- Department of Clinical Laboratory, Beijing Bo'ai Hospital, China Rehabilitation Research Center, Beijing, China
Cerebral palsy (CP) is a broad range of diseases with permanent and nonprogressive motor impairments, carrying a high cost for both the individual and the society. The characteristics of low bone mineral density and high risk of fractures suggest that bone metabolism disorders are present in CP. This study aims to investigate the association between indicators of bone metabolism and children with CP. A total of 139 children (75 children with CP and 64 healthy controls) were included in this cross-sectional study. Participants were divided into three age groups (0–2 years, 2.1–4 years, and 4.1–7 years). All children with CP were diagnosed according to clinical criteria and furtherly divided into clinical subtypes. The levels of total procollagen type I N-terminal propeptide (TPINP), N-MID osteocalcin (OC), beta-crosslaps (β-CTX), 25-hydroxyvitamin D (25-OHD) and parathyroid hormone (PTH) in the serum were measured with corresponding detection kits according to the manufacturer's instructions. Serum levels of TPINP and 25-OHD were lower with older age, whereas β-CTX and PTH were higher with older age. In the CP group, TPINP (age 0–2 years and 2.1–4 years) and OC (age 2.1–4 years) levels were higher, while β-CTX (age 2.1–4 years and 4.1–7 years) and PTH (age 2.1–4 years) values were lower than the control group. In addition, there were no statistically significant differences in the levels of these indicators among the CP subgroups with different clinical characteristics. Our study shows that bone turnover markers, indicators of bone metabolism, in children with CP differ significantly from healthy controls. The indicators we studied changed with age, and they did not correlate with disease severity.
Introduction
Cerebral palsy (CP) is the most prevalent physical disability in childhood, with a prevalence around 2 per 1,000 live births (1). CP is a heterogeneous group of disorders of movement and posture attributed to a defect or lesion in the developing fetal or infant brain (2). Although the etiology of CP is multifactorial and complex, the exact causative mechanisms contributing to pathogenesis remain unknown, making progress towards prevention and treatment strategies difficult (3).
The skeleton is an important component of vertebrates’ body, providing structural support for locomotion and protection for major organs (4). Meanwhile, bone tissue also functions as an endocrine organ (5). Bone cells in the human body are continuously involved in cellular metabolism, a dynamic process of osteoblastic bone formation and osteoclastic bone resorption (6). Studies on different populations (older adults, menopausal females, etc.) reports that bone metabolism changes are closely related to bone density and fractures (7–10), but this is less studied in children and especially in children with CP. Importantly, low bone mineral density is common in children with CP (8). Therefore, further investigation is required to determine the attributes of bone metabolism in children with CP.
TPINP, OC, β-CTX, 25-OHD and PTH are commonly used as important clinical indicators of bone metabolism. Of these, TPINP and OC are measured as indicators of bone formation, β-CTX can be used as an indicator of bone resorption. The other two metrics, 25-OHD and PTH, play central roles in regulating calcium-phosphate metabolism (11–14). These indicators can assist in disease diagnosis (15), treatment monitoring (16, 17), predicting changes in bone density (16), and predicting fracture risk (18) in populations with different physiological or pathological states (19). The skeletal expansion undergoes dramatic changes as humans develop from infancy to adulthood, especially in early childhood and puberty. Previous studies reported that serum levels of bone metabolism markers reflect skeletal development during puberty (20). As skeletal development is nonlinear during puberty, results of bone turnover markers must be interpreted in combination with the subject's maturation stage. To date, there is little research on differences in these indicators of bone metabolism by age in children with CP as compared to children without CP. As there are rapid changes in skeletal growth, knowledge of bone metabolism by age in children with CP may inform on biological aspects of poor bone health associated with CP.
The objective of this study was to test for differences in serum indicators of bone metabolism by age between children with and without CP.
Materials and methods
Subjects
A total of 139 children (75 children with CP and 64 typically developing children) were included in the present study. All the children with CP were enrolled from Beijing Bo’ ai Hospital, China Rehabilitation Research Center. Clinical data were obtained from all cases, including gestational age, gender, details of pregnancy and birth, head circumference, birth weight, Apgar scores, neonatal events, and height and weight at the time of the assessment. All patients were diagnosed according to the clinical criteria and classified into spastic (furtherly divided into hemiplegia, diplegia and quadriplegia), ataxic, dyskinetic subtypes on the basis of the guidelines proposed by the Surveillance of CP in Europe (SCPE) (21). Gross motor impairment was assessed by Gross Motor Function Classification System (GMFCS) (22), which ranges from level I to level V. Children with CP classified as GMFCS level I–III have minimal-moderate motor impairment and activity limitation. Children with CP classified as levels IV and V correspond with having no independent walking ability. Medical complications associated with CP were also recorded (e.g., cognitive impairment, epilepsy, feeding difficulties). Exclusion criteria included children with myopathy, hypotonia, neural tube defect, ataxia, chromosomal anomaly, genetic syndrome, or other chronic disease that could influence bone metabolism. Healthy children that did not have any history of neurological disorders and mental disorders were recruited from Maternal & Child Health Hospital in Fengtai and Xicheng Districts. The study was approved by the Ethics Committee of Beijing Bo’ai Hospital, China Rehabilitation Research Center.
Samples
Peripheral whole blood samples were drawn in procoagulant tubes from the median cubital vein after an eight hour fast and taken between 8:00 and 10:00 am to avoid diurnal variation. Then, the blood samples were centrifuged at 4,000 rpm for 10 min and aliquots of serum were immediately stored at −80°C until analysis. None of the samples used in the study showed hemolysis.
Analytical determinations
The levels of TPINP, OC, β-CTX, 25-OHD and PTH in the serum were measured with corresponding detection kits according to the manufacturer's instructions (Roche Diagnostics GmbH, Germany). Analysis was performed using the Roche Cobas e411 Automatic Electrochemiluminescence Immunoassay Instrument.
Statistical analysis
The statistical analyses were performed using GraphPad Prism (Version 7.00, GraphPad Software Inc., USA). Age was stratified into three groups: 0–2.0, 2.1–4.0, and 4.1–7 years old. The indicators of bone metabolism were presented as median [interquartile range (IQR)]. The differences in the distribution of clinical characteristics between the CP group and healthy controls were evaluated by Chi-Square test or Fisher's exact test for categorical variables (age, gender). The comparisons between the subgroups in the CP group were statistically evaluated by Student's t-test or Mann-Whitney U-test. The comparisons within groups were analyzed with a nonparametric Kruskal-Wallis test with a post hoc Dunn's test. A p-value <0.05 was considered statistically significant.
Exploratory analysis
The relationship between the primary bone resorption indicator, β-CTX, with the primary bone formation indicators, TPINP and OC, for both cohorts including all ages 0–7 years old was examined using simple linear regression. The regression equation, Pearson correlation coefficient, and p-value were reported for each cohort. Regression analyses were performed before and after excluding outliers, which was determined by values that were ≥3 standard deviations above the group mean. To assess for the possibility of de-coupling of bone resorption and formation between cohorts, the variance analysis of factorial design examined the main and interaction effects for group (CP vs. controls) and β-CTX for each bone formation indicator before and after adjusting for age (as continuous). The interaction effect was the primary focus of this analysis.
Results
Baseline characteristics
Descriptive characteristics of children with CP (n = 75) and healthy controls (n = 64) are presented in Table 1. Notably, there were no significant differences between groups for gender for the full cohorts (p = 0.386) or for continuous age within each age category (p > 0.120) (Table 1).
Comparison of different indicators of bone metabolism
The serum indicators of bone metabolism for children with CP and healthy controls by age group are presented in Figure 1. In general, and for both the CP and healthy control groups, TPINP and 25-OHD levels were lower with older age groups, PTH and β-CTX were higher with older age groups, and OC showed a bimodal pattern with age groups.
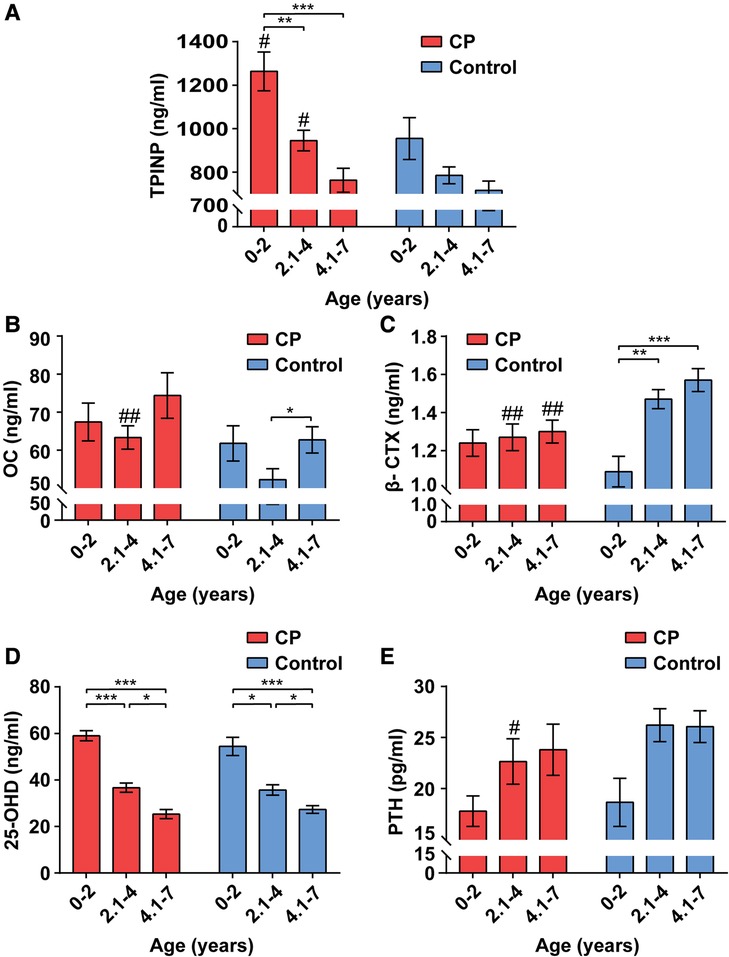
Figure 1. Alterations of bone metabolism in CP group and healthy control group by age ranges. The values of total procollagen type I N-terminal propeptide (TPINP) (A), N-MID osteocalcin (OC) (B), beta-crosslaps (β-CTX) (C), 25-hydroxyvitamin D (25-OHD) (D) and parathyroid hormone (PTH) (E) in different groups. Data represent the Median (1st, 3rd quartiles). # and * indicate inter-group and intra-group comparison results, respectively. *p < 0.05; **p < 0.01; ***p < 0.001; #p < 0.05; ##p < 0.01.
Among 0–2-year-olds, children with CP had higher serum levels of TPINP (p = 0.037) compared to healthy controls. Among 2.1–4-year-olds, children with CP had higher serum levels of TPINP (p = 0.015) and OC (p = 0.003) and lower levels of serum β-CTX (p = 0.004) and PTH (p = 0.032) compared to healthy controls. Among 4.1–7-year-olds, children with CP had lower serum levels β-CTX (p = 0.002) compared to healthy controls.
Association between different markers and clinical characteristics of CP
Due to a limited sample size, spastic and non-spastic (ataxic, dyskinetic, non-classified) types of CP were grouped. There were no statistical differences in the indicators of bone metabolism after stratifying the CP cohort by spastic (n = 57) or non-spastic (n = 18) type (Table 2). The descriptive characteristics of the spastic and non-spastic type CP groups are presented in Supplementary Table S1. Notably, age was similar (2.53 ± 1.59 vs. 2.27 ± 1.67, p = 0.387) but the spastic type CP group had a higher proportion of males (71.9% vs. 44.4%, p = 0.047).
Due to a limited sample size, GMFCS was stratified by I-III and IV/V. There were no statistical differences in the indicators of bone metabolism after stratifying the CP cohort by GMFCS I-III (n = 50) or GMFCS IV/V (n = 25) type (Table 3). The descriptive characteristics of the GMFCS I-III and GMFCS IV/V groups are presented in Supplementary Table S2. Notably, the GMFCS I-III group was older on average (2.81 ± 1.65 vs.1.78 ± 1.26, p = 0.040) but there were no differences in gender (male, 68.0% vs. 60.0%).
The spastic CP was further subdivided into hemiplegia, diplegia and quadriplegia. The basic clinical information is shown in Supplementary Table S3. There were no significant differences in age (2.03 ± 1.37 vs. 2.72 ± 1.57 vs. 2.73 ± 1.98, p = 0.290) and gender (p = 0.490) between the groups. We did not find any significant differences in variances among groups (Table 4).
Exploratory analysis
The association between β-CTX and TPINP and between β-CTX and OC is presented in Figure 2. There was evidence of 2 outliers for the TPINP values in the CP group. There was a positive, weak relationship between β-CTX and TPINP in the CP and controls groups, before and after excluding the outliers. The relationship was also positive and stronger between β-CTX and OC for both groups, especially in CP. There was no strong evidence of a group by β-CTX interaction for TPINP after excluding the 2 outliers before and after adjusting for age (p for interaction, 0.683 and 0.299, respectively). There was no evidence of a group by β-CTX interaction for OC before and after adjusting for age (p for interaction, 0.766 and 0.807).
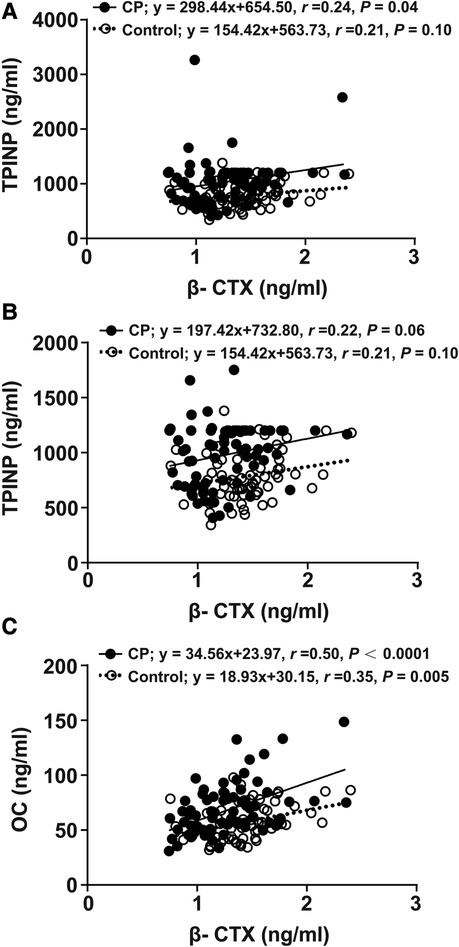
Figure 2. Linear regression analyses of indicators between the CP group and the control group. β-CTX regressed with TPINP (A), β-CTX regressed with TPINP (2 significant outliers were removed) (B), β-CTX regressed with OC (C). β-CTX, beta-crosslaps; TPINP, total procollagen type I N-terminal propeptide; OC, N-MID osteocalcin; CP, Cerebral palsy.
Discussion
In this study, we found significant differences in certain indicators of bone metabolism at specific age groups in children with CP when compared to a cohort of healthy controls. There was no evidence that the indicators of bone metabolism were mainly driven by clinical characteristics of CP, including the type of CP (e.g., spastic vs. non-spastic) or the severity of motor important via GMFCS.
In this study, we selected two indicators reflecting bone formation, including TPINP and OC. Type I procollagen, an osteoblast product, can form Type I collagen via enzymatic cleavage the pro-peptide (23). During the process, N-terminal propeptide, also referred to as TPINP, is released into circulation (24). This process corresponds to the formation of bone matrix (25). Thus, the level of TPINP is considered a reliable marker of bone formation. It is stable and no circadian rhythm (26). In this study, we found that TPINP concentrations gradually decreases with age (in the age band 0–7 years) in the healthy control groups, which is consistent with previous studies (27, 28). Meanwhile, our results showed that children with CP had higher serum levels TPINP than healthy controls at ages 0–4 years old, indicating higher levels of bone formation. As prior research has reported that children with CP have low bone density (29) and notable defects in bone size, shape or structure (30–32), we speculate that the higher bone formation in 0–4 year olds with CP is ineffective and even harmful, such as heterotopic ossification (33, 34). In addition, children with CP have increased fracture risk associated with low bone mineral density and high bone fragility (35). Fracture healing is a process of bone remodeling that is often accompanied by a significant enhancement of bone formation (36, 37).
OC is one of the most abundant non-collagenous proteins in bone tissue, which is secreted by osteoblasts (38). OC is partly involved in bone matrix formation and partly secreted into the peripheral blood (39, 40). Therefore, serum OC levels are used as a valuable indicator of bone formation (41). Although the circadian rhythm plays a role in serum concentrations of OC (42), blood was taken from participants around the same time during the day, thus mitigating bias in group differences due to the timing of blood draw. In this study, the bimodal pattern of serum levels of OC by age group is similar to that of a previous study (28). Further, among 2.1–4-year-olds, children with CP had higher levels of OC than healthy controls, which is consistent with the higher levels of the bone formation indicator, TPINP.
β-CTX is known as a marker of bone resorption, which reflects the degree of bone matrix degradation (43). Mature type I collagen of bone matrix degrades into β-CTX and releases into the bloodstream in the process of bone metabolism (44, 45). Our results showed a gain in bone resorption with age in the control groups. This may promote new bone formation, as bone resorption is a source of bone formation-stimulating factors (46). We found lower levels of β-CTX in the group with CP among 2.1–7-year-olds, which is not conducive to bone formation. The reasons may be due to exercise restriction and decrease in muscle stimulation (47).
The above data, taken together, suggest that bone formation in children with CP was higher than that in healthy children in different age groups. On the contrary, children with CP had lower bone resorption than healthy children with older age, particularly after age 2. Skeletal growth is the results of a dynamic balance between bone formation and bone resorption (48, 49), and this balance has been demonstrated to be disrupted in many pediatric diseases (50). In our study, imbalance in bone metabolism was also found in disease group, potentially causing growth restriction to some extent. These features deserve the physicians’ attention in the future. Some cytokines have multiple effects on RANKL-RANK/osteoprotegerin and WNT-ß-catenin signaling pathways which control osteoclastogenesis and osteoblastogenesis, respectively. Alterations in their levels may influence bone remodeling both in inherited and acquired pediatric diseases (50–52). In another study, genetic variants ultimately lead to defects in hormonal signaling, paracrine signaling and extracellular matrix (48). Therefore, further studies of the specific mechanisms of altered bone metabolism in children with CP are needed in the above and other areas.
25-OHD is necessary for the maintenance of calcium homeostasis (53). As 25-OHD is the main storage form of vitamin D, its levels are used clinically to determine vitamin D status (54). Dietary intake and skin synthesis are two major sources of vitamin D (55). Our findings showed that the 25-OHD values of patients with CP were similar to those in the control groups, which is consistent with previous studies (56, 57). In addition, we observed the levels of 25-OHD decreased significantly with age. One possible reason is that exogenous supplementation of vitamin D is reduced in children older than 2 years (58).
PTH is the most important endocrine regulator of calcium and phosphorus concentration in extracellular fluid (59). It is secreted by the parathyroid gland in response to low Ca2+ concentrations of extracellular (60). The primary role of PTH is to increase tubular reabsorption of Ca2+ in the kidney and promote renal excretion of phosphate (61). Previous studies suggested that CP is associated with phosphorus-calcium metabolic disorders (62). Similarly, our study demonstrated that the level of PTH was significantly lower in the CP group at age 2.1–4. Future studies are needed to determine if appropriate treatment for phosphorus-calcium metabolic disorders can improve bone metabolism associated with CP.
In our study, concentrations of five indicators revealed no significant difference between GMFCS (I-III) and GMFCS (IV/V), spastic CP and non-spastic CP, or hemiplegia, diplegia and quadriplegia. Further, the exploratory analysis did not find compelling evidence that the relationship between the primary bone resorption marker, β-CTX, with the primary bone formation markers, TPINP and OC, differed between groups. Thus, given the higher y-intercept, bone formation may slightly outpace bone resorption in this cohort of children with CP as compared to healthy controls. However, these analyses were exploratory and should be interested as hypothesis-generating as opposed to confirmation of associations.
There were limitations in this present study. First, there was a limited sample size, especially for healthy group from years 0 to 2. Nevertheless, our findings in healthy groups are consistent with existing literature (28), suggesting the methods used in this study can be appropriate to make interpretations for the findings in children with CP. Second, the indicators of bone metabolism used in this study are typical and commonly used in clinical practice, but they do not fully capture the complexity of bone metabolism during development and for skeletally complex populations, like CP. Third, the mechanisms of abnormal bone metabolism in CP are not well understood, which may serve as a new direction for our future research.
Conclusion
In summary, this study demonstrates that certain indicators of bone metabolism in children with CP differ from healthy controls at certain pre-pubertal ages. These findings may be helpful for understanding bone health and development in children with CP from the context of bone metabolism.
Data availability statement
The raw data supporting the conclusions of this article will be made available by the authors, without undue reservation.
Ethics statement
The studies involving human participants were reviewed and approved by The Ethics Committee of the Beijing Bo’ai Hospital of China Rehabilitation Research Center. Written informed consent from the participants’ legal guardian/next of kin was not required to participate in this study in accordance with the national legislation and the institutional requirements.
Author contributions
ZL designed the study and revised the manuscript. WX accomplished statistical analysis and drafted the manuscript. LL, ND, and LC carried out experiments, collected the data. All authors contributed to the article and approved the submitted version.
Acknowledgments
The authors would like to appreciate all subjects participated in the study.
Conflict of interest
The authors declare that the research was conducted in the absence of any commercial or financial relationships that could be construed as a potential conflict of interest.
Publisher's note
All claims expressed in this article are solely those of the authors and do not necessarily represent those of their affiliated organizations, or those of the publisher, the editors and the reviewers. Any product that may be evaluated in this article, or claim that may be made by its manufacturer, is not guaranteed or endorsed by the publisher.
Supplementary material
The Supplementary Material for this article can be found online at: https://www.frontiersin.org/articles/10.3389/fped.2023.1214608/full#supplementary-material
References
1. Moreno-De-Luca A, Millan F, Pesacreta DR, Elloumi HZ, Oetjens MT, Teigen C, et al. Molecular diagnostic yield of exome sequencing in patients with cerebral palsy. JAMA. (2021) 325:467–75. doi: 10.1001/jama.2020.26148
2. Jin SC, Lewis SA, Bakhtiari S, Zeng X, Sierant MC, Shetty S, et al. Mutations disrupting neuritogenesis genes confer risk for cerebral palsy. Nat Genet. (2020) 52:1046–56. doi: 10.1038/s41588-020-0695-1
3. Korzeniewski SJ, Slaughter J, Lenski M, Haak P, Paneth N. The complex aetiology of cerebral palsy. Nat Rev Neurol. (2018) 14:528–43. doi: 10.1038/s41582-018-0043-6
4. de Lageneste OD, Julien A, Abou-Khalil R, Frangi G, Carvalho C, Cagnard N, et al. Periosteum contains skeletal stem cells with high bone regenerative potential controlled by Periostin. Nat Commun. (2018) 9:773. doi: 10.1038/s41467-018-03124-z
5. Berger JM, Singh P, Khrimian L, Morgan DA, Chowdhury S, Arteaga-Solis E, et al. Mediation of the acute stress response by the Skeleton. Cell Metab. (2019) 30:890–902.e8. doi: 10.1016/j.cmet.2019.08.012
6. McKay RR, Bosse D, Gray KP, Michaelson MD, Krajewski K, Jacene HA, et al. Radium-223 dichloride in combination with vascular endothelial growth factor-targeting therapy in advanced renal cell carcinoma with bone metastases. Clin Cancer Res. (2018) 24:4081–8. doi: 10.1158/1078-0432.CCR-17-3577
7. Moon YJ, Zhang Z, Bang IH, Kwon OK, Yoon SJ, Kim JR, et al. Sirtuin 6 in preosteoclasts suppresses age- and estrogen deficiency-related bone loss by stabilizing estrogen receptor alpha. Cell Death Differ. (2019) 26:2358–70. doi: 10.1038/s41418-019-0306-9
8. Salimi S, Shardell M, Miller R, Gruber-Baldini AL, Orwig D, Fedarko N, et al. Soluble tumor necrosis factor alpha receptor 1, bone resorption, and bone mineral density in the year following hip fractures: the Baltimore hip studies. J Bone Miner Res. (2018) 33:1649–56. doi: 10.1002/jbmr.3457
9. Ensrud KE, Kats AM, Boyd CM, Diem SJ, Schousboe JT, Taylor BC, et al. Association of disease definition, comorbidity burden, and prognosis with hip fracture probability among late-life women. JAMA Intern Med. (2019) 179:1095–103. doi: 10.1001/jamainternmed.2019.0682
10. Barera G, Beccio S, Proverbio MC, Mora S. Longitudinal changes in bone metabolism and bone mineral content in children with celiac disease during consumption of a gluten-free diet. Am J Clin Nutr. (2004) 79:148–54. doi: 10.1093/ajcn/79.1.148
11. Kadriu B, Gold PW, Luckenbaugh DA, Lener MS, Ballard ED, Niciu MJ, et al. Acute ketamine administration corrects abnormal inflammatory bone markers in major depressive disorder. Mol Psychiatry. (2018) 23:1626–31. doi: 10.1038/mp.2017.109
12. Starup-Linde J, Lykkeboe S, Handberg A, Vestergaard P, Hoyem P, Fleischer J, et al. Glucose variability and low bone turnover in people with type 2 diabetes. Bone. (2021) 153:116159. doi: 10.1016/j.bone.2021.116159
13. Veletic I, Manshouri T, Multani AS, Yin CC, Chen L, Verstovsek S, et al. Myelofibrosis osteoclasts are clonal and functionally impaired. Blood. (2019) 133:2320–4. doi: 10.1182/blood-2018-10-878926
14. Fan Y, Hanai JI, Le PT, Bi R, Maridas D, DeMambro V, et al. Parathyroid hormone directs bone marrow mesenchymal cell fate. Cell Metab. (2017) 25:661–72. doi: 10.1016/j.cmet.2017.01.001
15. Wang C, Zhou YL, Cai H, Cheng XQ, Zhang W, Kang WY, et al. Markedly elevated serum total N-terminal propeptide of type I collagen is a novel marker for the diagnosis and follow up of patients with POEMS syndrome. Haematologica. (2014) 99:e78–e80. doi: 10.3324/haematol.2013.102962
16. Matsumoto T, Sone T, Soen S, Tanaka S, Yamashita A, Inoue T. Abaloparatide increases lumbar spine and hip BMD in Japanese patients with osteoporosis: the phase 3 ACTIVE-J study. J Clin Endocrinol Metab. (2022) 107:e4222–31. doi: 10.1210/clinem/dgac486
17. Leach K, Gregory KJ, Kufareva I, Khajehali E, Cook AE, Abagyan R, et al. Towards a structural understanding of allosteric drugs at the human calcium-sensing receptor. Cell Res. (2016) 26:574–92. doi: 10.1038/cr.2016.36
18. Swanson CM, Srikanth P, Lee CG, Cummings SR, Jans I, Cauley JA, et al. Associations of 25-hydroxyvitamin D and 1,25-dihydroxyvitamin D with bone mineral density, bone mineral density change, and incident nonvertebral fracture. J Bone Miner Res. (2015) 30:1403–13. doi: 10.1002/jbmr.2487
19. Eastell R, Hannon RA. Biomarkers of bone health and osteoporosis risk. Proc Nutr Soc. (2008) 67:157–62. doi: 10.1017/S002966510800699X
20. Banica T, Vandewalle S, Zmierczak HG, Goemaere S, De Buyser S, Fiers T, et al. The relationship between circulating hormone levels, bone turnover markers and skeletal development in healthy boys differs according to maturation stage. Bone. (2022) 158:116368. doi: 10.1016/j.bone.2022.116368
21. Surveillance of Cerebral Palsy in Europe. Surveillance of cerebral palsy in Europe: a collaboration of cerebral palsy surveys and registers. Surveillance of cerebral palsy in Europe (SCPE). Dev Med Child Neurol. (2000) 42:816–24. doi: 10.1017/s0012162200001511
22. Rosenbaum PL, Palisano RJ, Bartlett DJ, Galuppi BE, Russell DJ. Development of the gross motor function classification system for cerebral palsy. Dev Med Child Neurol. (2008) 50:249–53. doi: 10.1111/j.1469-8749.2008.02045.x
23. Koromani F, Oei L, Shevroja E, Trajanoska K, Schoufour J, Muka T, et al. Vertebral fractures in individuals with type 2 diabetes: more than skeletal complications alone. Diabetes Care. (2020) 43:137–44. doi: 10.2337/dc19-0925
24. Gensure RC, Makitie O, Barclay C, Chan C, Depalma SR, Bastepe M, et al. A novel COL1A1 mutation in infantile cortical hyperostosis (caffey disease) expands the spectrum of collagen-related disorders. J Clin Invest. (2005) 115:1250–7. doi: 10.1172/JCI22760
25. Shi Q, Rondon-Cavanzo EP, Dalla Picola IP, Tiera MJ, Zhang X, Dai K, et al. In vivo therapeutic efficacy of TNFalpha silencing by folate-PEG-chitosan-DEAE/siRNA nanoparticles in arthritic mice. Int J Nanomedicine. (2018) 13:387–402. doi: 10.2147/IJN.S146942
26. Wolthers OD, Heuck C, Heickendorff L. Diurnal variations in serum and urine markers of type I and type III collagen turnover in children. Clin Chem. (2001) 47:1721–2. doi: 10.1093/clinchem/47.9.1721
27. Manjon Llorente G, Fernandez-Espuelas C, Gonzalez Lopez JM, Ruiz-Echarri MP, Baldellou Vazquez A. [Normal values of bone turnover markers in childhood]. An Pediatr (Barc). (2004)60:330–6. doi: 10.1016/s1695-4033(04)78279-x
28. Bayer M. Reference values of osteocalcin and procollagen type I N-propeptide plasma levels in a healthy central European population aged 0-18 years. Osteoporos Int. (2014) 25:729–36. doi: 10.1007/s00198-013-2485-4
29. Modlesky CM, Zhang C. Complicated muscle-bone interactions in children with cerebral palsy. Curr Osteoporos Rep. (2020) 18:47–56. doi: 10.1007/s11914-020-00561-y
30. Whitney DG, Singh H, Miller F, Barbe MF, Slade JM, Pohlig RT, et al. Cortical bone deficit and fat infiltration of bone marrow and skeletal muscle in ambulatory children with mild spastic cerebral palsy. Bone. (2017) 94:90–7. doi: 10.1016/j.bone.2016.10.005
31. Modlesky CM, Subramanian P, Miller F. Underdeveloped trabecular bone microarchitecture is detected in children with cerebral palsy using high-resolution magnetic resonance imaging. Osteoporos Int. (2008) 19:169–76. doi: 10.1007/s00198-007-0433-x
32. Modlesky CM, Whitney DG, Singh H, Barbe MF, Kirby JT, Miller F. Underdevelopment of trabecular bone microarchitecture in the distal femur of nonambulatory children with cerebral palsy becomes more pronounced with distance from the growth plate. Osteoporos Int. (2015) 26:505–12. doi: 10.1007/s00198-014-2873-4
33. Inan M, Chan G, Dabney K, Miller F. Heterotopic ossification following hip osteotomies in cerebral palsy: incidence and risk factors. J Pediatr Orthop. (2006) 26:551–6. doi: 10.1097/01.bpo.0000217725.16417.af
34. Botte MJ, Keenan MA, Abrams RA, von Schroeder HP, Gellman H, Mooney V. Heterotopic ossification in neuromuscular disorders. Orthopedics. (1997) 20:335–41; quiz 342–3. doi: 10.3928/0147-7447-19970401-11
35. Presedo A, Dabney KW, Miller F. Fractures in patients with cerebral palsy. J Pediatr Orthop. (2007) 27:147–53. doi: 10.1097/BPO.0b013e3180317403
36. Wang Y, Malcolm DW, Benoit DSW. Controlled and sustained delivery of siRNA/NPs from hydrogels expedites bone fracture healing. Biomaterials. (2017) 139:127–38. doi: 10.1016/j.biomaterials.2017.06.001
37. Wang H, Tian L, Liu J, Goldstein A, Bado I, Zhang W, et al. The osteogenic niche is a calcium reservoir of bone micrometastases and confers unexpected therapeutic vulnerability. Cancer Cell. (2018) 34:823–39 e7. doi: 10.1016/j.ccell.2018.10.002
38. Lutsey PL, Norby FL, Ensrud KE, MacLehose RF, Diem SJ, Chen LY, et al. Association of anticoagulant therapy with risk of fracture among patients with atrial fibrillation. JAMA Intern Med. (2020) 180:245–53. doi: 10.1001/jamainternmed.2019.5679
39. Elefteriou F, Benson MD, Sowa H, Starbuck M, Liu X, Ron D, et al. ATF4 Mediation of NF1 functions in osteoblast reveals a nutritional basis for congenital skeletal dysplasiae. Cell Metab. (2006) 4:441–51. doi: 10.1016/j.cmet.2006.10.010
40. Wojda SJ, McGee-Lawrence ME, Gridley RA, Auger J, Black HL, Donahue SW. Yellow-bellied marmots (Marmota flaviventris) preserve bone strength and microstructure during hibernation. Bone. (2012) 50:182–8. doi: 10.1016/j.bone.2011.10.013
41. Sukumar D, Shapses SA, Schneider SH. Vitamin D supplementation during short-term caloric restriction in healthy overweight/obese older women: effect on glycemic indices and serum osteocalcin levels. Mol Cell Endocrinol. (2015) 410:73–7. doi: 10.1016/j.mce.2015.01.002
42. Heuck C, Skjaerbaek C, Wolthers OD. Diurnal rhythm of serum osteocalcin in normal children. Acta Paediatr. (1998) 87:930–2. doi: 10.1080/080352598750031563
43. Escudier B, Powles T, Motzer RJ, Olencki T, Aren Frontera O, Oudard S, et al. Cabozantinib, a new standard of care for patients with advanced renal cell carcinoma and bone metastases? Subgroup analysis of the METEOR trial. J Clin Oncol. (2018) 36:765–72. doi: 10.1200/JCO.2017.74.7352
44. Wattanathorn J, Somboonporn W, Sungkamanee S, Thukummee W, Muchimapura S. A double-blind placebo-controlled randomized trial evaluating the effect of polyphenol-rich herbal congee on bone turnover markers of the perimenopausal and menopausal women. Oxid Med Cell Longevity. (2018) 2018:2091872. doi: 10.1155/2018/2091872
45. Zhang H, Wu M, Zhu X, Li C, Li X, Sun J, et al. A phase I, randomized, single-dose study to evaluate the biosimilarity of QL1206 to denosumab among Chinese healthy subjects. Front Pharmacol. (2020) 11:01329. doi: 10.3389/fphar.2020.01329
46. Weivoda MM, Chew CK, Monroe DG, Farr JN, Atkinson EJ, Geske JR, et al. Identification of osteoclast-osteoblast coupling factors in humans reveals links between bone and energy metabolism. Nat Commun. (2020) 11:87. doi: 10.1038/s41467-019-14003-6
47. Willerslev-Olsen M, Petersen TH, Farmer SF, Nielsen JB. Gait training facilitates central drive to ankle dorsiflexors in children with cerebral palsy. Brain. (2015) 138:589–603. doi: 10.1093/brain/awu399
48. Faienza MF, Chiarito M, Brunetti G, D’Amato G. Growth plate gene involment and isolated short stature. Endocrine. (2021) 71:28–34. doi: 10.1007/s12020-020-02362-w
49. Lu JX, Pan H, Hu XQ, Huang ZW, Zhang Q. Effects of milk powder intervention on bone mineral density and indicators related to bone metabolism in Chinese adolescents. Osteoporos Int. (2019) 30:2231–9. doi: 10.1007/s00198-019-05105-4
50. Brunetti G, D’Amato G, Chiarito M, Tullo A, Colaianni G, Colucci S, et al. An update on the role of RANKL-RANK/osteoprotegerin and WNT-ss-catenin signaling pathways in pediatric diseases. World J Pediatr. (2019) 15:4–11. doi: 10.1007/s12519-018-0198-7
51. Faienza MF, Ventura A, Delvecchio M, Fusillo A, Piacente L, Aceto G, et al. High sclerostin and dickkopf-1 (DKK-1) serum levels in children and adolescents with type 1 diabetes mellitus. J Clin Endocrinol Metab. (2017) 102:1174–81. doi: 10.1210/jc.2016-2371
52. Brunetti G, Papadia F, Tummolo A, Fischetto R, Nicastro F, Piacente L, et al. Impaired bone remodeling in children with osteogenesis imperfecta treated and untreated with bisphosphonates: the role of DKK1, RANKL, and TNF-alpha. Osteoporos Int. (2016) 27:2355–65. doi: 10.1007/s00198-016-3501-2
53. Boyle MP, Noschese ML, Watts SL, Davis ME, Stenner SE, Lechtzin N. Failure of high-dose ergocalciferol to correct vitamin D deficiency in adults with cystic fibrosis. Am J Respir Crit Care Med. (2005) 172:212–7. doi: 10.1164/rccm.200403-387OC
54. Kestenbaum B, Katz R, de Boer I, Hoofnagle A, Sarnak MJ, Shlipak MG, et al. Vitamin D, parathyroid hormone, and cardiovascular events among older adults. J Am Coll Cardiol. (2011) 58:1433–41. doi: 10.1016/j.jacc.2011.03.069
55. Jensen MB, Lawaetz JG, Andersson AM, Petersen JH, Nordkap L, Bang AK, et al. Vitamin D deficiency and low ionized calcium are linked with semen quality and sex steroid levels in infertile men. Hum Reprod. (2016) 31:1875–85. doi: 10.1093/humrep/dew152
56. Langley CK, Onambele-Pearson GL, Sims DT, Hussain A, Buffey AJ, Bardwell HL, et al. Musculoskeletal health in active ambulatory men with cerebral palsy and the impact of vitamin D. Nutrients. (2021) 13:2481. doi: 10.3390/nu13072481
57. Tasdemir HA, Buyukavci M, Akcay F, Polat P, Yildiran A, Karakelleoglu C. Bone mineral density in children with cerebral palsy. Pediatr Int. (2001) 43:157–60. doi: 10.1046/j.1442-200x.2001.01352.x
58. Rosendahl J, Valkama S, Holmlund-Suila E, Enlund-Cerullo M, Hauta-Alus H, Helve O, et al. Effect of higher vs standard dosage of vitamin D3 supplementation on bone strength and infection in healthy infants: a randomized clinical trial. JAMA Pediatr. (2018) 172:646–54. doi: 10.1001/jamapediatrics.2018.0602
59. Meijerink M, van den Broek TJ, Dulos R, Garthoff J, Knippels L, Knipping K, et al. Network-based selection of candidate markers and assays to assess the impact of oral immune interventions on gut functions. Front Immunol. (2019) 10:2672. doi: 10.3389/fimmu.2019.02672
60. Centeno PP, Herberger A, Mun HC, Tu C, Nemeth EF, Chang W, et al. Phosphate acts directly on the calcium-sensing receptor to stimulate parathyroid hormone secretion. Nat Commun. (2019) 10:4693. doi: 10.1038/s41467-019-12399-9
61. Tamura T, Noda H, Joyashiki E, Hoshino M, Watanabe T, Kinosaki M, et al. Identification of an orally active small-molecule PTHR1 agonist for the treatment of hypoparathyroidism. Nat Commun. (2016) 7:13384. doi: 10.1038/ncomms13384
Keywords: cerebral palsy, bone metabolism, bone formation, bone resorption, phosphorus-calcium metabolism
Citation: Xing W, Liang L, Dong N, Chen L and Liu Z (2023) Abnormal changes of bone metabolism markers with age in children with cerebral palsy. Front. Pediatr. 11:1214608. doi: 10.3389/fped.2023.1214608
Received: 30 April 2023; Accepted: 17 July 2023;
Published: 1 August 2023.
Edited by:
Daniel Graham Whitney, University of Michigan, United StatesReviewed by:
Barbier Vincent, Centre Hospitalier Universitaire (CHU) d’Amiens, FranceMaria Felicia Faienza, University of Bari Aldo Moro, Italy
© 2023 Xing, Liang, Dong, Chen and Liu. This is an open-access article distributed under the terms of the Creative Commons Attribution License (CC BY). The use, distribution or reproduction in other forums is permitted, provided the original author(s) and the copyright owner(s) are credited and that the original publication in this journal is cited, in accordance with accepted academic practice. No use, distribution or reproduction is permitted which does not comply with these terms.
*Correspondence: Zhizhong Liu lzzlab@126.com