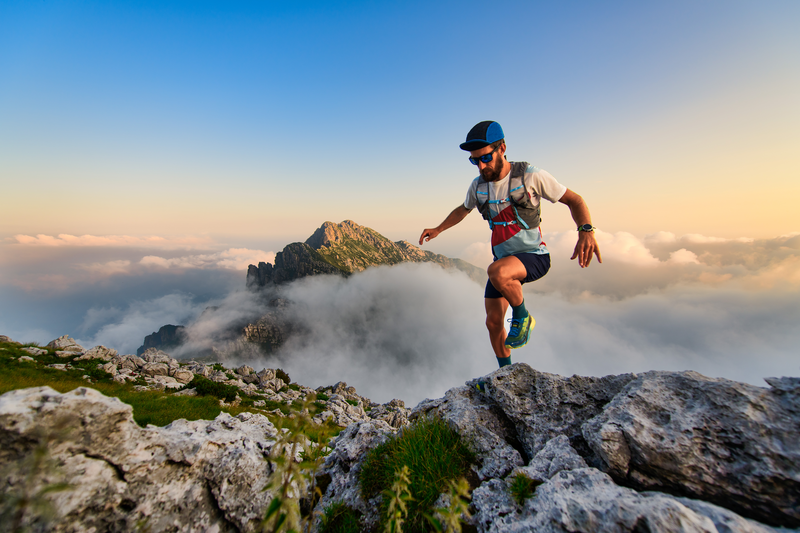
94% of researchers rate our articles as excellent or good
Learn more about the work of our research integrity team to safeguard the quality of each article we publish.
Find out more
SYSTEMATIC REVIEW article
Front. Pediatr. , 06 September 2023
Sec. Pediatric Immunology
Volume 11 - 2023 | https://doi.org/10.3389/fped.2023.1253333
The catastrophic coronavirus disease 2019 (COVID-19) pandemic has raised many health questions, and whether breast milk from SARS-CoV-2 infected mothers may be a vector for SARS-CoV-2 transmission has become a hot topic of concern worldwide. Currently, there are extremely limited and conflicting data on the risk of infection in infants through breastfeeding. For this reason, we investigated almost all current clinical studies and systematically analyzed the presence of SARS-CoV-2 and antibodies in the breast milk of mothers infected with SARS-CoV-2, their effects on newborns, and the mechanisms involved. A total of 82 studies were included in this review, of which 66 examined the presence of SARS-CoV-2 in breast milk samples from mothers diagnosed with COVID-19, 29 reported results of antibody detection of SARS-CoV-2 in breast milk, and 13 reported both nucleic acid and antibody test results. Seventeen studies indicated the presence of detectable SARS-CoV-2 nucleic acid in breast milk samples, and only two studies monitored viral activity, both of which reported that infectious viruses could not be cultured from RNA-positive breast milk samples. All 29 studies indicated the presence of at least one of the three antibodies, IgA, IgG and IgM, in breast milk. Five studies indicated the presence of at least one antibody in the serum of breastfed newborns. No COVID-19-related deaths were reported in all 1,346 newborns. Our study suggests that direct breastfeeding does not pose an additional risk of infection to newborns and that breast milk is a beneficial source of anti-SARS-CoV-2 antibodies that provide passive immune protection to infants. In addition, direct breastfeeding would provide maternal benefits. Our review supports the recommendation to encourage direct breastfeeding under appropriate infection control guidelines.
Systematic Review Registration: https://www.crd.york.ac.uk/PROSPERO/#myprospero, identifier: 458043.
During the global pandemic of COVID-19, the safety of direct breastfeeding by infected mothers to their infants has become one of the hottest topics. Few conditions are considered to be clear contraindications to breastfeeding. Known pathogens that can be transmitted in breastfeeding include HIV (1), human T-lymphotropic virus 1 (2), and cytomegalovirus (3). Previously, some studies showed the presence of SARS-CoV-2 in the breast milk of a few cases (4–6), a situation that caused considerable alarm. There is insufficient evidence as to whether breastfeeding is a possible mode of vertical mother-to-child transmission.
Breast milk is the gold standard for infant feeding. Not only does breastfeeding provide the best source of nutrition for the newborn and a powerful first barrier against infection, but it also has the emotional stimulation to enhance dynamic, two-way communication between mother and infant, laying the foundation for a physical and psychological bond between mother and child (7). In the short and long term, breastfeeding provides tremendous health benefits for both child and mother, especially the immunological properties of breast milk that make it a protective factor against infant morbidity and mortality (8). For infants, passive immunity is derived mainly from breast milk. SARS-CoV-2-specific antibodies have been reported to be detected in the breast milk of infected women (9, 10). The transfer of these antibodies to infants through breast milk may protect against SARS-CoV-2 infection. So, are SARS-CoV-2 antibodies present in breast milk or not? Could they provide benefits to newborns? Although the World Health Organization declared on May 5, 2023, that the spread of COVID-19 was no longer a “public health emergency of international concern”, the global interest in ensuring that mothers and infants are not separated and that breastfeeding mothers are provided with the necessary support and/or breast milk for their infants has been well established for some time, and there are very limited and conflicting data on the presence of SARS-CoV-2 in breast milk and the potential for vertical transmission in breast milk. Therefore, there is a strong need for a comprehensive analysis and discussion of the evidence regarding the potential for vertical transmission of breastfeeding and the presence of anti-SARS-CoV-2 antibodies in breast milk. Here, we plan to use this systematic review to synthesize the data published to date on the risk of SARS-CoV-2 transmission through breast milk, to provide a comprehensive summary of the presence and characteristics of antibodies in breast milk and their effects on infants, to provide evidence for assessing the risk-benefit of breast milk transmission vs. breastfeeding, and to provide a basis for the management of mother-infant dyads and optimal health strategies for breastfeeding in similar outbreaks in the future.
Is breast milk of SARS-CoV-2 infected mothers a possible carrier of SARS-CoV-2 transmission? What is more important, breastfeeding or breast milk transmission?
An experienced information specialist conducted a comprehensive search of PubMed, MEDLINE, CNKI, Wanfang Database, bioRxiv, medRxiv, Embase, and Cochrane Library online databases, and to maximize the scope of the review without time and language restrictions, the last data update was April 30, 2023. We used the keywords “SARS-CoV-2”, “COVID-19”, “breast milk”, and “antibody” individually or in combination to achieve a comprehensive literature search. We also searched the gray literature of the preprint servers bioRxiv and medRxiv. In addition, we manually searched the references of the original articles included in the study to avoid missing important literature that was not noted in the initial search. Inclusion criteria were (i) the presence of SARS-CoV-2 RNA or/and the presence of anti-SARS-CoV-2 antibodies in mothers diagnosed with SARS-CoV-2 infection and (ii) breast milk samples. Studies that included the same study population but reported different data and outcomes were also included. Exclusion criteria were (i) questionnaires; (ii) studies that explored breastfeeding intentions only (psychological); (iii) studies in which mothers were not diagnosed with SARS-CoV-2 infection; (iv) artificial vaccinations or mixed groups with vaccinations that could not be grouped separately; (v) studies with severely missing data on mothers, breast milk samples, or newborns; and (vi) studies that tested only breast milk studies that detect factors other than SARS-CoV-2 RNA or antibodies in breast milk, including cytokines, chemical elements, proteomes, and nerve growth factors. None of the literature types were restricted. See Supplementary Material S1 for detailed search strategies.
After completing the initial search, two independent reviewers conducted a screening process, and literature with quantifiable evidence was included in our review. We excluded duplicate publications and duplicate case studies (we identified duplicates based on author name, location, participant admission date, maternal and neonatal characteristics, and publication date). One reviewer reviewed the selected articles in their entirety, and studies that contained complete data descriptions were used for data graphing. Any conflicts that arose during the data extraction process were resolved by consensus with a third reviewer. All seven authors participated in the discussion and decided on the topic.
As of April 30, 2023, we retrieved a total of 1,274 publications, and after screening by the inclusion criteria described above, we qualified 561 full-text papers, plus four manually retrieved papers, resulting in 82 papers for inclusion in this review. We extracted data for each paper regarding the first author's name, country, study design, basic demographic characteristics of participants, maternal samples, breast milk samples, SARS-CoV-2 RNA or antibody test results, and number of newborns and feeding patterns for tabulation and discussion. We did not perform any meta-analysis of the data obtained but used a narrative synthesis because, as expected, there was substantial heterogeneity among the studies we retrieved, making it difficult to make meaningful comparisons between studies. Figure 1 shows a visual representation containing the workflow.
A total of 82 studies were finally included (4, 6, 9–88), including three studies published in Chinese (25, 27, 28). They came from 21 countries, with 26 studies from China (31.7%). The earliest study start time is December 2019 (24), and the longest study span is 12 months (86). Thirteen studies reported both nucleic acid test results and antibody test results. Of 82 studies, 33 case reports and nine case series were included. A total of 1,755 mothers and 1,346 newborns and at least 2,043 breast milk samples were included in the studies. The maximum sample size of mothers included in the study was 165, the maximum sample size of breast milk was 316, and the maximum sample size of newborns was 165. The follow-up period ranged from three days to 11 months. The characteristics of the included studies are listed in Table 1.
A total of 66 studies examined the presence of SARS-CoV-2 in breast milk samples from mothers with a confirmed diagnosis of COVID-19 (4, 6, 9, 11–73). All included studies used RT-PCR assays. Fifty-five studies reported the timing of breast milk specimen collection, with the earliest being immediately after delivery and the longest span of breast milk specimen collection being 206 days. A summary of studies on the detection of SARS-CoV-2 in human milk is presented in Table 2.
Of these 66 studies, 17 reported positive results for SARS-CoV-2 nucleic acid in breast milk samples (4, 6, 11, 14, 17, 18, 29, 32, 33, 36, 38, 39, 46, 47, 55, 59, 72), but only two studies monitored viral activity (6, 47). The remaining 49 studies showed negative results for SARS-CoV-2 in breast milk. Thirty-eight studies reported full or partial use of breastfeeding for newborns. Of the 17 studies that were positive for SARS-CoV-2 RNA in breast milk, 179 newborns in 11 studies were given breast milk, and only 18 (10%) had a positive nasopharyngeal swab result (one study did not report a positive result in newborns). Notably, 53 (15.2%) of the 16 studies with negative breast milk samples had positive nasopharyngeal swabs in newborns. Interestingly, three newborns in three studies of 22 formula-fed newborns also showed positive nasopharyngeal swab results (11, 31, 46). No deaths were reported in all 1,346 neonates.
Twenty-nine studies reported results of antibody testing against SARS-CoV-2 in at least 1,279 breast milk samples from mothers with a confirmed diagnosis of COVID-19 (9, 10, 62–88), and the majority of mothers included in these studies had mild disease severity. All 29 studies showed the presence of at least one of the three antibodies, IgA, IgG and IgM, in breast milk. Twenty-seven of these studies reported the method of detection, and 23 studies used an ELISA assay. The longest duration of antibodies was ten months (80). Twenty-three studies found IgA antibodies in breast milk samples, 17 studies found IgG antibodies in breast milk samples, and 11 studies reported the presence of IgM antibodies in breast milk. Six studies indicated that all three antibodies, IgA, IgG and IgM, were detected in breast milk (10, 68, 69, 79, 87, 88). Nine studies also reported serum antibodies in newborns born to mothers, five studies reported the presence of at least one antibody in the serum of newborns (63, 70–72, 88), most of whom were breastfed, four studies reported negative serum antibodies in newborns (62, 69, 75, 78), with one study detecting IgA antibodies in the saliva of newborns (75). Table 3 presents a summary of studies in which antibodies against SARS-CoV-2 were detected in human milk.
Table 3. Characteristics of included studies of detection anti-SARS-CoV-2 antibodies in human breast milk.
Of the 66 studies examining the presence of SARS-CoV-2 in breast milk samples, 17 studies reported positive results for SARS-CoV-2 nucleic acid in 38 breast milk samples. Zhu et al. (17) and Thanigainathan et al. (39) retested breast milk collected from the same mother the next day of the positive samples and showed different results. The results of Zhu et al. suggested that the breast milk was still positive for SARS-CoV-2, while the results of Thanigainathan et al. were negative. Similarly, Tam et al. (32) repeated the test on breast milk samples from the same mother on day 5 and day 15 after the onset of symptoms, and the results were positive. In contrast, Hinojosa-Velasco et al. (11) reported a mother whose breast milk showed a positive result on day 1 after delivery but a negative result when her breast milk sample was retested on day 13 after delivery. A report from Groß et al. (4) of 11 breast milk samples from two mothers found positive breast milk samples from the same mother on four consecutive days. Costa et al. (36) also reported three positive results for SARS-CoV-2 in six breast milk samples from one woman. Kilic et al. (38) investigated breast milk samples from 15 COVID-19 mothers, and SARS-CoV-2 RNA was detected in the breast milk of four mothers. A study from Italy tested ten breast milk samples collected from the same mother during the first five days after the birth of her newborn, and three samples were positive for SARS-CoV-2 RT-PCR (46). The remaining 49 studies, including at least 1,229 breast milk samples, did not detect SARS-CoV-2 RNA. These data suggest that SARS-CoV-2 RNA is indeed present in the breast milk of COVID-19 mothers.
Although SARS-CoV-2 RNA was detected in 38 breast milk from 17 studies, only two studies monitored viral activity (6, 47). Krogstad et al. (47) performed viral cultures on 160 breast milk samples and did not detect the virus in any culture, although SARS-CoV-2 RNA was detectable in 9.2% of these milk samples. Notably, they artificially added virus to breast milk samples from control experiments and infectious SARS-CoV-2 could be cultured despite several freeze-thaw cycles. Chambers et al. (6) added replication-competent SARS-CoV-2 to two breast milk samples that were pasteurized. The authors failed to detect SARS-CoV-2 RNA or culturable virus in the Holder pasteurized breast milk samples. In contrast, the two unpasteurized breast milk samples from the control group were found to be positive for viral RNA. These studies suggest that SARS-CoV-2 virus particles detected in breast milk are not infectious. In fact, there are no reports of transmission of SARS-CoV and Middle East Respiratory Syndrome coronavirus (MERS-CoV) through breast milk.
Of the 179 children given breast milk in the 17 breast milk SARS-CoV-2 positive studies, only 18 (10%) of these children had positive nasopharyngeal swab SARS-CoV-2 nucleic acid results and the majority (90%) of newborns had negative nasopharyngeal swab results.
Of the 26 studies in which breast milk samples tested negative for nucleic acid, 219 children were given breast milk, and 11 of these studies found 24 children with positive nasopharyngeal swab nucleic acid test results for SARS-CoV-2.
Results from three of the eight formula-fed studies showed that three children tested positive for SARS-CoV-2 on nucleic acid from nasopharyngeal swabs (11, 31, 46).
The mothers included in this review were all SARS-CoV-2 positive mothers, and 50 children born to these positive mothers in 16 studies turned out to be SARS-CoV-2 positive, but the breast milk of these mothers tested negative for SARS-CoV-2.
In addition, the largest cohort study published to date showed no evidence of vertical mother-to-child transmission when newborns were roomed with their mothers and breastfed. On the other hand, mother-child separation does not guarantee a virus-free environment for the infant.
These findings provide strong evidence that there is no necessary link between breastfeeding and a child being nucleic acid positive, suggesting that breast milk is not a source of vertical transmission. All this evidence supports the view that mothers infected with SARS-CoV-2 do not put their newborns at additional risk of infection through breastfeeding.
1. The first is respiratory contamination from the mother
Mothers do not wear masks when collecting breast milk samples or breastfeeding, which increases the likelihood that breast milk or the newborn will be contaminated with the mother's respiratory secretions, especially during the acute symptomatic phase when the mother's respiratory viral load is extremely high (89).
2. The second is pollution from the environment
Because breastfeeding involves a range of intimate behaviors between mother and infant, including hand contact and skin-to-skin contact with the breast and contact with the surface of the container holding the milk, the risk of environmental contamination of breast milk or the newborn is possible (90).
3. Exposure to a SARS-CoV-2 infected third party
Newborns were infected with SARS-CoV-2 after delivery through close contact with infected family members (43), especially considering that in these cases, parents, relatives, caregivers of newborns, and individuals in their communities were diagnosed with COVID-19 and were susceptible to horizontal human-to-human transmission (91).
We believe that even if the detectable SARS-CoV-2 RNA in breast milk samples is the result of contamination, this is still an important finding because it is a potential and unanticipated source of exposure for uninfected infants. This emphasizes the importance of proper breast hygiene before feeding.
SARS-CoV-2 enters host cells by binding to the ACE2 receptor (92, 93). The ACE2 receptor is expressed in both female reproductive organs and breast tissue (94). Although ACE2 receptor expression is extremely low in breast tissue (only 5%), SARS-CoV-2 could theoretically be present in any tissue with an ACE2 receptor and, therefore, SARS-CoV-2 may still be shed from the breast milk of infected mothers during lactation.
Human milk has the potential to be contaminated with SARS-CoV-2 RNA from the infant's oropharynx to the breast. If the infant is infected earlier than the infant's parents, there is a possibility of “reverse” vertical transmission from the infant to the mother, a phenomenon that has been observed in other pathogens such as HIV (95, 96) and Ebola (97). In this case, one possible mechanism of maternal infection is retrograde flow, in which milk and saliva move from the infant's mouth back to the mammary gland during suckling.
It is known that some viruses can be transmitted through breast milk and that higher serum viral load can increase the risk of transmission (98). In the case of HIV and HTLV-1, the level of the virus in breast milk correlates with the amount of virus in the whole body (2, 99, 100).
In addition to this, Bastug et al. (59) believe that the method of detection of the virus in breast milk, the timing of sample collection, and the transportation and storage of the samples are all potential factors that could lead to a positive result.
Although 17 studies reported the presence of detectable SARS-CoV-2 RNA in breast milk samples, however, it is reassuring, that longitudinal follow-up of most studies showed no adverse outcomes in infants who still continued to be breastfed, including those in whom viral RNA was detectable on the skin of the mother's breast (73). Of the 1,346 newborns included, only three were admitted to the NICU (60, 72). Notably, in the context of the COVID-19 pandemic at the time, most of the newborns in the study were admitted to the NICU for isolation and observation rather than for any absolute indication. No deaths due to COVID-19 were reported in all neonates.
There are several possible reasons why the majority of studies (74%) failed to detect SARS-CoV-2 RNA in the breast milk of COVID-19 mothers. One of the most important reasons could be due to the extremely low level of ACE2 expression in the breast (94, 101, 102). Indeed, ACE2 needs to be co-expressed with protease, TMPRSS2, or CTSB/L to activate the S protein and promote the entry of SARS-CoV-2 into host cells. However, only 5% of mammary cells express ACE2 (103). The second reason is the antiviral mechanism of SARS-CoV-2 by specific substances in breast milk. Breast milk contains whey protein (104), lactoferrin (105), and mucin (106), which can block virus entry and replication by binding to various receptors of SARS-CoV-2. In addition, the presence of antibodies in breast milk, especially IgA antibodies, which have neutralizing activity against SARS-CoV-2, is also believed to be one of the reasons for the absence of SARS-CoV-2 in breast milk (67). Third, if breast milk samples are collected farther back in time from the time of infection, the viral load in breast milk will be lower, especially for asymptomatic mothers, who may not be able to detect SARS-CoV-2 RNA in breast milk because it is difficult for them to determine the onset of infection.
All 29 studies that reported antibody test results indicated the presence of at least one of three antibodies in breast milk, IgA, IgG and IgM, primarily IgA but also IgG and IgM against SARS-CoV-2 RBD. These studies showed the presence of different types of antibodies and different rates of positivity in breast milk, while four studies indicated the presence of one or more antibodies in all (100%) of their respective breast milk samples (10, 55, 70, 77). In a multicenter study from the United States that examined 316 breast milk samples for levels of anti-RBD IgA, Pace et al. (67) found that 75% (n = 316) of the milk samples contained anti-RBD IgA for at least two months. This is by far the largest study of breast milk sample size. A large prospective cohort study by Juncker et al. (80) showed that of 165 participants who had confirmed SARS-CoV-2 infection by PCR, 98 (59%) had SARS-CoV-2 specific IgA antibodies in their breast milk. Such antibodies were still present at least ten months after infection. In Brazil, Dutra et al. (86) conducted a cross-sectional study of 165 participants infected with SARS-CoV-2 during pregnancy and their newborns, collecting postpartum colostrum samples from mothers. The results showed that 117 (70.9%) women were positive for anti-SARS-CoV-2 IgA in colostrum and confirmed that the presence of anti-SARS-CoV-2 IgA in colostrum was independently associated with lower clinical signs in newborns. Two (1.2%) participants were also found to be positive for anti-SARS-CoV-2 IgG in colostrum. These two studies are the largest samples of mothers included to date (80, 86). The study by Bode et al. (82) showed that detectable IgA antibodies were found in breast milk even eight months after onset. IgM antibodies were also present in breast milk, albeit at lower concentrations (9, 10, 62, 68, 87). In general, IgM and IgA-like responses often occur earlier after the onset of the disease, while IgG responses occur later (107). It is known that antibody abundance in breast milk is variable and there is a high degree of intra- and inter-individual variability in the three antibody classes (108). A study by Fox et al. (79) has demonstrated much higher titers of IgA than expected in 12/15 milk samples. The difference between IgA and IgG may be due to the fact that systemic IgG-secreting B cells are shorter-lived than IgA-secreting B cells in the submucosa of the breast. It is likely that antibody secretion also depends on the time elapsed after the onset of the disease, and that antibody levels in breast milk decline over time (84, 109). Those participants who showed only IgA antibodies in breast milk were more likely to be enrolled immediately after SARS-CoV-2 infection, whereas those who showed only IgG antibodies in breast milk were more likely to be enrolled some time after infection. Breast milk samples were collected closest to the time point of SARS-CoV-2 infection where antibody levels were likely to be highest. In addition, the severity of symptoms may have influenced the residence time of antibodies in breast milk, with more severe SARS-CoV-2 infections resulting in immune responses with higher antibody levels (110, 111).
Large amounts of Igs in the mother's body can be transmitted to the newborn through breast milk (112, 113). IgG antibodies are a possible indication of immunity or resistance. Of the 29 included studies that reported breast milk antibody test results, five showed that neonates were seropositive for antibodies to SARS-CoV-2 (63, 70–72, 88). Interestingly, Conti et al. (75) also detected the presence of IgA antibodies in the saliva of newborns. In China, Gao et al. (71) conducted an observational clinical analysis of 14 mother-infant pairs and found that three of the breast milk samples tested positive for SARS-CoV-2 IgM or IgG, and the corresponding three newborns fed by breast milk tested negative for SARS-CoV-2 RNA at birth, while they tested positive for SARS-CoV-2 IgG, and one of the neonates also tested positive for IgM. No SARS-CoV-2 nucleic acid was detected in breast milk samples at different stages. This suggests that specific antibodies to SARS-CoV-2 can be transferred to the infant through breast milk and provide potential protection to the neonate. Similarly, the studies of Dong et al. (63) and Fenizia et al. (72) support this idea. At present, it is unclear which factors affect the efficiency of the transfer of these maternal antibodies, and further studies are needed.
It is well known that antibodies can bind to the RBD of the surface spike protein of SARS-CoV-2, preventing the virus from binding to the ACE2 receptor of the target cell (114). This implies that in the context of COVID-19, antibodies are able to limit virus transmission. The secretory immunoglobulin A (sIgA) of anti-SARS-CoV-2 neutralizes SARS-CoV-2 before they reach and bind to epithelial cells. Secretory IgA acts directly on the mucosal surface to inhibit microbial binding to host epithelial cell receptors and to trap pathogenic microorganisms in mucus, enhancing ciliary activity and thereby eliminating invading pathogens (115, 116), providing durable passive immunity to newborns and infants (117). In addition to IgA, IgG in breast milk can attack viral envelope glycoproteins and provide the body with potent systemic antibodies. IgG also acts as an antibody that degrades intracellular viruses by binding to a crystallizable cytosolic fragment motif containing −21 tripartite receptor (TRIM21) (118). These antibodies can be passed on to offspring through breastfeeding, preventing or reducing the severity of disease in newborns. It has been found that even partially degraded IgA, IgG and IgM antibodies, if their antigen-binding fragment (Fab) fraction remains intact, still function and bind to the antigen, leading to target degradation and elimination from the intestine (119). This suggests that any neutralizing SARS-CoV-2 targeting antibodies present in breast milk may have a protective effect on infants receiving breast milk. In addition, intestinal Fc receptors may transport antibodies from the gut to the vascular circulation, further aiding systemic defense. IgA spiking antigen immune complexes would also stimulate active immunity and contribute to the maturation of the infant's immune system (75). This will facilitate the protection of the newborn from a variety of viral pathogens. This active form of protection of human milk against viruses cannot be provided to the infant through artificial formula.
Typically, maternal IgG antibodies are retained in the infant for more than six months, and infants who are unable to produce immunoglobulins are protected by maternal antibodies for up to 12 months after birth (120). Intriguingly, some studies suggest that antibodies transferred to newborns through breast milk are lower than expected (121) and decline in the infant faster (122) and for a shorter duration than expected (63).
These data highlight that preferential transfer of SARS-CoV-2 specific IgA and IgM and/or IgG in breast milk to newborns can create a non-pathological, but highly protective barrier against COVID-19 disease in breastfed infants. Unfortunately, some studies have focused on the presence and characteristics of breast milk antibodies, and incomplete information about newborns has weakened the support for the protective effect of antibodies on infant and child health. Also, these studies did not consider the effect of drugs used to treat COVID-19 on breast milk antibodies. Larger sample sizes and long-term follow-up studies with long-term follow-up of antibody class, duration, and titer changes are needed to better understand the association of SARS-CoV-2 antibodies in milk with COVID-19 outcomes in infants.
Breast milk is rich in nutrients (123) and since it requires no preparation and is always at the right temperature, it helps to provide a complete, balanced, adequate, and proper diet (124). Not only is it the gold standard source of nutrition for infants, but it is the primary source of passive and active immunization for newborns (81), and breastfeeding can reduce neonatal mortality (125). The role of breastfeeding in protecting infants from infection is also crucial. The immaturity of an infant's immune system at birth increases the risk of infection from external factors, including viruses and bacteria (126), and breastfeeding can significantly reduce the risk of infection in infants (127). Breast milk contains antibodies, linoleic acid (128, 129), milk fat globule membranes, human milk oligosaccharides, osteopontin, and other antiviral components (130, 131). These nutrients bind to some of the receptors required for viral entry into host cells, limiting the ability of viruses to enter and achieving antiviral [including papillomavirus (132), human immunodeficiency virus (133), rotavirus (134), chikungunya virus (135) and Zika virus (135)], antiparasitic (136) and antifungal effects (137).
In addition, the large presence of bioactive mediators in breast milk [IL-1b, IL-2, IL-6, IL-8, IL-10, IL-12, IL-18, IFN-γ, TNFα, TGF-b, granulocyte colony-stimulating factor (G-CSF), macrophage colony-stimulating factor (M-CSF), granulocyte-macrophage colony-stimulating factor (GM-CSF)] (138, 139), which can compensate for the lack of cytokine pool in newborns. On the other hand, the benefits of breast milk are much broader, considering the emotional, cognitive, psychological, socioeconomic, and environmental development of the child and mother (140, 141). Mother-infant separation hurts the physical and mental health of both mother and infant (142), and separation of mother and infant after birth is considered a source of stress, leading to an increased incidence of developmental problems in children (143). For mothers, early cessation of breastfeeding is a significant cause of maternal depressive symptoms in the postpartum period (144). In addition, a reduced risk of breast cancer or reproductive system cancers has been observed in breastfeeding women (145). Breastfeeding mothers are less likely to develop hypertension, diabetes, hyperlipidemia, cardiovascular disease, and metabolic syndrome (146, 147).
To our knowledge, this is the largest systematic review of SARS-CoV-2 and its antibodies in breast milk to date, with the largest number of included studies, the most comprehensive assessment parameters, and the most thorough assessment of virological evidence. The studies that examined nucleic acids included different time points of breast milk collection, included cases at different gestational weeks, and had more representative findings. The geographic distribution of the included study population was globally representative (21 countries including Arab countries). In studies examining antibodies, the presence and effectiveness of antibodies in breast milk against the virus were linked to the clinical outcomes of newborns. Our review answers a series of real-world questions of great relevance, particularly those that physicians and mothers are eager to answer. These data will have implications beyond the epidemic, providing objective, evidence-based data for developing optimal strategies for breastfeeding and maternal and infant management in similar epidemic pandemics in the future.
Our study also has some limitations. First, most studies did only qualitative SARS-CoV-2 RNA analysis and lacked precise viral load assessment, and only two studies performed cell culture. Second, most studies were retrospective, observational, and cross-sectional, and some studies did not focus on the presence of nucleic acids or antibodies but simply reported nucleic acid or antibody results, and these limitations limited our ability to study the long-term health effects of breastfeeding on infants born to SARS-CoV-2-infected mothers. Third, the small size of the sample for most of the studies, which included 33 case reports and nine case series. Despite repeatedly reading and analyzing the included literature, we could not confirm that we had excluded all potentially repeatedly reported cases. Also, there is a risk of publication bias given that COVID-19-positive cases in infants and breast milk are most likely to be reported and published. Finally, given the importance of summarizing all available cases, we did not assess the quality of the studies included in this review.
In conclusion, regardless of whether the breast milk of confirmed COVID-19 mothers was positive or negative for SARS-CoV-2 RNA, direct breastfeeding did not pose an additional risk of infection to the neonate, and in any case, neonatal outcomes were favorable, with no neonatal COVID-19-related deaths and good health outcomes for all infants in all studies included in this review. Also, breast milk secreted by infected mothers is a beneficial source of anti-SARS-CoV-2 antibodies that neutralize the activity of SARS-CoV-2. Not only does direct breastfeeding not exacerbate the severity of the disease, but it may provide passive immune protection to the infant. In addition, direct breastfeeding would benefit the mother by reducing depression and anxiety in the mother. Notably, given the potential for respiratory or contact transmission (horizontal transmission) of SARS-CoV-2 during breastfeeding, breastfeeding should be conducted under appropriate infection control guidelines.
The original contributions presented in the study are included in the article/Supplementary Material, further inquiries can be directed to the corresponding author.
YH: Conceptualization, Data curation, Formal Analysis, Investigation, Methodology, Software, Visualization, Writing – original draft, Writing – review & editing. JL: Data curation, Investigation, Methodology, Resources, Visualization, Writing – review & editing. XH: Data curation, Investigation, Resources, Writing – review & editing. HL: Writing – review & editing. NW: Writing – review & editing. JW: Data curation, Investigation, Resources, Writing – review & editing. ZJ: Data curation, Investigation, Resources, Writing – review & editing.
The author(s) declare that no financial support was received for the research, authorship, and/or publication of this article.
Many thanks to Han Boning for editing the manuscript in English. Many thanks to Zhao Kai for his help in English during the drafting of the manuscript.
The authors declare that the research was conducted in the absence of any commercial or financial relationships that could be construed as a potential conflict of interest.
The Supplementary Material for this article can be found online at: https://www.frontiersin.org/articles/10.3389/fped.2023.1253333/full#supplementary-material.
All claims expressed in this article are solely those of the authors and do not necessarily represent those of their affiliated organizations, or those of the publisher, the editors and the reviewers. Any product that may be evaluated in this article, or claim that may be made by its manufacturer, is not guaranteed or endorsed by the publisher.
1. Dunn D, Newell M, Ades A, Peckham C. Risk of human immunodeficiency virus type 1 transmission through breastfeeding. Int J Gynecol Obstet. (1993) 41(1):111. doi: 10.1016/0020-7292(93)90174-u
2. Rosadas C, Taylor GP. Mother-to-child HTLV-1 transmission: unmet research needs. Front Microbiol. (2019) 10:999. doi: 10.3389/fmicb.2019.00999
3. Kurath S, Halwachs-Baumann G, Resch B. Transmission of cytomegalovirus via breast milk to the prematurely born infant: a systematic review. Clin Microbiol Infec. (2010) 16(8):1172–8. doi: 10.1111/j.1469-0691.2010.03140.x
4. Groß R, Conzelmann C, Müller JA, Stenger S, Steinhart K, Kirchhoff F. Detection of SARS-CoV-2 in human breastmilk. Lancet. (2020) 395:1757–8. doi: 10.1016/S0140-6736(20)31181-8
5. Centeno-Tablante E, Medina-Rivera M, Finkelstein JL, Rayco-Solon P, Garcia-Casal MN, Rogers L, et al. Transmission of SARS-CoV-2 through breast milk and breastfeeding: a living systematic review. Ann N Y Acad Sci. (2021) 1484:32–54. doi: 10.1111/nyas.14477
6. Chambers C, Krogstad P, Bertrand K, Contreras D, Tobin NH, Bode L, et al. Evaluation for SARS-CoV-2 in breast milk from 18 infected women. JAMA. (2020) 324:1347–8. doi: 10.1101/2020.06.12.20127944
7. Ahn S, Corwin EJ. The association between breastfeeding, the stress response, inflammation, and postpartum depression during the postpartum period: prospective cohort study. Int J Nurs Stud. (2015) 52(10):1582–90. doi: 10.1016/j.ijnurstu.2015.05.017
8. Victora CG, Bahl R, Barros AJ, França GV, Horton S, Krasevec J, et al. Breastfeeding in the 21st century: epidemiology, mechanisms, and lifelong effect. Lancet. (2016) 387(10017):475–90. doi: 10.1016/S0140-6736(15)01024-7
9. Peng S, Zhu H, Yang L, Cao L, Huang X, Dynes M, et al. A study of breastfeeding practices, SARS-CoV-2 and its antibodies in the breast milk of mothers confirmed with COVID-19. Lancet Reg Health West Pac. (2020) 4:100045. doi: 10.1016/j.lanwpc.2020.100045
10. Demers-Mathieu V, DaPra C, Mathijssen G, Sela DA, Järvinen KM, Seppo A, et al. Human milk antibodies against S1 and S2 subunits from SARS-CoV-2, HCoV-OC43, and HCoV-229E in mothers with a confirmed COVID-19 PCR, viral symptoms, and unexposed mothers. Int J Mol Sci. (2021) 22(4):1–13. doi: 10.3390/ijms22041749
11. Hinojosa-Velasco A, de Oca PVB, García-Sosa LE, Mendoza-Durán JG, Pérez-Méndez MJ, Dávila-González E, et al. A case report of newborn infant with severe COVID-19 in Mexico: detection of SARS-CoV-2 in human breast milk and stool. Int J Infect Dis. (2020) 100:21–4. doi: 10.1016/j.ijid.2020.08.055
12. Olivini N, Calò Carducci FI, Santilli V, De Ioris MA, Scarselli A, Alario D, et al. A neonatal cluster of novel coronavirus disease 2019: clinical management and considerations. Ital J Pediatr. (2020) 46(1):180. doi: 10.1186/s13052-020-00947-9
13. Sahin D, Tanacan A, Erol SA, Anuk AT, Eyi EGY, Ozgu-Erdinc AS, et al. A pandemic center’s experience of managing pregnant women with COVID-19 infection in Turkey: a prospective cohort study. Int J Gynecol Obstet. (2020) 151(1):74–82. doi: 10.1002/ijgo.13318
14. Lugli L, Bedetti L, Lucaccioni L, Gennari W, Leone C, Ancora G, et al. An uninfected preterm newborn inadvertently fed SARS-CoV-2-positive breast milk. Pediatrics. (2020) 146(6). doi: 10.1542/peds.2020-004960
15. Cui Y, Tian M, Huang D, Wang X, Huang Y, Fan L, et al. A 55-day-old female infant infected with 2019 novel coronavirus disease: presenting with pneumonia, liver injury, and heart damage. J Infect Dis. (2020) 221(11):1775–81. doi: 10.1093/infdis/jiaa113
16. Gómez-Torres N, Sánchez-García L, Castro I, Arroyo R, Cabañas F, González-Sánchez R, et al. Metataxonomic analysis of milk samples from SARS-CoV-2-positive and SARS-CoV-2-negative women. Front Nutr. (2022) 9:853576. doi: 10.3389/fnut.2022.853576
17. Zhu C, Liu W, Su H, Li S, Shereen MA, Lv Z, et al. Breastfeeding risk from detectable severe acute respiratory syndrome coronavirus 2 in breastmilk. J Infection. (2020) 81(3):452–82. doi: 10.1016/j.jinf.2020.06.001
18. Kunjumon B, Wachtel EV, Lumba R, Quan M, Remon J, Louie M, et al. Breast milk and breastfeeding of infants born to SARS-CoV-2 positive mothers: a prospective observational cohort study. Am J Perinat. (2021) 38(11):1209–16. doi: 10.1055/s-0041-1731451
19. Kam KQ, Yung CF, Cui L, Tzer Pin Lin R, Mak TM, Maiwald M, et al. A well infant with coronavirus disease 2019 with high viral load. Clin Infect Dis. (2020) 71(15):847–9. doi: 10.1093/cid/ciaa201
20. Lang GJ, Zhao H. Can SARS-CoV-2-infected women breastfeed after viral clearance? J Zhejiang Univ-Sc B. (2020) 21(5):405–7. doi: 10.1631/jzus.B2000095
21. Yan J, Guo J, Fan C, Juan J, Yu X, Li J, et al. Coronavirus disease 2019 in pregnant women: a report based on 116 cases. Am J Obstet Gynecol. (2020) 223(1):111.e1–14. doi: 10.1016/j.ajog.2020.04.014
22. Chen H, Guo J, Wang C, Luo F, Yu X, Zhang W, et al. Clinical characteristics and intrauterine vertical transmission potential of COVID-19 infection in nine pregnant women: a retrospective review of medical records. Lancet. (2020) 395(10226):809–15. doi: 10.1016/S0140-6736(20)30360-3
23. Liu W, Wang J, Li W, Zhou Z, Liu S, Rong Z. Clinical characteristics of 19 neonates born to mothers with COVID-19. Front Med-Prc. (2020) 14(2):193–8. doi: 10.1007/s11684-020-0772-y
24. Chen L, Li Q, Zheng D, Jiang H, Wei Y, Zou L, et al. Clinical characteristics of pregnant women with COVID-19 in Wuhan, China. New Engl J Med. (2020) 382(25):e100. doi: 10.1056/NEJMc2009226
25. Zhuang SY, Guo JJ, Cao YM, Chen HJ, Xu D, Li JF, Zhang YZ. Perinatal COVID-19: a case report. Chin J Perinat Med. (2020) 2:85–9; (in Chinese). doi: 10.3760/cma.j.issn.1007-9408.2020.02.003
26. Mao LJ, Xu J, Xu ZH, Xia XP, Li B, He JG, et al. A child with household transmitted COVID-19. BMC Infect Dis. (2020) 20(1):329. doi: 10.1186/s12879-020-05056-w
27. Lei D, Wang C, Li CY, Fang CC, Yang WB, Cheng BH, et al. Clinical characteristics of COVID-19 in pregnancy: analysis of nine cases. Chin J Perinat Med. (2020) 3:159–65; (in Chinese). doi: 10.3760/cma.j.cn113903-20200216-00117
28. Chen X, Li Y, Wang J, Cai H, Cao H, Sheng J. Pregnant women complicated with COVID-19: a clinical analysis of 3 cases. J Zhejiang Univ (Med Sci). (2020) 49(2): 240–4; (in Chinese). doi: 10.3785/j.issn.1008-9292.2020.03.08
29. Wu Y, Liu C, Dong L, Zhang C, Chen Y, Liu J, et al. Coronavirus disease 2019 among pregnant Chinese women: case series data on the safety of vaginal birth and breastfeeding. Bjog-Int J Obstet Gy. (2020) 127(9):1109–15. doi: 10.1111/1471-0528.16276
30. Piersigilli F, Carkeek K, Hocq C, van Grambezen B, Hubinont C, Chatzis O, et al. COVID-19 in a 26-week preterm neonate. Lancet Child Adolesc. (2020) 4(6):476–8. doi: 10.1016/S2352-4642(20)30140-1
31. Wang S, Guo L, Chen L, Liu W, Cao Y, Zhang J, et al. A case report of neonatal 2019 coronavirus disease in China. Clin Infect Dis. (2020) 71(15):853–7. doi: 10.1093/cid/ciaa225
32. Tam PCK, Ly KM, Kernich ML, Spurrier N, Lawrence D, Gordon DL, et al. Detectable severe acute respiratory syndrome coronavirus 2 (SARS-CoV-2) in human breast milk of a mildly symptomatic patient with coronavirus disease 2019 (COVID-19). Clin Infect Dis. (2021) 72(1):128–30. doi: 10.1093/cid/ciaa673
33. Bertino E, Moro GE, De Renzi G, Viberti G, Cavallo R, Coscia A, et al. Detection of SARS-CoV-2 in milk from COVID-19 positive mothers and follow-up of their infants. Front Pediatr. (2020) 8:597699. doi: 10.3389/fped.2020.597699
34. Dong L, Pei S, Ren Q, Fu S, Yu L, Chen H, et al. Evaluation of vertical transmission of SARS-CoV-2 in utero: nine pregnant women and their newborns. Placenta. (2021) 111:91–6. doi: 10.1016/j.placenta.2021.06.007
35. Prasad A, Yankappa N, Kumar P, Chaudhary BK, Pati BK, Anant M, et al. Excretion of SARS-CoV-2 in breast milk: a single-centre observational study. BMJ Paediatr Open. (2021) 5(1):e001087. doi: 10.1136/bmjpo-2021-001087
36. Costa S, Posteraro B, Marchetti S, Tamburrini E, Carducci B, Lanzone A, et al. Excretion of SARS-CoV-2 in human breast milk. Clin Microbiol Infec. (2020) 26(10):1430–2. doi: 10.1016/j.cmi.2020.05.027
37. Yin MZ, Zhang LJ, Deng GT, Han CF, Shen MX, Sun HY, et al. Severe acute respiratory syndrome Coronavirus 2 (SARS-CoV-2) infection during pregnancy in China: a retrospective cohort study. (medRxiv preprint, posted April 11, 2020). Available at: https://www.medrxiv.org/content/10.1101/2020.04.07.20053744v1 (Accessed April 15, 2023). doi: 10.1101/2020.04.07.20053744
38. Kilic T, Kilic S, Berber NK, Gunduz A, Ersoy Y. Investigation of SARS-CoV-2 RNA in milk produced by women with COVID-19 and follow-up of their infants: a preliminary study. Int J Clin Pract. (2021) 75(7):e14175. doi: 10.1111/ijcp.14175
39. Thanigainathan S, Kaliyaperumal V, Sivanandan S, Rengaraj S, Dhodapkar R, Bethou A. Is SARS-CoV-2 transmitted through breastfeeding? Indian J Pediatr. (2021) 88(8):800–1. doi: 10.1007/s12098-021-03681-0
40. Li Y, Zhao R, Zheng S, Chen X, Wang J, Sheng X, et al. Lack of vertical transmission of severe acute respiratory syndrome coronavirus 2, China. Emerg Infect Dis. (2020) 26(6):1335–6. doi: 10.3201/eid2606.200287
41. Perrone S, Giordano M, Meoli A, Deolmi M, Marinelli F, Messina G, et al. Lack of viral transmission to preterm newborn from a COVID-19 positive breastfeeding mother at 11 days postpartum. J Med Virol. (2020) 92(11):2346–7. doi: 10.1002/jmv.26037
42. Kalafat E, Yaprak E, Cinar G, Varli B, Ozisik S, Uzun C, et al. Lung ultrasound and computed tomographic findings in pregnant woman with COVID-19. Ultrasound Obst Gyn. (2020) 55(6):835–7. doi: 10.1002/uog.22034
43. Salvatori G, De Rose DU, Concato C, Alario D, Olivini N, Dotta A, et al. Managing COVID-19-positive maternal-infant dyads: an Italian experience. Breastfeed Med. (2020) 15(5):347–8. doi: 10.1089/bfm.2020.0095
44. AlZaghal LA, AlZaghal N, Alomari SO, Obeidat N, Obeidat B, Hayajneh WA. Multidisciplinary team management and cesarean delivery for a Jordanian woman infected with SARS-COV-2: a case report. Case Rep Womens Health. (2020) 27:e00212. doi: 10.1016/j.crwh.2020.e00212
45. Marín Gabriel MÁ, Malalana Martínez AM, Marín Martínez ME, Anel Pedroche J. Negative transmission of SARS-CoV-2 to hand-expressed colostrum from SARS-CoV-2-positive mothers. Breastfeed Med. (2020) 15(8):492–4. doi: 10.1089/bfm.2020.0183
46. Buonsenso D, Costa S, Sanguinetti M, Cattani P, Posteraro B, Marchetti S, et al. Neonatal late onset infection with severe acute respiratory syndrome coronavirus 2. Am J Perinat. (2020) 37(8):869–72. doi: 10.1055/s-0040-1710541
47. Krogstad P, Contreras D, Ng H, Tobin N, Chambers CD, Bertrand K, et al. No evidence of infectious SARS-CoV-2 in human milk: analysis of a cohort of 110 lactating women. medRxiv. (2021). doi: 10.1101/2021.04.05.21254897
48. Hall M, Hölbfer S, Endress D, Aicher G, Drechsel-Burkhard G, Maier B. One year into the SARS-CoV-2 pandemic: perinatal outcome and data on the transmission of 116 pregnant women. J Perinat Med. (2022) 50(4):404–6. doi: 10.1515/jpm-2021-0271
49. Takahashi K, Sato T, Kamide T, Hoshina T, Kanuka H, Kumazawa K, et al. Perinatal management of a pregnant woman with COVID-19: a case report from Japan. Taiwan J Obstet Gyne. (2022) 61(2):378–81. doi: 10.1016/j.tjog.2022.02.033
50. Fan C, Lei D, Fang C, Li C, Wang M, Liu Y, et al. Perinatal transmission of 2019 coronavirus disease-associated severe acute respiratory syndrome coronavirus 2: should we worry? Clin Infect Dis. (2021) 72(5):862–4. doi: 10.1093/cid/ciaa226
51. Liu W, Liu Y, Liu Z, Hong C, Liu J, Luo S, et al. A case study supporting lack of SARS-CoV-2 spread to a 3-month old infant through exclusive breastfeeding. J Hum Lact. (2021) 37(2):269–72. doi: 10.1177/0890334421991072
52. Chu H, Li J, Yan J, Bai T, Schnabl B, Zou L, et al. Persistent SARS-CoV-2 RNA positive in feces but negative in breastmilk: a case report of COVID-19 in a breastfeeding patient. Front Med (Lausanne). (2020) 7:562700. doi: 10.3389/fmed.2020.562700
53. Dong L, Tian J, He S, Zhu C, Wang J, Liu C, et al. Possible vertical transmission of SARS-CoV-2 from an infected mother to her newborn. JAMA-J AM Med Assoc. (2020) 323(18):1846–8. doi: 10.1001/jama.2020.4621
54. Mattar CN, Kalimuddin S, Sadarangani SP, Tagore S, Thain S, Thoon KC, et al. Pregnancy outcomes in COVID-19: a prospective cohort study in Singapore. Ann Acad Med Singap. (2020) 49(11):857–69. doi: 10.47102/annals-acadmedsg.2020437
55. Kirtsman M, Diambomba Y, Poutanen SM, Malinowski AK, Vlachodimitropoulou E, Parks WT, et al. Probable congenital SARS-CoV-2 infection in a neonate born to a woman with active SARS-CoV-2 infection. Can Med Assoc J. (2020) 192(24):E647–50. doi: 10.1503/cmaj.200821
56. Han MS, Seong MW, Heo EY, Park JH, Kim N, Shin S, et al. Sequential analysis of viral load in a neonate and her mother infected with severe acute respiratory syndrome coronavirus 2. Clin Infect Dis. (2020) 71(16):2236–9. doi: 10.1093/cid/ciaa447
57. Peng Z, Wang J, Mo Y, Duan W, Xiang G, Yi M, et al. Unlikely SARS-CoV-2 vertical transmission from mother to child: a case report. J Infect Public Heal. (2020) 13(5):818–20. doi: 10.1016/j.jiph.2020.04.004
58. Xiong X, Wei H, Zhang Z, Chang J, Ma X, Gao X, et al. Vaginal delivery report of a healthy neonate born to a convalescent mother with COVID-19. J Med Virol. (2020) 92(9):1657–9. doi: 10.1002/jmv.25857
59. Bastug A, Hanifehnezhad A, Tayman C, Ozkul A, Ozbay O, Kazancioglu S, et al. Virolactia in an asymptomatic mother with COVID-19. Breastfeed Med. (2020) 15(8):488–91. doi: 10.1089/bfm.2020.0161
60. Schoenmakers S, Snijder P, Verdijk RM, Kuiken T, Kamphuis SSM, Koopman LP, et al. Severe acute respiratory syndrome coronavirus 2 placental infection and inflammation leading to fetal distress and neonatal multi-organ failure in an asymptomatic woman. J Pediat Inf Dis Soc. (2021) 10(5):556–61. doi: 10.1093/jpids/piaa153
61. Sharma R, Seth S, Sharma R, Yadav S, Mishra P, Mukhopadhyay S. Perinatal outcome and possible vertical transmission of coronavirus disease 2019: experience from North India. Clin Exp Pediatr. (2021) 64(5):239–46. doi: 10.3345/cep.2020.01704
62. Luo QQ, Xia L, Yao DJ, Wu M, Wang HB, Luo MH, et al. Breastfeeding in mothers with COVID-19: insights from laboratory tests and follow-up from early outbreak of the pandemic in China. J Womens Health. (2021) 30(11):1546–55. doi: 10.1089/jwh.2020.8978
63. Dong Y, Chi X, Hai H, Sun L, Zhang M, Xie WF, et al. Antibodies in the breast milk of a maternal woman with COVID-19. Emerg Microbes Infect. (2020) 9(1):1467–9. doi: 10.1080/22221751.2020.1780952
64. De Socio GV, Malincarne L, Arena S, Troiani S, Benedetti S, Camilloni B, et al. Delivery in asymptomatic Italian woman with SARS-CoV-2 infection. Mediterr J Hematol Infect Dis. (2020) 12(1):e2020033. doi: 10.4084/MJHID.2020.033
65. Young BE, Seppo AE, Diaz N, Rosen-Carole C, Nowak-Wegrzyn A, Cruz Vasquez JM, et al. Association of human milk antibody induction, persistence, and neutralizing capacity with SARS-CoV-2 infection vs. mRNA vaccination. JAMA Pediatr. (2022) 176(2):159–68. doi: 10.1001/jamapediatrics.2021.4897
66. Calabretto M, D’Alisa R, Faraone S, Mazzuti L, Pecorini F, Turriziani O. Detection of SARS-CoV-2 RNA and antibodies in breast milk of infected mothers. J Med Virol. (2023) 95(1):e28142. doi: 10.1002/jmv.28142
67. Pace RM, Williams JE, Järvinen KM, Meehan CL, Martin MA, Ley SH, et al. Milk from women diagnosed with COVID-19 does not contain SARS-CoV-2 RNA but has persistent levels of SARS-CoV-2-specific IgA antibodies. Front Immunol. (2021) 12:801797. doi: 10.3389/fimmu.2021.801797
68. Bäuerl C, Randazzo W, Sánchez G, Selma-Royo M, García Verdevio E, Martínez L, et al. SARS-CoV-2 RNA and antibody detection in breast milk from a prospective multicentre study in Spain. Arch Dis Child-Fetal. (2022) 107(2):216–21. doi: 10.1136/archdischild-2021-322463
69. Walczak A, Wilks K, Shakhovskoy R, Baird T, Schlebusch S, Taylor C, et al. COVID-19 in a complex obstetric patient with cystic fibrosis. Infect Dis Health. (2020) 25(4):239–41. doi: 10.1016/j.idh.2020.07.002
70. Yu Y, Li Y, Hu Y, Li B, Xu J. Breastfed 13 month-old infant of a mother with COVID-19 pneumonia: a case report. Int Breastfeed J. (2020) 15(1):68. doi: 10.1186/s13006-020-00305-9
71. Gao X, Wang S, Zeng W, Chen S, Wu J, Lin X, et al. Clinical and immunologic features among COVID-19-affected mother-infant pairs: antibodies to SARS-CoV-2 detected in breast milk. New Microbes New Infect. (2020) 37:100752. doi: 10.1016/j.nmni.2020.100752
72. Fenizia C, Biasin M, Cetin I, Vergani P, Mileto D, Spinillo A, et al. Analysis of SARS-CoV-2 vertical transmission during pregnancy. Nat Commun. (2020) 11(1):5128. doi: 10.1038/s41467-020-18933-4
73. Pace RM, Williams JE, Järvinen KM, Belfort MB, Pace CDW, Lackey KA, et al. Characterization of SARS-CoV-2 RNA, antibodies, and neutralizing capacity in milk produced by women with COVID-19. mBio. (2021) 12(1):1–11. doi: 10.1128/mBio.03192-20
74. Demers-Mathieu V, DaPra C, Medo E. Comparison of severe acute respiratory syndrome coronavirus 2-specific antibodies’ binding capacity between human milk and serum from coronavirus disease 2019-recovered women. Breastfeed Med. (2021) 16(5):393–401. doi: 10.1089/bfm.2020.0381
75. Conti MG, Terreri S, Piano Mortari E, Albano C, Natale F, Boscarino G, et al. Immune response of neonates born to mothers infected with SARS-CoV-2. JAMA Netw Open. (2021) 4(11):e2132563. doi: 10.1001/jamanetworkopen.2021.32563
76. van Keulen BJ, Romijn M, Bondt A, Dingess KA, Kontopodi E, van der Straten K, et al. Human milk from previously COVID-19-infected mothers: the effect of pasteurization on specific antibodies and neutralization capacity. Nutrients. (2021) 13(5):1–14. doi: 10.3390/nu13051645
77. Lebrão CW, Cruz MN, Silva MHD, Dutra LV, Cristiani C, Affonso Fonseca FL, et al. Early identification of IgA anti-SARSCoV-2 in milk of mother with COVID-19 infection. J Hum Lact. (2020) 36(4):609–13. doi: 10.1177/0890334420960433
78. Favara DM, Ceron-Gutierrez ML, Carnell GW, Heeney JL, Corrie P, Doffinger R. Detection of breastmilk antibodies targeting SARS-CoV-2 nucleocapsid, spike and receptor-binding-domain antigens. Emerg Microbes Infect. (2020) 9(1):2728–31. doi: 10.1080/22221751.2020.1858699
79. Fox A, Marino J, Amanat F, Krammer F, Hahn-Holbrook J, Zolla-Pazner S, et al. Robust and specific secretory IgA against SARS-CoV-2 detected in human milk. iScience. (2020) 23(11):101735. doi: 10.1016/j.isci.2020.101735
80. Juncker HG, Romijn M, Loth VN, Ruhé EJM, Bakker S, Kleinendorst S, et al. Antibodies against SARS-CoV-2 in human milk: milk conversion rates in the Netherlands. J Hum Lact. (2021) 37(3):469–76. doi: 10.1177/08903344211018185
81. Szczygioł P, Łukianowski B, Kościelska-Kasprzak K, Jakuszko K, Bartoszek D, Krajewska M, et al. Antibodies in the breastmilk of COVID-19 recovered women. BMC Pregnancy Childbirth. (2022) 22(1):635. doi: 10.1186/s12884-022-04945-z
82. Bode L, Bertrand K, Najera JA, Furst A, Honerkamp-Smith G, Shandling AD, et al. Characterization of SARS-CoV-2 antibodies in human milk from 21 women with confirmed COVID-19 infection. Pediatr Res. (2023) 93(6):1626–33. doi: 10.1038/s41390-022-02360-w
83. Bobik TV, Kostin NN, Skryabin GA, Tsabai PN, Simonova MA, Knorre VD, et al. Epitope-specific response of human milk immunoglobulins in COVID-19 recovered women. Pathogens. (2021) 10(6):1–9. doi: 10.3390/pathogens10060705
84. Duncombe CJ, McCulloch DJ, Shuey KD, Logue JK, Franko NM, Wolf CR, et al. Dynamics of breast milk antibody titer in the six months following SARS-CoV-2 infection. J Clin Virol. (2021) 142:104916. doi: 10.1016/j.jcv.2021.104916
85. Juncker HG, Romijn M, Loth VN, Caniels TG, de Groot CJM, Pajkrt D, et al. Human milk antibodies against SARS-CoV-2: a longitudinal follow-up study. J Hum Lact. (2021) 37(3):485–91. doi: 10.1177/08903344211030171
86. Dutra LV, Silva MH, Lebrão CW, Affonso-Fonseca FL, Suano-Souza FI. Impact of the presence anti-SARS-CoV-2 IgA in the colostrum of women infected by COVID-19 during the pregnancy in neonatal clinical outcomes: a cross-sectional study. Matern Child Hlth J. (2023) 27(4):737–46. doi: 10.1007/s10995-022-03553-9
87. Narayanaswamy V, Pentecost B, Alfandari D, Chin E, Minor K, Kastrinakis A, et al. Humoral and cell-mediated immune response in colostrum from women diagnosed positive for SARS-CoV-2. Breastfeed Med. (2021) 16(12):987–94. doi: 10.1089/bfm.2021.0082
88. Wachman EM, Snyder-Cappione J, Devera J, Boateng J, Dhole Y, Clarke K, et al. Maternal, infant, and breast milk antibody response following COVID-19 infection in early versus late gestation. Pediatr Infect Dis J. (2023) 42(3):e70–6. doi: 10.1097/INF.0000000000003802
89. Woelfel R, Corman VM, Guggemos W, Seilmaier M, Zange S, Mueller MA. Clinical presentation and virological assessment of hospitalized cases of coronavirus disease 2019 in a travel-associated transmission cluster. medRxiv. (2020). doi: 10.1101/2020.03.05.20030502
90. Favre G, Pomar L, Qi X, Nielsen-Saines K, Musso D, Baud D. Guidelines for pregnant women with suspected SARS-CoV-2 infection. Lancet Infect Dis. (2020) 20(6):652–3. doi: 10.1016/S1473-3099(20)30157-2
91. Bai Y, Yao L, Wei T, Tian F, Jin DY, Chen L, et al. Presumed asymptomatic carrier transmission of COVID-19. JAMA-J Am Med Assoc. (2020) 323(14):1406–7. doi: 10.1001/jama.2020.2565
92. Jackson CB, Farzan M, Chen B, Choe H. Mechanisms of SARS-CoV-2 entry into cells. Nat Rev Mol Cell Bio. (2022) 23(1):3–20. doi: 10.1038/s41580-021-00418-x
93. Lu R, Zhao X, Li J, Niu P, Yang B, Wu H, et al. Genomic characterisation and epidemiology of 2019 novel coronavirus: implications for virus origins and receptor binding. Lancet. (2020) 395(10224):565–74. doi: 10.1016/S0140-6736(20)30251-8
94. Goad J, Rudolph J, Rajkovic A. Female reproductive tract has low concentration of SARS-CoV2 receptors. PLoS One. (2020) 15(12):e0243959. doi: 10.1371/journal.pone.0243959
95. Belitsky V. Children infect mothers in AIDS outbreak at a soviet hospital. Nature. (1989) 337(6207):493. doi: 10.1038/337493a0
96. Little KM, Kilmarx PH, Taylor AW, Rose CE, Rivadeneira ED, Nesheim SR. A review of evidence for transmission of HIV from children to breastfeeding women and implications for prevention. Pediatr Infect Dis J. (2012) 31(9):938–42. doi: 10.1097/INF.0b013e318261130f
97. Sissoko D, Keïta M, Diallo B, Aliabadi N, Fitter DL, Dahl BA, et al. Ebola virus persistence in breast milk after no reported illness: a likely source of virus transmission from mother to child. Clin Infect Dis. (2017) 64(4):513–6. doi: 10.1093/cid/ciw793
98. Lackey KA, Pace RM, Williams JE, Meehan CL, McGuire MA, McGuire MK. SARS-CoV-2 and human milk: what is the evidence? Matern Child Nutr. (2020) 16(4):e13032. doi: 10.1111/mcn.13032
99. John-Stewart G, Mbori-Ngacha D, Ekpini R, Janoff EN, Nkengasong J, Read JS, et al. Breast-feeding and transmission of HIV-1. J Acq Immun Def Synd. (2004) 35(2):196–202. doi: 10.1097/00126334-200402010-00015
100. Golden B, Cooper E, Cundall D, Moy R. Effect of zidovudine on perinatal HIV-1 transmission and maternal viral load. Lancet. (1999) 354(9173):158–9. doi: 10.1016/s0140-6736(05)75286-7
101. Trypsteen W, Van Cleemput J, Snippenberg WV, Gerlo S, Vandekerckhove L. On the whereabouts of SARS-CoV-2 in the human body: a systematic review. Plos Pathog. (2020) 16(10):e1009037. doi: 10.1371/journal.ppat.1009037
102. Venkatakrishnan AJ, Puranik A, Anand A, Zemmour D, Yao X, Wu X, et al. Knowledge synthesis of 100 million biomedical documents augments the deep expression profiling of coronavirus receptors. Elife. (2020) 9:1–25. doi: 10.7554/eLife.58040
103. Hennighausen L, Lee HK. Activation of the SARS-CoV-2 receptor Ace2 through JAK/STAT-dependent enhancers during pregnancy. Cell Rep. (2020) 32(13):108199. doi: 10.1016/j.celrep.2020.108199
104. Fan H, Hong B, Luo Y, Peng Q, Wang L, Jin X, et al. The effect of whey protein on viral infection and replication of SARS-CoV-2 and pangolin coronavirus in vitro. Signal Transduct Tar. (2020) 5(1):275. doi: 10.1038/s41392-020-00408-z
105. Habib HM, Ibrahim S, Zaim A, Ibrahim WH. The role of iron in the pathogenesis of COVID-19 and possible treatment with lactoferrin and other iron chelators. Biomed Pharmacother. (2021) 136:111228. doi: 10.1016/j.biopha.2021.111228
106. Morniroli D, Consales A, Crippa BL, Vizzari G, Ceroni F, Cerasani J, et al. The antiviral properties of human milk: a multitude of defence tools from mother nature. Nutrients. (2021) 13(2):1–7. doi: 10.3390/nu13020694
107. Sterlin D, Mathian A, Miyara M, Mohr A, Anna F, Claër L, et al. IgA dominates the early neutralizing antibody response to SARS-CoV-2. Sci Transl Med. (2021) 13(577):1–10. doi: 10.1126/scitranslmed.abd2223
108. Martínez-Fleta P, Alfranca A, González-Álvaro I, Casasnovas JM, Fernández-Soto D, Esteso G, et al. SARS-CoV-2 cysteine-like protease antibodies can be detected in serum and saliva of COVID-19-seropositive individuals. J Immunol. (2020) 205(11):3130–40. doi: 10.4049/jimmunol.2000842
109. Mallon PWG, Tinago W, Leon AG, McCann K, Kenny G, McGettrick P, et al. Dynamic change and clinical relevance of postinfectious SARS-CoV-2 antibody responses. Open Forum Infect Dis. (2021) 8(8):1–8. doi: 10.1093/ofid/ofab122
110. Low JM, Low YW, Zhong Y, Lee CYC, Chan M, Ng NBH, et al. Titres and neutralising capacity of SARS-CoV-2-specific antibodies in human milk: a systematic review. Arch dis Child-Fetal. (2022) 107(2):174–80. doi: 10.1136/archdischild-2021-322156
111. Dan JM, Mateus J, Kato Y, Hastie KM, Yu ED, Faliti CE, et al. Immunological memory to SARS-CoV-2 assessed for up to 8 months after infection. Science. (2021) 371(6529):1–22. doi: 10.1126/science.abf4063
112. Falsaperla R, Leone G, Familiari M, Ruggieri M. COVID-19 vaccination in pregnant and lactating women: a systematic review. Expert Rev Vaccines. (2021) 20(12):1619–28. doi: 10.1080/14760584.2021.1986390
113. Van de Perre P. Transfer of antibody via mother’s milk. Vaccine. (2003) 21(24):3374–6. doi: 10.1016/s0264-410x(03)00336-0
114. Lan J, Ge J, Yu J, Shan S, Zhou H, Fan S, et al. Structure of the SARS-CoV-2 spike receptor-binding domain bound to the ACE2 receptor. Nature. (2020) 581(7807):215–20. doi: 10.1038/s41586-020-2180-5
115. Mantis NJ, Rol N, Corthesy B. Secretory IgA’s complex roles in immunity and mucosalhomeostasis in the gut. Mucosal Immunol. (2011) 4(6):603–11. doi: 10.1038/mi.2011.41
116. Hutchings AB, Helander A, Silvey KJ, Chandran K, Lucas WT, Nibert ML, et al. Secretory immunoglobulin A antibodies against the sigma1 outer capsid protein of reovirus type 1 lang prevent infection of mouse peyer’s patches. J Virol. (2004) 78(2):947–57. doi: 10.1128/jvi.78.2.947-957.2004
117. Demers-Mathieu V, Underwood MA, Beverly RL, Nielsen SD, Dallas DC. Comparison of human milk immunoglobulin survival during gastric digestion between preterm and term infants. Nutrients. (2018) 10(5):1–13. doi: 10.3390/nu10050631
118. French MA, Tjiam MC, Abudulai LN, Fernandez S. Antiviral functions of human immunodeficiency virus type 1 (HIV-1)-specific IgG antibodies: effects of antiretroviral therapy and implications for therapeutic HIV-1 vaccine design. Front Immunol. (2017) 8:780. doi: 10.3389/fimmu.2017.00780
119. Hurley WL, Theil PK. Perspectives on immunoglobulinsin colostrum and milk. Nutrients. (2011) 3(4):442–74. doi: 10.3390/nu3040442
120. Zinkernagel RM. Maternal antibodies, childhood infections, and autoimmune diseases. N Engl J Med. (2001) 345(18):1331–5. doi: 10.1056/NEJMra012493
121. Joseph NT, Dude CM, Verkerke HP, Irby LS, Dunlop AL, Patel RM, et al. Maternal antibody response, neutralizing potency, and placental antibody transfer after severe acute respiratory syndrome coronavirus 2 (SARS-CoV-2) infection. Obstet Gynecol. (2021) 138(2):189–97. doi: 10.1097/AOG.0000000000004440
122. Wang X, Yang P, Zheng J, Liu P, Wei C, Guo J, et al. Dynamic changes of acquired maternal SARS-CoV-2 IgG in infants. Sci Rep. (2021) 11(1):8021. doi: 10.1038/s41598-021-87535-x
123. Abiri B, Guest PC, Vafa M. How do we manage breastfeeding during the COVID-19 pandemic. Adv Exp Med Biol. (2021) 1327:129–37. doi: 10.1007/978-3-030-71697-4_10
124. Martin CR, Ling PR, Blackburn GL. Review of infant feeding: key features of breast milk and infant formula. Nutrients. (2016) 8(5):1–11. doi: 10.3390/nu8050279
125. Eidelman AI. Breastfeeding and the use of human milk: an analysis of the American academy of pediatrics 2012 breastfeeding policy statement. Breastfeed Med. (2012) 7(5):323–4. doi: 10.1089/bfm.2012.0067
126. Vassilopoulou E, Feketea G, Koumbi L, Mesiari C, Berghea EC, Konstantinou GN. Breastfeeding and COVID-19: from nutrition to immunity. Front Immunol. (2021) 12:661806. doi: 10.3389/fimmu.2021.661806
127. Mohandas S, Pannaraj PS. Beyond the bacterial microbiome: virome of human milk and effects on the developing infant. Nestle Nutr Inst Workshop Ser. (2020) 94:86–93. doi: 10.1159/000504997
128. Shoemark DK, Colenso CK, Toelzer C, Gupta K, Sessions RB, Davidson AD, et al. Molecular simulations suggest vitamins, retinoids and steroids as ligands of the free fatty acid pocket of the SARS-CoV-2 spike protein*. Angew Chem int Edit. (2021) 60(13):7098–110. doi: 10.1002/anie.202015639
129. Prentice A, Jarjou LM, Drury PJ, Dewit O, Crawford MA. Breast-milk fatty acids of rural Gambian mothers: effects of diet and maternal parity. J Pediatr Gastr Nutr. (1989) 8(4):486–90. doi: 10.1097/00005176-198905000-00011
130. Brink LR, Chichlowski M, Pastor N, Thimmasandra Narayanappa A, Shah N. In the age of viral pandemic, can ingredients inspired by human milk and infant nutrition be repurposed to support the immune system. Nutrients. (2021) 13(3). doi: 10.3390/nu13030870
131. Sullivan SE, Thompson LA. Best practices for COVID-19-positive or exposed mothers-breastfeeding and pumping milk. JAMA Pediatr. (2020) 174(12):1228. doi: 10.1001/jamapediatrics.2020.3341
132. Drobni P, Näslund J, Evander M. Lactoferrin inhibits human papillomavirus binding and uptake in vitro. Antivir Res. (2004) 64(1):63–8. doi: 10.1016/j.antiviral.2004.05.005
133. Puddu P, Borghi P, Gessani S, Valenti P, Belardelli F, Seganti L. Antiviral effect of bovine lactoferrin saturated with metal ions on early steps of human immunodeficiency virus type 1 infection. Int J Biochem Cell B. (1998) 30(9):1055–62. doi: 10.1016/s1357-2725(98)00066-1
134. Superti F, Siciliano R, Rega B, Giansanti F, Valenti P, Antonini G. Involvement of bovine lactoferrin metal saturation, sialic acid and protein fragments in the inhibition of rotavirus infection. Biochim Biophys Acta. (2001) 1528(2–3):107–15. doi: 10.1016/s0304-4165(01)00178-7
135. Carvalho C, Casseb S, Gonçalves RB, Silva E, Gomes A, Vasconcelos P. Bovine lactoferrin activity against chikungunya and Zika viruses. J Gen Virol. (2017) 98(7):1749–54. doi: 10.1099/jgv.0.000849
136. Passali D, Damiani V, Cianfrone F, Passali GC, Bellussi LM, Ciprandi G. Lactoferrin: a potential candidate to fight respiratory infections in the pandemic COVID-19 era. J Biol Reg Homeos Ag. (2021) 35(1 Suppl 2):9–13. doi: 10.23812/21-1supp2-3
137. Kell DB, Heyden EL, Pretorius E. The biology of lactoferrin, an iron-binding protein that can help defend against viruses and bacteria. Front Immunol. (2020) 11:1221. doi: 10.3389/fimmu.2020.01221
138. Chirico G, Marzollo R, Cortinovis S, Fonte C, Gasparoni A. Antiinfective properties of human milk. J Nutr. (2008) 138(9):1801S–6S. doi: 10.1093/jn/138.9.1801S
139. Dawod B, Marshall JS. Cytokines and soluble receptors in breast milk as enhancers of oral tolerance development. Front Immunol. (2019) 10:16. doi: 10.3389/fimmu.2019.00016
140. Aparicio M, Browne PD, Hechler C, Beijers R, Rodríguez JM, de Weerth C, et al. Human milk cortisol and immune factors over the first three postnatal months: relations to maternal psychosocial distress. PLoS One. (2020) 15(5):e0233554. doi: 10.1371/journal.pone.0233554
141. Shamir R. The benefits of breast feeding. Nestle Nutr Inst Workshop Ser. (2016) 86:67–76. doi: 10.1159/000442724
142. Császár-Nagy N, Bókkon I. Mother-newborn separation at birth in hospitals: a possible risk for neurodevelopmental disorders? Neurosci Biobehav Rev. (2018) 84:337–51. doi: 10.1016/j.neubiorev.2017.08.013
143. Bergman NJ. Birth practices: maternal-neonate separation as a source of toxic stress. Birth Defects Res. (2019) 111(15):1087–109. doi: 10.1002/bdr2.1530
144. Woolhouse H, James J, Gartland D, McDonald E, Brown SJ. Maternal depressive symptoms at three months postpartumand breastfeeding rates at six months postpartum: implications for primary care in a prospective cohort studyof primiparous women in Australia. Women Birth. (2016) 29:381–7. doi: 10.1016/j.wombi.2016.05.008
145. Dieterich CM, Felice JP, O’Sullivan E, Rasmussen KM. Breastfeeding and health outcomes for the mother-infant dyad. Pediatr Clin N Am. (2013) 60(1):31–48. doi: 10.1016/j.pcl.2012.09.010
146. Rameez RM, Sadana D, Kaur S, Ahmed T, Patel J, Khan MS, et al. Association of maternal lactation with diabetes and hypertension: a systematic review and meta-analysis. JAMA Netw Open. (2019) 2(10):e1913401. doi: 10.1001/jamanetworkopen.2019.13401
Keywords: breast milk, breastfeeding, SARS-CoV-2, COVID-19, lactation, vertical transmission, antibodies
Citation: He Y-f, Liu J-q, Hu X-d, Li H-m, Wu N, Wang J and Jiang Z-g (2023) Breastfeeding vs. breast milk transmission during COVID-19 pandemic, which is more important? Front. Pediatr. 11:1253333. doi: 10.3389/fped.2023.1253333
Received: 6 July 2023; Accepted: 23 August 2023;
Published: 6 September 2023.
Edited by:
Elizabeth Secord, Wayne State University, United StatesReviewed by:
Tudor Lucian Pop, University of Medicine and Pharmacy Iuliu Hatieganu, Romania© 2023 He, Liu, Hu, Li, Wu, Wang and Jiang. This is an open-access article distributed under the terms of the Creative Commons Attribution License (CC BY). The use, distribution or reproduction in other forums is permitted, provided the original author(s) and the copyright owner(s) are credited and that the original publication in this journal is cited, in accordance with accepted academic practice. No use, distribution or reproduction is permitted which does not comply with these terms.
*Correspondence: Yan-fei He aGV5YW5mZWlsY0AxNjMuY29t
Disclaimer: All claims expressed in this article are solely those of the authors and do not necessarily represent those of their affiliated organizations, or those of the publisher, the editors and the reviewers. Any product that may be evaluated in this article or claim that may be made by its manufacturer is not guaranteed or endorsed by the publisher.
Research integrity at Frontiers
Learn more about the work of our research integrity team to safeguard the quality of each article we publish.