- 1Department of Pediatrics, The Hospital for Sick Children, University of Toronto, Toronto, ON, Canada
- 2Department of Pediatrics, McMaster Children's Hospital, McMaster University, Hamilton, ON, Canada
Introduction: Children with restrictive cardiomyopathy (RCM) traditionally have a poor prognosis, with most patients either dying or requiring heart transplantation within 2 years of diagnosis. The development of symptoms in RCM suggests advanced disease. However, as screening practices evolve and lead to diagnosis of early disease, identifying appropriate timing of transplant listing becomes increasingly important. In this context we compared outcomes of children with RCM presenting with clinical symptoms to those asymptomatic at initial presentation.
Methods: This retrospective cohort study included 25 patients with RCM presenting to a quaternary care center between 2001 and 2018. Times to transplantation, death, and a composite outcome of adverse cardiac events (CPR, cardioversion, inotropic support, mechanical ventilation, mechanical support, or heart transplant) were compared between those symptomatic and asymptomatic at presentation.
Results: At 2 years following diagnosis, patients asymptomatic at presentation had a significantly better transplant-free survival at 57% compared to 17% for symptomatic patients (p = 0.03). Those asymptomatic at diagnosis also had significantly improved cardiac event-free survival at 71% compared to symptomatic patients at 25% (p = 0.01). In multivariable analysis, cardiac symptoms at presentation remained an independent risk factor for heart-transplant or death [hazard ratio 5.17 (1.28–20.85), p = 0.02].
Conclusion: Patients with RCM who are symptomatic at time of diagnosis have significantly worse transplant-free survival and cardiac event-free survival. Given current practice variability in timing of transplant listing, the presence of any cardiac symptoms is an important negative prognostic marker and should prompt urgent transplant listing.
1. Introduction
Restrictive cardiomyopathy (RCM) is the least common form of cardiomyopathy, accounting for 2%–5% of pediatric cardiomyopathies (1–3). RCM is characterized by diastolic dysfunction, with normal or decreased volume of both ventricles associated with biatrial enlargement, normal left ventricular wall thickness and atrioventicular valves, and impaired ventricular filling with restrictive physiology (3, 4).
Pediatric RCM has been associated with worse outcomes than other forms of cardiomyopathies with approximately 50% of patients either dying or requiring heart transplant within 2 years of diagnosis (2, 5–12). This is contributed to at least in part by challenges in establishing diagnosis due to absence of symptoms in early disease and difficulties in using currently available mechanical circulatory support (MCS) options to support RCM patients in end-stage heart failure to transplant (13). Of those who do present with symptoms, presentation varies from palpitations, chest pain, syncope, congestive heart failure (CHF) symptoms, and even sudden cardiac death, which has been attributed to subendocardial ischemia secondary to high diastolic pressures (1, 3, 14). Unlike cardiomyopathies characterized by systolic ventricular dysfunction, where evidence-based guidelines exist on timing of heart transplant listing based on clinical status and heart failure severity, risk factors for death in RCM are less well-established and listing practices are largely center dependent (2, 15–17).
As cardiomyopathy screening practices evolve, and children are identified at earlier disease stages, ascertaining those patients in whom there is a clear survival benefit from early listing for transplant becomes critical. Therefore, our study sought to ascertain transplant-free survival and cardiac event-free survival in pediatric RCM patients who present with and without cardiac symptoms at time of diagnosis.
2. Materials and methods
2.1. Construction of the study cohort
The SickKids Heart Failure (HF) database is a retrospective cohort study of pediatric patients with cardiomyopathy or HF seen at a single, quaternary cardiac transplant center, the Hospital for Sick Children, Toronto, ON and diagnosed between January 2001 and July 2018 (18). Subjects were identified by screening the electronic medical record and the electronic echocardiogram (echo) database for the diagnosis of “restrictive cardiomyopathy”. Subjects were considered to meet diagnostic criteria for RCM if they had a documented diagnosis by a HF cardiologist and demonstrated evidence on echo of normal or reduced ventricular volumes, with atrial enlargement, normal ventricular wall thickness and atrioventricular valves, and diastolic dysfunction (4). Patients with increased posterior wall thickness and/or septal thickness (z-score >2) were reviewed by 2 HF pediatric cardiologists (EJ and AJ). Other subtypes that were identified were those with RCM and significant ventricular hypertrophy (RCM with HCM features), but who did not meet criteria for HCM (19). Similarly, patients with increased myocardial trabeculation, but who did not fulfill diagnostic criteria for left ventricular non compaction (LVNC), were reviewed and included if the patients were felt to have predominantly RCM; these patients were classified as RCM with features of left ventricular non compaction (RCM with LVNC features) (20). Therefore, these 2 groups of patients were included as they would not have fit into a primary diagnosis of either HCM or LVNC.
Subjects were excluded if they were 18 years of age or older, had congenital heart disease, metabolic disease, or were never evaluated by a cardiologist at our institution. The RCM patients were then divided into those without cardiac symptoms (asymptomatic patients) to patients with cardiac-related symptoms (symptomatic patients) at the time of initial diagnosis.
2.2. Data collection
Baseline characteristics including sex, age at diagnosis, status at diagnosis, family history of cardiomyopathy, and pathogenic genetic mutation were compared between the asymptomatic and symptomatic RCM patients. Cardiac investigations including basic functional echos, electrocardiograms (ECGs), cardiac magnetic resonance imaging (MRI), and Holter monitors at diagnosis and during follow-up were also compared between the 2 groups. Echo z-scores were calculated relative to body-surface area as per the Pediatric Heart Network calculator (21). The presence of ectopy on ECG was defined as frequent premature ventricular contractions or non-sustained ventricular tachycardia (VT) (3 or more beats at >120 beats per minute). On Holter monitoring, the presence of ectopy was defined as >500 ventricular extrasystoles in 24 hours, runs of ventricular ectopy of >30 seconds in duration, or non-sustained VT (3 or more beats at >120 beats per minute for < 30 seconds). Ventricular tachycardia was defined as sustained ventricular arrhythmia at >120 beats per minute for >30 seconds (19, 22). Signs of ischemia were defined as episodic T wave inversion, new ST depression with cardiac symptoms, or ST depression >3 mm on Holter monitoring.
Cardiac care needs during follow-up were also compared, including use of heart failure medications, number of cardiac outpatient visits, cardiac hospitalizations, and intensive care unit (ICU) admissions.
2.3. Endpoints
Study participants were followed from date of diagnosis until end of the study period, or until death, heart transplant, or transition to adult cardiac care. Primary outcome was transplant-free survival. Secondary outcomes included cardiac event-free survival, defined as survival without incurring cardiopulmonary resuscitation (CPR), cardioversion, inotropic support, mechanical ventilation, MCS, or heart transplant. Time to symptom onset was examined for patients who were asymptomatic at diagnosis.
2.4. Statistical analysis
Descriptive statistics were reported as mean ± standard deviation or median and interquartile range (IQR). Baseline, operative, and echo characteristics were compared between the asymptomatic and symptomatic patients at time of RCM diagnosis using Chi-square, student's t-test, and Wilcoxon rank-sum tests as appropriate. Simple group comparisons were made with the log-rank test and displayed using the Kaplan–Meier method.
Candidate variables were identified for risk factor analysis. Bootstrap aggregation with resampling (500 resamples) was used for variable selection (23). Candidate variables that were found in at least 25% of the bootstrap models were subjected to a backward selection, with a retention threshold of p < 0.05. The final multivariable Cox regression model was obtained. The level of statistical significance was set at p = 0.05. Statistical analyses were performed using SAS 9.4 software Statistical Analysis System (RRID:SCR_008567).
3. Results
3.1. Baseline characteristics of cohort
A total of 25 patients with RCM met inclusion criteria, of whom 7 (28%) were asymptomatic at diagnosis and 18 (72%) were symptomatic (Table 1). Most symptomatic patients presented with CHF 14 (78%), with the remainder presenting with cardiac arrest 2 (11%), chest pain 1 (4%), or hemoptysis 1 (4%). Asymptomatic patients were younger at diagnosis (age <3 years: 100% vs. 39%, p = 0.05) and were more likely to be outpatient at time of diagnosis (86% vs. 28%, p = 0.05). There were no significant differences between groups for presence of a pathogenic mutation in MYH7, MYBPC3, or TTN (29% vs. 39%, p = 1.00), or a first degree relative with cardiomyopathy (14% vs. 11%, p = 0.83) (Table 1).
Symptomatic patients were more likely to have RCM with HCM features than asymptomatic patients (39% vs. 0%, p = 0.05). There was no significant difference in incidence of RCM with LVNC features between groups (Table 1). On baseline ECGs, there were no significance differences in terms of presence of left ventricular (LV) hypertrophy (29% vs. 44%, p = 0.66), right ventricular (RV) hypertrophy (43% vs. 39%, p = 1.00), q-waves (0% vs. 17%, p = 0.53), or presence of ischemic changes (14% vs. 44%, p = 0.16).
3.2. Follow-up care
The median time to primary end-point of death, transplant, or transition to adult services was 0.97 years [IQR: 0.54–2.17]. The asymptomatic group was followed for a longer during the study period than the symptomatic group, (2.26 years [IQR: 0.97–4.11] vs. 0.76 years [IQR: 0.20–1.71] respectively, (p = 0.03). On follow-up investigations, there were no significant differences between the asymptomatic and symptomatic groups for the presence of at least moderately reduced LV systolic function (14% vs. 33%, p = 0.63), at least moderately reduced RV function (14% vs. 33%, p = 0.63), or at least moderate mitral regurgitation (14% to 17%, p = 1.00) on echos (Supplementary Table S1). Of the entire RCM cohort, 40% (n = 10) had a cardiac MRI, demonstrating no significant differences between groups for moderate to severely reduced LV systolic function (25% vs. 17%, p = 1.00), moderate to severely reduced RV systolic function (25% vs. 0%, p = 0.4), or evidence of fibrosis (0% vs. 17%, p = 1.00). On follow-up ECGs, there were no significant differences between the asymptomatic and symptomatic groups for the presence of LV hypertrophy (29% vs. 56%, p = 0.38), RV hypertrophy (57% vs. 56%, p = 0.94), q-waves (0% vs. 22%, p = 0.90), ischemic changes (29% vs. 56%, p = 0.38), or ventricular ectopy (17% vs. 11%, p = 1.00). Similarly, on Holter monitoring, we observed no significant differences in terms of the presence of ischemic changes (43% vs. 56%, p = 0.67), ectopy (29% vs. 0%, p = 0.47), or ventricular tachycardia (14% vs. 6%, p = 0.49) between the 2 groups.
Despite longer follow-up of the asymptomatic group during the study period, we observed no differences between groups in the median number of outpatient cardiac appointments (7 [IQR: 0–16] vs. 4 [IQR: 0–11], p = 0.83) or median number of inpatient cardiac admissions (1 [IQR: 0–2] vs. 1 [IQR: 1–3], p = 0.2) (Table 2). Additionally, there were no significant differences between groups in exposure to beta-blockers (14% vs. 44%, p = 0.35), angiotensin converting enzyme (ACE) inhibitors (0% vs. 11%, p = 1.00), or need for inotropic support (43% vs. 28%, p = 0.64). Moreover, there were also no significant differences in implantable cardiac defibrillator (ICD) use (29% vs. 22%, p = 1.00), extracorporeal membrane oxygenation (ECMO) support (0% vs. 17%, p = 0.53), mechanical ventilation (14% vs. 44%, p = 0.35), need for CPR (0% vs. 28%, p = 0.27), or cardioversion (0% vs. 6%, p = 0.52).
3.3. Transplant-free survival
At 2 years following RCM diagnosis, the cohort's overall transplant-free survival was 28% [95% CI 16%–61%] (Figure 1A). Amongst the 2 groups, patients without symptoms at diagnosis had a significantly better transplant-free survival 2 years following diagnosis at 57% [95% CI 20%–96%] compared to 17% [95% CI 2%–62%] for patients with symptoms at diagnosis (p = 0.03) (Figure 1B). Additionally, patients diagnosed with RCM who were greater than 3 years of age at time of diagnosis had a significantly improved 2-year transplant-free survival compared to patients who were less than 3 years of age [33% vs. 14% (p = 0.02)] (Figure 1C). There was no significant difference in 2-year transplant-free survival between the RCM, RCM with HCM features, and RCM with LVNC features [31% vs. 14% vs. 40%, (p = 0.82)] (Figure 1D).
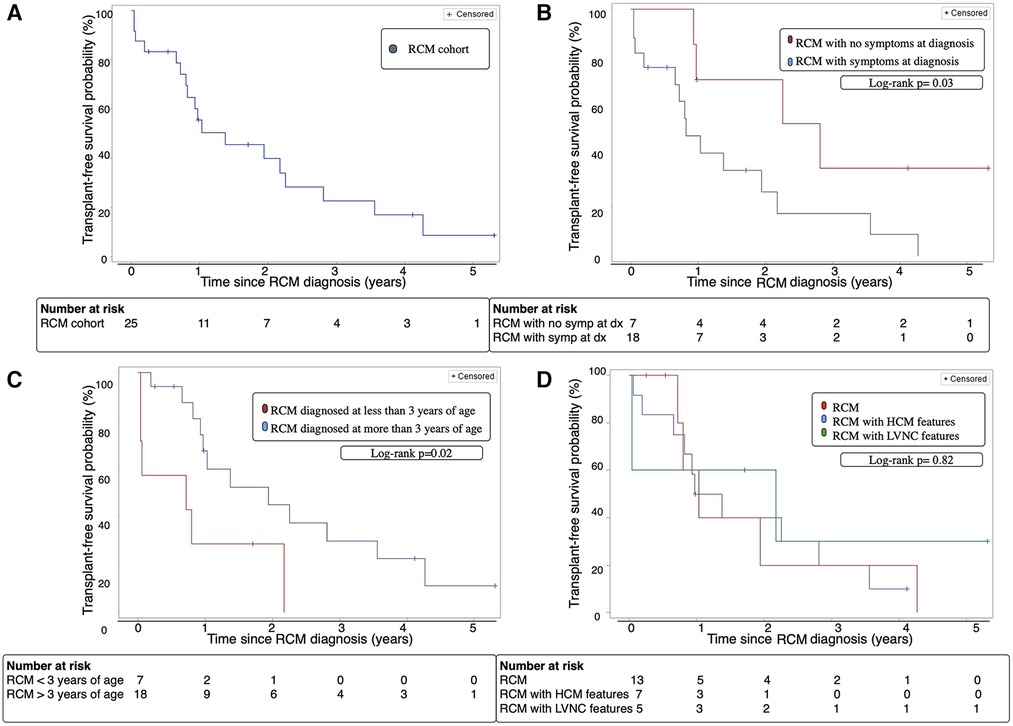
Figure 1. (A) Transplant-free survival of the entire RCM cohort over time from diagnosis. (B) Transplant-free survival of patients with RCM with and without symptoms at diagnosis over time from diagnosis. (C) Transplant-free survival of RCM patients diagnosed before or after age 3 years of age over time from diagnosis. (D) Transplant-free survival of cohort with classic RCM phenotype vs. RCM with HCM features vs. RCM with LVNC features. Dx; diagnosis, HCM; hypertrophic cardiomyopathy, LVNC; left ventricular non-compaction cardiomyopathy, RCM; restrictive cardiomyopathy, Symp; symptoms.
Of the entire cohort, 64% patients were listed for heart-transplant during the study period, 4 (57%) patients in the asymptomatic group and 12 (66%) patients in the symptomatic group, p = 0.66 (Table 2). At time of listing, half of those patients were listed as high priority (Canadian Pediatric Heart Transplant status 3 and 4) (24). All the asymptomatic patients and 66% symptomatic patients that were listed received a heart transplant during the study period, p = 0.57. The median waitlist time was 101 days (15–273), with no significant difference between the groups. There were no deaths in patients asymptomatic at diagnosis during the study period, whereas 39% (n = 7) of the symptomatic patients died (p = 0.13). The causes of death included heart failure (22%), sudden cardiac death (11%), and stroke (5%).
Independent risk factors for heart-transplant or death included younger age at diagnosis [HR 0.85 (0.76–0.96), p = 0.007], symptoms at diagnosis [HR 5.17 (1.28–20.85), p = 0.02], and ever requiring admission to the ICU [HR 6.3 (1.77–22.47), p = 0.005] (Table 3).
3.4. Cardiac event-free survival
As described above, cardiac event-free survival was defined as survival without incurring CPR, cardioversion, inotropic support, mechanical ventilation, MSC, or heart transplant. The overall cardiac event free survival was 39% at 2 years after diagnosis (Figure 2A).
Patients who were asymptomatic at diagnosis had significantly improved cardiac event-free survival after 2 years compared to symptomatic patients; 71% [95% CI 15–93] vs. 25% [95% CI 3–61], p = 0.01 (Figure 2B). Event-free survival was not significantly different for younger RCM patients (43% vs. 29%, p = 0.08) (Figure 2C), or those with RCM, RCM with HCM features, and RCM with LVNC features (38% vs. 20% vs. 60%, p = 0.68) (Figure 2D).
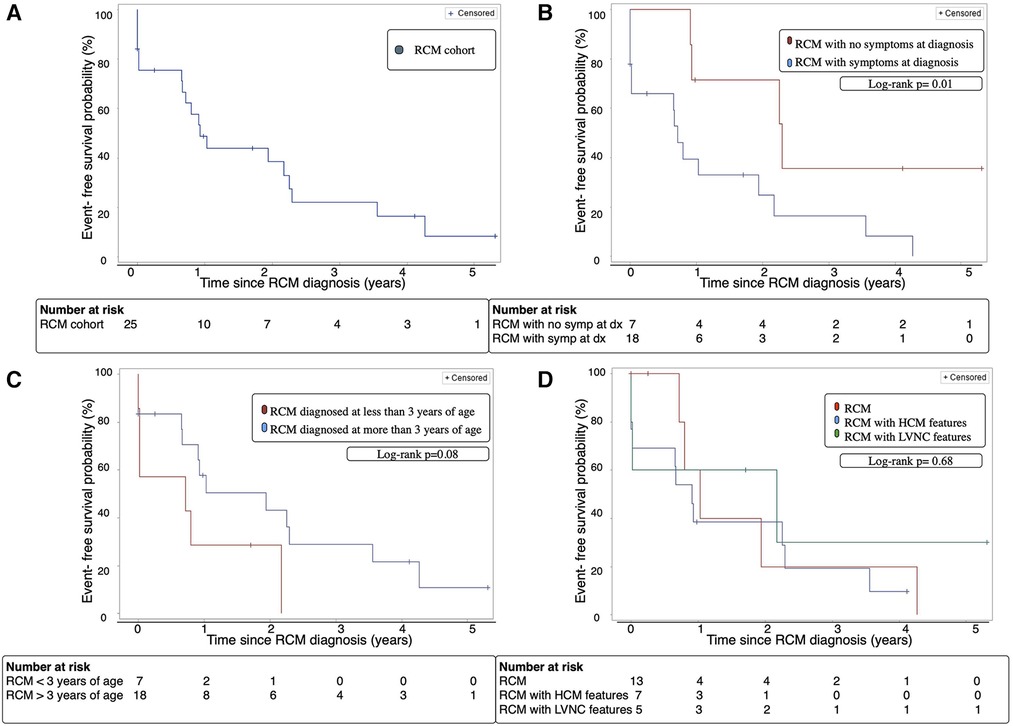
Figure 2. (A) Cardiac event-free survival of the entire RCM cohort. (B) Cardiac event-free survival of patients with RCM with and without symptoms at diagnosis. (C) Cardiac event-free survival of RCM patients diagnosed before or after age 3 years. (D) Cardiac event-free survival of cohort with classic RCM phenotype vs. RCM with HCM features vs. RCM with LVNC features. Cardiac event: cardiopulmonary resuscitation, cardioversion, inotropic support, mechanical ventilation, mechanical circulatory support, heart transplant or death, Dx; diagnosis, HCM; hypertrophic cardiomyopathy, LVNC; left ventricular non-compaction cardiomyopathy, RCM; restrictive cardiomyopathy, Symp; symptoms.
3.5. Time to symptom development
The median time for symptom development in the asymptomatic group was 11.9 months [IQR:3.0–24.7]. When we assessed RCM patients who were initially asymptomatic from the time they developed symptoms, there were no significant differences in transplant-free survival at 2 years after symptom onset (40% vs. 26%, p = 0.5, Figure 3A) or cardiac event-free survival at 2 years after symptom onset (40% vs. 25%, p = 0.36, Figure 3B) compared to patients who were symptomatic at time of diagnosis.
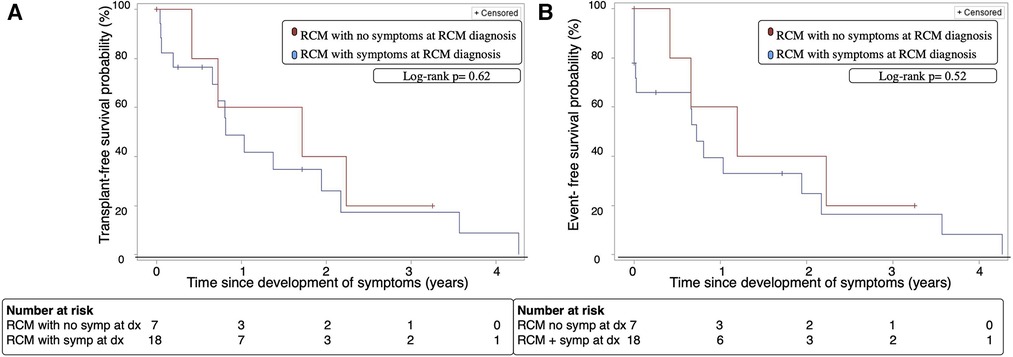
Figure 3. (A) Transplant-free survival of patients with RCM with and without symptoms at diagnosis over time from onset of symptoms. (B) Cardiac-event free survival of patients with RCM with and without symptoms at diagnosis over time from onset of symptoms. Event; cardiopulmonary resuscitation, cardioversion, inotropic support, mechanical ventilation, mechanical circulatory support, heart transplant or death, Dx; diagnosis, RCM; restrictive cardiomyopathy, Symp; symptoms.
4. Discussion
4.1. Symptomatic presentation and outcomes
In this 16-year cohort study, we provide insights into the significance of cardiac symptom development on outcomes of pediatric RCM. We found that patients with any cardiac symptoms at time of diagnosis had a significantly worse transplant-free survival compared to asymptomatic patients. In addition, patients symptomatic at diagnosis were more likely to experience adverse cardiac events including resuscitated cardiac arrest, need for inotropic support, mechanical ventilation, and MCS. Furthermore, repeat time-to-event analysis performed from time of onset of symptoms for those who developed symptoms after initial diagnosis showed similar time to death or transplant from symptom onset as those with symptoms at diagnosis. This emphasizes the importance of prompt evaluation and listing in RCM patients presenting with cardiac symptoms.
Asymptomatic patients in our cohort were followed for longer with overall lower burden of care needs (fewer outpatient visits and admissions per year) than those patients who were symptomatic at time of diagnosis. However, ultimately the proportion of patients requiring advanced heart failure therapies, including MCS and ICDs, was similar between groups, and outcomes after onset of symptoms were similar to those symptomatic at time of diagnosis. Similar findings have been shown in previous studies, with the North American Pediatric Cardiomyopathy Registry (PCMR) group demonstrating that CHF at RCM diagnosis was an independent risk factor for time to death or transplant (2). Wittekind et al. also found that greater than 2 heart failure admissions was associated with a worse composite outcome of death, transplant, MCS, and cardiac arrest (25). While our database did not include detailed information on reason for initial referral, our data suggests that patients presenting for screening with early disease have a less hazardous risk profile during early follow-up than those presenting with cardiac symptoms.
4.2. Age at presentation and outcomes
Consistent with previous reports, our study also demonstrated that patients who were younger at diagnosis of RCM were at increased risk of adverse outcomes, with children diagnosed at less than 3 years of age having both a worse transplant-free survival and cardiac-event-free survival (16). On multivariate analysis, younger age at diagnosis remained an independent risk factor for heart transplant or death. While variability exists in previous reports of risk of death vs. transplant in infants with RCM, which may be influenced by transplant listing practices, our data clearly illustrates a poor cardiac risk profile in this cohort with increased need for advanced cardiac therapies (25). The correlation between younger age at diagnosis and poor outcomes is likely multifactorial, contributed to by difficulty assessing and diagnosing heart failure symptoms in infants, challenges with ICD insertion in this cohort, lack of viable options for durable MCS, and poorer outcomes on MCS. This is largely due to challenges achieving adequate decompression using conventional left ventricular apical cannulation in the presence of restrictive physiology with enlarged atria. Atrial cannulation may be considered, but is particularly challenging in smaller infants, and existing data on MCS use in children with RCM suggests significantly lower survival rates on support in those less than 3 years at time of implant (26).
4.3. Transplant listing in pediatric RCM
Given survival outcomes and long-term morbidities after transplant, and geographic variations in waitlist times and listing priority for RCM patients, ascertaining the most appropriate time for listing remains a challenge (27–29). This is further complicated by the risk of evolving pulmonary hypertension in those with significant diastolic dysfunction and left atrial hypertension-increasing waitlist and perioperative risks. Compared to other forms of cardiomyopathies, which are characterized by systolic ventricular dysfunction, and where clear guidelines exist on timing of heart transplant listing, listing practices for RCM are largely center dependent due to lack of consensus statements (2, 15–17). Recent changes in the US Organ Procurement and Transplantation Network (OPTN) transplant listing criteria deemed inotrope dependent RCM patients no longer eligible for status 1A, and RCM patients listed under 1 year of age automatically status 1B eligible. However, studies have demonstrated this change to have lead to increased mortality in RCM patients not listed as status 1A exception. The Canadian Heart Transplant Listing Criteria affords a higher listing status (higher priority akin to United Network for Organ Sharing (UNOS) status 1B) to those with RCM over other cardiomyopathies who do not need hospitalization. At our institution, medical management of RCM patients includes discussion around ICD implantation, diuretics for symptom control, and anticoagulation/antiplatelet therapy due to the risk of thromboembolism. ACE-inhibitors and beta-blocker are not routinely used in these patients. The majority of patients that present with RCM undergo a discussion and evaluation for heart transplantation. The decision to list a patient for transplantation is dependent on multiple factors, pulmonary vascular resistance being one of them. However, we do not employ a hard cut-off in this group, but rather anticipate the need for aggressive right ventricular support post-transplant, including MCS. Prior to transplantation, the suitable donor organ is selected based on appropriate size match, which needs to be individualized based on recipient size and cardiac size on imaging. Our data, demonstrating variability in outcomes based on clinical status at time of presentation, reiterates the need for larger multicenter cohort studies to facilitate appropriate risk stratification of pediatric RCM patients and better inform transplant listing practices (30–36).
4.4. Outcomes in RCM with LVNC or HCM features
A unique aspect of this study was the assessment of myocardial phenotypes in RCM, which includes patients across the spectrum with RCM with HCM features and RCM with LVNC features. When compared to the classic RCM cohort, there were no differences in transplant-free survival or cardiac event-free survival amongst patients with RCM with HCM features and RCM with LVNC features. Our study excluded patients with congenital heart disease and metabolic disease, and evaluated only RCM phenotypes that did not fulfill diagnostic criteria for other types of cardiomyopathies (HCM or LVNC), therefore placing an emphasis on predominantly restrictive disease secondary to RCM. This would suggest that in isolated RCM, the appearance of hypertrophied or trabeculated myocardium does not influence outcomes. However, it has been shown that HCM with restrictive physiology, though still a severe entity, may not have the same survival outcomes as RCM, highlighting the importance of careful attention to functional imaging at the time of diagnosis (2, 37).
4.5. Limitations
This was a single center, retrospective study with a small sample size. As such, we were underpowered to study the relationship between individual clinical and genetic parameters and outcomes. Given the long period under study and evolution of echocardiographic protocols for diastolic function over time, we were unable to provide a detailed assessment of diastolic function or analyze the relationship between echocardiographic parameters and outcomes. However, given the rarity of pediatric RCM, many studies to-date have also limited by similar factors (5–12, 25, 38–39).
5. Conclusion
Children with RCM remain a high-risk group with a significantly increased risk of death or cardiac events than other cardiomyopathy cohorts. Our data delineates the significant difference in transplant and cardiac event-free survival outcomes in those presenting with cardiac symptoms vs. those presenting asymptomatic or on screening, and indicates the need for urgent evaluation and listing in those presenting at younger age or with cardiac symptoms. While cardiac transplant remains the only viable life-prolonging intervention in RCM, this study highlights the need for further research to facilitate risk stratification and predict optimal timing of transplant listing in patients presenting with early disease.
Data availability statement
The original contributions presented in the study are included in the article/Supplementary Material, further inquiries can be directed to the corresponding author.
Ethics statement
The studies involving humans was approved by the Research Ethics Board, The Hospital for Sick Children. The studies were conducted in accordance with the local legislation and institutional requirements. Written informed consent for participation was not required from the participants or the participants’ legal guardians/next of kin in accordance with the national legislation and institutional requirements.
Author contributions
ML: Conceptualization, Data curation, Writing – original draft, Visualization. AL: Data curation, Writing – review & editing. JA: Data curation, Writing – review & editing. LF: Writing – review & editing. KG: Writing – review & editing. KA: Data curation, Writing – review & editing. SM: Data curation, Writing – review & editing. DN: Writing – review & editing. AJ: Conceptualization, Methodology, Supervision, Writing – review & editing, Funding acquisition. EJ-S-M: Conceptualization, Formal Analysis, Funding acquisition, Investigation, Methodology, Supervision, Validation, Visualization, Writing – review & editing.
Funding
The author(s) declare financial support was received for the research, authorship, and/or publication of this article.
This study was funded by The Cardiac Precision Medicine Program of the Ted Rogers Centre for Heart Research and The Emmet Jeffrey Foster Endowment SickKids Foundation Fund.
Acknowledgments
Thank you to DN for her work on the SickKids Heart Failure database.
Conflicts of interest
AL was supported by a Heart Failure Research Fellowship from Bristol-Myers Squibb, Mitacs, and Myant during this research study. AJ is a medical monitor for the Pumpkin trial and employed as a consultant for Abbott.
The remaining authors declare that the research was conducted in the absence of any commercial or financial relationships that could be construed as a potential conflict of interest.
Publisher's note
All claims expressed in this article are solely those of the authors and do not necessarily represent those of their affiliated organizations, or those of the publisher, the editors and the reviewers. Any product that may be evaluated in this article, or claim that may be made by its manufacturer, is not guaranteed or endorsed by the publisher.
Supplementary material
The Supplementary Material for this article can be found online at: https://www.frontiersin.org/articles/10.3389/fped.2023.1264751/full#supplementary-material
References
1. Price JF, Jeewa A, Denfield SW. Clinical characteristics and treatment of cardiomyopathies in children. CCR. (2016) 12(2):85–98. doi: 10.2174/1573403X12666160301115543
2. Webber SA, Lipshultz SE, Sleeper LA, Lu M, Wilkinson JD, Addonizio LJ, et al. Outcomes of restrictive cardiomyopathy in childhood and the influence of phenotype: a report from the pediatric cardiomyopathy registry. Circulation. (2012) 126(10):1237–44. doi: 10.1161/CIRCULATIONAHA.112.104638
3. Albakri A. Restrictive cardiomyopathy: a review of literature on clinical status and meta-analysis of diagnosis and clinical management. Pediatr Dimens. (2018) 3(2):1–14. doi: 10.15761/PD.1000169
4. Maron BJ, Towbin JA, Thiene G, Antzelevitch C, Corrado D, Arnett D, et al. Contemporary definitions and classification of the cardiomyopathies. Circulation. (2006) 113(14):1807–16. doi: 10.1161/CIRCULATIONAHA.106.174287
5. Weller RJ, Weintraub R, Addonizio LJ, Chrisant MRK, Gersony WM, Hsu DT. Outcome of idiopathic restrictive cardiomyopathy in children. Am J Cardiol. (2002) 90(5):501–6. doi: 10.1016/S0002-9149(02)02522-5
6. Cetta F, O’Leary PW, Seward JB, Driscoll DJ. Idiopathic restrictive cardiomyopathy in childhood: diagnostic features and clinical course. Mayo Clin Proc. (1995) 70(7):634–40. doi: 10.4065/70.7.634
7. Anderson HN, Cetta F, Driscoll DJ, Olson TM, Ackerman MJ, Johnson JN. Idiopathic restrictive cardiomyopathy in children and young adults. Am J Cardiol. (2018) 121(10):1266–70. doi: 10.1016/j.amjcard.2018.01.045
8. Chen SC, Balfour IC, Jureidini S. Clinical spectrum of restrictive cardiomyopathy in children. J Heart Lung Transplant. (2001) 20(1):90–2. doi: 10.1016/S1053-2498(00)00162-5
9. Lewis AB. Clinical profile and outcome of restrictive cardiomyopathy in children. Am Heart J. (1992) 123(6):1589–93. doi: 10.1016/0002-8703(92)90814-C
10. Russo LM. Idiopathic restrictive cardiomyopathy in children. Heart. (2005) 91(9):1199–202. doi: 10.1136/hrt.2004.043869
11. Hayashi T, Tsuda E, Kurosaki K, Ueda H, Yamada O, Echigo S. Electrocardiographic and clinical characteristics of idiopathic restrictive cardiomyopathy in children. Circ J. (2007) 71(10):1534–9. doi: 10.1253/circj.71.1534
12. Denfield SW, Rosenthal G, Gajarski RJ, Bricker JT, Schowengerdt KO, Price JK, et al. Restrictive cardiomyopathies in childhood. Etiologies and natural history. Tex Heart Inst J. (1997) 24(1):38–44.9068138
13. Muchtar E, Blauwet LA, Gertz MA. Restrictive cardiomyopathy: genetics, pathogenesis, clinical manifestations, diagnosis, and therapy. Circ Res. (2017) 121(7):819–37. doi: 10.1161/CIRCRESAHA.117.310982
14. Kucera F, Fenton M. Update on restrictive cardiomyopathy. Paediatr Child Health (Oxford). (2017) 27(12):567–71. doi: 10.1016/j.paed.2017.10.002
15. Dipchand AI, Naftel DC, Feingold B, Spicer R, Yung D, Kaufman B, et al. Outcomes of children with cardiomyopathy listed for transplant: a multi-institutional study. J Heart Lung Transplant. (2009) 28(12):1312–21. doi: 10.1016/j.healun.2009.05.019
16. Zangwill SD, Naftel D, L’Ecuyer T, Rosenthal D, Robinson B, Kirklin JK, et al. Outcomes of children with restrictive cardiomyopathy listed for heart transplant: a multi-institutional study. J Heart Lung Transplant. (2009) 28(12):6. doi: 10.1016/j.healun.2009.06.028
17. Mehra MR, Canter CE, Hannan MM, Semigran MJ, Uber PA, Baran DA, et al. The 2016 international society for heart lung transplantation listing criteria for heart transplantation: a 10-year update. J Heart Lung Transplant. (2016) 35(1):1–23. doi: 10.1016/j.healun.2015.10.023
18. Cardoso B, Jeewa A, Minn S, Ashkanase J, Lynch A, Jean-St-Michel E. Left ventricular noncompaction cardiomyopathy: left ventricular dilation and dysfunction at baseline portend the risk of death or heart transplantation. Can J Cardiol. (2022) 38(6):754–62. doi: 10.1016/j.cjca.2022.01.030
19. Gersh BJ, Maron BJ, Bonow RO, Dearani JA, Fifer MA, Link MS, et al. 2011 ACCF/AHA guideline for the diagnosis and treatment of hypertrophic cardiomyopathy: executive summary: a report of the American college of cardiology foundation/American heart association task force on practice guidelines. Circulation. (2011) 124(24):2761–96. doi: 10.1161/CIR.0b013e318223e230
20. Gebhard C, Stähli BE, Greutmann M, Biaggi P, Jenni R, Tanner FC. Reduced left ventricular compacta thickness: a novel echocardiographic criterion for non-compaction cardiomyopathy. J Am Soc Echocardiogr. (2012) 25(10):1050–7. doi: 10.1016/j.echo.2012.07.003
21. Lopez L, Colan S, Stylianou M, Granger S, Trachtenberg F, Frommelt P, et al. Relationship of echocardiographic Z scores adjusted for body surface area to age, sex, race, and ethnicity: the pediatric heart network normal echocardiogram database. Circ Cardiovasc Imaging. (2017) 10(11):e006979. doi: 10.1161/CIRCIMAGING.117.006979
22. Marcus FI, McKenna WJ, Sherrill D, Basso C, Bauce B, Bluemke DA, et al. Diagnosis of arrhythmogenic right ventricular cardiomyopathy/dysplasia: proposed modification of the task force criteria. Eur Heart J. (2010) 31(7):806–14. doi: 10.1093/eurheartj/ehq025
23. Jean-St-Michel E, Meza JM, Maguire J, Coles J, McCrindle BW. Survival to stage II with ventricular dysfunction: secondary analysis of the single ventricle reconstruction trial. Pediatr Cardiol. (2018) 39(5):955–66. doi: 10.1007/s00246-018-1845-4
24. Isaac D, Chan M, Haddad H, Cheung A. Cardiac Transplantation: Eligibility and Listing Criteria in Canada.:13.
25. Wittekind SG, Ryan TD, Gao Z, Zafar F, Czosek RJ, Chin CW, et al. Contemporary outcomes of pediatric restrictive cardiomyopathy: a single-center experience. Pediatr Cardiol. (2019) 40(4):694–704. doi: 10.1007/s00246-018-2043-0
26. Su JA, Menteer J. Outcomes of Berlin heart EXCOR® pediatric ventricular assist device support in patients with restrictive and hypertrophic cardiomyopathy. Pediatr Transplant. (2017) 21(8):1–6. doi: 10.1111/petr.13048
27. Singh TP, Cherikh WS, Hsich E, Harhay MO, Hayes D Jr, Perch M, et al. International society for heart and lung transplantation. The international thoracic organ transplant registry of the international society for heart and lung transplantation: twenty-fifth pediatric heart transplantation report-2022; focus on infant heart transplantation. J Heart Lung Transplant. (2022) 41(10):1357–65. doi: 10.1016/j.healun.2022.07.019
28. Rossano JW, Cherikh WS, Chambers DC, Goldfarb S Jr, Khush HD KK, et al.. The international thoracic organ transplant registry of the international society for heart and lung transplantation: twenty-first pediatric heart transplantation report-2018; focus theme: multiorgan transplantation. J Heart Lung Transplant. (2018) 37(10):1184–95. doi: 10.1016/j.healun.2018.07.018
29. Alcorn JB. Changes to OPTN Bylaws and Policies from actions at June Board of Directors Meeting. Available at: https://optn.transplant.hrsa.gov/media/1822/optn_policy_notice_07-24-2014.pdf (Accessed January 10, 2019).
30. Merlo M, Cannatà A, Gobbo M, Stolfo D, Elliott PM, Sinagra G. Evolving concepts in dilated cardiomyopathy. Eur J Heart Fail. (2018) 20(2):228–39. doi: 10.1002/ejhf.1103
31. van Velzen HG, Schinkel AFL, Baart SJ, Oldenburg RA, Frohn-Mulder IME, van Slegtenhorst MA, et al. Outcomes of contemporary family screening in hypertrophic cardiomyopathy. Circ Genom Precis Med. (2018) 11(4):e001896. doi: 10.1161/CIRCGEN.117.001896
32. Ommen SR, Mital S, Burke MA, Day SM, Deswal A, Elliott P, et al. 2020 AHA/ACC guideline for the diagnosis and treatment of patients with hypertrophic cardiomyopathy. Circulation. (2020) 142(25):e558–631. doi: 10.1161/CIR.0000000000000937
33. McNally EM, Mestroni L. Dilated cardiomyopathy. Circ Res. (2017) 121(7):731–48. doi: 10.1161/CIRCRESAHA.116.309396
34. Parker LE, Landstrom AP. The clinical utility of pediatric cardiomyopathy genetic testing: from diagnosis to a precision medicine-based approach to care. Prog Pediatr Cardiol. (2021) 62:101413. doi: 10.1016/j.ppedcard.2021.101413
35. Chintanaphol M, Orgil BO, Alberson NR, Towbin JA, Purevjav E. Restrictive cardiomyopathy: from genetics and clinical overview to animal modeling. RCM. (2022) 23(3):108. doi: 10.31083/j.rcm2303108
36. Davies RR, Haldeman S, Pizarro C. Regional variation in survival before and after pediatric heart transplantation–an analysis of the UNOS database. Am J Transplant. (2013) 13(7):1817–29. doi: 10.1111/ajt.12259
37. Maskatia SA, Decker JA, Spinner JA, Kim JJ, Price JF, Jefferies JL, et al. Restrictive physiology is associated with poor outcomes in children with hypertrophic cardiomyopathy. Pediatr Cardiol. (2012) 33(1):141–9. doi: 10.1007/s00246-011-0106-6
Keywords: restrictive cardiomyopathy, symptoms, outcomes, heart transplant, transplant-free survival
Citation: Lorenzo M, Lynch A, Ashkanase J, Fazari L, George K, Arathoon K, Minn S, Nicolson D, Jeewa A and Jean-St-Michel E (2023) Symptomatic presentation influences outcomes in pediatric restrictive cardiomyopathy. Front. Pediatr. 11:1264751. doi: 10.3389/fped.2023.1264751
Received: 21 July 2023; Accepted: 19 September 2023;
Published: 19 October 2023.
Edited by:
Inga Voges, University Medical Center Schleswig-Holstein, GermanyReviewed by:
Keiichi Hirono, University of Toyama, JapanFabrizio De Rita, Newcastle upon Tyne Hospitals NHS Foundation Trust, United Kingdom
© 2023 Lorenzo, Lynch, Ashkanase, Fazari, George, Arathoon, Minn, Nicolson, Jeewa and Jean-St-Michel. This is an open-access article distributed under the terms of the Creative Commons Attribution License (CC BY). The use, distribution or reproduction in other forums is permitted, provided the original author(s) and the copyright owner(s) are credited and that the original publication in this journal is cited, in accordance with accepted academic practice. No use, distribution or reproduction is permitted which does not comply with these terms.
*Correspondence: Melissa Lorenzo bWVsaXNzYS5sb3JlbnpvQHNpY2traWRzLmNh