- 1The University of Queensland Centre for Clinical Research (UQCCR), Faculty of Medicine, The University of Queensland, Brisbane, QLD, Australia
- 2Central Microbiology, Pathology Queensland, Brisbane, QLD, Australia
- 3Blood and Bone Marrow Transplant Program, Queensland Children’s Hospital, Brisbane, QLD, Australia
- 4Infection Management and Prevention Service, Queensland Children’s Hospital, Brisbane, QLD, Australia
Cytomegalovirus (CMV) is a ubiquitous virus which causes a mild illness in healthy individuals. In immunocompromised individuals, such as children receiving haematopoietic stem cell transplantation, CMV can reactivate, causing serious disease and increasing the risk of death. CMV can be effectively treated with antiviral drugs, but antiviral resistance is an increasingly common complication. Available therapies are associated with adverse effects such as bone marrow suppression and renal impairment, making the choice of appropriate treatment challenging. New agents are emerging and require evaluation in children to establish their role. This review will discuss established and emerging diagnostic tools and treatment options for CMV, including antiviral resistant CMV, in children undergoing haematopoietic stem cell transplant.
1. Introduction
Cytomegalovirus (CMV) is a double stranded, linear DNA virus that is approximately 236 kilobase pairs (kbp) in length and belongs to the Herpesviridae family. The Herpesviridae family is divided into three sub-families, based on their biological properties: alpha herpesviruses (including the causative agents of “cold sores” and “genital herpes”, Herpes Simplex virus), beta herpesviruses (including CMV) and gamma herpesviruses (including Epstein Barr virus).
CMV is a ubiquitous herpesvirus that results in mild, self-limiting infections in healthy individuals. The seroprevalence in Australia is estimated to be over 30% in infants, increasing to over 50% by adulthood, and is higher in lower socio-economic areas (1, 2). As with other herpesvirus infections, CMV remains latent after primary infection, for the life of the host. This latent CMV can reactivate during periods of stress or when the host immune system becomes compromised, such as during solid organ transplantation (SOT) or haematopoietic stem cell transplantation (HSCT). In HSCT, CMV disease including pneumonitis, hepatitis, colitis and retinitis occurs in approximately 50% of patients with CMV reactivation, without antiviral intervention. Reactivation is associated with an approximately 3-fold increase in non-relapse mortality in HSCT recipients (3–5). In this setting, CMV reactivation may require prolonged antiviral treatment. This may select sub-populations of virus with reduced antiviral susceptibility, leading to CMV antiviral resistance and treatment failure (6). Current testing for antiviral resistance in CMV is difficult to access, slow and expensive, limiting its diagnostic value.
2. Cytomegalovirus in haematopoietic stem cell transplantation
In HSCT, donor progenitors of the immune system are transplanted into patients as definitive treatment for a range of haematological, immune, metabolic, and other disorders. The indications for HSCT in children have grown dramatically over the last four decades (7). Immunosuppressive drugs, graft failure, and Graft vs. Host Disease (GvHD) all result in significant immune suppression and play a role in the increased risk of morbidity and mortality in children receiving HSCT (8–10). Paediatric HSCT recipients require extended CMV monitoring after GvHD due to increased risk of developing CMV reactivation long after transplantation (11, 12). Infectious complications are common and the risk of infection increases with the intensity and duration of immunosuppressive therapy (9, 13).
Children undergoing HSCT may experience either primary infection with CMV or reactivation of a latent infection. Recent studies estimate that CMV reactivation occurs in approximately 20% of HSCT recipients (14, 15). It commonly presents asymptomatically and occurs an average of 8-weeks post-transplantation (8, 16, 17). The risk of CMV reactivation in patients is associated with the serostatus of the patient (recipient) and the transplant donor. Unlike in solid organ transplantation, seropositive HSCT recipients are at highest risk of reactivation owing to the prolonged absence of T-cell mediated immunity to control CMV replication (10, 13, 18).
3. The diagnostic approach to CMV in HSCT
Prevention of CMV disease may be achieved by a prophylactic or pre-emptive approach. Antiviral prophylaxis in HSCT is complicated by drug-drug interactions and toxicity, so it is common practice to pursue a pre-emptive approach in which regular surveillance of blood for CMV reactivation initiates pre-emptive therapy. Pre-emptive therapy is the most-used approach in Australasian paediatric HSCT recipients (19, 20). The 2017 European Conference on Infections in Leukaemia (ECIL7) recommend weekly sampling until at least 100 days post-transplant; however, sampling frequency and duration may be increased in patients deemed to be at high risk of infection (21, 22). In some cases, CMV reactivation or primary infections may occur more than 10 years post transplantation. These late reactivations typically have worse outcomes, likely due to delayed diagnosis (23–25).
3.1. Polymerase chain reaction
PCR is the most common method used in diagnostic laboratories for CMV detection and can be qualitative or quantitative (qPCR). PCR assays target and amplify conserved sections of the CMV genome with the inclusion of fluorescent dyes or probes used to assess the presence of the target within a patient's sample. qPCR has superseded testing for CMV antigenemia, and may be performed on various specimen types, including blood, urine, bronchoalveolar lavage and organ tissues (26). Surveillance as part of a pre-emptive treatment approach is performed on blood.
Interpretation of qPCR results are complicated by a lack of standardised diagnostic methods, variable specimen types, and differences in collection schedules and reference standards. In 2010, the World Health Organisation (WHO) established an international standard to be used for qPCR to ensure the reproducibility of results and to reduce variation in reporting between laboratories (27, 28). Nonetheless, there is no consensus for the viral copy number at which treatment should be initiated (21).
The monitoring of CMV viral loads can help to infer the emergence of antiviral resistance. Consensus recommendations define refractory infection when the viral load increases by more than 1 log10 after at least 2 weeks of appropriate antiviral therapy. In the absence of cell-mediated immunity following HSCT, viral loads may take several weeks to reduce despite effective treatment. However, it would be reasonable in this setting to initiate testing for antiviral resistance (21).
4. Antiviral resistance in CMV
Antiviral resistance in CMV occurs due to single nucleotide mutations in genes targeted by CMV antiviral drugs, such as DNA polymerase (UL54), terminase subunit (UL56, UL51 & UL89) and viral protein kinase (UL97). The genes and specific single nucleotide polymorphisms (SNPs) associated with CMV antiviral resistance are summarised in Table 1. Mutations in the viral protein kinase (UL97) are the most common mutations reported and reduce the phosphorylation of Ganciclovir, preventing Ganciclovir from being added to the growing DNA chain and causing it to be ineffective (29). Mutations in UL97 may also prevent its binding of Maribavir, causing Maribavir to become ineffective (30). Mutations in the viral DNA polymerase (UL54) can cause resistance to Ganciclovir, Cidofovir and Foscarnet. Some mutations cause increased exonuclease activity of the viral DNA polymerase, which allows the cleavage of areas containing Ganciclovir or Cidofovir thereby reducing their effectiveness (31). Structural changes in the viral DNA polymerase prevent its binding to Foscarnet, causing Foscarnet to become ineffective (32). Mutations in any of the genes that make up the viral terminase subunit (UL56, UL51 and UL89) can prevent its binding to Letermovir, allowing it to continue cleaving the concatemers and hence causing Letermovir to become ineffective (33).
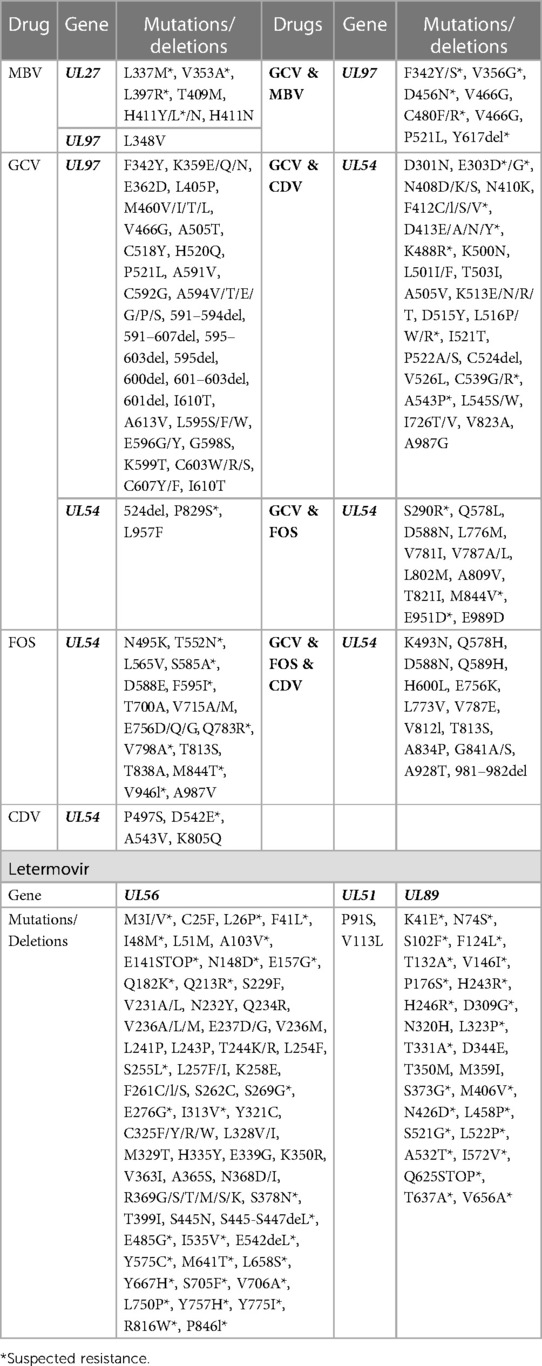
Table 1. List of mutations and deletions causing CMV antiviral resistance, ganciclovir [(GCV) including valganciclovir], cidofovir (CDV), foscarnet (FOS) and maribavir (MBV) (32, 33, 49, 85, 115–124).
Mutations in the CMV genome may arise under selective pressure, such as prolonged or subtherapeutic antiviral therapy (10, 34). Mixed infections in which different variants of CMV, exhibiting different susceptibility profiles occur. These are of particular concern in HSCT recipients who may experience prolonged periods of CMV reactivation despite prolonged antiviral therapy (26, 35–37).
The prevalence of Ganciclovir resistant CMV infections in HSCT recipients has been reported up to 9.4% (25, 38–42). Due to the limitations of resistance testing these rates may be under-estimated (40, 41, 43). Antiviral resistance is often associated with multiple resistance mutations. An Australian study found rates of infections in solid organ transplant (SOT) recipients harbouring both UL54 and UL97 mutations had increased over a six-year period (26). There is little information regarding mortality in antiviral resistant CMV infections. One study reported mortality to be 11% higher in SOT recipients with Ganciclovir resistant CMV infections, while two other studies reported mortality of 25% and 69% respectively (6, 39, 44).
Although Letermovir is a relatively new drug, there are reports of CMV resistance to Letermovir developing rapidly (45, 46). Maribavir may be useful in treating Ganciclovir resistant CMV infections (47, 48). Cross resistance to Maribavir and Ganciclovir arises rarely despite their dissimilar mechanisms of action (49). Mutations in UL27 gene have been associated with reduced effectiveness of Maribavir, however, this could be a result of counteractivity for the loss of the viral protein kinase, rather than causing Maribavir resistance (49).
5. CMV antiviral resistance testing
Sanger sequencing is the most commonly used method to identify mutations in UL97 and UL54 known to be associated with resistance to ganciclovir and foscarnet (26, 34, 37, 50). Sanger sequencing requires viral loads of at least 1,000 IU/ml for successful characterisation, and is unable to differentiate variants that make up less than 20%–30% of the overall viral population. As a consequence this method may fail to identify mixed infections, or low-levels of resistance in a predominantly susceptible population (35, 51). Patients should be tested for antiviral resistance whenever resistance is suspected, even after mutations have been identified, as additional resistance mutations can develop (26). The requirement of high viral loads, alongside the extended turnaround times can hinder the desirability of sequencing for antiviral resistance testing. Next generation sequencing (NGS) technologies are a higher resolution tool capable of detecting minority variants down to as little as 1%, and may be performed in samples with a lower viral load (52–54). Currently, due to the cost per sample, lack of availability of NGS within all pathology laboratories, specialised skills required for analysis, and scarcity of databases which summarise the clinically relevant antiviral resistance mutations for use in a bioinformatics pipeline, NGS assays are not widely used for detection of CMV antiviral resistance.
6. Novel diagnostic approaches
6.1. Interferon gamma release assay for CMV (IGRA-CMV)
A recently commercialised diagnostic assay is the IGRA-CMV which measures interferon-γ that is released by the patients' T-lymphocytes when exposed to CMV antigens ex vivo (55, 56). These assays indicate immunity to CMV and correlate with the subsequent risk of progression to CMV reactivation or disease. The IGRA-CMV has been evaluated in HSCT recipients and has demonstrated the potential to assist clinicians when to start and stop antiviral treatments (55–57). The ECIL7 supports the use of IGRA in addition to qPCR to support risk stratification and guide the treatment of HSCT recipients with CMV infections, but there are currently no clinically validated thresholds for intervention (21).
6.2. Digital PCR
Digital PCR offers advantages over conventional PCR methods by quantifying viral loads without the need for a calibration curve. It exhibits improved precision without a loss of sensitivity (58). It is also possible to quantify CMV antiviral resistant mutations, however this method requires unique assays for every mutation, in addition to specialised instrumentation (59). The role of digital PCR for CMV monitoring is currently limited by the workflow advantages of conventional PCR methods.
6.3. Viable and non-viable CMV
The COVID-19 pandemic has sparked discussions around viable and non-viable virus in patients with detectable SARS-CoV-2 long after clinical symptoms have ceased (60, 61). Persistent viraemia despite appropriate treatment complicates treatment decisions. Differentiating between viable and non-viable CMV could allow for early detection of drug failure and may also provide a means of assessing drug resistance without relying on detection of specific resistance mutations. To date no such commercial systems exist for CMV.
7. Established therapies
7.1. Ganciclovir
Ganciclovir is a first-line antiviral drug used for treating CMV (21). It acts as a guanosine analogue that is initially phosphorylated by viral protein kinase (UL97) (62–64). The phosphorylated form competes with guanosine triphosphate by incorporating itself into the growing DNA chain, stalling the DNA polymerase (UL54) and preventing further transcription of the viral DNA (64). Due to the requirement of CMV viral protein kinase activity for initial phosphorylation, Ganciclovir may have reduced efficacy at low CMV viral loads (65).
Importantly, Ganciclovir can also disrupt transcription of human cellular DNA, hence it can be toxic to the patient (66, 67). Ganciclovir is given intravenously, requiring patients to attend hospitals to receive treatment and often requiring central venous lines. Twenty years after the development of Ganciclovir, its prodrug, Valganciclovir was approved for use in 2001. Valganciclovir has the same mode of action and efficacy as Ganciclovir (68), however it can be taken orally and has a favourable toxicity profile (8). Ganciclovir may still be required in severe CMV infections and where oral absorption cannot be assumed (26). Effective ganciclovir exposures may be assessed by therapeutic drug monitoring (TDM), though this remains uncommon (69).
There is controversy surrounding appropriate dosing of Ganciclovir in paediatric patients. Institutional guidelines vary in their use of weight or body surface area-based dosing and there are few data to support a pharmacodynamic target. Ganciclovir is known to cause bone marrow suppression, and may cause higher rates of hepatotoxicity in children compared with adults, making appropriate dosing a priority (70). Though weight-based dosing achieved lower ganciclovir exposures than body-surface area based dosing in paediatric SOT recipients, these exposures achieved a virological clearance (71).
7.2. Foscarnet
Foscarnet binds to the viral DNA polymerase (UL54), occupying the position where phosphates of incoming deoxynucleotide triphosphates (dNTPs) would sit, hence preventing normal pyrophosphate release and halting the viral DNA polymerase (72). Foscarnet must be given intravenously, and is associated with significant renal toxicity (34). It has fewer effects on the bone marrow and as such, may be used as first-line therapy in the immediate post-transplant period. It is otherwise used as a second-line therapy when there are adverse events associated with ganciclovir or there is identified or suspected resistance to Ganciclovir (21, 34). Renal dysfunction caused by Foscarnet can persist in patients for up to 6 months after the discontinuation of the drug (34). Paediatric patients experience higher rates of Foscarnet related thrombocytopenia compared with adults (70). One study used a combination of Ganciclovir and Foscarnet to treat high-risk paediatric HSCT recipients and found alternating drugs daily was very effective in preventing the development of CMV disease, however 30% of patients discontinued treatment due to toxicity (73).
7.3. Cidofovir
Cidofovir is a cytosine analogue that is phosphorylated by cellular enzymes and incorporated into DNA to slow elongation (74). Cidofovir has a long intracellular half-life, which is advantageous as it is not orally available and must be administered intravenously (75). An orally available lipid prodrug of Cidofovir (Brincidofovir) failed to reduce CMV infection in a randomised controlled-trial of prophylaxis in adults undergoing HSCT, and caused significant gastro-intestinal toxicity which limited its use (76).
The half-life of Cidofovir is longer in paediatric patients, however it is similarly tolerated to adults (75). Cidofovir has limited effectiveness in treating Ganciclovir-resistant infections and is associated with significant ocular and renal toxicity in paediatric patients (77).
8. Emerging therapies
8.1. Letermovir
Letermovir was approved by the FDA in 2017 for prophylaxis in adult HSCT recipients at high risk of CMV reactivation (78). The CMV genome is circularised during replication, allowing it to replicate rapidly in both directions, the product of which is a very long strand of DNA of multiple sets of CMV genomes linked end to end, known as concatemers (79). These concatemers are normally cleaved and packaged into individual virions by the viral terminase subunit (UL51, UL56 & UL89); however, Letermovir binds to the viral terminase subunit, preventing this mechanism and leaving the long viral DNA incapacitated (80, 81).
Letermovir specifically targets CMV and has no effect on other herpesviruses (82), and can be administered both intravenously and orally, with side-effects reported to be uncommon (83). Patients receiving cyclosporin or tacrolimus (used for immunosuppression in transplant recipients) in addition to Letermovir must be monitored closely, as drug-drug interactions can lead to increased absorption of cyclosporin (84). As with the other drugs, there is evidence of resistance developing after treatment (85).
Letermovir significantly reduced the number of CMV infections compared to placebo in a Phase 3 randomised controlled trial of prophylaxis in adults, and was well tolerated (86). It has become the drug of choice for prophylaxis in centres in Germany, Italy, Japan, and the USA (87–90). Data are emerging to support the use of letermovir to prevent CMV in children following HSCT (91, 92), while it has also been used successfully as pre-emptive therapy (93, 94). A Phase 2 study of Letermovir prophylaxis in children undergoing HSCT is ongoing (ClinicalTrials.gov identifier NCT03940586) (95).
8.2. Maribavir
Maribavir is orally available and functions by competing with adenosine triphosphate (ATP) to bind to the viral protein kinase (48). When bound to Maribavir, the viral protein kinase is unable to phosphorylate nucleotides, resulting in halted transcription of the viral DNA (48). Maribavir was approved by the FDA in 2021 for treating CMV infections that were not responding to conventional therapies (96). Considering Maribavir prevents the viral protein kinase from phosphorylating nucleotides, it also prevents phosphorylation of Ganciclovir, causing it to become ineffective, hence Maribavir and Ganciclovir cannot be administered together (97). Maribavir is ineffective at preventing CMV when used as a prophylactic treatment, which may be explained by the non-essential nature of the viral protein kinase (CMV can recruit host cell kinases to continue viral replication) (98). Resistance may also develop rapidly, with one study reporting resistance developing at an average of 16 weeks after starting Maribavir therapy (52). To the extent of our knowledge, there have been no studies examining the tolerability of Maribavir in paediatric patients.
8.3. CMV-specific cytotoxic T cells (CMV-CTLs)
There has been substantial interest in the adoptive transfer of donor-derived CMV-CTLs to treat refractory CMV infections following HSCT since its first demonstration (99). The use of CMV-CTLs appears to accelerate CMV-specific immune reconstitution and reduce viral load (100), and is particularly attractive in the context of refractory or resistant infection (101). The techniques required to obtain CMV-CTLs are time-consuming and costly, so far limiting their widespread evaluation, and adoption into clinical practice. Despite this, their promise is reflected by inclusion in international guidelines such as ECIL7 which support their use for pre-emptive therapy in high-risk patients, and for the treatment of antiviral resistant infections (21).
Posoleucel is a third-party multi-virus T cell therapy which has been evaluated in children and adults undergoing HSCT. In an open-label Phase 2 study of 58 patients (including 18 children) there was a complete response in 11/24 patients and partial improvement in 12/24 patients with CMV infections suspected to be antiviral resistant. Posoleucel was effective in 10/12 patients with two or more viral infections. It was well tolerated with no evidence of an increased risk of GvHD (102). Larger studies are required to prove efficacy of this therapy and demonstrate the risk of GvHD, in addition to how the drug is tolerated in paediatric HSCT recipients.
8.4. CMV vaccines
There is presently no licensed vaccine to prevent CMV despite numerous candidates being evaluated in clinical trials (103). An mRNA vaccine candidate supported by Moderna TX, Inc (104). is currently undergoing a phase 3 trial in women of childbearing age, with the aim of preventing congenital CMV. It is expected to complete in 2025 (ClinicalTrials.gov identifier NCT05085366) (105). Also under development is a CMV bivalent subunit vaccine, which has demonstrated promising immune responses in mice, with the hopes to begin human trials in the coming years (106). The availability of a vaccine may not improve care for immunocompromised patients due to their inability to mount a suitable immune response.
9. Off label treatment options
9.1. Leflunomide
Leflunomide is an immunosuppressant used in rheumatoid arthritis. It has shown effectiveness as an off-label treatment for CMV infections by interfering with virion assembly (107). Successful treatment of Ganciclovir resistant infections has been reported with a combination of Foscarnet and Leflunomide (108). However, Leflunomide has displayed reduced effectiveness in the presence of higher CMV loads (109, 110). Despite Leflunomide being well tolerated for treating rheumatoid arthritis, higher doses are required to treat CMV, causing side-effects, such as diarrhoea, anaemia, and increased liver function tests (109). ECIL7 recommends Leflunomide is only used to treat antiviral resistant CMV infections and should be used in conjunction with antiviral therapy (21, 22).
9.2. Artesunate
Artesunate is an antimalarial drug that has been used off-label for the treatment of CMV infections (111, 112). Artesunate binds to a human cellular protein called vimentin; vimentin is normally cleaved during CMV infection, suggesting it has a role in controlling CMV. Artesunate binding prevents the cleavage of vimentin, in turn inhibiting CMV infection (113). Effectiveness of Artesunate in combination with conventional drugs has been demonstrated in cell culture (114). Clinical studies have reported mixed efficacy in controlling CMV infection in HSCT recipients (112), and its use is only recommended when resistance has developed to conventional drugs (21, 22).
10. Summary
CMV is effectively treated using conventional (Ganciclovir and Valganciclovir, Foscarnet and Cidofovir) and emerging antiviral drugs (Maribavir and Letermovir). There is also an immunological approach to treatment (CMV-CTLs) and off-label drugs that have shown some effectiveness in treating refractory CMV infections (Leflunomide and Artesunate). Antiviral resistance in CMV is a growing problem in children undergoing HSCT and there remain significant limitations in our diagnostic approach to resistance. NGS may guide a more nuanced approach to the treatment of mixed infections.
Author contributions
JH, ES, DW, and AI conceived the study, JH performed the literature search and drafted the manuscript. All authors contributed to the writing and review of the manuscript and are accountable for the content of the work. All authors contributed to the article and approved the submitted version.
Funding
This work was supported by the Children's Hospital Foundation (project ID RPC00095). Our work is also supported by an Australian Research Council Hub grant for antimicrobial resistance (project ID IH190100021).
Conflict of interest
The authors declare that the research was conducted in the absence of any commercial or financial relationships that could be construed as a potential conflict of interest.
Publisher's note
All claims expressed in this article are solely those of the authors and do not necessarily represent those of their affiliated organizations, or those of the publisher, the editors and the reviewers. Any product that may be evaluated in this article, or claim that may be made by its manufacturer, is not guaranteed or endorsed by the publisher.
References
1. Stagno S, Cloud GA. Working parents: the impact of day care and breast-feeding on cytomegalovirus infections in offspring. Proc Natl Acad Sci U S A. (1994) 91(7):2384–9. doi: 10.1073/pnas.91.7.2384
2. Seale H, MacIntyre CR, Gidding HF, Backhouse JL, Dwyer DE, Gilbert L. National serosurvey of cytomegalovirus in Australia. Clin Vaccine Immunol. (2006) 13(11):1181–4. doi: 10.1128/CVI.00203-06
3. Green ML, Leisenring WM, Xie H, Walter RB, Mielcarek M, Sandmaier BM, et al. CMV Reactivation after allogeneic HCT and relapse risk: evidence for early protection in acute myeloid leukemia. Blood. (2013) 122(7):1316–24. doi: 10.1182/blood-2013-02-487074
4. Teira P, Battiwalla M, Ramanathan M, Barrett AJ, Ahn KW, Chen M, et al. Early cytomegalovirus reactivation remains associated with increased transplant-related mortality in the current era: a CIBMTR analysis. Blood. (2016) 127(20):2427–38. doi: 10.1182/blood-2015-11-679639
5. Ozdemir E, Saliba RM, Champlin RE, Couriel DR, Giralt SA, de Lima M, et al. Risk factors associated with late cytomegalovirus reactivation after allogeneic stem cell transplantation for hematological malignancies. Bone Marrow Transplant. (2007) 40(2):125–36. doi: 10.1038/sj.bmt.1705699
6. Fisher CE, Knudsen JL, Lease ED, Jerome KR, Rakita RM, Boeckh M, et al. Risk factors and outcomes of ganciclovir-resistant cytomegalovirus infection in solid organ transplant recipients. Clin Infect Dis. (2017) 65(1):57–63. doi: 10.1093/cid/cix259
7. D’Souza A, Fretham C, Lee SJ, Arora M, Brunner J, Chhabra S, et al. Current use of and trends in hematopoietic cell transplantation in the United States. Biol Blood Marrow Transplant. (2020) 26(8):e177–82. doi: 10.1016/j.bbmt.2020.04.013
8. Sharma SK, Kumar S, Agrawal N, Singh L, Mukherjee A, Seth T, et al. Cytomegalovirus reactivation following hematopoietic stem cell transplantation. J Infect Dev Ctries. (2013) 7(12):1003–7. doi: 10.3855/jidc.2947
9. Fan J, Jing M, Yang M, Xu L, Liang H, Huang Y, et al. Herpesvirus infections in hematopoietic stem cell transplant recipients seropositive for human cytomegalovirus before transplantation. Int J Infect Dis. (2016) 46:89–93. doi: 10.1016/j.ijid.2016.03.025
10. Ljungman P, Brand R, Hoek J, de la Camara R, Cordonnier C, Einsele H, et al. Donor cytomegalovirus status influences the outcome of allogeneic stem cell transplant: a study by the European group for blood and marrow transplantation. Clin Infect Dis. (2014) 59(4):473–81. doi: 10.1093/cid/ciu364
11. Jaing TH, Chang TY, Chen SH, Wen YC, Yu TJ, Lee CF, et al. Factors associated with cytomegalovirus infection in children undergoing allogeneic hematopoietic stem-cell transplantation. Medicine (Baltimore). (2019) 98(4):e14172. doi: 10.1097/MD.0000000000014172
12. Rowe RG, Guo D, Lee M, Margossian S, London WB, Lehmann L. Cytomegalovirus infection in pediatric hematopoietic stem cell transplantation: risk factors for primary infection and cases of recurrent and late infection at a single center. Biol Blood Marrow Transplant. (2016) 22(7):1275–83. doi: 10.1016/j.bbmt.2016.04.004
13. George B, Pati N, Gilroy N, Ratnamohan M, Huang G, Kerridge I, et al. Pre-transplant cytomegalovirus (CMV) serostatus remains the most important determinant of CMV reactivation after allogeneic hematopoietic stem cell transplantation in the era of surveillance and preemptive therapy. Transpl Infect Dis. (2010) 12(4):322–9. doi: 10.1111/j.1399-3062.2010.00504.x
14. Hiwarkar P, Gaspar HB, Gilmour K, Jagani M, Chiesa R, Bennett-Rees N, et al. Impact of viral reactivations in the era of pre-emptive antiviral drug therapy following allogeneic haematopoietic SCT in paediatric recipients. Bone Marrow Transplant. (2013) 48(6):803–8. doi: 10.1038/bmt.2012.221
15. Silcock R, Mitchell K, Fraser C, Clark J. Epidemiology and outcome for viremia in children undergoing bone marrow transplant: a retrospective cohort study. Transpl Infect Dis. (2021) 23(4):e13580. doi: 10.1111/tid.13580
16. Marchesi F, Pimpinelli F, Dessanti ML, Gumenyuk S, Palombi F, Pisani F, et al. Evaluation of risk of symptomatic cytomegalovirus reactivation in myeloma patients treated with tandem autologous stem cell transplantation and novel agents: a single-institution study. Transpl Infect Dis. (2014) 16(6):1032–8. doi: 10.1111/tid.12309
17. Marchesi F, Pimpinelli F, Gumenyuk S, Renzi D, Palombi F, Pisani F, et al. Cytomegalovirus reactivation after autologous stem cell transplantation in myeloma and lymphoma patients: a single-center study. World J Transplant. (2015) 5(3):129–36. doi: 10.5500/wjt.v5.i3.129
18. Seckert CK, Renzaho A, Reddehase MJ, Grzimek NK. Hematopoietic stem cell transplantation with latently infected donors does not transmit virus to immunocompromised recipients in the murine model of cytomegalovirus infection. Med Microbiol Immunol. (2008) 197(2):251–9. doi: 10.1007/s00430-008-0094-1
19. Al Yazidi LS, Mitchell R, Palasanthiran P, O’Brien TA, McMullan B. Management and prevention of cytomegalovirus infection in paediatric hematopoietic stem cell transplant (HSCT) recipients: a binational survey. Pediatr Transplant. (2019) 23(5):e13458. doi: 10.1111/petr.13458
20. Camacho-Bydume C, Mauguen A, Rodriguez-Sanchez MI, Klein E, Kernan NA, Prockop S, et al. Time to initiation of pre-emptive therapy for cytomegalovirus impacts overall survival in pediatric hematopoietic stem cell transplant recipients. Cytotherapy. (2022) 24(4):428–36. doi: 10.1016/j.jcyt.2021.10.002
21. Ljungman P, de la Camara R, Robin C, Crocchiolo R, Einsele H, Hill JA, et al. Guidelines for the management of cytomegalovirus infection in patients with haematological malignancies and after stem cell transplantation from the 2017 European Conference on Infections in Leukaemia (ECIL 7). Lancet Infect Dis. (2019) 19(8):e260–72. doi: 10.1016/S1473-3099(19)30107-0
22. Styczynski J. Management of herpesvirus infections in hematopoietic cell transplant recipients. Transplantology. (2021) 2(1):8–21. doi: 10.3390/transplantology2010002
23. Burgan H, Gosteli G, Giovannini M, Lienhard R, Clerc O. Very-late-onset cytomegalovirus disease: a case-report and review of the literature. BMC Res Notes. (2017) 10(1):210. doi: 10.1186/s13104-017-2532-x
24. Limaye AP, Bakthavatsalam R, Kim HW, Randolph SE, Halldorson JB, Healey PJ, et al. Impact of cytomegalovirus in organ transplant recipients in the era of antiviral prophylaxis. Transplantation. (2006) 81(12):1645–52. doi: 10.1097/01.tp.0000226071.12562.1a
25. Li F, Kenyon KW, Kirby KA, Fishbein DP, Boeckh M, Limaye AP. Incidence and clinical features of ganciclovir-resistant cytomegalovirus disease in heart transplant recipients. Clin Infect Dis. (2007) 45(4):439–47. doi: 10.1086/519941
26. Iwasenko JM, Scott GM, Naing Z, Glanville AR, Rawlinson WD. Diversity of antiviral-resistant human cytomegalovirus in heart and lung transplant recipients. Transpl Infect Dis. (2011) 13(2):145–53. doi: 10.1111/j.1399-3062.2010.00584.x
27. Pang XL, Fox JD, Fenton JM, Miller GG, Caliendo AM, Preiksaitis JK, et al. Interlaboratory comparison of cytomegalovirus viral load assays. Am J Transplant. (2009) 9(2):258–68. doi: 10.1111/j.1600-6143.2008.02513.x
28. Hayden RT, Shahbazian MD, Valsamakis A, Boonyaratanakornkit J, Cook L, Pang XL, et al. Multicenter evaluation of a commercial cytomegalovirus quantitative standard: effects of commutability on interlaboratory concordance. J Clin Microbiol. (2013) 51(11):3811–7. doi: 10.1128/JCM.02036-13
29. Hantz S, Michel D, Fillet AM, Guigonis V, Champier G, Mazeron MC, et al. Early selection of a new UL97 mutant with a severe defect of ganciclovir phosphorylation after valaciclovir prophylaxis and short-term ganciclovir therapy in a renal transplant recipient. Antimicrob Agents Chemother. (2005) 49(4):1580–3. doi: 10.1128/AAC.49.4.1580-1583.2005
30. Chou S, Hakki M, Villano S. Effects on maribavir susceptibility of cytomegalovirus UL97 kinase ATP binding region mutations detected after drug exposure in vitro and in vivo. Antiviral Res. (2012) 95(2):88–92. doi: 10.1016/j.antiviral.2012.05.013
31. Nishiyama Y, Maeno K, Yoshida S. Characterization of human cytomegalovirus-induced DNA polymerase and the associated 3′-to-5′, exonuclease. Virology. (1983) 124(2):221–31. doi: 10.1016/0042-6822(83)90339-2
32. Park KR, Kim YE, Shamim A, Gong S, Choi SH, Kim KK, et al. Analysis of novel drug-resistant human cytomegalovirus DNA polymerase mutations reveals the role of a DNA-binding loop in phosphonoformic acid resistance. Front Microbiol. (2022) 13:771978. doi: 10.3389/fmicb.2022.771978
33. Chou S, Satterwhite LE, Ercolani RJ. New locus of drug resistance in the human cytomegalovirus UL56 gene revealed by in vitro exposure to letermovir and ganciclovir. Antimicrob Agents Chemother. (2018) 62(9):e00922–18. doi: 10.1128/AAC.00922-18
34. Avery RK, Arav-Boger R, Marr KA, Kraus E, Shoham S, Lees L, et al. Outcomes in transplant recipients treated with foscarnet for ganciclovir-resistant or refractory cytomegalovirus infection. Transplantation. (2016) 100(10):e74–80. doi: 10.1097/TP.0000000000001418
35. Sahoo MK, Lefterova MI, Yamamoto F, Waggoner JJ, Chou S, Holmes SP, et al. Detection of cytomegalovirus drug resistance mutations by next-generation sequencing. J Clin Microbiol. (2013) 51(11):3700–10. doi: 10.1128/JCM.01605-13
36. Volfova P, Lengerova M, Lochmanova J, Dvorakova D, Ricna D, Palackova M, et al. Detecting human cytomegalovirus drug resistant mutations and monitoring the emergence of resistant strains using real-time PCR. J Clin Virol. (2014) 61(2):270–4. doi: 10.1016/j.jcv.2014.07.008
37. Iwasenko JM, Scott GM, Ziegler JB, Rawlinson WD. Emergence and persistence of multiple antiviral-resistant CMV strains in a highly immunocompromised child. J Clin Virol. (2007) 40(2):152–5. doi: 10.1016/j.jcv.2007.07.003
38. Limaye AP, Corey L, Koelle DM, Davis CL, Boeckh M. Emergence of ganciclovir-resistant cytomegalovirus disease among recipients of solid-organ transplants. Lancet. (2000) 356(9230):645–9. doi: 10.1016/S0140-6736(00)02607-6
39. Minces LR, Nguyen MH, Mitsani D, Shields RK, Kwak EJ, Silveira FP, et al. Ganciclovir-resistant cytomegalovirus infections among lung transplant recipients are associated with poor outcomes despite treatment with foscarnet-containing regimens. Antimicrob Agents Chemother. (2014) 58(1):128–35. doi: 10.1128/AAC.00561-13
40. Hantz S, Garnier-Geoffroy F, Mazeron MC, Garrigue I, Merville P, Mengelle C, et al. Drug-resistant cytomegalovirus in transplant recipients: a French cohort study. J Antimicrob Chemother. (2010) 65(12):2628–40. doi: 10.1093/jac/dkq368
41. Eid AJ, Arthurs SK, Deziel PJ, Wilhelm MP, Razonable RR. Emergence of drug-resistant cytomegalovirus in the era of valganciclovir prophylaxis: therapeutic implications and outcomes. Clin Transplant. (2008) 22(2):162–70. doi: 10.1111/j.1399-0012.2007.00761.x
42. Allice T, Busca A, Locatelli F, Falda M, Pittaluga F, Ghisetti V. Valganciclovir as pre-emptive therapy for cytomegalovirus infection post-allogenic stem cell transplantation: implications for the emergence of drug-resistant cytomegalovirus. J Antimicrob Chemother. (2009) 63(3):600–8. doi: 10.1093/jac/dkn521
43. Almyroudis NG, Jakubowski A, Jaffe D, Sepkowitz K, Pamer E, O’Reilly RJ, et al. Predictors for persistent cytomegalovirus reactivation after T-cell-depleted allogeneic hematopoietic stem cell transplantation. Transpl Infect Dis. (2007) 9(4):286–94. doi: 10.1111/j.1399-3062.2007.00235.x
44. Isada CM, Yen-Lieberman B, Lurain NS, Schilz R, Kohn D, Longworth DL, et al. Clinical characteristics of 13 solid organ transplant recipients with ganciclovir-resistant cytomegalovirus infection. Transpl Infect Dis. (2002) 4(4):189–94. doi: 10.1034/j.1399-3062.2002.t01-1-02008.x
45. Cherrier L, Nasar A, Goodlet KJ, Nailor MD, Tokman S, Chou S. Emergence of letermovir resistance in a lung transplant recipient with ganciclovir-resistant cytomegalovirus infection. Am J Transplant. (2018) 18(12):3060–4. doi: 10.1111/ajt.15135
46. Jung S, Michel M, Stamminger T, Michel D. Fast breakthrough of resistant cytomegalovirus during secondary letermovir prophylaxis in a hematopoietic stem cell transplant recipient. BMC Infect Dis. (2019) 19(1):388. doi: 10.1186/s12879-019-4016-1
47. Avery RK, Marty FM, Strasfeld L, Lee I, Arrieta A, Chou S, et al. Oral maribavir for treatment of refractory or resistant cytomegalovirus infections in transplant recipients. Transpl Infect Dis. (2010) 12(6):489–96. doi: 10.1111/j.1399-3062.2010.00550.x
48. Shannon-Lowe CD, Emery VC. The effects of maribavir on the autophosphorylation of ganciclovir resistant mutants of the cytomegalovirus UL97 protein. Herpesviridae. (2010) 1(1):4. doi: 10.1186/2042-4280-1-4
49. Chou S, Song K, Wu J, Bo T, Crumpacker C. Drug resistance mutations and associated phenotypes detected in clinical trials of maribavir for treatment of cytomegalovirus infection. J Infect Dis. (2022) 226(4):576–84. doi: 10.1093/infdis/jiaa462
50. Scott GM, Isaacs MA, Zeng F, Kesson AM, Rawlinson WD. Cytomegalovirus antiviral resistance associated with treatment induced UL97 (protein kinase) and UL54 (DNA polymerase) mutations. J Med Virol. (2004) 74(1):85–93. doi: 10.1002/jmv.20150
51. Lopez-Aladid R, Guiu A, Mosquera MM, Lopez-Medrano F, Cofan F, Linares L, et al. Improvement in detecting cytomegalovirus drug resistance mutations in solid organ transplant recipients with suspected resistance using next generation sequencing. PLoS One. (2019) 14(7):e0219701. doi: 10.1371/journal.pone.0219701
52. Houldcroft CJ, Bryant JM, Depledge DP, Margetts BK, Simmonds J, Nicolaou S, et al. Detection of low frequency multi-drug resistance and novel putative maribavir resistance in immunocompromised pediatric patients with cytomegalovirus. Front Microbiol. (2016) 7:1317. doi: 10.3389/fmicb.2016.01317
53. Schindele B, Apelt L, Hofmann J, Nitsche A, Michel D, Voigt S, et al. Improved detection of mutated human cytomegalovirus UL97 by pyrosequencing. Antimicrob Agents Chemother. (2010) 54(12):5234–41. doi: 10.1128/AAC.00802-10
54. Garrigue I, Moulinas R, Recordon-Pinson P, Delacour ML, Essig M, Kaminski H, et al. Contribution of next generation sequencing to early detection of cytomegalovirus UL97 emerging mutants and viral subpopulations analysis in kidney transplant recipients. J Clin Virol. (2016) 80:74–81. doi: 10.1016/j.jcv.2016.04.017
55. Nesher L, Shah DP, Ariza-Heredia EJ, Azzi JM, Siddiqui HK, Ghantoji SS, et al. Utility of the enzyme-linked immunospot interferon-gamma-release assay to predict the risk of cytomegalovirus infection in hematopoietic cell transplant recipients. J Infect Dis. (2016) 213(11):1701–7. doi: 10.1093/infdis/jiw064
56. Yong MK, Cameron PU, Slavin M, Morrissey CO, Bergin K, Spencer A, et al. Identifying cytomegalovirus complications using the quantiferon-CMV assay after allogeneic hematopoietic stem cell transplantation. J Infect Dis. (2017) 215(11):1684–94. doi: 10.1093/infdis/jix192
57. Chemaly RF, El Haddad L, Winston DJ, Rowley SD, Mulane KM, Chandrasekar P, et al. Cytomegalovirus (CMV) cell-mediated immunity and CMV infection after allogeneic hematopoietic cell transplantation: the REACT study. Clin Infect Dis. (2020) 71(9):2365–74. doi: 10.1093/cid/ciz1210
58. Sedlak RH, Cook L, Cheng A, Magaret A, Jerome KR. Clinical utility of droplet digital PCR for human cytomegalovirus. J Clin Microbiol. (2014) 52(8):2844–8. doi: 10.1128/JCM.00803-14
59. Bogozalec Kosir A, Cvelbar T, Kammel M, Grunert HP, Zeichhardt H, Milavec M. Digital PCR method for detection and quantification of specific antimicrobial drug-resistance mutations in human cytomegalovirus. J Virol Methods. (2020) 281:113864. doi: 10.1016/j.jviromet.2020.113864
60. Cuevas-Ferrando E, Giron-Guzman I, Falco I, Perez-Cataluna A, Diaz-Reolid A, Aznar R, et al. Discrimination of non-infectious SARS-CoV-2 particles from fomites by viability RT-qPCR. Environ Res. (2022) 203:111831. doi: 10.1016/j.envres.2021.111831
61. Kim JY, Bae JY, Bae S, Cha HH, Kwon JS, Suh MH, et al. Diagnostic usefulness of subgenomic RNA detection of viable SARS-CoV-2 in patients with COVID-19. Clin Microbiol Infect. (2022) 28(1):101–6. doi: 10.1016/j.cmi.2021.08.009
62. Scott GM, Ng HL, Morton CJ, Parker MW, Rawlinson WD. Murine cytomegalovirus resistant to antivirals has genetic correlates with human cytomegalovirus. J Gen Virol. (2005) 86(Pt 8):2141–51. doi: 10.1099/vir.0.80910-0
63. Okleberry KM, Warren RP, Smee DF. Metabolism of ganciclovir and cidofovir in cells infected with drug-resistant and wild-type strains of murine cytomegalovirus. Antiviral Res. (1997) 35(2):83–90. doi: 10.1016/S0166-3542(97)00013-2
64. Wagner M, Michel D, Schaarschmidt P, Vaida B, Jonjic S, Messerle M, et al. Comparison between human cytomegalovirus pUL97 and murine cytomegalovirus (MCMV) pM97 expressed by MCMV and vaccinia virus: pM97 does not confer ganciclovir sensitivity. J Virol. (2000) 74(22):10729–36. doi: 10.1128/JVI.74.22.10729-10736.2000
65. Rose J, Emery VC, Kumar D, Asberg A, Hartmann A, Jardine AG, et al. Novel decay dynamics revealed for virus-mediated drug activation in cytomegalovirus infection. PLoS Pathog. (2017) 13(4):e1006299. doi: 10.1371/journal.ppat.1006299
66. Gohring K, Hamprecht K, Jahn G. Antiviral drug- and multidrug resistance in cytomegalovirus infected SCT patients. Comput Struct Biotechnol J. (2015) 13:153–9. doi: 10.1016/j.csbj.2015.01.003
67. El Helou G, Razonable RR. Safety considerations with current and emerging antiviral therapies for cytomegalovirus infection in transplantation. Expert Opin Drug Saf. (2019) 18(11):1017–30. doi: 10.1080/14740338.2019.1662787
68. Humar A, Kumar D, Preiksaitis J, Boivin G, Siegal D, Fenton J, et al. A trial of valganciclovir prophylaxis for cytomegalovirus prevention in lung transplant recipients. Am J Transplant. (2005) 5(6):1462–8. doi: 10.1111/j.1600-6143.2005.00866.x
69. Stockmann C, Roberts JK, Knackstedt ED, Spigarelli MG, Sherwin CM. Clinical pharmacokinetics and pharmacodynamics of ganciclovir and valganciclovir in children with cytomegalovirus infection. Expert Opin Drug Metab Toxicol. (2015) 11(2):205–19. doi: 10.1517/17425255.2015.988139
70. Heston SM, Young RR, Tanaka JS, Jenkins K, Vinesett R, Saccoccio FM, et al. Risk factors for CMV viremia and treatment-associated adverse events among pediatric hematopoietic stem cell transplant recipients. Open Forum Infect Dis. (2022) 9(2):ofab639. doi: 10.1093/ofid/ofab639
71. Peled O, Berkovitch M, Rom E, Bilavsky E, Bernfeld Y, Dorfman L, et al. Valganciclovir dosing for cytomegalovirus prophylaxis in pediatric solid-organ transplant recipients: a prospective pharmacokinetic study. Pediatr Infect Dis J. (2017) 36(8):745–50. doi: 10.1097/INF.0000000000001595
72. Oberg B. Antiviral effects of phosphonoformate (PFA, foscarnet sodium). Pharmacol Ther. (1989) 40(2):213–85. doi: 10.1016/0163-7258(89)90097-1
73. Shereck EB, Cooney E, van de Ven C, Della-Lotta P, Cairo MS. A pilot phase II study of alternate day ganciclovir and foscarnet in preventing cytomegalovirus (CMV) infections in at-risk pediatric and adolescent allogeneic stem cell transplant recipients. Pediatr Blood Cancer. (2007) 49(3):306–12. doi: 10.1002/pbc.21043
74. De Clercq E, Holy A, Rosenberg I, Sakuma T, Balzarini J, Maudgal PC. A novel selective broad-spectrum anti-DNA virus agent. Nature. (1986) 323(6087):464–7. doi: 10.1038/323464a0
75. Caruso Brown AE, Cohen MN, Tong S, Braverman RS, Rooney JF, Giller R, et al. Pharmacokinetics and safety of intravenous cidofovir for life-threatening viral infections in pediatric hematopoietic stem cell transplant recipients. Antimicrob Agents Chemother. (2015) 59(7):3718–25. doi: 10.1128/AAC.04348-14
76. Marty FM, Winston DJ, Chemaly RF, Mullane KM, Shore TB, Papanicolaou GA, et al. A randomized, double-blind, placebo-controlled phase 3 trial of oral brincidofovir for cytomegalovirus prophylaxis in allogeneic hematopoietic cell transplantation. Biol Blood Marrow Transplant. (2019) 25(2):369–81. doi: 10.1016/j.bbmt.2018.09.038
77. Mehta Steinke SA, Alfares M, Valsamakis A, Shoham S, Arav-Boger R, Lees L, et al. Outcomes of transplant recipients treated with cidofovir for resistant or refractory cytomegalovirus infection. Transpl Infect Dis. (2021) 23(3):e13521. doi: 10.1111/tid.13521
78. Novel Drug Approvals for 2017 (2019). Available at: https://www.fda.gov/drugs/new-drugs-fda-cders-new-molecular-entities-and-new-therapeutic-biological-products/novel-drug-approvals-2017 (cited August 23, 2022).
79. McVoy MA, Adler SP. Human cytomegalovirus DNA replicates after early circularization by concatemer formation, and inversion occurs within the concatemer. J Virol. (1994) 68(2):1040–51. doi: 10.1128/jvi.68.2.1040-1051.1994
80. Champier G, Couvreux A, Hantz S, Rametti A, Mazeron MC, Bouaziz S, et al. Putative functional domains of human cytomegalovirus pUL56 involved in dimerization and benzimidazole D-ribonucleoside activity. Antivir Ther. (2008) 13(5):643–54. doi: 10.1177/135965350801300504
81. Chou S. A third component of the human cytomegalovirus terminase complex is involved in letermovir resistance. Antiviral Res. (2017) 148:1–4. doi: 10.1016/j.antiviral.2017.10.019
82. Marschall M, Stamminger T, Urban A, Wildum S, Ruebsamen-Schaeff H, Zimmermann H, et al. In vitro evaluation of the activities of the novel anticytomegalovirus compound AIC246 (letermovir) against herpesviruses and other human pathogenic viruses. Antimicrob Agents Chemother. (2012) 56(2):1135–7. doi: 10.1128/AAC.05908-11
83. Chemaly RF, Ullmann AJ, Stoelben S, Richard MP, Bornhauser M, Groth C, et al. Letermovir for cytomegalovirus prophylaxis in hematopoietic-cell transplantation. N Engl J Med. (2014) 370(19):1781–9. doi: 10.1056/NEJMoa1309533
84. Kropeit D, von Richter O, Stobernack HP, Rubsamen-Schaeff H, Zimmermann H. Pharmacokinetics and safety of letermovir coadministered with cyclosporine A or tacrolimus in healthy subjects. Clin Pharmacol Drug Dev. (2018) 7(1):9–21. doi: 10.1002/cpdd.388
85. Chou S. Rapid in vitro evolution of human cytomegalovirus UL56 mutations that confer letermovir resistance. Antimicrob Agents Chemother. (2015) 59(10):6588–93. doi: 10.1128/AAC.01623-15
86. Marty FM, Ljungman P, Chemaly RF, Maertens J, Dadwal SS, Duarte RF, et al. Letermovir prophylaxis for cytomegalovirus in hematopoietic-cell transplantation. N Engl J Med. (2017) 377(25):2433–44. doi: 10.1056/NEJMoa1706640
87. Martino M, Pitino A, Gori M, Bruno B, Crescimanno A, Federico V, et al. Letermovir prophylaxis for cytomegalovirus infection in allogeneic stem cell transplantation: a real-world experience. Front Oncol. (2021) 11:740079. doi: 10.3389/fonc.2021.740079
88. Sassine J, Khawaja F, Shigle TL, Handy V, Foolad F, Aitken SL, et al. Refractory and resistant cytomegalovirus after hematopoietic cell transplant in the letermovir primary prophylaxis era. Clin Infect Dis. (2021) 73(8):1346–54. doi: 10.1093/cid/ciab298
89. Derigs P, Radujkovic A, Schubert ML, Schnitzler P, Schoning T, Muller-Tidow C, et al. Letermovir prophylaxis is effective in preventing cytomegalovirus reactivation after allogeneic hematopoietic cell transplantation: single-center real-world data. Ann Hematol. (2021) 100(8):2087–93. doi: 10.1007/s00277-020-04362-2
90. Yoshimura H, Satake A, Ishii Y, Ichikawa J, Saito R, Konishi A, et al. Real-world efficacy of letermovir prophylaxis for cytomegalovirus infection after allogeneic hematopoietic stem cell transplantation: a single-center retrospective analysis. J Infect Chemother. (2022) 28(9):1317–23. doi: 10.1016/j.jiac.2022.05.019
91. Kuhn A, Puttkammer J, Madigan T, Dinnes L, Khan S, Ferdjallah A, et al. Letermovir as cytomegalovirus prophylaxis in a pediatric cohort: a retrospective analysis. Transplant Cell Ther. (2023) 29(1):62 e1–e4. doi: 10.1016/j.jtct.2022.10.005
92. Daukshus NP, Cirincione A, Siver M, Mathew S, Kunvarjee B, Chan A, et al. Letermovir for cytomegalovirus prevention in adolescent patients following hematopoietic cell transplantation. J Pediatric Infect Dis Soc. (2022) 11(7):337–40. doi: 10.1093/jpids/piac017
93. Chiereghin A, Belotti T, Borgatti EC, Fraccascia N, Piccirilli G, Fois M, et al. Off-label use of letermovir as preemptive anti-cytomegalovirus therapy in a pediatric allogeneic peripheral blood stem cell transplant. Infect Drug Resist. (2021) 14:1185–90. doi: 10.2147/IDR.S296927
94. Korholz KF, Fuller MA, Hennies M, Holterhus M, Hagedorn S, Ahlmann M, et al. Letermovir for prophylaxis and pre-emptive therapy of cytomegalovirus infection in paediatric allogeneic haematopoietic cell transplant patients. Paediatr Drugs. (2023) 25(2):225–32. doi: 10.1007/s40272-022-00547-6
95. Letermovir Treatment in Pediatric Participants Following Allogeneic Haematopoietic Stem Cell Transplantation (HSCT) (MK-8228-030) (2022). Available at: https://clinicaltrials.gov/ct2/show/record/NCT03940586?term=letermovir&cntry=AU&draw=2&rank=1 (cited August 23, 2022).
96. Novel Drug Approvals for 2021 (2022). Available at: https://www.fda.gov/drugs/new-drugs-fda-cders-new-molecular-entities-and-new-therapeutic-biological-products/novel-drug-approvals-2021 (cited August 23, 2022).
97. Chou S, Ercolani RJ, Derakhchan K. Antiviral activity of maribavir in combination with other drugs active against human cytomegalovirus. Antiviral Res. (2018) 157:128–33. doi: 10.1016/j.antiviral.2018.07.013
98. Marty FM, Ljungman P, Papanicolaou GA, Winston DJ, Chemaly RF, Strasfeld L, et al. Maribavir prophylaxis for prevention of cytomegalovirus disease in recipients of allogeneic stem-cell transplants: a phase 3, double-blind, placebo-controlled, randomised trial. Lancet Infect Dis. (2011) 11(4):284–92. doi: 10.1016/S1473-3099(11)70024-X
99. Walter EA, Greenberg PD, Gilbert MJ, Finch RJ, Watanabe KS, Thomas ED, et al. Reconstitution of cellular immunity against cytomegalovirus in recipients of allogeneic bone marrow by transfer of T-cell clones from the donor. N Engl J Med. (1995) 333(16):1038–44. doi: 10.1056/NEJM199510193331603
100. Schmitt A, Tonn T, Busch DH, Grigoleit GU, Einsele H, Odendahl M, et al. Adoptive transfer and selective reconstitution of streptamer-selected cytomegalovirus-specific CD8+ T cells leads to virus clearance in patients after allogeneic peripheral blood stem cell transplantation. Transfusion. (2011) 51(3):591–9. doi: 10.1111/j.1537-2995.2010.02940.x
101. Ke P, Bao X, Zhou J, Li X, Zhuang J, He X, et al. Donor CMV-specific cytotoxic T lymphocytes successfully treated drug-resistant cytomegalovirus encephalitis after allogeneic hematopoietic stem cell transplantation. Hematology. (2020) 25(1):43–7. doi: 10.1080/16078454.2019.1710945
102. Pfeiffer T, Tzannou I, Wu M, Ramos C, Sasa G, Martinez C, et al. Posoleucel, an allogeneic, off-the-shelf multivirus-specific T-cell therapy, for the treatment of refractory viral infections in the post-HCT setting. Clin Cancer Res. (2023) 29(2):324–30. doi: 10.1158/1078-0432.CCR-22-2415
103. Plotkin SA, Boppana SB. Vaccination against the human cytomegalovirus. Vaccine. (2019) 37(50):7437–42. doi: 10.1016/j.vaccine.2018.02.089
104. John S, Yuzhakov O, Woods A, Deterling J, Hassett K, Shaw CA, et al. Multi-antigenic human cytomegalovirus mRNA vaccines that elicit potent humoral and cell-mediated immunity. Vaccine. (2018) 36(12):1689–99. doi: 10.1016/j.vaccine.2018.01.029
105. A Study to Evaluate the Efficacy, Safety, and Immunogenicity of mRNA-1647 Cytomegalovirus (CMV) Vaccine in Healthy Participants 16 to 40 Years of Age. (2022). Available at: https://clinicaltrials.gov/ct2/show/record/NCT05085366 (cited August 23, 2022).
106. Dasari V, Beckett K, Horsefield S, Ambalathingal G, Khanna R. A bivalent CMV vaccine formulated with human compatible TLR9 agonist CpG1018 elicits potent cellular and humoral immunity in HLA expressing mice. PLoS Pathog. (2022) 18(6):e1010403. doi: 10.1371/journal.ppat.1010403
107. Waldman WJ, Knight DA, Blinder L, Shen J, Lurain NS, Miller DM, et al. Inhibition of cytomegalovirus in vitro and in vivo by the experimental immunosuppressive agent leflunomide. Intervirology. (1999) 42(5–6):412–8. doi: 10.1159/000053979
108. Andrassy J, Illner WD, Rentsch M, Jaeger G, Jauch KW, Fischereder M. Leflunomide: a treatment option for ganciclovir-resistant cytomegalovirus infection after renal transplantation. NDT Plus. (2009) 2(2):149–51. doi: 10.1093/ndtplus/sfp004
109. Avery RK, Mossad SB, Poggio E, Lard M, Budev M, Bolwell B, et al. Utility of leflunomide in the treatment of complex cytomegalovirus syndromes. Transplantation. (2010) 90(4):419–26. doi: 10.1097/TP.0b013e3181e94106
110. Gokarn A, Toshniwal A, Pathak A, Arora S, Bonda A, Punatar S, et al. Use of leflunomide for treatment of cytomegalovirus infection in recipients of allogeneic stem cell transplant. Biol Blood Marrow Transplant. (2019) 25(9):1832–6. doi: 10.1016/j.bbmt.2019.04.028
111. Germi R, Mariette C, Alain S, Lupo J, Thiebaut A, Brion JP, et al. Success and failure of artesunate treatment in five transplant recipients with disease caused by drug-resistant cytomegalovirus. Antiviral Res. (2014) 101:57–61. doi: 10.1016/j.antiviral.2013.10.014
112. Wolf DG, Shimoni A, Resnick IB, Stamminger T, Neumann AU, Chou S, et al. Human cytomegalovirus kinetics following institution of artesunate after hematopoietic stem cell transplantation. Antiviral Res. (2011) 90(3):183–6. doi: 10.1016/j.antiviral.2011.03.184
113. Roy S, Kapoor A, Zhu F, Mukhopadhyay R, Ghosh AK, Lee H, et al. Artemisinins target the intermediate filament protein vimentin for human cytomegalovirus inhibition. J Biol Chem. (2020) 295(44):15013–28. doi: 10.1074/jbc.RA120.014116
114. Kaptein SJ, Efferth T, Leis M, Rechter S, Auerochs S, Kalmer M, et al. The anti-malaria drug artesunate inhibits replication of cytomegalovirus in vitro and in vivo. Antiviral Res. (2006) 69(2):60–9. doi: 10.1016/j.antiviral.2005.10.003
115. Chou S. Advances in the genotypic diagnosis of cytomegalovirus antiviral drug resistance. Antiviral Res. (2020) 176:104711. doi: 10.1016/j.antiviral.2020.104711
116. Lurain NS, Chou S. Antiviral drug resistance of human cytomegalovirus. Clin Microbiol Rev. (2010) 23(4):689–712. doi: 10.1128/CMR.00009-10
117. Razonable RR. Drug-resistant cytomegalovirus: clinical implications of specific mutations. Curr Opin Organ Transplant. (2018) 23(4):388–94. doi: 10.1097/MOT.0000000000000541
118. Jo H, Kwon DE, Han SH, Min SY, Hong YM, Lim BJ, et al. De novo genotypic heterogeneity in the UL56 region in cytomegalovirus-infected tissues: implications for primary letermovir resistance. J Infect Dis. (2020) 221(9):1480–7. doi: 10.1093/infdis/jiz642
119. Douglas CM, Barnard R, Holder D, Leavitt R, Levitan D, Maguire M, et al. Letermovir resistance analysis in a clinical trial of cytomegalovirus prophylaxis for hematopoietic stem cell transplant recipients. J Infect Dis. (2020) 221(7):1117–26. doi: 10.1093/infdis/jiz577
120. Van Leer Buter CC, de Voogd DWK, Blokzijl H, de Joode AAE, Berger SP, Verschuuren EAM, et al. Antiviral-resistant cytomegalovirus infections in solid organ transplantation in The Netherlands. J Antimicrob Chemother. (2019) 74(8):2370–6. doi: 10.1093/jac/dkz196
121. Komatsu TE, Hodowanec AC, Colberg-Poley AM, Pikis A, Singer ME, O’Rear JJ, et al. In-depth genomic analyses identified novel letermovir resistance-associated substitutions in the cytomegalovirus UL56 and UL89 gene products. Antiviral Res. (2019) 169:104549. doi: 10.1016/j.antiviral.2019.104549
122. Santos Bravo M, Plault N, Sanchez-Palomino S, Rodriguez C, Navarro Gabriel M, Mosquera MM, et al. Genotypic and phenotypic study of antiviral resistance mutations in refractory cytomegalovirus infection. J Infect Dis. (2022) 226(9):1528–36. doi: 10.1093/infdis/jiac349
123. Muller C, Tilloy V, Frobert E, Feghoul L, Garrigue I, Lepiller Q, et al. First clinical description of letermovir resistance mutation in cytomegalovirus UL51 gene and potential impact on the terminase complex structure. Antiviral Res. (2022) 204:105361. doi: 10.1016/j.antiviral.2022.105361
Keywords: haematopoietic stem cell transplant, cytomegalovirus, antiviral resistance, pediatric, emerging therapies
Citation: Hume J, Sweeney EL, Lowry K, Fraser C, Clark JE, Whiley DM and Irwin AD (2023) Cytomegalovirus in children undergoing haematopoietic stem cell transplantation: a diagnostic and therapeutic approach to antiviral resistance. Front. Pediatr. 11:1180392. doi: 10.3389/fped.2023.1180392
Received: 9 March 2023; Accepted: 15 May 2023;
Published: 30 May 2023.
Edited by:
Ramos Amador Jose T., Complutense University of Madrid, SpainReviewed by:
Jian Zhou, Henan Provincial Cancer Hospital, ChinaFeng Chen, The First Affiliated Hospital of Soochow University, China
Sheng-Li Xue, The First Affiliated Hospital of Soochow University, China
© 2023 Hume, Sweeney, Lowry, Fraser, Clark, Whiley and Irwin. This is an open-access article distributed under the terms of the Creative Commons Attribution License (CC BY). The use, distribution or reproduction in other forums is permitted, provided the original author(s) and the copyright owner(s) are credited and that the original publication in this journal is cited, in accordance with accepted academic practice. No use, distribution or reproduction is permitted which does not comply with these terms.
*Correspondence: Adam D. Irwin YS5pcndpbkB1cS5lZHUuYXU=