- 1Pharmacy Department, Women’s Wellness and Research Center, Hamad Medical Corporation, Doha, Qatar
- 2Neonatal Intensive Care Unit, Women’s Wellness and Research Center, Hamad Medical Corporation, Doha, Qatar
- 3Pediatric Department, Children’s Hospital of Eastern Ontario and Ottawa Hospital, University of Ottawa, Ottawa, ON, Canada
- 4Pediatric Department, Weill Cornell Medicine-Qatar, Doha, Qatar
Introduction: Determining the optimal dexamethasone dosage for facilitating extubation in extremely low birth weight (ELBW) infants with bronchopulmonary dysplasia (BPD) remains uncertain. This study aims to compare the effectiveness of low-dose (DART) and enhanced low-dose dexamethasone regimens in achieving successful extubation in these infants.
Methods: We conducted a retrospective cohort study at the Women's Wellness and Research Center (WWRC) involving ELBW infants who received dexamethasone for BPD prevention or treatment, or for extubation between January 1st, 2015, and December 31st, 2019. Our goal was to assess successful extubation within various time points of treatement.
Results: A total of 77 patients, matched in gestational age and BW, were enrolled in the study, receiving a total of 121 dexamethasone courses. Low-dose dexamethasone courses were administered 75 times to 49 infants, while 46 courses of enhanced low-dose were given to 28 infants. Treatment commenced at 30.8 ± 3.4 weeks post-menstrual age, compared to 32.1 ± 2.5 weeks in the enhanced low-dose group (p = 0.014). The median (IQR) course duration was seven (3–10) days in the low-dose group, while it was 10 (8–14) days in the enhanced low-dose group (p < 0.001). The median (IQR) course dose was 0.73 (0.53–0.86) mg/kg in the low-dose group and 1.27 (0.97–2.05) mg/kg in the enhanced low-dose group (p < 0.001). There were no differences in extubation success at any time point between the two groups at 72 h and seven days after treatment initiation, by course completion, and within seven days after treatment completion. However, regression analysis identified several predictors of successful extubation; baseline FiO2, course duration, and duration of invasive mechanical ventilation were negatively associated with successful extubation at various time points, while received dose per kg and cumulative dose positively correlated with successful extubation at different time points. No significant differences were observed in secondary outcomes, including death or BPD.
Conclusion: The choice between low-dose and enhanced low-dose dexamethasone regimens may not significantly impact extubation success. However, careful consideration of dosing, ventilation status, and treatment duration remains crucial in achieving successful extubation. This study highlights the need for personalized dexamethasone therapy in ELBW infants.
Introduction
Bronchopulmonary dysplasia (BPD) is a common neonatal respiratory disease affecting preterm neonates, particularly those who are extremely low birth weight (ELBW) (birth weight less than 1,000 g) (1–4). BPD is a significant cause of morbidity and mortality in this population, with prominent outcomes including cardiovascular and neurodevelopmental delay (5–7). Several factors contribute to BPD in these infants, including perinatal inflammation, sepsis, necrotizing enterocolitis (NEC), and the need for mechanical ventilation and oxygen supplementation (6, 7). These conditions collectively promote damage and inflammation in the alveoli and airways, resulting in abnormal lung development and BPD onset (4). Despite neonatal care advancements, BPD prevalence remains high, and previous therapies primarily focused on mitigating its effects rather than prevention or cure.
In recent years, studies have explored therapies aimed at reducing the BPD prevalence in preterm infants and improving their short-term and long-term outcomes (3–5). Among these therapies, postnatal steroids (PNS) have gained significant attention for their ability to reduce lung inflammation (8–14). PNS achieve this by suppressing lymphocytes and enhancing the effects of anti-inflammatory cytokines (9). PNS therapy is commonly used to treat and prevent BPD and to facilitate extubation from mechanical ventilation (12, 13). However, their use in neonates, especially preterm infants, is associated with potential adverse effects, including impaired growth and development, an increased risk of infection, and neurodevelopmental problems (2, 14–17, 18).
Studies have assessed the efficacy and safety of PNS, particularly dexamethasone, with various doses or durations in neonates (19–22). The most commonly studied protocol is the DART (Dexamethasone: A Randomized Trial) protocol, which involves a lower dose (total 0.89 mg/kg) over 10 days for the treatment of BPD in preterm infants (23). Despite its long-standing use, BPD remains a significant cause of neonatal morbidity. In recent years, clinicians have started to explore different dosing regimens and durations for dexamethasone to evaluate potential benefits (20, 21, 24).
The efficacy and safety of low-dose dexamethasone (0.08 mg/kg/day for seven days) compared to high dose (0.5 mg/kg/day for three days followed by 0.3 mg/kg/day for four days) was evaluated in a study involving preterm neonates with BPD (25). The study found that low-dose dexamethasone therapy was associated with lower incidence of adverse effects, including growth failure and hypertension, compared to conventional dexamethasone therapy with similar pulmonary outcomes. Another study used early dexamethasone therapy at a higher overall dose (0.5 mg/kg/day for seven days) vs. placebo (0.9% normal saline) (21). This study found that dexamethasone improved short-term pulmonary outcome compared to placebo, with no reported serious adverse effects in either group.
These findings underscore the variability in current research regarding the optimal duration and dose for dexamethasone. The American Academy of Pediatrics (AAP) state that doses ≥0.5 mg/kg are associated with adverse outcomes. However, insufficient data is available on the potential benefits and risks of lower doses of dexamethasone in neonates (26).
Overall, studies suggest that the optimal dexamethasone regimen is unclear. However, doses higher than those recommended in the low-dose DART protocol show promise in effectively reducing BPD without significant differences in adverse events. Neonatologists often favor the DART regimen due to the lack of established evidence for alternative dexamethasone dose regimens.
The objective of this study is to conduct a retrospective analysis to investigate the clinical efficacy of low-dose dexamethasone dose (DART protocol) compared to enhanced low-dose dexamethasone regimens in facilitating extubation, and to examine their effects on significant neonatal outcomes in ELBW infants with evolving or established BPD.
Materials and methods
Study population
This retrospective cohort study was conducted at the Women's Wellness and Research Center (WWRC) (former Women's Hospital) in Doha, Qatar. The study included ELBW infants who received dexamethasone for the prevention or treatment of BPD. The study period lasted from January 2015 to December 2019.
We identified ELBW infants who were intubated for more than two weeks thus qualifying for PNS dexamethasone treatment per unit protocol. Pharmacy records were reviewed to identify those infants who were administered the dexamethasone regimen, as prescribed by the attending physician's discretion. We categorized infants into two groups based on the dexamethasone dosage they received. The first group received a low dose as per the DART protocol, which amounted to a cumulative dose of 0.89 mg/kg spread over 10 days. The second group received a cumulative dose exceeding 0.89 mg/kg, which is referred to as the “enhanced low-dose dexamethasone”. To determine the doses of dexamethasone received, we considered the actual doses administered, regardless of the ordered dose. Dosing weight was based on the weight recorded at the time of prescription.
We identified a total of 94 infants as receiving dexamethasone; however, 17 infants were excluded from the study. The exclusion criteria were as follows: 12 infants had higher gestational age and birth weight (BW), three infants had chromosomal anomalies, one infant received steroids during subglottic correction surgery, and one infant had congenital surfactant deficiency (Figure 1). Infants who received hydrocortisone were also excluded from the study.
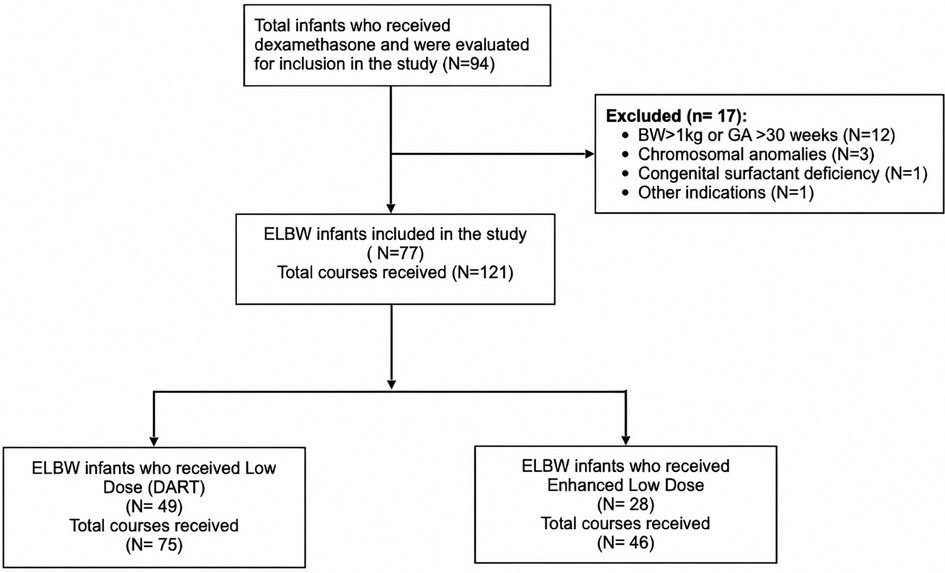
Figure 1. Flow diagram of inclusion criteria and dexamethasone dose regimens. BW, birth weight; GA, gestational age; ELBW, extremely low birth weight. DART, dexamethasone; a randomized trial.
This study was approved and received ethical approval by the WWRC Medical Research Center (MRC)/Institutional Review Board (IRB) under protocol number MRC-01-19-301. The study did not receive any external funding, and informed consent was waived due to its retrospective nature. As the largest tertiary care center in Qatar, WWRC recorded 70,000 births during the study period, with 700 ELBW infants admitted to the neonatal intensive care unit (NICU). Dexamethasone was administered in our practice to infants with evolving or established BPD to facilitate extubation, based on the discretion of the neonatologist regarding the timing of initiation and dosage. The utilization of other systemic PNS such as hydrocortisone was rarely employed in our unit during the study period except as a vasopressor for hypotension.
Data collection
The main objective of this study was to assess the achievement of successful extubation in ELBW with either evolving or established BPD after administering dexamethasone treatment. In this study, we employed Jensen's definition of BPD (1). Data on successful extubation were collected within 72 h and seven days after treatment initiation, by course completion, and within seven days after treatment completion. Secondary objectives were to evaluate for significant neonatal outcomes in the NICU, which included respiratory outcomes, death, and other significant neonatal morbidities. Neonatal data included gestational age (GA), gender, BW, small for gestational age (SGA), ethnicity, APGAR scores, and discharge weight. Respiratory characteristics of the study infants included surfactant administration, mechanical ventilation for 28 days, mode of ventilation at 36 weeks post-menstrual age (PMA), total invasive and noninvasive ventilation days, and mean fraction of inspired oxygen (FiO2). Physicians collected obstetric data by reviewing mothers’ charts for clinical and demographic information, as well as pharmacological information from the pharmacy database. Maternal data included demographics, ethnicity, antenatal steroids administered, mode of delivery, preterm premature rupture of membranes (PPROM), oligohydramnios, chorioamnionitis, GBS status, and illness (maternal hypertension and gestational diabetes). Information pertaining to the course encompassed details such as the examethasone dose administered per kilogram, the total number of courses undertaken, the day of life at which the course was initiated, the duration of the course, and the cumulative dose administered. This comprehensive dataset was complemented by an analysis of respiratory parameters both before and after the initiation of each course. Ventilator-associated pneumonia (VAP), sepsis, necrotizing enterocolitis (NEC), and intestinal perforation that resulted in interruption or treatment of the medication were recorded. A secondary medical outcomes included VAP, sepsis, NEC, retinopathy of prematurity (ROP), intraventricular hemorrhage (IVH), periventricular leukomalacia (PVL), neurodevelopment impairment (NDI), grade of BPD, death, and death or BPD. In our study, neurodevelopmental assessments were conducted on infants aged between 18 and 24 months’ corrected age using both the Bayley Infant Neurodevelopmental Screener (BINS) and additional neurologic examinations, including the Alberta Infant Motor Scale (AIMS). NDI in our study was specifically defined as any level of risk identified by the BINS and AIMS across its four domains, which encompass neurologic integrity including motor, cognitive, and language deficits.
Statistical analysis
Descriptive analyses were conducted to evaluate patient characteristics and clinical variables. Chi-square or Fisher's exact test were employed for binary variables to analyze differences between groups (low-dose and enhanced low-dose dexamethasone). Non-parametric continuous variables were analyzed using the Mann–Whitney U-test. Mean and standard deviation (SD) or median and interquartile range (IQR) were used to present continuous variables, while numbers and percentages were used for categorical variables. Stepwise logistic regression (backward) was conducted to examine the associations between various exposures and successful extubation at different time points after controlling for potential confounders. The multivariable model included all significant variables associated with successful extubation identified in the univariate analyses with a significance of 0.1. All p-values were two-tailed, and values below 0.05 were considered statistically significant. Statistical analyses were performed using SPSS for Windows (version 29.0, IBM Corp., Armonk, NY, USA).
Results
We evaluated 94 children who received dexamethasone treatment in the NICU for inclusion in the study. After excluding 17 infants, the study included 77 patients who received a total of 121 doses of dexamethasone (Figure 1). The characteristics of study subjects are summarized in Table 1. The study population was divided between those who received low-dose dexamethasone (n = 49) vs. enhanced low- dose dexamethasone (n = 28). The GA of neonates in the low-dose group was 24.9 ± 1.6 weeks vs. 25.3 ± 1.2 weeks in the enhanced low-dose group. The BW of neonates in both groups was respectively 748 ± 114 vs. 765 ± 124 g. Majority of patients were male in both groups (65% vs. 71%; p = 0.581). There were various ethnicities amongst the groups such as African (6.1% vs. 10.7%) and European (8.3% vs. 4.6%) without any visible differences. However, Asian population was much higher in the enhanced low-dose group 42.9% vs. low-dose 22.4%, while Middle Eastern ethnicity was higher in the low-dose group 63.3% vs. 42.9%. Difference in APGAR scores at 1 and 5 min showed no statistical significance between the groups. Discharge weight was significantly higher in the enhanced low-dose dexamethasone group compared to low-dose group (4,080 vs. 3,305 g; p = 0.048).
Maternal characteristics between the groups were further analyzed in Table 1 with a median age of mothers being 29.1 ± 6.6 in the low-dose group vs. 31.2 ± 7.2 enhanced low-dose group. Multiple gestations were much higher in the low-dose group 34.7% vs. 14.3% in the enhanced low-dose group, while not significant p = 0.053. Medical conditions during pregnancy were also analyzed including maternal hypertension (12.2% vs. 21.4% p = 0.311), gestational diabetes (22.5% vs. 21.4%; p = 0.999) and positive GBS screening (10.6% vs. 21.4%; p = 0.2), with no statistical significance between the groups. Other maternal factors examined were chorioamnionitis, PPROM, and oligohydramnios, did not show significant difference. The majority of mothers in both groups received at least one dose of antenatal steroids, with similar percentages in both groups, 91.7% vs. 96.4%. Additionally, nearly two-thirds of mothers received 2 doses of antenatal steroids in each group.
The respiratory characteristics of infants that received dexamethasone regimens were analyzed between the groups in Table 2. No statistical significance was found between the number of surfactant doses received whether none, one or ≥ two used between the two groups (p-value = 0.156). Receiving mechanical ventilation was assessed at 28 days between the low-dose and enhanced low-dose (83.7% vs. 92.8%; p = 0.312) and at 36 weeks PMA (22.5 vs. 22.2; p = 0.859), showed no statistical significance. No statistical significance was also observed between median invasive ventilation days (p = 0.787). However, the low-dose group received more non-invasive ventilation days compared to the enhanced low-dose group (40 vs. 60; p-value = 0.026). Additionally, infants who received dexamethasone therapy in the first 28 days were 37.4% vs. 25%; p = 0.377. Other respiratory characteristics analyzed were median FiO2 at 28 days and at 36 weeks (40 vs. 57; p-value = 0.210) and (25 vs. 29; p-value = 0.08), respectively. The proportions of infants who received repeated courses of dexamethasone (38.8% vs. 39.3%; p-value = 0.965), showed no differences between the two groups.
Results of respiratory outcomes associated with different dexamethasone regimens are summarized in Table 3. The low-dose group were administered 75 courses vs. 46 in the enhanced low-dose group. The dosing weight for all courses was 1,696 ± 1,174 g in the low-dose group vs. 1,907 ± 1,280 g in the enhanced low-dose group p = 0.244. The dosing weight in grams at the start of the first dose was also evaluated and showed no significant differences between the groups p = 0.154. Day of life in terms of all courses and first course were analyzed and showed no significance, p = 0.571 and p = 0.603, respectively. Assessment of PMA in weeks at the start of all doses showed no significant difference between the groups (33.7 ± 6.7 vs. 35.3 ± 6.6; p = 0.112). However, PMA at the first course was found to be significant amongst the groups (30.8 ± 3.4 vs. 32.1 ± 2.5; p = 0.014). The median course dose per kg (mg) received was found to be higher in the enhanced low-dose group (0.73 vs. 1.27; p = <0.001). Also, evaluation of the median course duration (in days) between the groups was lower in the low-dose group (7 vs. 10. days p = <0.001). Statistical significance was observed in the course cumulative doses (mg) between the groups, with the enhanced low-dose group having a higher median dose (0.79 vs. 1.65; p = 0.001), and a higher total cumulative doses (mg) received throughout the NICU stay (1.00 vs. 1.99 (p < 0.001).
Other parameters assessed in Table 3 included the median baseline FiO2 (%) before dexamethasone course and it was significant between the groups (45 vs. 63; p = 0.042). On the other hand, median FiO2 (%) after dexamethasone course showed no significance (35 vs. 40; p = 0.527). The vast majority (>90%) of patients in each group were on invasive ventilation, and there was no difference between the groups (p = 0.124). The proportions of infants extubated at different time points from dexamethasone treatment initiation were assessed between the low-dose and enhanced low-dose groups. There were no significant differences between the groups in extubation rates within 72 h (25% vs. 22.2%; p = 0.735) or following seven days from initiating therapy (41.2% vs. 31.1%; p = 0.278). Further analysis of potential extubation either at the end of treatment course or seven days post treatment showed no statistical difference between the groups (45.6% vs. 53.3%; p = 0.420 and (54.4% vs. 64.4%; p = 0.389). Reduction in respiratory support of any kind was not significant between the groups, p = 0.316. Infants who received enhanced low-dose were extubated at a mean age of 63 vs. 49 days of life compared to infants who received a lower dose of dexamethasone; however, this finding was not statistically significant (p = 0.174). Both the low-dose and enhanced low-dose groups had a high but non-significant incidence of VAP during treatment (21.3% vs. 30.4%; p = 0.260) and sepsis (13.3% vs. 8.7%; p = 0.439), while the presence of NEC was low (1.3% vs. 0.0%; p = 0.999), respectively.
Secondary outcomes associated with dexamethasone regimen usage were also analyzed throughout the study and are summarized in Table 4. Several neonatal-specific factors were assessed between the low-dose and enhanced low-dose groups, including VAP (61% vs. 61%; p = 0.965), sepsis (45% vs. 50%; p = 0.666), NEC (18.4% vs. 7.1%; p = 0.310), any ROP (71.4% vs. 74%; p = 0.805), severe ROP (40.8% vs. 37%; p = 0.747), treated ROP (8.8% vs. 19%; p = 0.408), medically treated PDA (53% vs. 53.6%; p = 0.966), with only two infants in each group requiring device closure of the PDA, and moderate and severe BPD (85.4% vs. 100%; p = 0.074). Additional analysis of long-term outcomes such as NDI showed no significant difference between the groups (30.7% vs. 29.1%; p = 0.893). There were 13 (16.9%) death cases during the NICU hospitalizations (22.4% vs. 7.1%; p = 0.117), with a median day of death of 92 vs. 145 days, p = 0.194 in the low-dose and enhanced low-dose groups. Infants who died before and after 36-weeks PMA were four and seven in the low-dose group and one and one in the enhanced low-dose group. Additionally, the incidence of death or severe BPD was 30.6% vs. 25%; p = 0.6 between the two groups.
Univariate and stepwise multivariate regression analyses were conducted to identify significant variables associated with successful extubation at various time points: 72 h post dexamethasone treatment, within seven days of treatment initiation, by the end of dexamethasone treatment, and within seven days of dexamethasone treatment completion, as summarized in Table 5.
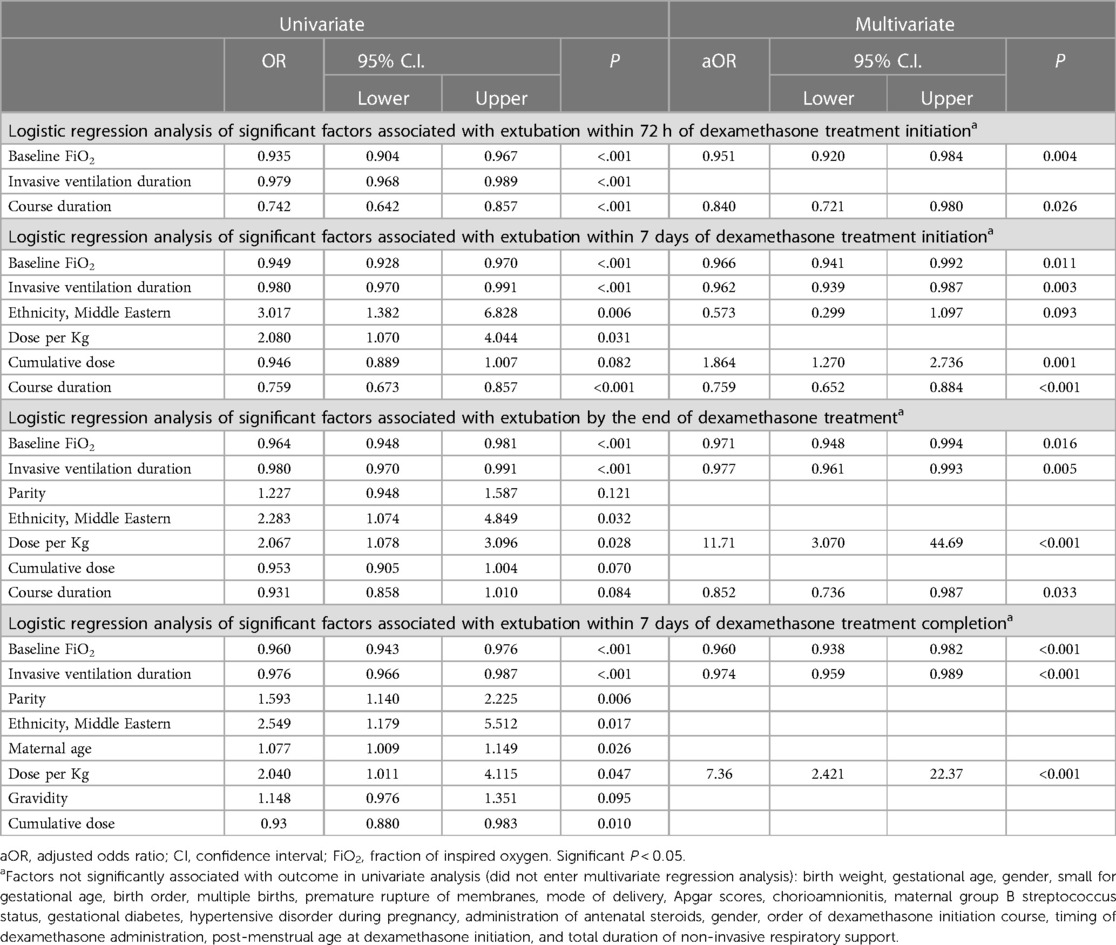
Table 5. Regression analysis of variables associated with successful extubation at various time points following initiation of dexamethasone treatment.
Among the demographic and clinical variables examined, the following predictors were found to be associated with successful extubation at 72 h post dexamethasone treatment initiation in the univariate regression model: baseline FiO2, duration of invasive ventilation, and course duration. However, in the multivariate analysis, only baseline FiO2 (adjusted odds ratio [aOR]: 0.951, 95% confidence interval [CI]: 0.920–0.984, p = 0.004) and course duration (aOR: 0.840, CI: 0.721–0.980, p = 0.026) remained significantly associated with successful extubation at this time point.
Similarly, for successful extubation at seven days post treatment initiation, the univariate regression model identified the following predictors: baseline FiO2, duration of invasive ventilation, ethnicity, dose per kg, cumulative dose, and course duration. In the multivariate analysis, baseline FiO2 (aOR: 0.966, CI: 0.941–0.992, p = 0.011), duration of invasive ventilation (aOR: 0.962, CI: 0.939–0.987, p = 0.003), cumulative dose (aOR: 1.864, CI: 1.270–2.736, p = 0.001), and course duration (aOR: 0.759, CI: 0.652–0.884, p < 0.001) remained significant.
Additionally, regarding extubation by the end of the dexamethasone course treatment, out of the significant predictors found in the univariate regression analysis (baseline FiO2, duration of invasive ventilation, parity, ethnicity, dose per kg, cumulative dose, and course duration), only baseline FiO2 (aOR:0.971, CI: 0.948–0.994, p = 0.016), dose per kg (aOR:11.71, CI: 3.070–44.691, p < 0.001), duration of invasive ventilation (aOR:0.977, CI: 0.961–0.993, p = 0.005), and course duration (aOR:0.852, CI: 0.736–0.987, p = 0.033) remained significant in the multivariate analysis.
Finally, the regression analysis within seven days of completing dexamethasone treatment showed a significant association in the univariate analysis for several factors, including baseline FiO2, duration of invasive ventilation, parity, ethnicity, maternal age, dose per kg, gravidity, and cumulative dose. From these predictors, baseline FiO2 (aOR:0.960, CI: 0.93–0.982, p < 0.0001), duration of invasive ventilation (aOR:0.974, CI: 0.95–0.989, p < 0.001), and dose per kg (aOR:7.361, CI: 2.42–22.37, p < 0.001) remained significant in the multivariate analysis.
Discussion
BPD remains a challenging respiratory outcome among ELBW neonates in the NICU (1–4). The administration of PNS can be considered as a crucial therapy for these neonates to improve parenchymal lung disease and facilitate extubation (3–6, 8–10). Although DART protocol is the mainstay therapy in our unit, higher dose regimens such as enhanced low-dose dexamethasone, are currently used on a case-by-case basis by neonatologists. This practice aligns with the common approach of utilizing this dose of dexamethasone (DART protocol) for evolving or established BPD suggested by Doyle LW et al. (23). The same author conducted a meta-analysis of 23 RCTs on the use of systemic PNS for the prevention of BPD in preterm neonates (27). Their analysis revealed that two-thirds of the studies included in the meta-analysis utilized dexamethasone (n = 21) for BPD prevention.
Neonates in both groups showed similar neonatal-specific characteristics. However, it was observed that neonates receiving the enhanced low-dose dexamethasone regimen had a higher weight at the time of hospital discharge, required higher FiO2, and remained longer on non-invasive respiratory support. Furthermore, they were extubated later compared to infants who received a lower dose of dexamethasone, although this latter finding was not statistically significant. These observations suggest a more severe manifestation of BPD. Thus, providing a potential explanation for the increased utilization of the enhanced low-dose dexamethasone regimen by attending neonatologists in this group. According to our study, the enhanced low-dose dexamethasone regimen resulted in a significantly longer duration of therapy and a higher cumulative dose compared to the low-dose dexamethasone protocol. This was expected as the enhanced low-dose regimen involves a higher overall dose and/or a longer duration of therapy. Bonnie L Marr et al. conducted a randomized controlled trial (RCT) in which they compared a 42-day course of dexamethasone to a nine-day course for the treatment of BPD in extremely preterm neonates (28). The study found that the 42-day group had a significantly higher overall dose of dexamethasone compared to the 9-day group (P < 0.001). These findings are expected with all studies that utilize a higher dexamethasone dose than the standard DART protocol dose.
Our study findings showed no difference between the groups in terms of development of any adverse outcomes including NDI at 18–24 months. These findings suggest that the safety profile of dexamethasone at an enhanced low-dose is comparable to that of the low-dose (DART protocol). A meta-analysis by Ramaswamy et al. assessed the safety of 14 different PNS regimens used for the prevention of BPD in neonates and secondary outcomes included NDI (18–24 months) (29). It was found that moderately early (2–4 mg/kg) of dexamethasone was potentially the most appropriate regimen for preventing BPD. Also, none of the interventions were associated with an increased or decreased risk of NDI. Linan Zeng et al., conducted a meta-analysis which included 47 RCTs with 6,747 participants and focused on the efficacy and safety of high dose dexamethasone (>3 mg/kg) compared to other corticosteroids for prevention of BPD in preterm infants (30). The meta-analyses concluded that higher-doses are effective, but not without the potential increased risk of adverse neurodevelopment effects. A systematic review conducted in 2017 by Onland W. et al., which compared different corticosteroid regimens on mortality and neurodevelopmental outcomes (31). In a subgroup analysis of the study, it was concluded that there were no differences between high and low-dose regimens on death or any abnormal neurodevelopmental outcomes. Other studies have suggested that the use of dexamethasone at various high doses could potentially be harmful for overall brain development (32, 33). In our study, enhanced low-dose dexamethasone, which is considered a lower cumulative dose compared to those referred to as “moderate to high” in the previously mentioned studies carries a considerably less risk for NDI at 18–24 months as suggested by the results. However, a longer-term follow-up beyond two years on NDI is warranted to further confirm the findings of our study.
Our study did not show that an enhanced low-dose dexamethasone is associated with a higher rate of extubation at various time points. Nonetheless, regression analysis showed that a higher dose per kilogram or higher cumulative dose with a shorter duration are associated with successful extubation. These findings are supported by recommendations by Zeng et al, who suggested aggressive initiation seems more effective (30). However, higher doses for longer durations are not recommended based on safety. Moreover, the regression analysis also showed that enhanced low dose dexamethasone dose significantly correlated with higher chances of neonatal extubation within different time frames of receiving dexamethasone course. This is an essential finding as it further sheds light on the potential importance of increasing dexamethasone dosing within ranges that are still considered to be safe (34). Studies have found similar findings where moderately high doses of dexamethasone were associated with a significant improvement in FiO2 (30, 35, 36). Despite the higher extubation rates associated with the enhanced low dose in our study, no differences were found between the rates of BPD outcomes between the groups.
Similarly, death was not clinically significant although higher in the low dose dexamethasone group. These findings are supported by a meta-analysis and other studies which showed no difference of composite death between various dexamethasone regimens (26, 37, 38).
This study has several strengths as it is the first of its kind to be conducted in Qatar. It also provides valuable insight on the use of dexamethasone in ELBW neonates with BPD as it compares two different dose regimens low dose (DART) and enhanced low-dose. The study focused on a vulnerable and specific population, ELBW neonates, for whom the optimal recommended dose of dexamethasone is still in question. In addition, matching was conducted to help control for potential confounding factors by matching patients in terms of birth weight and gestational age. This enhances the validity of the comparison between the groups. Regression analysis was used to help identify any predictors of successful extubation. This statistical approach adds depth to the findings by highlighting factors that influence extubation outcomes in ELBW infants. The consideration of secondary outcomes, such as death or BPD, provides a comprehensive view of the safety and efficacy of the treatments. Despite its strengths this study contained limitations such as its retrospective nature which has inherent biases with data collection and dependence on existing medical records. Recall bias also may potentially impact the accuracy of the data recorded. Although the study attempted to control for potential confounding factors however, some variables which were not accounted for could potentially influence the extubation outcomes. In addition, the study may be underpowered, due to the small sample size, to fully form conclusions on safety and efficacy outcomes. The need for further research to evaluate the findings of this study with larger sample sizes and longer follow-up data are warranted to help draw more clear conclusions.
Conclusion
Our findings showed no significant differences in extubation success rates between the two regimens at various time points following treatment initiation. However, we identified important predictors of successful extubation, including baseline FiO2, course duration, and duration of invasive mechanical ventilation, which negatively impacted extubation success. Conversely, received dose per kilogram and cumulative dose positively correlated with successful extubation at different time points. These results underscore the need for a personalized approach to dexamethasone therapy in ELBW infants with BPD, considering individual patient characteristics and clinical factors.
Data availability statement
The raw data supporting the conclusions of this article will be made available by the authors, without undue reservation.
Ethics statement
The studies involving humans were approved by Medical Research Center Hamad Medical Corporation Doha, Qatar. The studies were conducted in accordance with the local legislation and institutional requirements. Written informed consent for participation was not required from the participants or the participants’ legal guardians/next of kin because The study was retrospective in nature and thus does not require informed consent.
Author contributions
HA: Data curation, Methodology, Writing – original draft, Writing – review & editing. IA: Data curation and Manuscript review. NI: Methodology, Project administration, Supervision, Validation, Visualization and Manuscript review. FK: Data curation and Manuscript review. AK: Data curation and Manuscript review. ST: Data curation and Manuscript review. MB: Project administration, Supervision and Manuscript submission. AG: Conceptualization, Data curation, Formal Analysis, Funding acquisition, Methodology, Project administration, Software, Supervision, Validation, Visualization, Writing – original draft and Writing – review & editing.
Funding
The author(s) declare financial support was received for the research, authorship, and/or publication of this article.
Study funding was provided by MRC of Hamad Medical Corporation (HMC). The funder had no involvement in the study design, data collection and analysis, decision to publish, or preparation of the manuscript.
Conflict of interest
All the authors are employed by Hamad Medical Corporation. The authors declare that the research was conducted in the absence of any commercial or financial relationships that could be construed as a potential conflict of interest.
Publisher's note
All claims expressed in this article are solely those of the authors and do not necessarily represent those of their affiliated organizations, or those of the publisher, the editors and the reviewers. Any product that may be evaluated in this article, or claim that may be made by its manufacturer, is not guaranteed or endorsed by the publisher.
References
1. Jensen EA, Dysart K, Gantz MG, McDonald S, Bamat NA, Keszler M, et al. The diagnosis of bronchopulmonary dysplasia in very preterm infants. An evidence-based approach. Am J Respir Crit Care Med. (2019) 200(6):751–9. doi: 10.1164/rccm.201812-2348OC
2. Walsh MC, Morris BH, Wrage LA, Vohr BR, Poole WK, Tyson JE, et al. Extremely low birthweight neonates with protracted ventilation: mortality and 18-month neurodevelopmental outcomes. J Pediatr. (2005) 146(6):798–804. doi: 10.1016/j.jpeds.2005.01.047
3. Doyle LW, Cheong JL. Postnatal corticosteroids to prevent or treat bronchopulmonary dysplasia–who might benefit? Semin Fetal Neonatal Med. (2017) 22(5):290–5. doi: 10.1016/j.siny.2017.07.003
4. Olaloko O, Mohammed R, Ojha U. Evaluating the use of corticosteroids in preventing and treating bronchopulmonary dysplasia in preterm neonates. Int J Gen Med. (2018) 11:265–74. doi: 10.2147/IJGM.S158184
5. Cuna A, Quiqley A, Varghese K, Ciccolari-Micaldi G, Oliveros C, Cheng AL, et al. Effectiveness and safety of repeat dexamethasone for bronchopulmonary dysplasia. J Perinatol. (2021) 41(8):1956–62. doi: 10.1038/s41372-021-01125-3
6. Htun ZT, Schulz EV, Desai RK, Marasch JL, McPherson CC, Mastrandrea LD, et al. Postnatal steroid management in preterm infants with evolving bronchopulmonary dysplasia. J Perinatol. (2021) 41(8):1783–96. doi: 10.1038/s41372-021-01083-w
7. Ryan RM, Ahmed Q, Lakshminrusimha S. Inflammatory mediators in the immunobiology of bronchopulmonary dysplasia. Clin Rev Allergy Immunol. (2008) 34:174–90. doi: 10.1007/s12016-007-8031-4
8. DeCastro M, El-Khoury N, Parton L, Ballabh P, LaGamma EF. Postnatal betamethasone vs dexamethasone in premature infants with bronchopulmonary dysplasia: a pilot study. J Perinatol. (2009) 29(4):297–304. doi: 10.1038/jp.2008.194
9. Baud O, Maury L, Lebail F, Ramful D, El Moussawi F, Nicaise C, et al. Effect of early low-dose hydrocortisone on survival without bronchopulmonary dysplasia in extremely preterm infants (PREMILOC): a double-blind, placebo-controlled, multicentre, randomised trial. Lancet. (2016) 387(10030):1827–36. doi: 10.1016/S0140-6736(16)00202-6
10. Lemyre B, Dunn M, Thebaud B. Postnatal corticosteroids to prevent or treat bronchopulmonary dysplasia in preterm infants. Paediatr Child Health. (2020) 25(5):322–6. doi: 10.1093/pch/pxaa073
11. Eichenwald EC, Stark AR. Are postnatal steroids ever justified to treat severe bronchopulmonary dysplasia? Arch Dis Child Fetal Neonatal Ed. (2007) 92(5):F334–7. doi: 10.1136/adc.2006.106583
12. Halliday HL. Update on postnatal steroids. Neonatology. (2017) 111(4):415–22. doi: 10.1159/000458460
13. Jobe AH. Postnatal corticosteroids for bronchopulmonary dysplasia. Clin Perinatol. (2009) 36(1):177–88. doi: 10.1016/j.clp.2008.09.016
14. Gupta S, Prasanth K, Chen CM, Yeh TF. Postnatal corticosteroids for prevention and treatment of chronic lung disease in the preterm newborn. Int J Pediatr. (2012) 2012. doi: 10.1155/2012/315642
15. Delara M, Chauhan BF, Le ML, Abou-Setta AM, Zarychanski R, W’tJong G. Efficacy and safety of pulmonary application of corticosteroids in preterm infants with respiratory distress syndrome: a systematic review and meta-analysis. Archives of Disease in Childhood-Fetal and Neonatal Edition. (2019) 104(2):F137–44. doi: 10.1136/archdischild-2017-314046
16. Doyle LW, Ehrenkranz RA, Halliday HL. Early (<8 days) postnatal corticosteroids for preventing chronic lung disease in preterm infants. Cochrane Database Syst Rev. (2014) (5):CD001146. doi: 10.1002/14651858.CD001146.pub4
17. Abman SH, Collaco JM, Shepherd EG, Keszler M, Cuevas-Guaman M, Welty SE, et al. Interdisciplinary care of children with severe bronchopulmonary dysplasia. J Pediatr. (2017) 181:12–28. doi: 10.1016/j.jpeds.2016.10.082
18. Cuna A, Govindarajan S, Oschman A, Dai H, Brophy K, Norberg M, et al. A comparison of 7-day versus 10-day course of low-dose dexamethasone for chronically ventilated preterm infants. J Perinatol. (2017) 37(3):301–5. doi: 10.1038/jp.2016.215
19. Cuna A, Lewis T, Dai H, Nyp M, Truog WE. Timing of postnatal corticosteroid treatment for bronchopulmonary dysplasia and its effect on outcomes. Pediatr Pulmonol. (2019) 54(2):165–70. doi: 10.1002/ppul.24202
20. Garland JS, Alex CP, Pauly TH, Whitehead VL, Brand J, Winston JF, et al. A three-day course of dexamethasone therapy to prevent chronic lung disease in ventilated neonates: a randomized trial. Pediatrics. (1999) 104(1):91–9. doi: 10.1542/peds.104.1.91
21. Kari MA, Heinonen K, Ikonen RS, Koivisto M, Raivio KO. Dexamethasone treatment in preterm infants at risk for bronchopulmonary dysplasia. Arch Dis Child. (1993) 68(5 Spec No):566–9. doi: 10.1136/adc.68.5_Spec_No.566
22. Shinwell ES, Karplus M, Zmora E, Reich D, Rothschild A, Blazer S, et al. Failure of early postnatal dexamethasone to prevent chronic lung disease in infants with respiratory distress syndrome. Arch Dis Child Fetal Neonatal Ed. (1996) 74(1):F33–7. doi: 10.1136/fn.74.1.F33
23. Doyle LW, Davis PG, Morley CJ, McPhee A, Carlin JB, Study Investigators DART. Low-dose dexamethasone facilitates extubation among chronically ventilator-dependent infants: a multicenter, international, randomized, controlled trial. Pediatrics. (2006) 117(1):75–83. doi: 10.1542/peds.2004-2843
24. Cummings JJ, Pramanik AK. Postnatal corticosteroids to prevent or treat chronic lung disease following preterm birth. Pediatrics. (2022) 149:e2022057530. doi: 10.1542/peds.2022-057530
25. Malloy CA, Hilal K, Rizvi Z, Weiss M, Muraskas JK. A prospective, randomized, double-masked trial comparing low dose to conventional dose dexamethasone in neonatal chronic lung disease. Internet Pediatr J Neonatol. (2005) 5(1):10473.
26. Onland W, De Jaegere AP, Offringa M, van Kaam AH. Effects of higher versus lower dexamethasone doses on pulmonary and neurodevelopmental sequelae in preterm infants at risk for chronic lung disease: a meta-analysis. Pediatrics. (2008) 122(1):92–101. doi: 10.1542/peds.2007-2258
27. Doyle LW, Cheong JL, Hay S, Manley BJ, Halliday HL. Late (≥7 days) systemic postnatal corticosteroids for prevention of bronchopulmonary dysplasia in preterm infants. Cochrane Database Syst Rev. (2021) 11. doi: 10.1002/14651858.CD001145.pub5
28. Marr BL, Mettelman BB, Bode MM, Gross SJ. Randomized trial of 42-day compared with 9-day courses of dexamethasone for the treatment of evolving bronchopulmonary dysplasia in extremely preterm infants. J Pediatr. (2019) 211:20–6. doi: 10.1016/j.jpeds.2019.04.047
29. Ramaswamy VV, Bandyopadhyay T, Nanda D, Bandiya P, Ahmed J, Garg A, et al. Assessment of postnatal corticosteroids for the prevention of bronchopulmonary dysplasia in preterm neonates: a systematic review and network meta-analysis. JAMA Pediatr. (2021) 175(6):e206826. doi: 10.1001/jamapediatrics.2020.6826
30. Zeng L, Tian J, Song F, Li W, Jiang L, Gui G, et al. Corticosteroids for the prevention of bronchopulmonary dysplasia in preterm infants: a network meta-analysis. Arch Dis Child Fetal Neonatal Ed. (2018) 103(6):F506–11. doi: 10.1136/archdischild-2017-313759
31. Onland W, van de Loo M, Offringa M, van Kaam A. Systemic corticosteroid regimens for prevention of bronchopulmonary dysplasia in preterm infants. Cochrane Database Syst Rev. (2023) 3. doi: 10.1002/14651858.CD010941.pub2
32. Murphy BP, Inder TE, Huppi PS, Warfield S, Zientara GP, Kikinis R, et al. Impaired cerebral cortical gray matter growth after treatment with dexamethasone for neonatal chronic lung disease. Pediatrics. (2001) 107(2):217–21. doi: 10.1542/peds.107.2.217
33. Yeh TF, Lin YJ, Lin HC, Huang CC, Hsieh WS, Lin CH, et al. Outcomes at school age after postnatal dexamethasone therapy for lung disease of prematurity. N Engl J Med. (2004) 350(13):1304–13. doi: 10.1056/NEJMoa032089
34. Dassios T, Kaltsogianni O, Greenough A. Second course of systemic dexamethasone: efficacy and respiratory function changes. J Matern Fetal Neonatal Med. (2022) 35(7):1401–4. doi: 10.1080/14767058.2020.1752653
35. Nguyen T, Jordan BK. Let’s talk about dex: when do the benefits of dexamethasone for prevention of bronchopulmonary dysplasia outweigh the risks?.. Newborn (Clarksville). (2022) 1(1):91. doi: 10.5005/jp-journals-11002-0009
36. Bloomfield FH, Knight DB, Harding JE. Side effects of 2 different dexamethasone courses for preterm infants at risk of chronic lung disease: a randomized trial. J Pediatr. (1998) 133(3):395–400. doi: 10.1016/S0022-3476(98)70277-X
37. Doyle LW, Halliday HL, Ehrenkranz RA, Davis PG, Sinclair JC. An update on the impact of postnatal systemic corticosteroids on mortality and cerebral palsy in preterm infants: effect modification by risk of bronchopulmonary dysplasia. J Pediatr. (2014) 165(6):1258–60. doi: 10.1016/j.jpeds.2014.07.049
Keywords: postnatal steroids, dexamethasone, extremely low birth weight, bronchopulmonary dysplasia, chronic lung disease of prematurity, Respiratory distress syndrome, preterm (birth), neonatal intensive care unit (NICU)
Citation: Al-taweel HM, Abdelhady ISI, Irfan N, Khzzam FA, Kamal A, Thazhe SBK, Bayoumi MAA and Gad A (2023) Comparing low-dose (DART) and enhanced low-dose dexamethasone regimens in preterm infants with bronchopulmonary dysplasia. Front. Pediatr. 11:1261316. doi: 10.3389/fped.2023.1261316
Received: 19 July 2023; Accepted: 27 September 2023;
Published: 31 October 2023.
Edited by:
Alain Cuna, Children’s Mercy Kansas City, United StatesReviewed by:
Noah H. Hillman, Saint Louis University, United StatesChristopher Nitkin, Children’s Mercy Kansas City, United States
© 2023 Al-taweel, Abdelhady, Irfan, Khzzam, Kamal, Thazhe, Bayoumi and Gad. This is an open-access article distributed under the terms of the Creative Commons Attribution License (CC BY). The use, distribution or reproduction in other forums is permitted, provided the original author(s) and the copyright owner(s) are credited and that the original publication in this journal is cited, in accordance with accepted academic practice. No use, distribution or reproduction is permitted which does not comply with these terms.
*Correspondence: Ashraf Gad agad2@hamad.qa