- 1Department of Immunology, ICMR—National Institute for Research in Tuberculosis, Chennai, India
- 2National Institutes of Health—International Center for Excellence in Research, Chennai, India
- 3Department of Clinical Research, ICMR—National Institute for Research in Tuberculosis, Chennai, India
- 4Department of Pulmonology, Institute of Child Health and Hospital for Children, Chennai, India
- 5General Pediatrics, Dr. Mehta’s Children’s Hospital, Chennai, India
- 6General Pediatrics, Rainbow Children’s Hospital, Chennai, India
- 7Laboratory of Parasitic Diseases, National Institute of Allergy and Infectious Diseases, National Institutes of Health, Bethesda, MD, United States
Background: Multisystem inflammatory syndrome (MIS) in children is considered to be a post-infectious complication of COVID-19. T-cell responses in children with this condition have not been well-studied.
Methods: We aimed to study the immune responses in children with MIS in comparison to children with acute COVID-19 and children with other infections. Whole blood was stimulated with severe acute respiratory syndrome coronavirus-2 (SARS-CoV-2)–specific antigens and flow cytometry was performed to examine CD4+ and CD8+ T-cell responses.
Results: Children with MIS had higher frequencies of CD4+ and CD8+ T cells expressing cytokines at baseline and upon SARS-CoV-2 antigen–specific stimulation in comparison to children with COVID-19 and/or other infections. Children with COVID-19 also exhibited higher frequencies of CD4+ and CD8+ T cells expressing cytokines at baseline and upon SARS-CoV-2 antigen–specific stimulation in comparison to children with other infections. At 6–9 months following treatment and recovery, this enhanced response against SARS-CoV-2 antigens was down modulated in children with MIS.
Conclusion: Our study, therefore, provides evidence of enhanced activation of CD4+ and CD8+ T-cell responses in children with MIS and reversal following recovery.
Introduction
Severe acute respiratory syndrome coronavirus-2 (SARS-CoV-2) caused the pandemic that surfaced at the end of 2019, known globally as COVID-19. The disease resulted in a high rate of morbidity and mortality across the globe. Although, the infection rate in children was similar to that of adults with respect to viral load (1), they were found largely to have less severe disease, perhaps as a result of better immune function or differential expression of the angiotensin-converting enzyme 2 (ACE2) receptors (2). Multisystem inflammatory syndrome (MIS) is a life-threatening inflammatory condition that affects the heart, lungs, kidneys, brain, skin, eyes, and gastrointestinal organs of children (MIS-C) and adolescents (MIS-A) (3). Their clinical presentations are similar to that of patients with Kawasaki disease (KD), toxic shock syndrome (TSS), and secondary hemophagocytic lymphohistiocytosis (SHLH) (4, 5). The manifestations of MIS-C were reported in pediatric cases in COVID-19 hotspots, and the Centers for Disease Control and Prevention (CDC) reported this condition in April 2020 (3).
The causes of MIS-C are unclear, but it is thought to be due to immune dysregulation. Initially, it was hypothesized that MIS-C was related to post-COVID-19 immune dysregulation based on laboratory reports (6). A major fraction of the children diagnosed with MIS has been reported to be seropositive for SARS-CoV-2-specific antibodies. However, most cases remain negative for viral RNA in RT-PCR (7, 8). The presence of autoantibodies with multiple antigenic targets has also been reported in acute MIS-C (9), though the role of autoantibodies has not been shown to be causal as of yet (10). Moreover, a genetic component to the occurrence of MIS-C has also been recently described (11, 12).
A few studies have focused on identifying the immune parameters at the cellular level that might help explain the differences between MIS-C and those from other groups (8, 10). Studies of components of the innate immune response in MIS-C showed marked neutrophil activation, degranulation signatures, and neutrophil extracellular traps (13). Rajamanickam et al. explored cellular biomarkers for MIS-C, inclusive of B cells, dendritic cells, monocytes, and T cells, which significantly distinguish MIS-C from other diseases that have overlapping clinical symptoms (14). Moreover, HLA-genotype alterations in the T cells of children with MIS were reported as MIS-C biomarkers (15). Similarly, there are studies demonstrating that following T-cell stimulation by SARS-CoV-2-specific antigens in children with MIS, there was a dysregulated increase of Type 1, Type 2, Type 17, and other pro-inflammatory cytokines and chemokines when compared to the healthy controls (16). It was also reported that T cells with specific β-chain receptors are minimally responsive to SARS-CoV-2-specific antigens but do respond to non-viral antigens in the majority of the children with MIS (17, 18). Although reported as a post-infectious complication, MIS-C has been found to have its own distinct features when compared to the acute SARS-CoV-2 infection. In most of the previously published studies, the comparison was either between the pediatric or adult COVID-19 groups or the healthy controls. In this study, we have compared children with MIS with other infections that had similar clinical presentations as MIS-C. Our study focused on identifying differences in the immunological responses to SARS-CoV-2 antigen stimulation at a cellular level in three patient groups—MIS-C, acute COVID-19, and other infections.
Materials and methods
Ethics statement
Informed consent was obtained from parents/guardians of all children along with assent where appropriate. The Internal Ethics Committee (IEC) of the participating institutes approved the study.
Study population
Children, both male and female, between the ages of 12 months and 15 years, with clinical presentations of MIS-C, acute COVID-19, other infectious diseases [dengue fever, scrub typhus fever, and Salmonella typhi infection (enteric fever)] admitted to the Institute of Child Health, Dr. Mehta's Children Hospital, and Rainbow Children's Hospital, between December 2020 and May 2021, were included in this study. The study population was classified into three groups: MIS-C (n = 15), acute COVID-19 (n = 10), and other infectious diseases (n = 10). In the MIS-C group, eight children were evaluated 6–9 months following release from the hospital. The demographic and epidemiological data for this cohort have been previously reported (16) (Table 1).
Sample collection
Blood was collected in heparin tubes (BD Biosciences) and processed within 4 h of collection. Sampling at baseline in all children was done prior to them being given any form of immunomodulatory treatment. Study staff involved in immunological assays was blinded to all clinical data, and all the collected samples from the different collaborating hospitals were processed at the NIH-ICER Lab. Acute COVID-19 disease and severity of COVID-19 were defined according to the Ministry of Health and Family Welfare (MoHFW) guidelines. Diagnosis and treatment of MIS-C was done according to the CDC definition for MIS-C. Dengue fever was confirmed by either serology or NS1 positivity. Scrub typhus was confirmed by serology. Confirmed culture was used to diagnose enteric fever.
In vitro culture
Whole-blood cell cultures were performed to determine the intracellular levels of cytokines. Briefly, whole blood was diluted 1:1 with an RPMI-1640 medium, supplemented with penicillin/streptomycin (100 U/100 mg/ml), L-glutamine (2 mM), and (4-(2-hydroxyethyl)-1-piperazineethanesulfonic acid) (10 mM) (all from Invitrogen, Carlsbad, CA, USA), and distributed in 12-well tissue culture plates (Costar). The cultures were then stimulated with SARS-CoV-2 S-RBD (Receptor Binding Domain), SARS-CoV-2 ICL (Irradiated Cell Lysate), or Mycobacterium tuberculosis M.tb WCL (Whole Cell Lysate, H37Rv, BEI Resources) (non-viral antigen control) or media alone in the presence of the co-stimulatory molecules (CD49d/CD28) at 37° for 12 h. Brefeldin A (10 μg/ml) was added 4 h prior to harvest. After 12 h, centrifugation, red blood cell lysis, and washing were performed. The cells were then washed and permeabilized with BD Perm/Wash buffer (BD Biosciences) and stained with intracellular markers for an additional 60 min before washing and acquisition. Antibodies containing surface and intracellular markers were FITC-CD8 (SK1), APC R700-CD3 (UCHT1), BV510-CD4 (SK3), BV421-IL2 (5344.111), APC-TNF-α (6401.1111), and BV650-IL17A (N49-653), from BD Biosciences, as well as using PerCP-Cy5.5-IFN-γ (4S.B3) from eBioscience-Invitrogen. Flow cytometry was performed on a FACS Celesta flow cytometer with FACS Diva software v.7 (Becton Dickinson, Franklin Lakes, NJ, USA). The lymphocyte gating was set by forward and side scatter and at least ∼100,000 lymphocyte events were acquired. Data were collected and analyzed using Flow Jo 10.7.1 software (TreeStar Inc., Ashland, CA, USA). All data are depicted as frequencies of CD4+ and CD8+ T cells expressing cytokine(s) and other immune markers.
Statistical analyses
The data were analyzed statistically with the geometric mean of each group. The differences between the three groups were analyzed with the Kruskal–Wallis test and with Dunn's multiple comparisons test. The comparative analyses between baseline and post-treatment was done by applying the non-parametric Wilcoxon matched-pairs signed rank test. All the above analyses were performed by using GraphPad PRISM Version 9.4. (GraphPad Software, Inc., San Diego, CA, USA). The heat map representation showing hierarchical clusters was done with R by applying the Euclidean distance measure and Ward.d2 method after normalizing the frequencies of cytokine expressions by CD4+ and CD8+ T cells with the z-score.
Results
Enhanced frequencies of SARS-CoV-2-specific CD4+ Th1 and Th17 cells in MIS-C
To elucidate the impact of MIS-C on CD4+ T-cell responses, we measured the frequencies of CD4+ T cells expressing IFN-γ IL-2, TNF-α, and IL-17A at baseline (no stimulation) and following stimulation with either SARS-CoV-2-specific antigens or M.tb WCL. The gating strategy and a representative flow cytometry contour plot are shown in Supplementary Figure S1A. As shown in Figure 1A, at baseline, there were significantly increased frequencies of CD4+ T cells expressing IFN-γ IL-2, TNF-α, and IL-17A in MIS-C compared with children with COVID-19 or with other infectious diseases. In response to SARS-CoV-2 S-RBD (Figure 1B) and SARS-CoV-2 ICL (Figure 1C), we observed significantly increased frequencies of CD4+ T cells expressing IFN-γ, IL-2, and IL-17A in MIS-C compared to children with COVID-19 and other infectious diseases. In contrast, no significant differences were seen in the frequencies of CD4+ T cells expressing cytokines upon M.tb WCL stimulation (Figure 1D).
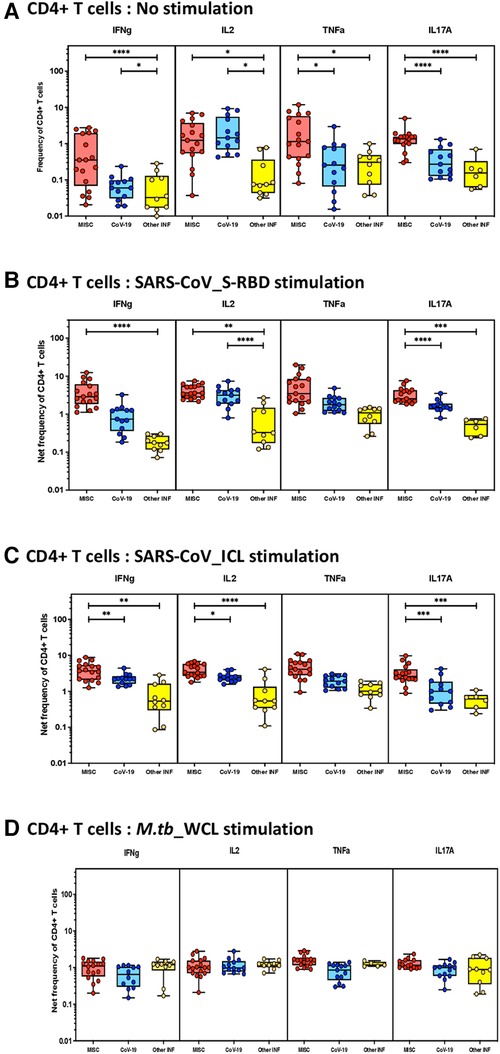
Figure 1. Children with MIS show enhanced frequencies of SARS-CoV-2-specific CD4+ Th1 and Th17 cells. The net frequencies of Th1 and Th17 cytokines by CD4+ T cells with or without SARS-CoV-2-antigenic stimulation were compared statistically among MIS-C, children with COVID-19, and other infectious diseases. (A) CD4+ T cells at baseline or no stimulation; (B) CD4+ T cells at SARS-CoV S-RBD stimulation; (C) CD4+ T cells at SARS-CoV ICL stimulation; and (D) CD4+ T cells at M.tb WCL stimulation. Each circle in the scatter plot represents a single variable from an individual and the line at the center of the scatter plot is the geometric mean. The p-values were calculated using the Kruskal–Wallis test to show the significance and were represented as *, **, ***, and ****.
Enhanced frequencies of SARS-CoV-2-specific CD8+ Tc1 and Tc17 cells in MIS-C
To elucidate the impact of MIS-C on CD8+ T-cell responses, we measured the frequencies of CD8+ T cells expressing IFN-γ, IL-2, TNF-α, and IL-17A at baseline (no stimulation) and following stimulation with either SARS-CoV-2-specific antigens or M.tb WCL. The gating strategy and a representative flow cytometry contour plot are shown in Supplementary Figure S1B. As shown in Figure 2A, at baseline, there were significantly increased frequencies of CD8+ T cells expressing IFN-γ, TNF-α, and IL-17A in MIS-C compared to children with COVID-19 and other infectious diseases. In response to SARS-CoV-2 S-RBD (Figure 2B) and SARS-CoV-2 ICL (Figure 2C), we observed significantly increased frequencies of CD8+ T cells expressing IFN-γ, IL-2, TNF-α, and IL-17A in MIS-C compared to children with COVID-19 and other infectious diseases. In contrast, no significant differences were seen in the frequencies of CD8+ T cells expressing cytokines upon M.tb WCL stimulation (Figure 2D). A sub-analysis was conducted to assess the differences in immune responses in the cases of MIS-C among the children aged less than 5 years (n = 7) and those greater than 5 years (n = 8). We focused on CD4+ and CD8+ T cells expressing IFN-γ, IL-2, TNF-α, and IL-17A both at baseline (no stimulation) and upon antigen stimulation. The results revealed moderate statistical differences in CD4+ T cells for IFN-γ expression under no stimulation conditions and in CD8+ T cells for IL-2 expression (no stimulation) and IL-17A expression (RBD stimulation). However, for the rest of the analyzed cytokines, no significant differences were observed among the younger and older children with MIS (see Supplementary Table S1 for detailed data).
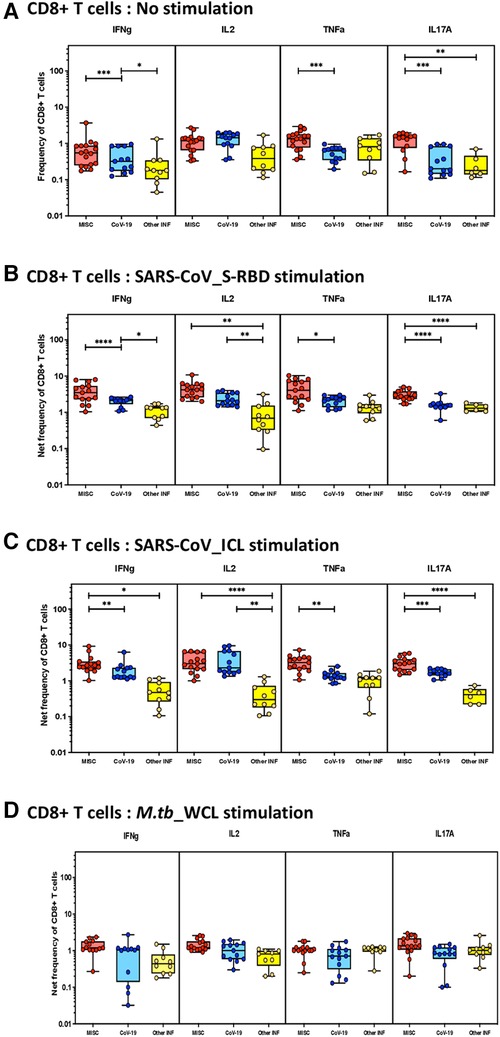
Figure 2. Children with MIS show enhanced frequencies of SARS-CoV-2-specific CD8+ Tc1 and Tc17 cells. The net frequencies of Tc1 and Tc17 cytokines by CD8+ T cells with or without SARS-CoV-2-antigenic stimulation were compared statistically among children with MIS, COVID-19, and other infectious diseases. (A) CD8+ T cells at baseline or no stimulation; (B) CD8+ T cells at SARS-CoV S-RBD stimulation; (C) CD8+ T cells at SARS-CoV ICL stimulation; and (D) CD8+ T cells at M.tb WCL stimulation. Each circle in the scatter plot represents a single variable from an individual and the line at the center of the scatter plot is the geometric mean. The p-values were calculated using the Kruskal–Wallis test to show the significance and were represented as *, **, ***, and ****.
T-cell signatures for MIS-C upon stimulation with SARS-CoV-2 antigen
To understand the differentiation of T cells upon SARS-CoV-2-specific antigen stimulation, a signature pattern was established for CD4+ Th1/17 and CD8+ Tc1/17 cytokines. The representative hierarchical cluster shows the distinction of MIS-C from the COVID-19 group and other infections group in both CD4+ and CD8+ T cells. The Th1/17 and Tc1/17 cytokine expressions were clustered separately between the study groups, and the signatures were seen with no stimulation and with stimulation of SARS-CoV-2 S-RBD and SARS-CoV-2 ICL antigens (Figures 3A,B).
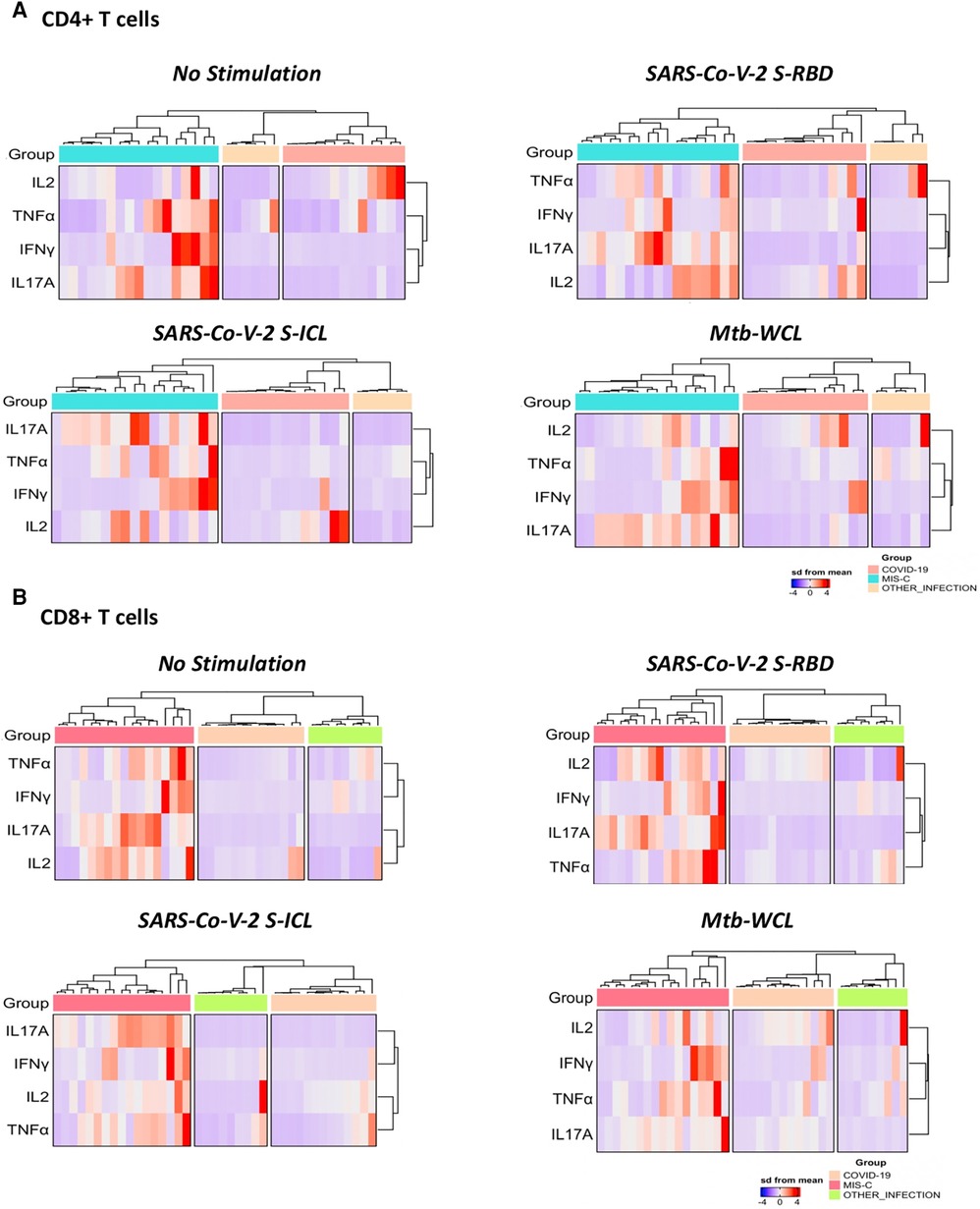
Figure 3. A signature profile of CD4+ T. (A) Cells expressing Th1/17 cytokines and CD8+ T. (B) Cells expressing Tc1/17 cytokines upon SARS-CoV-2-specific antigen stimulation in MIS-C, children with COVID-19, and children with other infectious diseases.
Antigen-specific CD4+ and CD8+ T-cell cytokine expressing frequencies are significantly diminished 6–9 months post recovery
To examine whether the elevated frequencies of CD4+ Th1 and Th17 cells are directly linked with MIS-C, we measured the frequencies of these CD4+ T cells in MIS-C before and after treatment/recovery (pre vs. post). As shown in Figure 4A, at 6–9 months following recovery, the frequencies of CD4+ Th1 and Th17 cells were significantly diminished compared with pre-treatment levels at both baseline (no stimulation) and as well as upon SARS-CoV-2 antigen stimulation. Similarly, as shown in Figure 4B, at 6–9 months following recovery, the frequencies of CD8+ Tc1 and Tc17 cells were significantly diminished compared with pre-treatment levels at both baseline (no stimulation) and as well as upon SARS-CoV-2 antigen stimulation.
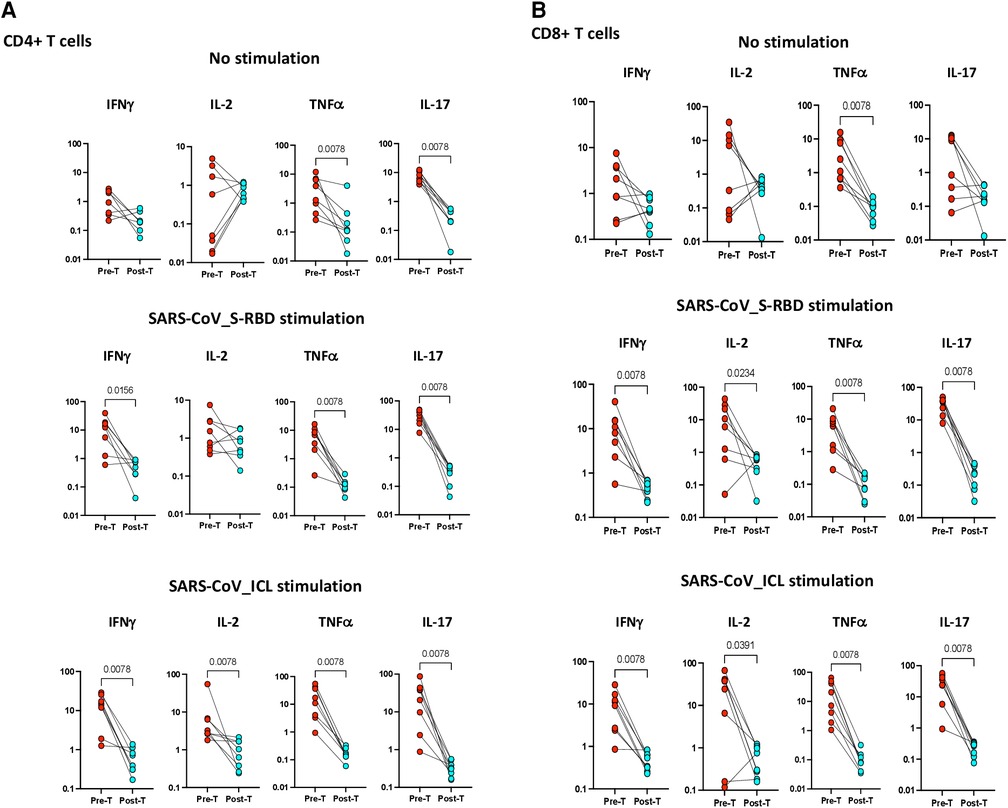
Figure 4. (A) Children with MIS show diminished frequencies of SARS-CoV-2-specific CD4+ Th1 and Th17 cells after treatment. The net frequencies of Th1 and Th17 cytokines by CD4+ T cells with or without SARS-CoV-2 antigenic stimulation were compared statistically between pre- and post-treatment of MIS-C. (Top) CD4+ T cells at baseline or no stimulation; (Middle) CD4+ T cells at SARS-CoV S-RBD stimulation; and (Bottom) CD4+ T cells at SARS-CoV ICL stimulation. Each circle in the scatter plot represents a single variable from an individual and the line connecting to the circle of the same individual from pre- to post-treatment. The p-values were calculated using the Wilcoxon test for matched pairs. (B) Children with MIS show diminished frequencies of SARS-CoV-2-specific CD8+ Tc1 and Tc17 cells after treatment. The net frequencies of Tc1 and Tc17 cytokines by CD8+ T cells with or without SARS-CoV-2 antigenic stimulation were compared statistically between pre- and post-treatment of MIS-C. (Top) CD8+ T cells at baseline or no stimulation; (Middle) CD8+ T cells at SARS-CoV S-RBD stimulation; and (Bottom) CD8+ T cells at SARS-CoV ICL stimulation. Each circle in the scatter plot represents a single variable from an individual and the line connecting to the circle of the same individual from pre- to post-treatment. The p-values were calculated using Wilcoxon test for matched pairs.
Discussion
The severe after-effects of COVID-19 infection sometimes manifest themselves as MIS-C that clinically overlaps with many inflammatory disorders (e.g., Kawasaki disease) (19). Our study focuses on the poorly understood immunologic phenomenon of MIS-C in association with COVID-19. We investigated T-cell responses to stimulation with SARS-CoV-2-specific antigens, since T cells are important for host protection. A modified immune pattern with cytokine storm, lymphopenia, innate and adaptive immune activation, and anti-viral and autoantibody production affecting physiological homeostasis are the characteristics of MIS-C (20). Previous studies have shown that children with MIS have presented with lymphopenia, which would thereby lead to a weakened immune system in the host (2). A cellular study on MIS-C immune signatures showcases reduced CD4+ and CD8+ T-cell memory subsets in children with MIS (14). Similarly, the downregulation of host-protecting T-cell responses was suggested as evidence of MIS-C and COVID-19 infection (21). Therefore, understanding the immune perturbations after COVID-19 infection may guide in delineating the pathogenesis of MIS-C.
In our study, children with MIS exhibited elevated CD4+ and CD8+ T cells expressing IFN-γ, IL2, TNF-α, and IL17A. The present study parallels that seen previously in which increased T-cell responses against SARS-CoV-2-specific peptides were observed in most children with MIS (17, 22). Also, the children with MIS have a more significant T-cell response against SARS-CoV-2 antigen than those without MIS (23). An immune profiling study by Vella et al. has shown the trend of lymphopenia with extreme T-cell activation and proliferation in children with MIS (24). The T cells expressing cytokines upon SARS-CoV-2-specific antigens were seen enhanced in MIS-C than in children and adults with COVID-19 infection alone (23). Similarly, another study subsequently reported CD4+ Th response and cytotoxic T-cell responses in the MIS-C cohort were elevated in comparison with children with COVID-19 (17). Previous studies from our lab have reported that immuno-phenotyping of the T-cell subsets showed a reduced CD4+ and CD8+ stem cell memory, central memory, effector memory, and terminal memory in children with MIS and reversal after recovery (14). However, a recent study by Rybkina et al. has shown that there are no statistical differences in the total CD4+ and CD8+ T-cell responses among acute MIS-C and children with COVID-19 (25).
Previously, our group has shown an increased level of cytokines in the antigen-specific culture supernatants including IFNγ, IL-2, IL-4, IL-13, and IL-17 in MIS-C in comparison with acute COVID-19 and other infectious diseases (16). However, in the current study, we have elucidated the antigen-specific cellular immune responses, and our results revealed that enhanced frequencies of SARS-CoV-2-specific CD4+ and CD8+ T cells expressing type 1 and type 17 cytokines are major characteristics of the MIS-C immune profile. In addition, in this current study, we compared the SARS-CoV-2-specific T-cell response in MIS-C to individuals presenting with other infections, such as dengue fever, enteric fever, and scrub typhus. The results clearly reveal that the enhanced antigen-specific response we observe in this study are only in MIS-C and children with COVID-19. In addition, we have also validated the specificity of antigenic response with the stimulation of M.tb-specific WCL antigen as control.
In most cases, MIS-C requires only symptomatic treatment, but some investigations show treatment of MIS-C with intravenous immunoglobulin (IVIg), corticosteroids, and inflammation inhibitors. Globally, the long-term complications of MIS-C remain unclear (26, 27). We found a pattern of decreased T-cell activation when we observed the SARS-CoV-2-specific antigen stimulation after 6–9 months of treatment in children with MIS. This observation was applicable to both CD4+ and CD8+ T cells. Our group has previously reported these diminished inflammatory marker responses after MIS-C in cell culture supernatants (16). The mechanism behind the alteration of T-cell activation between the pre- and post-treatment of MIS-C has to be further explored. Also, various other factors were seen that support inflammation during the MIS-C condition (28).
The incidence of T-cell alterations is one of the common features of MIS-C (29). Our strengths include the following: (1) Control children with febrile illness not previously reported in other cohorts (dengue, typhoid fever, scrub typhus); (2) Samples obtained from all children before immunomodulation; and (3) Long-term follow up of children. Our findings reinforce that MIS-C is characterized by enhanced antigen-specific immune responses, which while contributing to host resistance may also enhance host pathology and lead to organ damage. Our study adds to the growing literature in the field that MIS-C is a syndrome of complex immunological etiology. Findings from this study also clearly reveal that enhanced SARS-CoV-2 antigen–specific T-cell responses are an important immunological feature of MIS-C at baseline. Our study suffers from the limitation of a small sample size, and the likely disparity in timing since infection onset with SARS-CoV-2 in the MIS-C and acute COVID-19 groups. Our study also suffers from the limitation of not including children with other autoimmune conditions such as systemic lupus erythematosus and juvenile idiopathic arthiritis. However, conducting the study posed a challenge as these patients were already on immunosuppressive medications, which could potentially interfere with the immune assays we conducted. Nevertheless, our study adds to the growing body of evidence of alterations in T-cell responses, especially cytokine production, which is a key characteristic defect in MIS-C. In conclusion, the findings from the present study advance our knowledge on the immunology of MIS-C and COVID-19 in children, and may benefit researchers.
Data availability statement
The original contributions presented in the study are included in the article/Supplementary Material, further inquiries can be directed to the corresponding author.
Ethics statement
The studies involving humans were approved by the National Institute for Research in Tuberculosis. The studies were conducted in accordance with the local legislation and institutional requirements. Written informed consent for participation in this study was provided by the participants’ legal guardians/next of kin. Written informed consent was obtained from the individual(s), and minor(s)' legal guardian/next of kin, for the publication of any potentially identifiable images or data included in this article.
Author contributions
Designing the study: SB and NP. Conducting experiments: KA, AN, and RR. Acquiring data: NP, KA, AV, SH, and UR. Analyzing data: NP and KA. Funding acquisition: SB. Project administration: PV, ES, TS, RS, AT, SN, GR, SH, and TN. Writing the article: NP and SB. All authors contributed to the article and approved the submitted version.
Funding
This work was supported by the Division of Intramural Research, National Institute of Allergy and Infectious Diseases (NIAID), the National Institute of Health (NIH), National Institute of Research in Tuberculosis (NIRT), and the International Center for Excellence in Research (ICER).
Acknowledgments
We would like to thank our NIRT Director for the constant support throughout the study; we also thank all the Pediatric Consultants and infection control nurses at the Institute of Child Health and Hospital for Children, Chennai, India; Mehta's Children's Hospital, Chennai, India; and Rainbow Children's Hospital, Chennai, India, for their support and contributions. We also sincerely thank the NIAID-ICER staff for technical support.
Conflict of interest
The authors declare that the research was conducted in the absence of any commercial or financial relationships that could be construed as a potential conflict of interest.
Publisher's note
All claims expressed in this article are solely those of the authors and do not necessarily represent those of their affiliated organizations, or those of the publisher, the editors and the reviewers. Any product that may be evaluated in this article, or claim that may be made by its manufacturer, is not guaranteed or endorsed by the publisher.
Supplementary material
The Supplementary Material for this article can be found online at: https://www.frontiersin.org/articles/10.3389/fped.2023.1235342/full#supplementary-material.
Supplementary Figure S1
Gating strategy for T cells expressing type1 and type17 cytokines upon SARS-CoV-2-specific antigens. The gating strategy shows the whole blood flow analysis from the lymphocyte population. From the CD3+ T-cell gating, the CD4+ T helper and CD8+ T cytotoxic cells were gated. The type-1 and type-17 cytokine-expressing T-cell subsets gated from each T-cell subset are also shown.
Supplementary Table S1
Moderate changes seen between the MIS-C children with less then 5 years (n=7) vs greater than 5 years. MIS-C children frequencies and net frequencies of CD4+ T cells and CD8+ T cell populations expressing Th1 and Th17 cytokines with or without SARS-CoV-2 antigenic stimulation were compared statistically between of less then 5 years (n=7) and greater than 5 years (n=8). The P-values were calculated using Mann-Whitney test to show the significance and were represented as p<0.005.
References
1. Jones TC, Mühlemann B, Veith T, Biele G, Zuchowski M, Hofmann J, et al. “An analysis of SARS-CoV-2 viral load by patient age.” MedRxiv (2020).
2. Nakra NA, Blumberg DA, Herrera-Guerra A, Lakshminrusimha S. Multi-system inflammatory syndrome in children (MIS-C) following SARS-CoV-2 infection: review of clinical presentation, hypothetical pathogenesis, and proposed management. Children. (2020) 7(7):69. doi: 10.3390/children7070069
3. Multisystem inflammatory syndrome in children (MIS-C) and adults (MIS-A). Available at: https://www.cdc.gov/mis/mis-c.html (Accessed January 3, 2023).
4. Greene AG, Saleh M, Roseman E, Sinert R. Toxic shock-like syndrome and COVID-19: multisystem inflammatory syndrome in children (MIS-C). Am J Emerg Med. (2020) 38(11):2492.e5–.6. doi: 10.1016/j.ajem.2020.05.117
5. Gorelik M. Learning about Kawasaki disease from COVID-19 and the multisystem inflammatory syndrome in children. Curr Opin Pediatr. (2021) 33(6):603–9. doi: 10.1097/MOP.0000000000001047
6. Multisystem inflammatory syndrome in children and adolescents temporally related to COVID-19. WHO/2019-nCoV/Sci_Brief/Multisystem_Syndrome_Children/2020. Available at: https://www.who.int/publications/i/item/multisystem-inflammatory-syndrome-in-children-and-adolescents-with-covid-19 (Accessed June 6, 2020).
7. Gruber CN, Patel RS, Trachtman R, Lepow L, Amanat F, Krammer F, et al. Mapping systemic inflammation and antibody responses in multisystem inflammatory syndrome in children (MIS-C). Cell. (2020) 183(4):982–95.e14. doi: 10.1016/j.cell.2020.09.034
8. Consiglio CR, Cotugno N, Sardh F, Pou C, Amodio D, Rodriguez L, et al. The immunology of multisystem inflammatory syndrome in children with COVID-19. Cell. (2020) 183(4):968–81.e7. doi: 10.1016/j.cell.2020.09.016
9. Burbelo PD, Castagnoli R, Shimizu C, Delmonte OM, Dobbs K, Discepolo V, et al. Autoantibodies against proteins previously associated with autoimmunity in adult and pediatric patients with COVID-19 and children with MIS-C. Front Immunol. (2022) 13:841126. doi: 10.3389/fimmu.2022.841126
10. Brodsky NN, Ramaswamy A, Lucas CL. The mystery of MIS-C post-SARS-CoV-2 infection. Trends Microbiol. (2020) 28(12):956–8. doi: 10.1016/j.tim.2020.10.004
11. Lee M, Kang B, Lee J, Lee J, Jung ST, Son CY, et al. De novo selected hACE2 mimics that integrate hotspot peptides with aptameric scaffolds for binding tolerance of SARS-CoV-2 variants. Sci Adv. (2022) 8(43):eabq6207. doi: 10.1126/sciadv.abq6207
12. Santos-Rebouças CB, Piergiorge RM, Dos Santos Ferreira C, Zeitel RS, Gerber AL, Rodrigues MCF, et al. Host genetic susceptibility underlying SARS-CoV-2-associated multisystem inflammatory syndrome in Brazilian children. Mol Med. (2022) 28(1):153. doi: 10.1186/s10020-022-00583-5
13. Boribong BP, LaSalle TJ, Bartsch YC, Ellett F, Loiselle ME, Davis JP, et al. Neutrophil profiles of pediatric COVID-19 and multisystem inflammatory syndrome in children. bioRxiv [Preprint]. (2021). 2021.12.18.473308. doi: 10.1101/2021.12.18.473308 Update in Cell Rep Med. (2022) 3:100848.
14. Rajamanickam A, Nathella PK, Venkataraman A, Varadarjan P, Kannan S, Pandiarajan AN, et al. Unique cellular immune signatures of multisystem inflammatory syndrome in children. PLoS Pathog. (2022) 18(11):e1010915. doi: 10.1371/journal.ppat.1010915
15. Lam KP, Chiñas M, Julé AM, Taylor M, Ohashi M, Benamar M, et al. SARS-CoV-2-specific T cell responses in patients with multisystem inflammatory syndrome in children. Clin Immunol. (2022) 243:109106. doi: 10.1016/j.clim.2022.109106
16. Kumar NP, Venkataraman A, Nancy A, Moideen K, Varadarjan P, Selladurai E, et al. Enhanced severe acute respiratory syndrome coronavirus 2 antigen-specific systemic immune responses in multisystem inflammatory syndrome in children and reversal after recovery. J Infect Dis. (2022) 226(7):1215–23. doi: 10.1093/infdis/jiac304
17. Hsieh LE, Song J, Grifoni A, Shimizu C, Tremoulet AH, Dummer KB, et al. T cells in multisystem inflammatory syndrome in children (MIS-C) have a predominant CD4+ T helper response to SARS-CoV-2 peptides and numerous virus-specific CD4− CD8− double-negative T cells. Int J Mol Sci. (2022) 23(13):7219. doi: 10.3390/ijms23137219
18. Morita A, Hosaka S, Imagawa K, Ishiodori T, Nozaki Y, Murakami T, et al. Time course of peripheral immunophenotypes of multisystem inflammatory syndrome in children. Clin Immunol. (2022) 236:108955. doi: 10.1016/j.clim.2022.108955
19. Zhang QY, Xu BW, Du JB. Similarities and differences between multiple inflammatory syndrome in children associated with COVID-19 and Kawasaki disease: clinical presentations, diagnosis, and treatment. World J Pediatr. (2021) 17(4):335–40. doi: 10.1007/s12519-021-00435-y
20. Henderson LA, Yeung RSM. MIS-C: early lessons from immune profiling. Nat Rev Rheumatol. (2021) 17:75–6. doi: 10.1038/s41584-020-00566-y
21. Beckmann ND, Comella PH, Cheng E, Lepow L, Beckmann AG, Tyler SR, et al. Downregulation of exhausted cytotoxic T cells in gene expression networks of multisystem inflammatory syndrome in children. Nat Commun. (2021) 12:4854. doi: 10.1038/s41467-021-24981-1
22. Moreews M, Le Gouge K, Khaldi-Plassart S, Pescarmona R, Mathieu AL, Malcus C, et al. Polyclonal expansion of TCR vbeta 21.3+ CD4+ and CD8+ T cells is a hallmark of multisystem inflammatory syndrome in children. Sci Immunol. (2021) 6(59):eabh1516. doi: 10.1126/sciimmunol.abh1516
23. Conway SR, Lazarski CA, Field NE, Jensen-Wachspress M, Lang H, Kankate V, et al. SARS-CoV-2-specific T cell responses are stronger in children with multisystem inflammatory syndrome compared to children with uncomplicated SARS-CoV-2 infection. Front Immunol. (2022) 12:793197. doi: 10.3389/fimmu.2021.793197
24. Vella LA, Giles JR, Baxter AE, Oldridge DA, Diorio C, Kuri-Cervantes L, et al. Deep immune profiling of MIS-C demonstrates marked but transient immune activation compared to adult and pediatric COVID-19. Sci Immunol. (2021) 6(57):eabf7570. doi: 10.1126/sciimmunol.abf7570
25. Rybkina K, Bell JN, Bradley MC, Wohlbold T, Scafuro M, Meng W, et al. SARS-CoV-2 infection and recovery in children: distinct T cell responses in MIS-C compared to COVID-19. J Exp Med. (2023) 220(8):e20221518. doi: 10.1084/jem.20221518
26. Kabeerdoss J, Pilania RK, Karkhele R, Kumar TS, Danda D, Singh S. Severe COVID-19, multisystem inflammatory syndrome in children, and Kawasaki disease: immunological mechanisms, clinical manifestations and management. Rheumatol Int. (2021) 41(1):19–32. doi: 10.1007/s00296-020-04749-4
27. Mahmoud S, El-Kalliny M, Kotby A, El-Ganzoury M, Fouda E, Ibrahim H. Treatment of MIS-C in children and adolescents. Curr Pediatr Rep. (2022) 10(1):1–10. doi: 10.1007/s40124-021-00259-4
28. Okarska-Napierała M, Mańdziuk J, Feleszko W, Stelmaszczyk-Emmel A, Panczyk M, Demkow U, et al. Recurrent assessment of lymphocyte subsets in 32 patients with multisystem inflammatory syndrome in children (MIS-C). Pediatr Allergy Immunol. (2021) 32(8):1857–65. doi: 10.1111/pai.13611
Keywords: MIS-C, SARS-CoV-2, COVID-19, CD4+ T cells, CD8+ T cells, cellular immune responses
Citation: Pavan Kumar N, Abbas KM, Renji RM, Venkataraman A, Nancy A, Varadarjan P, Selladurai E, Sangaralingam T, Selvam R, Thimmaiah A, Natarajan S, Ramasamy G, Hissar S, Ranganathan UD, Nutman TB and Babu S (2023) Multisystem inflammatory syndrome in children characterized by enhanced antigen-specific T-cell expression of cytokines and its reversal following recovery. Front. Pediatr. 11:1235342. doi: 10.3389/fped.2023.1235342
Received: 6 June 2023; Accepted: 13 November 2023;
Published: 5 December 2023.
Edited by:
Rita Consolini, University of Pisa, ItalyReviewed by:
Matthew Adams, Wayne State University, United StatesGiorgio Costagliola, Azienda Ospedaliero Universitaria Pisana, Italy
© 2023 Pavan Kumar, Abbas, Renji, Venkataraman, Nancy, Varadarjan, Selladurai, Sangaralingam, Selvam, Thimmaiah, Natarajan, Ramasamy, Hissar, Ranganathan, Nutman and Babu. This is an open-access article distributed under the terms of the Creative Commons Attribution License (CC BY). The use, distribution or reproduction in other forums is permitted, provided the original author(s) and the copyright owner(s) are credited and that the original publication in this journal is cited, in accordance with accepted academic practice. No use, distribution or reproduction is permitted which does not comply with these terms.
*Correspondence: Nathella Pavan Kumar nathella.pk@icmr.gov.in
†These authors have contributed equally to this work