigenetic alterations. For example, a recent study by Lee, et al. was able to demonstrate that prenatal cannabinoid exposure resulted in significantly smaller hearts size relative to body weight in a rat model with postnatal cardiac remodeling and impaired cardiac function (54). Indeed, a causal inferential modelling and space-time statistical analysis showed a strong bivariate relationship of prenatal cannabinoid exposure and cardiac anomalies (55), thus stressing the need for continued investigations into the etiology of altered development. Preclinical animal studies allow the interrogation of the molecular consequences of long-term cannabinoid use (i.e., epigenetic alterations) that could potentially perpetuate abnormal gene expression and the associated behavioral consequences (56–59) Given that over 50% of all pregnancies in the United States are unintentional (i.e., potential exposure of both parents to cannabinoids 60) it is important to highlight the main consequences of perinatal cannabinoid smoking in such a profound manner that it will make an indelible mark on the psyches of women and men of reproductive age, especially, members of historically marginalized and urban populations, whose communities are being inundated with high potency, easily available natural and synthetic cannabis products, so that those who are contemplating having a baby will not dare use cannabinoids before conception, during and after pregnancy and lactation, or during their child's adolescence. With this goal in mind, we will focus on prenatal cannabinoid exposure by summarizing in chronological order the preclinical studies on the effects of PCE in rats and mice [see Supplementary Table S1 (61, 62)]. For the sake of brevity and because of the detailed nature of the table, we will not recap the experiments included in the table herein.
Contributions of clinical studies: what have they revealed about prenatal cannabinoid exposure?
Human studies of cannabinoid exposure during pregnancy that were performed in the 1980′s, when prevalence of use and THC potency were lower, partially support disturbances in fetal neurodevelopment, increased risks of stillbirth, increased incidence of fetal growth restriction, and long-term adverse neurodevelopmental outcomes (35). Additionally, retrospective studies that control for confounding variables (e.g., polysubstance use, inadequate sample size, unhealthy lifestyle) are scarce and, thus, yield contradictory results, as many of these studies rely solely on uncorroborated patient self-report, which increases recall bias, precluding a definitive causal relationship between negative developmental outcomes and perinatal cannabinoid use (19, 22, 63). Unlike the standardization of distinct types of alcohol pours by volume, there is no such standardization for cannabinoid potency because different strains of marijuana plants and the route of administration vary in potency. Currently, there is no dependable method to accurately quantify in biological samples the amount of cannabinoid used. Therefore, it should be a global imperative to develop and validate reliable analytical methods for cannabinoid screening (22, 64).
To date, there have been six longitudinal studies that investigate the developmental correlates of prenatal cannabis exposure (PCE). These include the Ottawa Prenatal Prospective Study (OPPS), Maternal Health Practices and Child Development Study (MHPCD), Generation R study (GenR), Adolescent Brain Cognitive Development Study (ABCD), Lifestyle and Early Achievement in Families study (LEAF) and the Norwegian Mother and Child Cohort Study (MoBa). In addition to these single cohort studies, the Environmental Influences on Child Health Outcomes (ECHO) program contains data on PCE. The study characteristics, enrollment criteria and outcome measurements of these longitudinal cohort studies should inform their findings given the everchanging landscape of cannabis use, potency, and social acceptability.
Epidemiological studies using sophisticated space-time and causal inferential statistical analyses have revealed multiple concerning associations, including higher congenital anomalies (65, 66) such as neurological anomalies (67), body wall anomalies (68), and uronephrological congenital anomalies (69). Specifically, the defects include severe microcephaly, craniosynostosis, microphthalmos, anencephalus, hydrocephalus, neural tube defects, omphalocele, diaphragmatic hernia, gastroschisis, bilateral renal agenesis, multicystic renal disease, hydronephrosis, and congenital posterior urethral valves (67–69). Additionally, rates of autism in the United States appear to be associated with PCE, which persists after controlling for other major covariates (70), although large studies are needed to confirm this relationship.
Together these analyses and longitudinal studies have demonstrated associations between PCE and congenital anomalies as well as negative neurocognitive outcomes in children from infancy to late adolescence. The most replicated associations are deficits in short term memory, impulse control, and attention deficiencies. A summary of clinical studies is presented in Table 1. For the sake of brevity and because of the detailed nature of the table, we will not recap the studies included in the table herein.
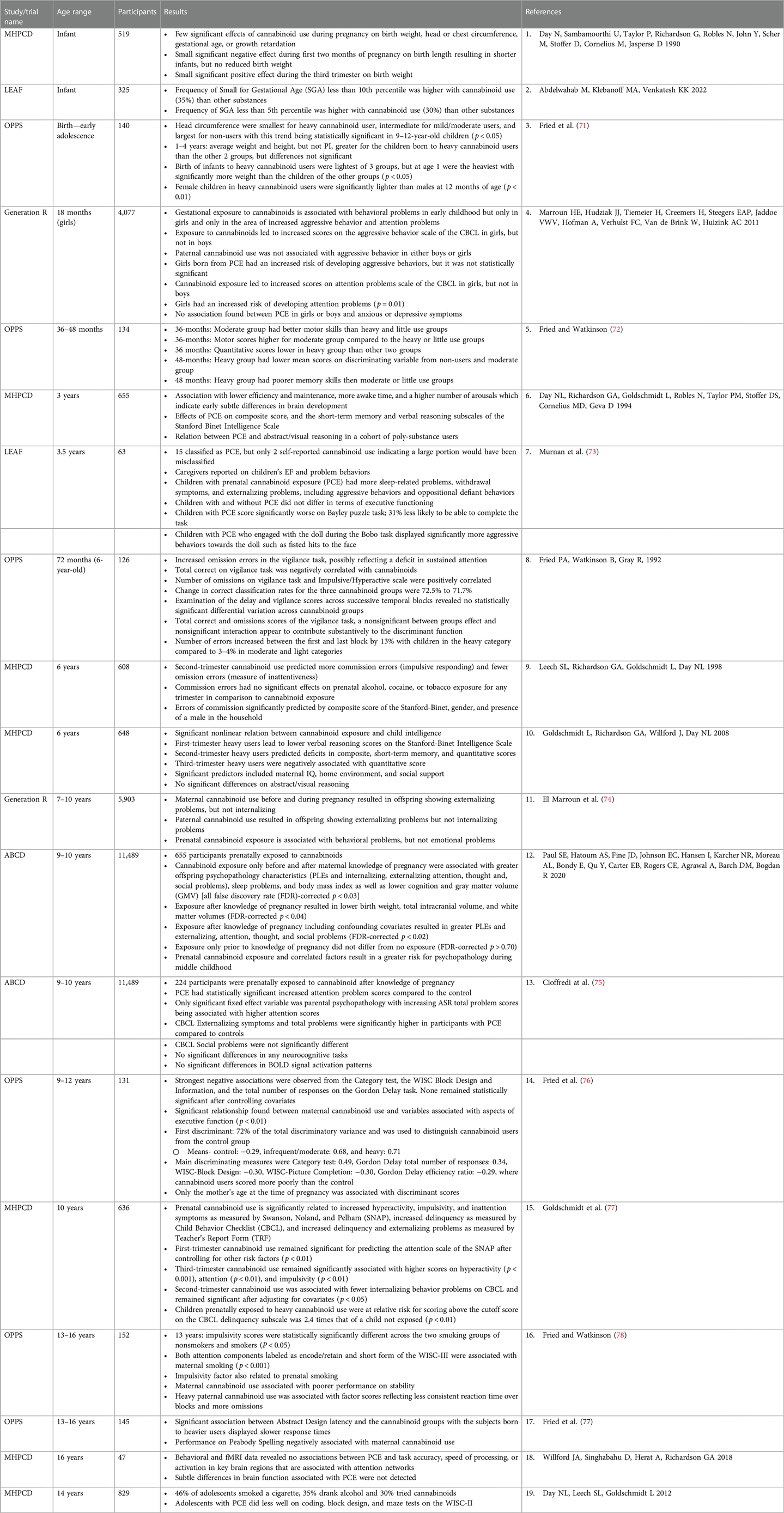
Table 1. Summary of selected clinical studies on the effects of perinatal cannabinoid exposure in infants to young adults.
The Ottawa prospective pregnancy study (OPPS)
Conducted in Ottawa, Canada the OPPS enrolled 698 pregnant individuals between 1978 and 1982 (71). Individuals were recruited from prenatal clinics at the major hospitals in Ottawa, and those with prenatal use of cannabinoids, alcohol, and tobacco were included as well as individuals with no prenatal use (79). The average age of pregnant individuals at enrollment was 29 years, mean family income comparable to the mean family income in the Ottawa metropolitan area, and only 4% had less than a high school education while 70% had more than a high school education (80). Prenatal drug exposures were measured by participant report at each of these interview time points including alcohol, cannabis, and tobacco. Cannabis was measured in marijuana “joints” per week and categorized into non-users, light users (≤1 joint per week or those exposed to second-hand cannabis smoke), moderate users (2–5 joints per week), and heavy users (>5 joints per week).
After the infants were born and initial studies completed, a subset of the original cohort was selected for follow up. This included 140 individuals who used cannabis during pregnancy, or individuals who were heavy users of alcohol or nicotine during pregnancy. Additionally, 50 individuals who reported no use were randomly selected to serve as controls (72). Follow up of this cohort spans 22 years since infants were born. Executive functioning deficits, including difficulty with impulsivity and attention, have been noted through adolescence (76, 78). Although the young adult imaging studies are much smaller in size, the follow up cohort established after birth maintained 80%–83% retention through mid-adolescence (78, 81).
The maternal health practices and child development project (MHPCD)
In 1982, the faculty at the Magee-Women's Hospital in Pittsburg, Pennsylvania began enrolling pregnant individuals in the MHPCD. Potential participants, at least four months into their pregnancy, were randomly selected from outpatient obstetric clinics. All individuals who reported cannabis use as more than two joints a month during their first trimester were chosen for continued follow up, and an equal number of participants who reported less use were randomly selected from the remaining subjects. They completed follow up intervals assessing drug use in their 3rd trimester and birth hospitalization (82). Unlike the OPPS cohort, the MHPCD cohort had a more diverse population of participants with lower average age (22 years) and income (69% less than $5,000 per year) (83).
Alcohol, cannabis, and tobacco exposures were assessed with questionnaires administered prospectively throughout pregnancy. Cannabis quantity was measured in marijuana joints per day, and like OPPS, the use of hashish was considered to count as more cannabis than marijuana and was multiplied by three to “convert” to joints. After the birth assessment, children in the MHPCD cohort were followed up at multiple time points. At 10 years of age, PCE was significantly related to abnormal executive functioning with increased hyperactivity, impulsivity, and inattention symptoms in children with exposure (77). At 22 years of age, most of the original cohort (69%, 524 individuals) was retained for continued assessment (84).
Generation R (GenR) study
The GenR study is a population-based prospective cohort study based in Rotterdam, Netherlands. Pregnant individuals and their partners residing in Rotterdam with delivery dates between April 2002 and January 2006 were eligible for participation. A total of 9,778 pregnant individuals were enrolled, and 1,232 participants were enrolled in the subgroup who would participate in more detailed assessments of health and development during the preschool years of the children. This cohort includes participants whose average maternal age at enrollment was 30.5 years (older than those previously mentioned), and a less diverse population with most participants being Dutch or other European ancestry and having at least secondary school education (89%) (85).
Cannabis exposure was measured via participant report during the first trimester with a question asking about cannabis use in the past 3 months (to capture before pregnancy) and had answers “No,” “Yes until I knew I was pregnant” and “Yes I still use.” Additionally, THC metabolites were evaluated in the third trimester using urine testing (74). Unlike OPPS and MHPCD, GenR was not specifically aimed to assess the impact of prenatal drug exposures, but rather to describe normal and abnormal growth and development as well as identify environmental, biological, and social factors, which influence growth and development. In addition to questionnaire and objective assessments this study included biospecimens such as maternal and child blood and urine (85). Interestingly, a sex effect has been noted thus far at age 18 months, with girls having behavioral issues not observed in boys (86) after controlling for parental education, national origin, and parental psychopathology.
The lifestyle and early achievement in families (LEAF) study
With an ambidirectional study design, the LEAF study adds prospective developmental follow up to an existing cohort study at the Ohio State University in Columbus, Ohio. The initial study enrolled participants while they were pregnant in 2010 and continues to enroll at the time of this publication. Initial inclusion criteria for pregnant participants include age 16–50 years, English speaking, and intent to delivery at the Ohio State Medical Center (87). The prenatal protocol includes a question about cannabinoid use during pregnancy. Medical records from the delivery hospitalization, including drug screen results, were obtained at the conclusion of the initial cohort study. Subsequently, a study sample was obtained from those who agreed to be contacted after the original study concluded, and whose children would be old enough for follow-up (71% of the original cohort). This resulted in a cohort of 361 children who were born between 2010 and 2016. Within this follow up cohort, the mean age during pregnancy was 26 years, 31% of the cohort had less than a high school education while 38% had at least some education after high school. The majority (63%) of the sample was African American (87).
Measurement of prenatal cannabis exposure in this cohort is multifaceted. Participants were considered cannabis exposed if there was prenatal self-report of cannabis use, if it was noted in the obstetric medical record, or if any of the urine samples collected during pregnancy had THC concentrations >15 ng/ml (87). There are 117 participants who meet these criteria for prenatal cannabis use. At 3.5 years of age, the PCE children had more sleep-related problems, aggressive behaviors, and oppositional defiant behaviors (73) after controlling for child race, child sex, prenatal tobacco exposure, maternal/caregiver marital status, household income, and maternal/caregiver executive functioning.
The Norwegian mother and child cohort study (MoBa)
Perhaps the largest pregnancy cohort study evaluating prenatal cannabinoid exposure is the Norwegian Mother and Child Cohort Study (MoBa) in which 114,000 children from Norway have been followed for up to 13 years of age, beginning at birth. Enrollment was open to all pregnant individuals in Norway between July 1999 and December 2008. A total of 41% of pregnant Norwegians agreed to be part of the study. Retention through pregnancy was maintained at 95% of those enrolled and fell to 77% by 18-month assessments (88). The data includes biosamples (whole blood, plasma, and urine) collected from pregnant individuals and their partners during pregnancy as well as the pregnant individual and their child at delivery (89). Of the total sample, 9,312 pregnant individuals reported lifetime cannabinoid use resulting in 10,373 pregnancies. However, in only 272 pregnancies had participants used cannabinoids during pregnancy. Cannabinoid use in this large study was self-reported and included only hashish, as it is historically the only form of cannabinoids used in Norway (90).
Adolescent brain child development study (ABCD)
ABCD represents the largest cohort study on child brain development in the United States to date. Baseline assessments began in 2016 when children were 9–10 years old and is planned to have continued assessments for 10 years (91). The study consists of 11,880 children and includes multiple batteries of assessments spanning health and behavior, including mental health, neurodevelopment and cognition, daily activities, exposures, substance use, and neuroimaging (92). Participants were recruited from 21 sites across the country with the goal of mirroring the demographics of the United States. Prenatal exposures, including cannabinoids, were assessed at time of enrollment, and generally categorized into use before knowledge of pregnancy and after. Caregivers were asked 2 questions: “Before you (or the biological mother)” and “Once you (or the biological mother) knew you were (she was) pregnant were you (was she) using any of the following?” The list that followed included tobacco, alcohol, marijuana, other illicit drugs as well as prescription drugs. Possible answers included, “yes,” “no,” and “don't know.” Duration and trimester of use was not collected. For those who reported use, daily frequency was collected by parent or caregiver retrospective report. For children with PCE at 9–10 years of age, significant increases in attention problems were noted (75).
Environmental influences on child health outcomes (ECHO) program
The Environmental Influences on Child Health Outcomes (ECHO) Program is focused on observational research, with support for individual cohort science but more importantly brings separate cohorts together into one large cohort consisting of children, mothers, and fathers (93). The children are followed long-term with data from over 105,000 participants and over 64,000 children as of April 2023 (93). This organization consists of 44 sites across the United States and Puerto Rico (94). Exposure during pregnancy is generally confirmed via self-report. There is an opportunity to use study samples such as urine and measure metabolites to confirm exposure (95). With an abundance of data that continues to be collected, findings from this observational collaboration are regularly being published.
Why should clinicians preemptively caution individuals of child-bearing age to take a pregnant pause from using cannabinoids during their pregnancies?
Since many pregnant individuals opt to use natural or synthetic CB during the perinatal period to alleviate pregnancy-related symptoms, pre-existing maladies, stress, and for its euphoric effects (41), clinicians should caution individuals of child-bearing age of the following: (1) In rats, THC and the primary metabolite, 11-OH-THC, readily cross the blood-brain-barrier to exert significant effects on the brain (96); (2) THC crosses the maternofetal placental barrier in humans and other mammals (11, 97, 98) to bind with CB1R and affect fetal growth and development; (3) In humans, rats, and mice, canonical and non-CB receptors/channels are expressed early in placental tissues(15); (4) The eCB is present and fully functional in early fetal development (99) and exists ubiquitously in the brain from the early embryonic stage through the postnatal stage, playing a pro-homeostatic role in early embryonic and prenatal neurodevelopment (13); (5) In humans, inhaled cannabinoids can be transferred into breast milk (100); (6) Several components of the eCB have been identified in the rat embryo, follicular fluid, ovaries, the placenta, and the uterus (14, 15); and (7) In mice, synthetic cannabinoids can target multiple sites and stages of pregnancy [See Figure 4 (11)]. Therefore, it is reasonable for clinicians to hypothesize that perinatal cannabinoid exposure could potentially have detrimental consequences on fetal neurodevelopment and outcomes.
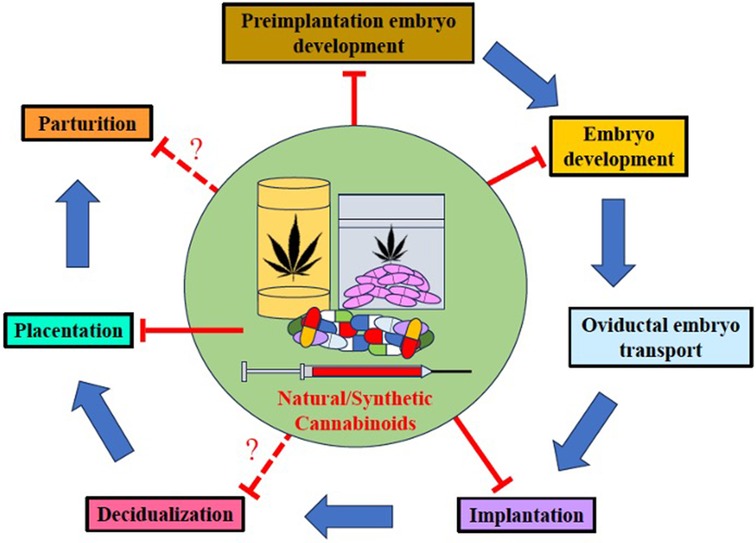
Figure 4. Natural and synthetic cannabinoids can target pregnancy events. Both natural and synthetic cannabinoids target multiple stages of pregnancy. Perinatal use of natural and synthetic cannabinoids disturbs several events in pregnancy including preimplantation embryo development, embryo development, oviductal embryo transport, implantation, placentation, and perhaps, decidualization and parturition [modified from Sun and Dey (11)].
Although the effects of cannabinoids on human fetal neurodevelopment remain to be unequivocally elucidated, some studies suggest that prenatal cannabinoid exposure may be linked to subsequent deficits in attention, learning and memory, critical thinking skills, and behavioral issues in exposed offspring (see Supplementary Table S1). These potential deleterious effects of cannabinoid use during pregnancy on offspring neurodevelopmental outcomes and cognition, which initially may be subtle or undetectable for years after parturition (i.e., the first hit) and manifest only after the second hit (e.g., maternal stressor, and postnatal and caregiver exposures) (35).
Summary and future directions
There are many problems with the existing human studies on perinatal cannabinoid exposure. Many of these studies were conducted over 40 years ago when cannabinoid potency was less, and its prevalence was lower. Several studies are contradictory and limited in breadth, contain experimental design flaws, or are fraught with confounding variables. For instance, retrospective studies, dependent on unreliable study participant self-disclosure (i.e., increased recall bias), were without quantitative urine or meconium assays to accurately assess exposure. Participants' lifestyle choices, which introduced concurrent disease states or polysubstance use, confounded many studies. Lastly in studies with low statistical power (overestimates of effect size and low reproducibility of results) and no standardized outcome measures (101, 102), the conditions preclude the determination of a cannabinoid-specific causal effect.
Given the absence of cause-and-effect evidence of perinatal cannabinoid exposure-induced detrimental fetal neurodevelopment consequences and outcomes, the authors suggest that pregnant individuals practice a cautious approach by taking a pregnant pause from using cannabinoid products (35). Additionally, pregnant individuals or those contemplating pregnancy should cease using cannabinoids for medicinal purposes when an alternative therapy with better pregnancy-specific safety data exists (19).
Author note
Through consulting with a University of New Mexico Health Sciences Librarian (Alexis Ellsworth-Kopkowski) searches were run through PubMed, Web of Science, and Google Scholar. The PubMed search was completed on May 23, 2023, utilizing the keywords: prenatal cannabis exposure and outcomes. These results were filtered to English with the publication years of 2017–2023. The Web of Science search was completed on June 16, 2023, utilizing the keywords: prenatal cannabis exposure and outcomes and human. These results were filtered to English with the publication years of 2017–2023. The Google Scholar search was completed on June 16, 2023, and was deduplicated against the PubMed and Web of Science citations. The keywords prenatal cannabis exposure and outcomes were utilized. These results were filtered to 2019–2023.
Author contributions
AL: Writing – original draft, Writing – review & editing. GD: Writing – original draft, Writing – review & editing. SD: Writing – original draft, Writing – review & editing. ZD: Writing – original draft, Writing – review & editing. LC: Conceptualization, Writing – original draft, Writing – review & editing. GM: Conceptualization, Writing – original draft, Writing – review & editing. JM: Conceptualization, Writing – original draft, Writing – review & editing.
Funding
The author(s) declare that no financial support was received for the research, authorship, and/or publication of this article.
Conflict of interest
The authors declare that the research was conducted in the absence of any commercial or financial relationships that could be construed as a potential conflict of interest.
Publisher's note
All claims expressed in this article are solely those of the authors and do not necessarily represent those of their affiliated organizations, or those of the publisher, the editors and the reviewers. Any product that may be evaluated in this article, or claim that may be made by its manufacturer, is not guaranteed or endorsed by the publisher.
Supplementary material
The Supplementary Material for this article can be found online at: https://www.frontiersin.org/articles/10.3389/fped.2023.1278227/full#supplementary-material
References
1. ElSohly MA, Mehmedic Z, Foster S, Gon C, Chandra S, Church JC. Changes in Cannabis potency over the last 2 decades (1995–2014): analysis of current data in the United States. Biol Psychiatry. (2016) 79(7):613–9. doi: 10.1016/j.biopsych.2016.01.004
2. ElSohly MA, Radwan MM, Gul W, Chandra S, Galal A. Phytochemistry of Cannabis sativa L. Prog Chem Org Nat Prod. (2017) 103:1–36. doi: 10.1007/978-3-319-45541-9_1
3. ElSohly MA, Chandra S, Radwan M, Majumdar CG, Church JC. A comprehensive review of Cannabis potency in the United States in the last decade. Biol Psychiatry Cogn Neurosci Neuroimaging. (2021) 6(6):603–6. doi: 10.1016/j.bpsc.2020.12.016
4. Grotenhermen F. Pharmacokinetics and pharmacodynamics of cannabinoids. Clin Pharmacokinet. (2003) 42(4):327–60. doi: 10.2165/00003088-200342040-00003
5. Pertwee RG. The diverse CB1 and CB2 receptor pharmacology of three plant cannabinoids: delta9-tetrahydrocannabinol, cannabidiol and delta9-tetrahydrocannabivarin. Br J Pharmacol. (2008) 153(2):199–215. doi: 10.1038/sj.bjp.0707442
6. Matsuda LA, Lolait SJ, Brownstein MJ, Young AC, Bonner TI. Structure of a cannabinoid receptor and functional expression of the cloned cDNA. Nature. (1990) 346(6284):561–4. doi: 10.1038/346561a0
7. Munro S, Thomas KL, Abu-Shaar M. Molecular characterization of a peripheral receptor for cannabinoids. Nature. (1993) 365(6441):61–5. doi: 10.1038/365061a0
8. Mechoulam R, Hanus LO, Pertwee R, Howlett AC. Early phytocannabinoid chemistry to endocannabinoids and beyond. Nat Rev Neurosci. (2014) 15(11):757–64. doi: 10.1038/nrn3811
9. Hu SS, Mackie K. Distribution of the endocannabinoid system in the central nervous system. Handb Exp Pharmacol. (2015) 231:59–93. doi: 10.1007/978-3-319-20825-1_3
10. Freeman TP, Craft S, Wilson J, Stylianou S, ElSohly M, Di Forti M, et al. Changes in delta-9-tetrahydrocannabinol (THC) and cannabidiol (CBD) concentrations in cannabis over time: systematic review and meta-analysis. Addiction. (2021) 116(5):1000–10. doi: 10.1111/add.15253
11. Sun X, Dey SK. Synthetic cannabinoids and potential reproductive consequences. Life Sci. (2014) 97(1):72–7. doi: 10.1016/j.lfs.2013.06.018
12. Orsolini L, Papanti D, Corkery J, De Luca MA, Cadoni C, Di Chiara G, et al. Is there a teratogenicity risk associated with Cannabis and synthetic Cannabimimetics’ (’Spice’) intake? CNS Neurol Disord Drug Targets. (2017) 16(5):585–91. doi: 10.2174/1871527316666170413101257
13. Di Marzo V. The endocannabinoidome as a substrate for noneuphoric phytocannabinoid action and gut microbiome dysfunction in neuropsychiatric disorders. Dialogues Clin Neurosci. (2020) 22(3):259–69. doi: 10.31887/DCNS.2020.22.3/vdimarzo
14. Martinez-Pena AA, Perono GA, Gritis SA, Sharma R, Selvakumar S, Walker OS, et al. The impact of early life exposure to Cannabis: the role of the endocannabinoid system. Int J Mol Sci. (2021) 22(16):1–22. doi: 10.3390/ijms22168576
15. Rokeby ACE, Natale BV, Natale DRC. Cannabinoids and the placenta: receptors, signaling and outcomes. Placenta. (2023) 135:51–61. doi: 10.1016/j.placenta.2023.03.002
16. Lowe H, Toyang N, Steele B, Bryant J, Ngwa W. The endocannabinoid system: a potential target for the treatment of various diseases. Int J Mol Sci. (2021) 22(17):1–42. doi: 10.3390/ijms22179472
17. Hasin DS, Borodovsky J, Shmulewitz D, Walsh C, Livne O, Struble CA, et al. Use of highly-potent cannabis concentrate products: more common in U.S. States with recreational or medical cannabis laws. Drug Alcohol Depend. (2021) 229(Pt B):109159. doi: 10.1016/j.drugalcdep.2021.109159
18. Ko JY, Farr SL, Tong VT, Creanga AA, Callaghan WM. Prevalence and patterns of marijuana use among pregnant and nonpregnant women of reproductive age. Am J Obstet Gynecol. (2015) 213(2):201.e1–201.e10. doi: 10.1016/j.ajog.2015.03.021
19. Opinion AC. Marijuana Use During Pregnancy and Lactation The American College of Obstetricians and Gynecologists 2017;130(Number 722) (ACOG Committee Opinion)).
20. Scheyer AF, Melis M, Trezza V, Manzoni OJJ. Consequences of perinatal Cannabis exposure. Trends Neurosci. (2019) 42(12):871–84. doi: 10.1016/j.tins.2019.08.010
21. UNODC. World Drug Report 2022. United Nations publication (2022). Available at: www.unodc.org/unodc/en/data-and-analysis/world-drug-report-2022.html
22. Thompson R, DeJong K, Lo J. Marijuana use in pregnancy: a review. Obstet Gynecol Surv. (2019) 74(7):415–28. doi: 10.1097/OGX.0000000000000685
23. Statistics NCfDA. Marijuana Addiction: Rates & Usage Statistics (2023). Available at: https://drugabusestatistics.org/marijuana-addiction/
24. Grant KS, Conover E, Chambers CD. Update on the developmental consequences of cannabis use during pregnancy and lactation. Birth Defects Res. (2020) 112(15):1126–38. doi: 10.1002/bdr2.1766
25. Tai H, Swartz MD, Marsden D, Perry CL. The future of substance abuse now: relationships among adolescent use of vaping devices, marijuana, and synthetic cannabinoids. Subst Use Misuse. (2021) 56(2):192–204. doi: 10.1080/10826084.2020.1849305
26. Lim CCW, Sun T, Leung J, Chung JYC, Gartner C, Connor J, et al. Prevalence of adolescent Cannabis vaping: a systematic review and meta-analysis of US and Canadian studies. JAMA Pediatr. (2022) 176(1):42–51. doi: 10.1001/jamapediatrics.2021.4102
27. Schulenberg JE PM, Johnston LD, O’Malley PM, Bachman JG, Miech RA. Monitoring the future national survey results on drug use, 1975–2020: Volume II, college students and adults ages 19–60. (2021). Available at: http://monitoringthefuture.org/pubs.html#monographs
28. Kowitt SD, Osman A, Meernik C, Zarkin GA, Ranney LM, Martin J, et al. Vaping cannabis among adolescents: prevalence and associations with tobacco use from a cross-sectional study in the USA. BMJ Open. (2019) 9(6):e028535. doi: 10.1136/bmjopen-2018-028535
29. NIDA. Marijuana and hallucinogen use among young adults reached all time-high in 2021. National Institute on Drug Abuse 2022. Available at: https://nida.nih.gov/news-events/news-releases/2022/08/marijuana-and-hallucinogen-use-among-young-adults-reached-all-time-high-in-2021
30. Heinbockel T, Csoka AB. Epigenetic effects of drugs of abuse. Int J Environ Res Public Health. (2018) 15(10). doi: 10.3390/ijerph15102098
31. NIDA. Marijuana and hallucinogen use among young adults reached all time-high in 2021. (2022). p. 1–7. Available at: https://nida.nih.gov/news-events/news-releases/2022/08/marijuana-and-hallucinogen-use-among-young-adults-reached-all-time-high-in-2021
32. Seely KA, Prather PL, James LP, Moran JH. Marijuana-based drugs: innovative therapeutics or designer drugs of abuse? Mol Interv. (2011) 11(1):36–51. doi: 10.1124/mi.11.1.6
33. Psychoyos D, Vinod KY. Marijuana, spice ‘herbal high’, and early neural development: implications for rescheduling and legalization. Drug Test Anal. (2013) 5(1):27–45. doi: 10.1002/dta.1390
34. Kemp AM, Clark MS, Dobbs T, Galli R, Sherman J, Cox R. Top 10 facts you need to know about synthetic cannabinoids: not so nice spice. Am J Med. (2016) 129(3):240–4.e1. doi: 10.1016/j.amjmed.2015.10.008
35. McLemore GL, Richardson KA. Data from three prospective longitudinal human cohorts of prenatal marijuana exposure and offspring outcomes from the fetal period through young adulthood. Data Brief. (2016) 9:753–7. doi: 10.1016/j.dib.2016.10.005
36. Navarrete F, Garcia-Gutierrez MS, Gasparyan A, Austrich-Olivares A, Femenia T, Manzanares J. Cannabis use in pregnant and breastfeeding women: behavioral and neurobiological consequences. Front Psychiatry. (2020) 11:586447. doi: 10.3389/fpsyt.2020.586447
37. Corsi DJ, Murphy MSQ, Cook J. The effects of Cannabis on female reproductive health across the life course. Cannabis Cannabinoid Res. (2021) 6(4):275–87. doi: 10.1089/can.2020.0065
38. Administration SAaMHS. Key substance use and mental health indicators in the United States: results from the 2017 national survey on drug use and health (HHS Publication No. SMA 18-5068, NSDUH Series H-53). (2018). Available at: https://www. samhsa.gov/data/
39. Scheyer AF, Borsoi M, Pelissier-Alicot AL, Manzoni OJJ. Maternal exposure to the cannabinoid agonist WIN 55,12,2 during lactation induces lasting behavioral and synaptic alterations in the rat adult offspring of both sexes. eNeuro. (2020) 7(5):1–11. doi: 10.1523/ENEURO.0144-20.2020
40. Young-Wolff KC, Ray GT, Alexeeff SE, Adams SR, Does MB, Ansley D, et al. Rates of prenatal cannabis use among pregnant women before and during the COVID-19 pandemic. JAMA. (2021) 326(17):1745–7. doi: 10.1001/jama.2021.16328
41. Vanstone M, Taneja S, Popoola A, Panday J, Greyson D, Lennox R, et al. Reasons for cannabis use during pregnancy and lactation: a qualitative study. CMAJ. (2021) 193(50):E1906–14. doi: 10.1503/cmaj.211236
42. Reece AS, Hulse GK. Impacts of cannabinoid epigenetics on human development: reflections on murphy et al. ‘cannabinoid exposure and altered DNA methylation in rat and human sperm’ epigenetics 2018; 13: 1208-1221. Epigenetics. (2019) 14(11):1041–56. doi: 10.1080/15592294.2019.1633868
43. Dupont C, Armant DR, Brenner CA. Epigenetics: definition, mechanisms and clinical perspective. Semin Reprod Med. (2009) 27(5):351–7. doi: 10.1055/s-0029-1237423
44. Basavarajappa BS, Subbanna S. Molecular insights into epigenetics and cannabinoid receptors. Biomolecules. (2022) 12(11):1–20. doi: 10.3390/biom12111560
45. Gaffuri AL, Ladarre D, Lenkei Z. Type-1 cannabinoid receptor signaling in neuronal development. Pharmacology. (2012) 90(1–2):19–39. doi: 10.1159/000339075
46. Manduca A, Campolongo P, Trezza V. Cannabinoid modulation of mother-infant interaction: is it just about milk? Rev Neurosci. (2012) 23(5–6):707–22. doi: 10.1515/revneuro-2012-0074
47. Lu HC, Mackie K. An introduction to the endogenous cannabinoid system. Biol Psychiatry. (2016) 79(7):516–25. doi: 10.1016/j.biopsych.2015.07.028
48. (SAMHSA) SAaMHSA. Preventing the use of marijuana: focus on women and pregnancy. SAMHSA Publication No PEP19-PL-Guide-2 2019(Substance Abuse and Mental Health Services Administration)).
49. DiNieri JA, Wang X, Szutorisz H, Spano SM, Kaur J, Casaccia P, et al. Maternal cannabis use alters ventral striatal dopamine D2 gene regulation in the offspring. Biol Psychiatry. (2011) 70(8):763–9. doi: 10.1016/j.biopsych.2011.06.027
50. Paoloni-Giacobino A. Post genomic decade–the epigenome and exposome challenges. Swiss Med Wkly. (2011) 141:w13321. doi: 10.4414/smw.2011.13321
51. Hales CN, Barker DJ. Type 2 (non-insulin-dependent) diabetes mellitus: the thrifty phenotype hypothesis. 1992. Int J Epidemiol. (2013) 42(5):1215–22. doi: 10.1093/ije/dyt133
52. Gicquel C, El-Osta A, Le Bouc Y. Epigenetic regulation and fetal programming. Best Pract Res Clin Endocrinol Metab. (2008) 22(1):1–16. doi: 10.1016/j.beem.2007.07.009
53. Donkin I, Barres R. Sperm epigenetics and influence of environmental factors. Mol Metab. (2018) 14:1–11. doi: 10.1016/j.molmet.2018.02.006
54. Lee K, Laviolette SR, Hardy DB. Exposure to Delta9-tetrahydrocannabinol during rat pregnancy leads to impaired cardiac dysfunction in postnatal life. Pediatr Res. (2021) 90(3):532–9. doi: 10.1038/s41390-021-01511-9
55. Reece AS, Hulse GK. European Epidemiological patterns of cannabis- and substance-related congenital cardiovascular anomalies: geospatiotemporal and causal inferential study. Environ Epigenet. (2022) 8(1):dvac015. doi: 10.1093/eep/dvac015
56. Szutorisz H, DiNieri JA, Sweet E, Egervari G, Michaelides M, Carter JM, et al. Parental THC exposure leads to compulsive heroin-seeking and altered striatal synaptic plasticity in the subsequent generation. Neuropsychopharmacology. (2014) 39(6):1315–23. doi: 10.1038/npp.2013.352
57. Szutorisz H, Egervari G, Sperry J, Carter JM, Hurd YL. Cross-generational THC exposure alters the developmental sensitivity of ventral and dorsal striatal gene expression in male and female offspring. Neurotoxicol Teratol. (2016) 58:107–14. doi: 10.1016/j.ntt.2016.05.005
58. Szutorisz H, Hurd YL. Epigenetic effects of cannabis exposure. Biol Psychiatry. (2016) 79(7):586–94. doi: 10.1016/j.biopsych.2015.09.014
59. Szutorisz H, Hurd YL. High times for cannabis: epigenetic imprint and its legacy on brain and behavior. Neurosci Biobehav Rev. (2018) 85:93–101. doi: 10.1016/j.neubiorev.2017.05.011
60. Schrott R, Murphy SK. Cannabis use and the sperm epigenome: a budding concern? Environ Epigenet. (2020) 6(1):dvaa002. doi: 10.1093/eep/dvaa002
61. Campolongo P, Trezza V, Ratano P, Palmery M, Cuomo V. Developmental consequences of perinatal cannabis exposure: behavioral and neuroendocrine effects in adult rodents. Psychopharmacology (Berl). (2011) 214(1):5–15. doi: 10.1007/s00213-010-1892-x
62. Mulligan MK, Hamre KM. Influence of prenatal cannabinoid exposure on early development and beyond. Adv Drug Alcohol Res (2023) 3:1–24. (Review) (In English). doi: 10.3389/adar.2023.10981
63. Metz TD, Stickrath EH. Marijuana use in pregnancy and lactation: a review of the evidence. Am J Obstet Gynecol. (2015) 213(6):761–78. doi: 10.1016/j.ajog.2015.05.025
64. Antunes M, Barroso M, Gallardo E. Analysis of cannabinoids in biological specimens: an update. Int J Environ Res Public Health. (2023) 20(3):1–36. doi: 10.3390/ijerph20032312
65. Reece AS, Hulse GK. Patterns of cannabis- and substance-related congenital general anomalies in Europe: a geospatiotemporal and causal inferential study. Pediatr Rep. (2023) 15(1):69–118. doi: 10.3390/pediatric15010009
66. Reece AS, Hulse GK. Geotemporospatial and causal inference epidemiological analysis of US survey and overview of cannabis, cannabidiol and cannabinoid genotoxicity in relation to congenital anomalies 2001–2015. BMC Pediatr. (2022) 22(1):47. doi: 10.1186/s12887-021-02996-3
67. Reece AS, Hulse GK. European Epidemiological patterns of cannabis- and substance-related congenital neurological anomalies: geospatiotemporal and causal inferential study. Int J Environ Res Public Health. (2022) 20(1):1–35. doi: 10.3390/ijerph20010441
68. Reece AS, Hulse GK. European epidemiological patterns of cannabis- and substance-related body wall congenital anomalies: geospatiotemporal and causal inferential study. Int J Environ Res Public Health. (2022) 19(15):1–38. doi: 10.3390/ijerph19159027
69. Reece AS, Hulse GK. Epidemiological patterns of cannabis- and substance- related congenital uronephrological anomalies in Europe: geospatiotemporal and causal inferential study. Int J Environ Res Public Health. (2022) 19(21):1–61. doi: 10.3390/ijerph192113769
70. Reece AS, Hulse GK. Impact of converging sociocultural and substance-related trends on US autism rates: combined geospatiotemporal and causal inferential analysis. Eur Arch Psychiatry Clin Neurosci. (2023) 273(3):699–717. doi: 10.1007/s00406-022-01446-0
71. Fried PA, Watkinson B, Gray R. Growth from birth to early adolescence in offspring prenatally exposed to cigarettes and marijuana. Neurotoxicol Teratol. (1999) 21(5):513–25. doi: 10.1016/s0892-0362(99)00009-4
72. Fried PA, Watkinson B. 36- and 48-month neurobehavioral follow-up of children prenatally exposed to marijuana, cigarettes, and alcohol. J Dev Behav Pediatr (1990) 11(2):49–58. Available at: https://www.ncbi.nlm.nih.gov/pubmed/2324288 doi: 10.1097/00004703-199004000-00003
73. Murnan AW, Keim SA, Yeates KO, Boone KM, Sheppard KW, Klebanoff MA. Behavioral and cognitive differences in early childhood related to prenatal marijuana exposure. J Appl Dev Psychol. (2021) 77:1–26. doi: 10.1016/j.appdev.2021.101348
74. El Marroun H, Bolhuis K, Franken IHA, Jaddoe VWV, Hillegers MH, Lahey BB, et al. Preconception and prenatal cannabis use and the risk of behavioural and emotional problems in the offspring; a multi-informant prospective longitudinal study. Int J Epidemiol. (2019) 48(1):287–96. doi: 10.1093/ije/dyy186
75. Cioffredi LA, Anderson H, Loso H, East J, Nguyen P, Garavan H, et al. Prenatal cannabis exposure predicts attention problems, without changes on fMRI in adolescents. Neurotoxicol Teratol. (2022) 91:107089. doi: 10.1016/j.ntt.2022.107089
76. Fried PA, Watkinson B, Siegel LS. Reading And language in 9- to 12-year olds prenatally exposed to cigarettes and marijuana. Neurotoxicol Teratol. (1997) 19(3):171–83. doi: 10.1016/s0892-0362(97)00015-9
77. Goldschmidt L, Day NL, Richardson GA. Effects of prenatal marijuana exposure on child behavior problems at age 10. Neurotoxicol Teratol. (2000) 22(3):325–36. doi: 10.1016/s0892-0362(00)00066-0
78. Fried PA, Watkinson B. Differential effects on facets of attention in adolescents prenatally exposed to cigarettes and marihuana. Neurotoxicol Teratol. (2001) 23(5):421–30. doi: 10.1016/s0892-0362(01)00160-x
79. Fried PA. The Ottawa prenatal prospective study (OPPS): methodological issues and findings–it’s easy to throw the baby out with the bath water. Life Sci. (1995) 56(23–24):2159–68. doi: 10.1016/0024-3205(95)00203-i
80. Fried PA, Innes KS, Barnes MV. Soft drug use prior to and during pregnancy: a comparison of samples over a four-year period. Drug Alcohol Depend. (1984) 13(2):161–76. doi: 10.1016/0376-8716(84)90056-5
81. Fried PA, Watkinson B, Gray R. Differential effects on cognitive functioning in 13- to 16-year-olds prenatally exposed to cigarettes and marihuana. Neurotoxicol Teratol. (2003) 25(4):427–36. doi: 10.1016/s0892-0362(03)00029-1
82. Day N, Sambamoorthi U, Taylor P, Richardson G, Robles N, Jhon Y, et al. Prenatal marijuana use and neonatal outcome. Neurotoxicol Teratol. (1991) 13(3):329–34. doi: 10.1016/0892-0362(91)90079-c
83. US Department of Commerce Bureau of the Census. General social and economic characteristics United States summary. (1983).
84. Willford JA, Goldschmidt L, De Genna NM, Day NL, Richardson GA. A longitudinal study of the impact of marijuana on adult memory function: prenatal, adolescent, and young adult exposures. Neurotoxicol Teratol. (2021) 84:106958. doi: 10.1016/j.ntt.2021.106958
85. Kooijman MN, Kruithof CJ, van Duijn CM, Duijts L, Franco OH, van IJzendoorn MH, et al. The generation R study: design and cohort update 2017. Eur J Epidemiol. (2016) 31(12):1243–64. doi: 10.1007/s10654-016-0224-9
86. El Marroun H, Tiemeier H, Jaddoe VW, Hofman A, Verhulst FC, van den Brink W, et al. Agreement between maternal cannabis use during pregnancy according to self-report and urinalysis in a population-based cohort: the generation R study. Eur Addict Res. (2011) 17(1):37–43. doi: 10.1159/000320550
87. Klebanoff MA, Fried P, Yeates KO, Rausch J, Wilkins DG, Blei H, et al. Lifestyle and early achievement in families (LEAF) study: design of an ambidirectional cohort study of prenatal marijuana exposure and child development and behaviour. Paediatr Perinat Epidemiol. (2020) 34(6):744–56. doi: 10.1111/ppe.12693
88. Magnus P, Irgens LM, Haug K, Nystad W, Skjaerven R, Stoltenberg C. Cohort profile: the Norwegian mother and child cohort study (MoBa). Int J Epidemiol. (2006) 35(5):1146–50. (In eng). doi: 10.1093/ije/dyl170
89. Carlsen HK, Steingrimsson S, Sigurdsson MI, Sigfusson S, Magnusson A. Cohort profile update: the Norwegian mother and child cohort study (MoBa). Int J Epidemiol. (2016) 45(2):382–8. (In eng). doi: 10.1093/ije/dyw029
90. Gabrhelik R, Mahic M, Lund IO, Bramness J, Selmer R, Skovlund E, et al. Cannabis use during pregnancy and risk of adverse birth outcomes: a longitudinal cohort study. Eur Addict Res. (2021) 27(2):131–41. doi: 10.1159/000510821
91. Potter AS, Dube SL, Barrios LC, Bookheimer S, Espinoza A, Feldstein Ewing SW, et al. Measurement of gender and sexuality in the adolescent brain cognitive development (ABCD) study. Dev Cogn Neurosci. (2022) 53:101057. (In eng). doi: 10.1016/j.dcn.2022.101057
92. Jernigan TL, Brown SA. Introduction. Dev Cogn Neurosci. (2018) 32:1–3. (In eng). doi: 10.1016/j.dcn.2018.02.002
93. About the ECHO Program. (2023). Available at: https://www.nih.gov/echo/about-echo-program
95. Moore BF, Salmons KA, Hoyt AT, Swenson KS, Bates EA, Sauder KA, et al. Associations between prenatal and postnatal exposure to Cannabis with cognition and behavior at age 5 years: the healthy start study. Int J Environ Res Public Health. (2023) 20(6):1–15. doi: 10.3390/ijerph20064880
96. Schou J, Prockop LD, Dahlstrom G, Rohde C. Penetration of delta-9-tetrahydrocannabinol and 11-OH-delta-9-tetrahydrocannabinol through the blood-brain barrier. Acta Pharmacol Toxicol (Copenh. (1977) 41(1):33–8. doi: 10.1111/j.1600-0773.1977.tb02120.x
97. Behnke M, Eyler FD. The consequences of prenatal substance use for the developing fetus, newborn, and young child. Int J Addict. (1993) 28(13):1341–91. doi: 10.3109/10826089309062191
98. Dong C, Chen J, Harrington A, Vinod KY, Hegde ML, Hegde VL. Cannabinoid exposure during pregnancy and its impact on immune function. Cell Mol Life Sci. (2019) 76(4):729–43. doi: 10.1007/s00018-018-2955-0
99. de Fonseca F R, Ramos JA, Bonnin A, Fernandez-Ruiz JJ. Presence of cannabinoid binding sites in the brain from early postnatal ages. Neuroreport. (1993) 4(2):135–8. doi: 10.1097/00001756-199302000-00005
100. Baker T, Datta P, Rewers-Felkins K, Thompson H, Kallem RR, Hale TW. Transfer of inhaled cannabis into human breast milk. Obstet Gynecol. (2018) 131(5):783–8. doi: 10.1097/AOG.0000000000002575
101. Button KS, Ioannidis JP, Mokrysz C, Nosek BA, Flint J, Robinson ES, et al. Power failure: why small sample size undermines the reliability of neuroscience. Nat Rev Neurosci. (2013) 14(5):365–76. doi: 10.1038/nrn3475
Keywords: prenatal cannabinoid exposure, prenatal cannabis exposure, neurodevelopmental outcome, intrauterine exposure, prenatal marijuana exposure
Citation: Lin A, Dent GL, Davies S, Dominguez ZM, Cioffredi L-A, McLemore GL and Maxwell JR (2023) Prenatal cannabinoid exposure: why expecting individuals should take a pregnancy pause from using cannabinoid products. Front. Pediatr. 11:1278227. doi: 10.3389/fped.2023.1278227
Received: 15 August 2023; Accepted: 18 September 2023;
Published: 11 October 2023.
Edited by:
Mohamed E. Abdel-Latif, The Canberra Hospital, AustraliaReviewed by:
Jonathan Michael Davis, Tufts University, United StatesAlbert Reece, University of Western Australia, Australia
© 2023 Lin, Dent, Davies, Dominguez, Cioffredi, McLemore and Maxwell. This is an open-access article distributed under the terms of the Creative Commons Attribution License (CC BY). The use, distribution or reproduction in other forums is permitted, provided the original author(s) and the copyright owner(s) are credited and that the original publication in this journal is cited, in accordance with accepted academic practice. No use, distribution or reproduction is permitted which does not comply with these terms.
*Correspondence: Jessie R. Maxwell anJtYXh3ZWxsQHNhbHVkLnVubS5lZHU=