- 1Department of Children and Adolescents, University Children’s Hospital, Ruhr University of Bochum, Bochum, Germany
- 2Pediatric Practice (Dr. Voigt, Dr. Heier), Stadtbergen, Germany
- 3GS Bio-Inhalation GmbH, Headquarters & Logistics, Gemuenden, Germany
- 4AspiAir GmbH, Gemuenden, Germany
- 5Independent Statistical Consultant, Warendorf, Germany
- 6Department for Children and Adolescents, Allergology, Pulmonology and Cystic Fibrosis, University Hospital, Goethe University, Frankfurt, Germany
- 7Department of Pediatric Pneumology, University Children's Hospital, Ruhr University of Bochum, Bochum, Germany
- 8Department of Pediatric Pneumology and Allergology, University Children’s Hospital Schleswig-Holstein, Lübeck, Germany
Background: Available data on aerosol emissions among children and adolescents during spontaneous breathing are limited. Our aim was to gain insight into the role of children in the spread of severe acute respiratory syndrome coronavirus 2 (SARS-CoV-2) and whether aerosol measurements among children can be used to help detect so-called superspreaders—infected individuals with extremely high numbers of exhaled aerosol particles.
Methods: In this prospective study, the aerosol concentrations of SARS-CoV-2 PCR-positive and SARS-CoV-2 PCR-negative children and adolescents (2–17 years) were investigated. All subjects were asked about their current health status and medical history. The exhaled aerosol particle counts of PCR-negative and PCR-positive subjects were measured using the Resp-Aer-Meter (Palas GmbH, Karlsruhe, Germany) and compared using linear regression.
Results: A total of 250 children and adolescents were included in this study, 105 of whom were SARS-CoV-2 positive and 145 of whom were SARS-CoV-2 negative. The median age in both groups was 9 years (IQR 7–11 years). A total of 124 (49.6%) participants were female, and 126 (50.4%) participants were male. A total of 81.9% of the SARS-CoV-2-positive group had symptoms of viral infection. The median particle count of all individuals was 79.55 particles/liter (IQR 44.55–141.15). There was a tendency for older children to exhale more particles (1–5 years: 79.54 p/L; 6–11 years: 77.96 p/L; 12–17 years: 98.63 p/L). SARS-CoV-2 PCR status was not a bivariate predictor (t = 0.82, p = 0.415) of exhaled aerosol particle count; however, SARS-CoV-2 status was shown to be a significant predictor in a multiple regression model together with age, body mass index (BMI), COVID-19 vaccination, and past SARS-CoV-2 infection (t = 2.81, p = 0.005). COVID-19 vaccination status was a highly significant predictor of exhaled aerosol particles (p < .001).
Conclusion: During SARS-CoV-2 infection, children and adolescents did not have elevated aerosol levels. In addition, no superspreaders were found.
1. Introduction
Severe acute respiratory syndrome coronavirus 2 (SARS-CoV-2) is a novel coronavirus that was first detected in Wuhan, China in December 2019 (1). SARS-CoV-2 is an enveloped RNA virus with a size of 60–160 nm, which is similar in size to influenza viruses (2, 3). In March 2020, the World Health Organization (WHO) declared the global spread of the pathogen a pandemic (4). By December 2022, over 640 million confirmed cases and over 6.6 million deaths attributed to the pandemic had been recorded (5).
Respiratory infections are transmitted through direct contact with an infected person, through indirect contact with a contaminated surface (fomite), or via droplets and aerosols in the surroundings of an infected person (6, 7). Interestingly, the airborne transmission of virus-laden particles (aerosol transmission) was not initially considered a relevant route of transmission (8, 9). Accordingly, the WHO declared on March 28, 2020, that the virus was transmitted via large droplets that fell to the ground close to infected individuals, as well as by touching contaminated surfaces (10). In addition, the WHO recommendation that masks help to control the transmission of SARS-CoV-2 was given rather late (11). Currently, it is well established that aerosols play a major role in SARS-CoV-2 transmission (9, 12). In particular, so-called “superspreading events”, in which a high number of SARS-CoV-2 infections occur, cannot be explained only by droplet transmission or smear infection (13, 14). The same holds true for the observed differences between indoor and outdoor transmission (15).
The physical behavior of exhaled aerosol particles depends on their size, density, and shape, as well as the ambient temperature, humidity, and air circulation, among other factors (8). During normal breathing, the human lungs produce aerosol particles. These particles are generated in the peripheral airways during inhalation by the reopening of collapsed airways and are released during exhalation (16–19). Studies have already shown that there is interindividual variation in aerosol emissions as well as variation between different activities (20–22). For instance, it has been shown that aerosol production increases during singing or shouting (22–25). Most respiratory aerosol particles are much smaller than 5 µm and therefore can penetrate deep into the respiratory tract to the bronchioli and alveoli (26–30). Approximately 85% of all exhaled SARS-CoV-2 RNA was found in particles smaller than 5 µm (31).
Children infected with coronavirus disease 2019 (COVID-19) often present with mild or no symptoms, and life-threatening conditions or death are rare (32). This might be explained by the lower prevalence of preexisting conditions such as hypertension, diabetes, and pulmonary disease among children or by higher exposure to other seasonal coronaviruses, leading to higher antibody titers in children, which might give them some protection against SARS-CoV-2 (33–35). Moreover, it is known that the binding of the SARS-CoV-2 spike protein to angiotensin-converting enzyme-2 (ACE2) allows the virus to enter cells (36). It has been hypothesized that the function of ACE2 is not yet mature in children, and therefore, its binding capacity is lower (34, 37). Bunyavanich et al. suggested that lower expression of ACE2 in the nasal epithelium of children results in lower susceptibility to SARS-CoV-2 infection (38). The role of ACE2 is still under debate, however, and more research is needed (36).
Unlike among adults, there is little knowledge about aerosol emissions among children (39). The question of whether children contribute substantially to the spread of SARS-CoV-2 has not yet been resolved, despite many observational studies being conducted. The aim of this prospective study was to gain insights into aerosol emission among children and adolescents. Specifically, we aimed to gain further insight into the role of children in SARS-CoV-2 transmission and to examine the differences in aerosol concentrations and particle size between SARS-CoV-2 PCR-positive and SARS-CoV-2 PCR-negative children and adolescents.
2. Methods
2.1. Study design
A monocentric prospective study was conducted to investigate the concentrations and size distribution of exhaled aerosols from SARS-CoV-2-positive and SARS-CoV-2-negative children and adolescents aged 2–17 years. PCR analysis for SARS-CoV-2 was performed in all subjects who did not have a current SARS-CoV-2 PCR test result (max. interval 48 h) at the time of measurement. The study was approved by the Ethics Committee of the Medical Faculty of the Ruhr-University Bochum (number 21-7365) and registered under DRKS00028539 in the German Register of Clinical Studies (DRKS). Detailed information was provided to the subjects and their guardians, and the aim and procedure of the study, as well as potential risks, were explained. Written informed consent was obtained from all study participants.
The study was supported by the Palas Company which provided the measurement equipment and by the “Ina und Gerhard Scheuch Stiftung für Aerosolforschung”.
2.2. Subject recruitment and data collection
The subjects were recruited between November 2021 and April 2022. Potential study participants or their guardians were recruited at cooperating pediatricians' offices, schools, and sport clubs, either by approaching them directly or by handing them written information. Parents received further written information after expressing interest.
Participants in the study were between 2 and 17 years of age and were divided into three age groups (2–5, 6–11, 12–17 years). Aerosol measurements were performed in subjects with and without acute SARS-CoV-2 infection. Acute respiratory infection was defined by the presence of at least one of the following symptoms: cough, rhinitis, and fever, with symptom onset in the previous 72 h. Healthy subjects were SARS-CoV-2 negative as well as free of signs of acute upper respiratory tract infection, such as rhinitis, cough, or fever.
Subjects who were unable to undergo the aerosol measurement, understand the content of the study, or did not have consent provided by their guardian were excluded from the study.
Due to the course of the current SARS-CoV-2 pandemic, longitudinal measurement of subjects was not feasible.
2.3. Clinical investigations
Before aerosol measurement, all subjects or their guardians answered questions regarding their current health status (weight, height), previous diseases (especially cardiac and pulmonary), allergies, medication, coronavirus vaccination status, past SARS-CoV-2 infection, physical fitness and tobacco exposure. Subjects with acute upper respiratory tract infection were asked about the time course and symptoms (cough, rhinitis, fever, etc.). All subjects were tested for SARS-CoV-2 via PCR before aerosol measurement. Variant-specific PCR tests were used to detect virus variants (e.g., Delta or Omicron) in most of the participants.
2.4. Aerosol measurement
Measurements of the exhaled particle concentrations and size distribution were performed using the Resp-Aer-Meter (Palas GmbH, Karlsruhe, Germany). For this purpose, the principle of optical light scattering by means of a white light LED sensor of the Fidas® system was used. With this measuring method, exhaled particles between 145 nm and 10 µm could be detected with high resolution. In the aerosol sensor, a polychromatic light source creates a precisely defined optical measurement volume. Scattered light pulses are generated by the particles when they pass the optical light source. The particle number and size are determined by the number and intensity of the scattered light pulses. The temperature and relative humidity in the exhaled air were also measured and considered. An integrated heating element prevented condensation and allowed larger droplets to evaporate before they reached the sensor (40).
During the measurement, subjects inhaled and exhaled orally through a mouthpiece. The mouthpiece was connected to a HEPA filter via a T-piece which was connected to the Resp-Aer-Meter via a tube. Particle-free air was inhaled through the HEPA filter. Nasal breathing was prevented during the measurement process by a nose clip so that the measurement would not be influenced by environmental aerosols. Air was continuously drawn in from the Resp-Aer-Meter via a T-piece for sampling and directed to the sensor. A sterile-packed breathing set was used for each measurement. The breathing set consisted of a mouthpiece, T-piece, antistatic connecting tubing, HEPA filter, and nose clip (Figure 1).
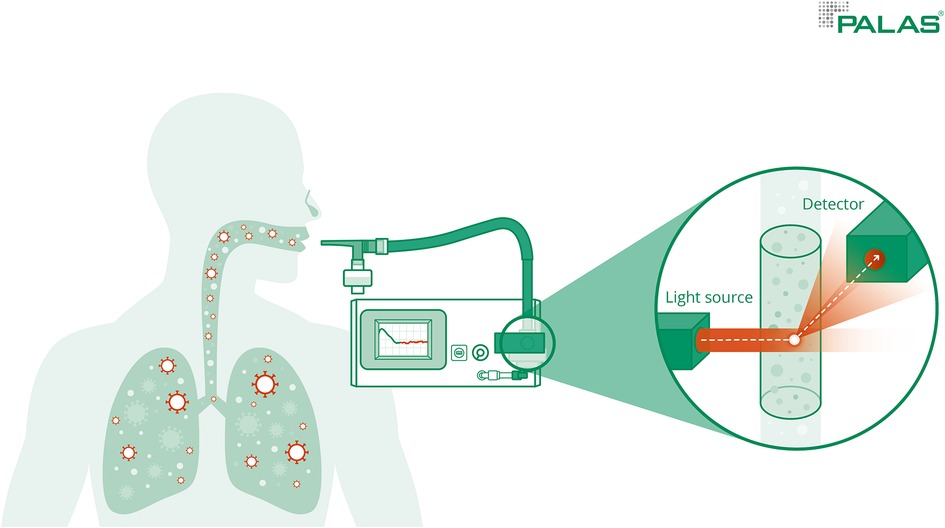
Figure 1. Measurement of aerosols. Measurement of the exhaled particle concentrations and size distribution was performed using the Resp-Aer-Meter using the principle of optical light scattering.
Prior to each measurement, a leak test was performed to ensure that the breathing set was fixed to the Resp-Aer-Meter without leakage. During the first minute of breathing, the washout phase took place. During this phase, environmental aerosol particles that were present in the lungs were washed out. This caused a rapid decrease in the particle concentration in the exhaled air. After a few breaths, the concentration did not further decline, and only the particles produced in the lungs were measured. The subsequent measurement phase lasted approximately 60–90 s. The results of the measurement were immediately available for documentation and evaluation. In addition to the mean value of the exhaled particle count/liter, the direct evaluation included a graphical chart of the measurement course and the aerosol particle size distribution (Figure 2).
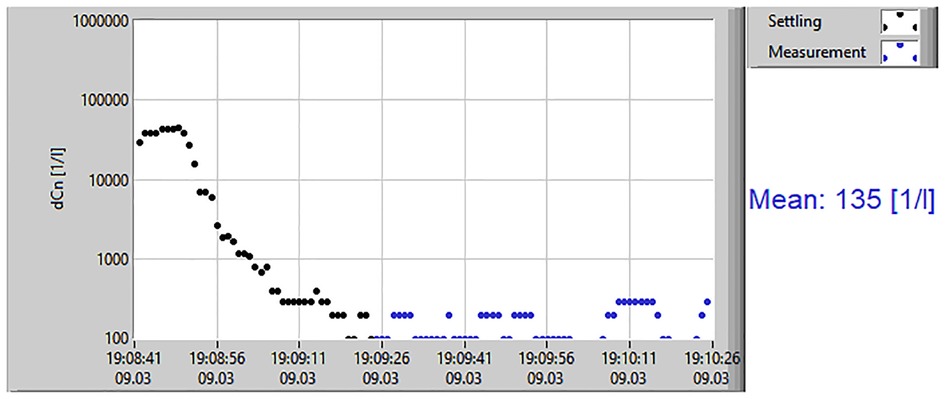
Figure 2. Results of aerosol measurement. The measurement results display the mean value of exhaled particles/liter, including a graphical chart of the measurement course.
2.5. Endpoints
The primary endpoint of this study was the aerosol concentration in exhaled air measured in particles/liter in children and adolescents with and without SARS-CoV-2 infection.
The secondary endpoints of the study were the influence of confounders such as age, sex, height, weight, BMI, symptoms, tobacco exposure, COVID-19 vaccination status, and SARS-CoV-2 infection on the exhaled aerosol concentration. In addition, the particle size distribution was analyzed.
2.6. Statistical methods
Statistical analysis was performed with R 4.1. The main hypothesis tested was that a current positive SARS-CoV-2 infection status was predictive of the particle count in the breath aerosol. Several additional demographic and health-related predictors, including age, sex, allergies, previous infections and diseases, and vaccination status, were considered.
All statistical testing was carried out using linear regression models, with the log-transformed particle count as the target variable. First, bivariate regression models were fitted for each predictor separately. Subsequently, all predictors were entered into a common regression model together with the SARS-CoV-2 infection status to detect potential interaction patterns.
Finally, a subset of predictors that applied only to SARS-CoV-2-positive patients, such as the time since symptom onset, or that were otherwise correlated with SARS-CoV-2 infection status, such as respiratory symptoms, were discarded. The remaining predictors were entered into a common multiple linear regression model, which included the main effects and interaction with the SARS-CoV-2 infection status for each predictor. This model was subjected to an automatized model selection procedure using the MASS::stepAIC function in R, which identified the subset of the original parameters that minimized the model's value on the Akaike Information Criterion (AIC). The AIC is a deviation measure for models that is penalized by model complexity (number of parameters). Hereafter, this model is referred to as the optimized model/regression.
3. Results
A total of 250 children and adolescents were included in this study. Of these subjects, 105 subjects tested positive for SARS-CoV-2 by PCR, and 145 subjects tested negative. Interestingly, testing for SARS-CoV-2 variants was performed in 89 (85%) subjects, and all tests (100%) were positive for the omicron variant. The characteristics of the subjects are shown in Table 1. The mean age of the SARS-CoV-2-positive group was slightly higher than that of the SARS-CoV-2-negative group (11 vs. 8 years). The sex distribution was almost balanced in both groups, with a slight predominance of the male sex in the SARS-CoV-2-positive group (54.3% vs. 47.6%). The median BMI was 17.96 in the SARS-CoV-2-positive group and 16.46 in the SARS-CoV-2-negative group.
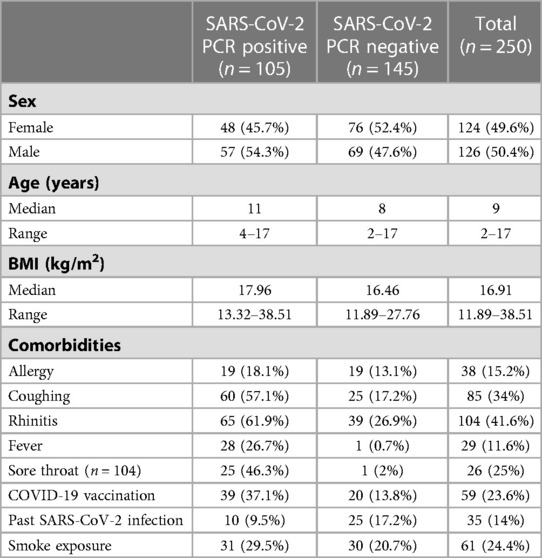
Table 1. Characteristics of severe acute respiratory syndrome coronavirus 2 (SARS-CoV-2) polymerase chain reaction (PCR)-positive and PCR-negative children and adolescents.
3.1. Medical history
Preexisting medical conditions were present in only 4.8% (5/105) of subjects in the SARS-CoV-2-positive group and in 2.8% (4/145) of the SARS-CoV-2-negative group. Allergies were reported in 18.1% (19/105) of SARS-CoV-2-positive subjects and in 13.1% (19/145) of SARS-CoV-2-negative subjects. A total of 9.5% (10/105) of SARS-CoV-2-positive subjects compared to 17.3% (25/145) of SARS-CoV-2-negative subjects were previously infected with SARS-CoV-2.
In the SARS-CoV-2-positive group, 37.1% (39/105) compared to 13.8% (20/145) of the SARS-CoV-2-negative subjects had been vaccinated for COVID-19. In the SARS-CoV-2-positive group, 29.5% of subjects lived in a smoking household; in the SARS-CoV-2-negative group, 20.87% of subjects lived in a smoking household.
As expected, 81.9% (86/105) of subjects in the SARS-CoV-2-positive group had symptoms of viral infection. The following symptoms were reported: cough in 57.1% (60/105) of subjects, rhinitis in 61.9% (65/105) of subjects, sore throat in 46.3% (25/54) of subjects, and fever in 26.7% (28/105) of subjects. In the SARS-CoV-2-negative group, 29% (42/145) of subjects had symptoms of acute infection and cough was reported in 17.2 (25/245) of subjects, rhinitis was reported in 26.9% (39/145) of subjects, sore throat was reported in 2% (1/50) of subjects, and fever was reported in 0.7% (1/145) of subjects.
3.2. Aerosol measurements
The median of all subjects' (SARS-CoV-2-positive and SARS-CoV-2-negative) mean particle counts was 79.55 p/L (IQR 44.55–141.15). Between SARS-CoV-2-positive subjects (median 82.72 p/L, IQR 44.55–149.52) and SARS-CoV-2-negative subjects (median 79.55, IQR 44.55–136.78), there was no significant difference in the number of exhaled particles (Figure 3). In the bivariate regression model, the aerosol particle count did not differ between the positive and negative groups (t = 0.82, p = 0.415). However, SARS-CoV-2 status was shown to be a significant predictor in the optimized model when controlling for the influence of age, BMI, vaccination status and past SARS-CoV-2 infection (t = .2.81 p = 0.005). Age had no effect on the aerosol particle count (bivariate model: t = 1.18, p = 0.24, optimized model: t = −1.68, p = 0.094), but there was a tendency for adolescents (age group 12–17) to exhale more particles than younger children (age group 2–5; 98 vs. 79 p/L) (Figure 4). Past SARS-CoV-2 infection was not a bivariate predictor (t = 1.4, p = 0.163) of the number of aerosol particles, but in the common regression model with age, BMI, SARS-CoV-2 status, and vaccination status, it showed a significant association with the number of exhaled aerosol particles (t = 2.26, p = 0.025) (Figure 5). The median mean particle count was 133 p/L among subjects with prior COVID-19 vaccination vs. 74.8 p/L among subjects without COVID-19 vaccination. Therefore, COVID-19 vaccination status was a highly significant predictor of exhaled aerosol particle count in the bivariate as well as the optimized model (p < 0.001) (Figure 6). In addition, there were no significant differences in the number of exhaled aerosol particles due to sex (p = 0.263), cough (p = 0.934), rhinitis (p = 0.472), sore throat (p = 0.423), fever (p = 0.343), allergies (p = 0.31), tobacco exposure (p = 0.332), and preexisting conditions (p = 0.605). There was a significant difference in the size distribution of exhaled aerosols between SARS-CoV-2- positive and SARS-CoV-2-negative subjects (p = 0.041). Although the median particle size in both groups was 0.21 µm, the distribution was much narrower in the SARS-CoV-2-positive group. The particle size was predominantly less than 0.5 µm in both groups (Figure 7).
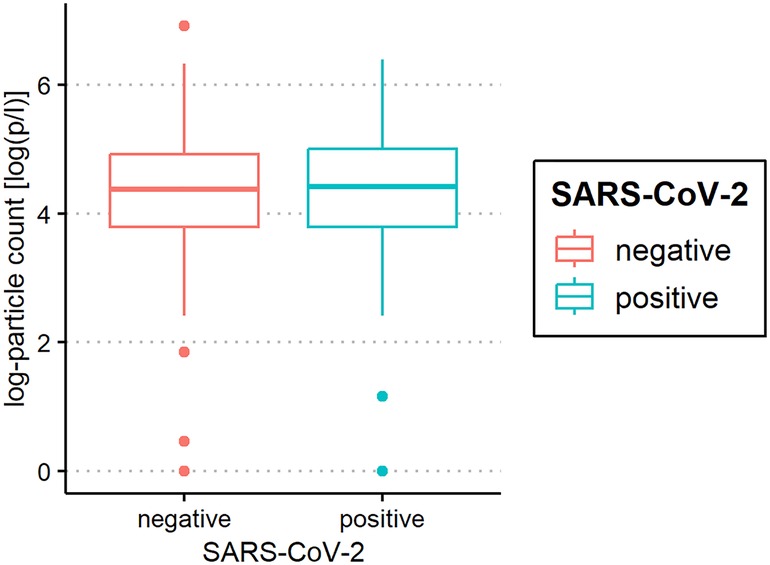
Figure 3. Aerosol measurement of severe acute respiratory syndrome coronavirus 2 (SARS-CoV-2) polymerase chain reaction (PCR) -positive and PCR-negative children and adolescents. Exhaled particle counts (in particles/liter, displayed on a logarithmic scale, y-axis) in SARS-CoV-2- PCR-positive and PCR-negative children and adolescents (x-axis).
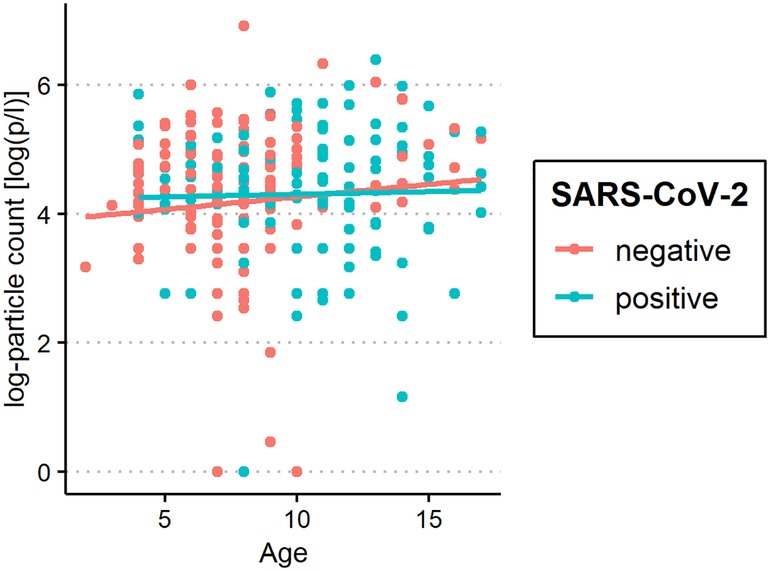
Figure 4. Aerosol measurement in relation to age among severe acute respiratory syndrome coronavirus 2 (SARS-CoV-2) polymerase chain reaction (PCR)-positive and PCR-negative subjects. Exhaled particle counts (in particles/liter, displayed on a logarithmic scale, y-axis) in SARS-CoV-2 PCR-positive and PCR-negative children and adolescents displaying the relation to age (x-axis).
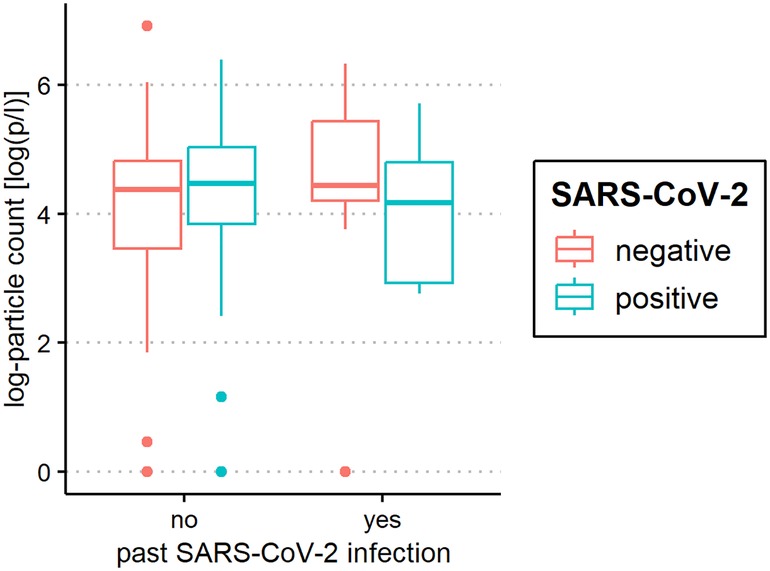
Figure 5. Aerosol measurement in relation to past severe acute respiratory syndrome coronavirus 2 (SARS-CoV-2) infection. Exhaled particle counts (in particles/liter, displayed on a logarithmic scale, y-axis) in SARS-CoV-2 polymerase chain reaction (PCR) -positive and PCR-negative children and adolescents displaying the relation to past coronavirus disease 2019 (COVID-19) infection (x-axis).
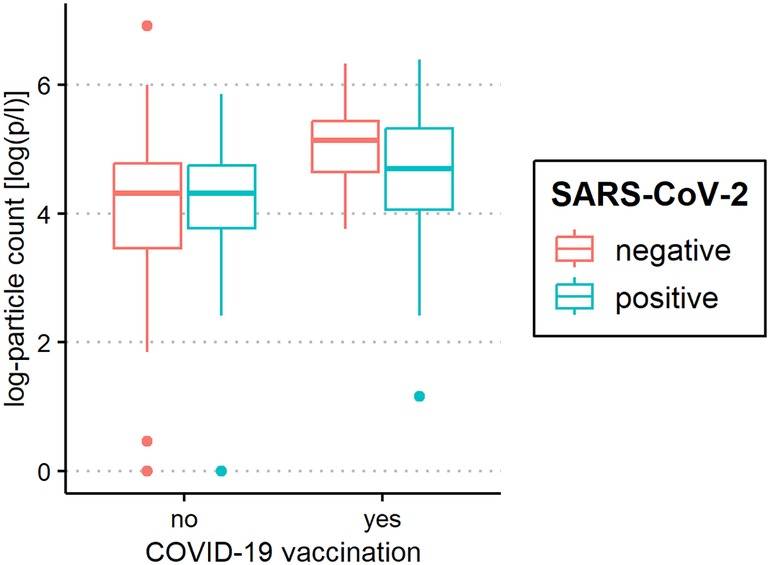
Figure 6. Aerosol measurement in relation to coronavirus disease 2019 (COVID-19) vaccination status. Exhaled particle counts (in particles/liter, displayed on a logarithmic scale, y-axis) in severe acute respiratory syndrome coronavirus 2 (SARS-CoV-2) polymerase chain reaction (PCR) -positive and PCR-negative children and adolescents displaying the relation to COVID-19 vaccination status (x-axis).
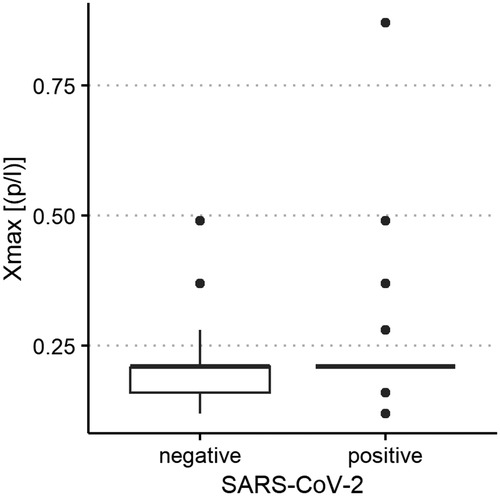
Figure 7. Aerosol particle size distribution of severe acute respiratory syndrome coronavirus 2 (SARS-CoV-2) polymerase chain reaction (PCR)-positive and PCR-negative children and adolescents. Maximum size of exhaled aerosol particles (in µm, displayed on the y-axis) in SARS-CoV-2 PCR-positive and PCR-negative children and adolescents (x-axis).
4. Discussion
The primary route of transmission of SARS-CoV-2 is via aerosols, as previously demonstrated in several studies (12, 30, 41). Children and adolescents have been shown to transmit the virus, yet they do not appear to be the drivers of virus spread (13, 41). It has been reported several times that transmission from adults to children is more common than vice versa (37, 42). Overall, children and adolescents appear to be less infectious than adults with SARS-CoV-2 (33, 43, 44). Presumably, the lower expression of ACE-2 receptors in children also results in lower susceptibility to SARS-CoV-2 since SARS-CoV-2 enters the body via human ACE-2 receptors (37, 38).
We found particle concentrations in children to be substantially lower than those in healthy adults, where an average concentration of 252 p/L has been reported previously using the same method (39). This might be due to the lower number of alveoli and terminal bronchioli in children's respiratory tracts, as these structures are thought to be the origin of aerosol production (41, 45, 46). Additionally, differences in respiratory maneuvers as well as surfactant production may influence aerosol production. Children also have a lower respiratory minute volume than adults. Edwards et al. described a correlation between the number of aerosol particles exhaled and age (47). However, a direct relationship between aerosol particle exhalation and age group was not observed in this study, but there was a tendency for older children to exhale more particles than younger children (98 vs. 79 p/L).
Contrary to previous findings of Edwards et al. and Gutmann et al. in adults, children suffering from SARS-CoV-2 infection were not found to exhale significantly more aerosol particles than uninfected children in this study (82.72 vs. 79.55 p/L). Additionally, no infected children were found to breathe more than 595 p/L, whereas so-called superspreaders in studies among adults exhaled >5,000 particles/liter and accounted for 15.6% of infected adults (39). At first glance, our aerosol measurements do not match the data from Gutmann et al. (48). This study reported significantly increased exhaled aerosol levels in SARS-CoV-2 PCR-positive children and adolescents (median 355.0 p/L) compared to SARS-CoV-2 PCR-negative participants (median 195.0 p/L; p < 0.001). One possible explanation is that Gutmann et al. measured aerosols when the delta variant was most prevalent from February–December 2021, whereas most of our measurements were performed when the omicron variant was the predominant variant. Indeed, 85% of our SARS-CoV-2-PCR-positive subjects were tested for virus variants, and in all cases, the omicron variant was detected, which is the predominant virus currently in Germany. Although the symptoms, such as runny nose, fever, and cough, of the delta and omicron COVID-19 variants are similar, several studies have shown that omicron causes milder disease. Apparently, patients infected with the omicron variant had less involvement of the lower respiratory tract and a reduced likelihood of hospital admission (49–52). There is increasing evidence that the SARS-CoV-2 omicron variant exhibits altered cell tropism to escape the immune pressure against ACE2-dependent viral entry provided by vaccination (53). Interestingly, it was demonstrated that the omicron variant replicates faster than other SARS-CoV-2 variants studied in the bronchi but less efficiently in the lung parenchyma (54). Thus, it is tempting to speculate that the delta variant may induce higher levels of aerosols due to higher parenchyma involvement than the omicron variant (55). In addition, Gutman et al. showed that patients with respiratory failure and pneumonia had significantly higher aerosol levels than patients with mild COVID-19 infection in ambulatory care (39).
Interestingly, in this study, the number of particles increased significantly after COVID-19 vaccination. The underlying mechanism of this finding is unclear. There are some reports that surfactant production is altered during COVID-19 infection (56, 57). Sinnberg et al. demonstrated that IgA autoantibodies to pulmonary surfactant proteins B and C are detectable in patients with COVID-19 and that these autoantibodies impair the ability of pulmonary surfactant to decrease surface tension (56). However, we do not know if such a phenomenon is present after COVID-19 vaccination.
This study had some limitations. For example, only mildly ill, nonhospitalized participants were included. It seems reasonable to assume that the number of exhaled aerosol particles is higher, especially in severely ill SARS-CoV-2-positive individuals, for whom respiratory tract damage can be assumed. Additionally, the measurement could not always be performed at the same time as the PCR test, but there was a maximum of 48 h between the measurement of the number of aerosol particles and the PCR test. The participants were not tested for any other respiratory pathogens besides SARS-CoV-2. Measurements of SARS-CoV-2-positive subjects were performed at different time points during the infection. Longitudinal measurements would be needed to assess the dynamics of aerosol production during the course of infection. Different environmental factors could also have influenced the results. Ambient conditions such as the humidity, season (weather, pollen count, etc.), environmental aerosols (urban area vs. rural area), were not held constant, even though they may play a role in the formation as well as in the measurement of aerosol particles. In addition to the interindividual variation in the measurement of exhaled aerosol particles, the measurements were also influenced by the participant's compliance and breathing technique. In particular, it was difficult for some young children of preschool and primary school age to keep the mouthpiece completely tight during the measurement and carry out the study procedures until completion. In this study, the majority of subjects were infected with the omicron variant of SARS-CoV-2. Previous studies that were carried out earlier in the pandemic likely had a very different distribution of SARS-CoV-2 variants, which might have influenced the number of exhaled aerosol particles. In this study, PCR or viral cultures of virus-containing particles were not analyzed. Furthermore, the participants were not asked to provide the exact date of their vaccination, so there are no data available about the time elapsed between vaccination and measurements.
In summary, the values of exhaled aerosol particles in children and adolescents are substantially lower overall than those in the studies conducted thus far in adults. Our results indicate that the measurement of exhaled aerosol particles is not suitable as a testing tool in children and adolescents to interrupt chains of infection. Although this study has shown that aerosol measurements in children and adolescents cannot detect and break chains of infection, it has provided further insight into aerosol production in this age group. Further studies are needed to gain a better understanding of the influencing factors on aerosol production.
Data availability statement
The raw data supporting the conclusions of this article will be made available by the authors, without undue reservation.
Ethics statement
The studies involving human participants were reviewed and approved by Ethics Committee of the Medical Faculty of the Ruhr-University Bochum. Written informed consent to participate in this study was provided by the participants’ legal guardian/next of kin.
Author contributions
PS, GS, RN and FB contributed to conception and design of the study. GS and RN organized the measurement equipment. PS organized the database. PS and MK performed the statistical analysis. PS wrote the first draft of the manuscript. PS, GS, MK, TL, SZ contributed to manuscript revision. All authors contributed to the article and approved the submitted version.
Funding
This study was supported by the Palas Company, which provided the measurement equipment, and by the Ina und Gerhard Scheuch Stiftung für Aerosolforschung.
Acknowledgements
We thank all children and adolescents who participated in this study and their guardians for their support. We acknowledge support by the Open Access Publication Funds of the Ruhr-Universität Bochum.
Conflict of interest
SZ reports, received grants from Palas GmbH, grants and personal fees from Allergy Therapeutics GmbH, grants and personal fees from Böhringer Ingelheim, personal fees from Novartis GmbH, personal fees from Lofarma GmbH, personal fees from IMS HEALTH GmbH & Co. OHG, personal fees from GSK, personal fees from Stallergen, personal fees from Engelhard Arzeneimittel, personal fees from Sanofi-Pasteur, personal fees from AstraZeneca, personal fees from Erydel, outside the submitted work. GS was employed by GS Bio-Inhalation GmbH, Headquarters & Logistics. RN was employed by AspiAir GmbH. MK was employed by Independent Statistical Consultant. The remaining authors declare that the research was conducted in the absence of any commercial or financial relationships that could be construed as a potential conflict of interest.
Publisher's note
All claims expressed in this article are solely those of the authors and do not necessarily represent those of their affiliated organizations, or those of the publisher, the editors and the reviewers. Any product that may be evaluated in this article, or claim that may be made by its manufacturer, is not guaranteed or endorsed by the publisher.
References
1. Huang C, Wang Y, Li X, Ren L, Zhao J, Hu Y, et al. Clinical features of patients infected with 2019 novel coronavirus in Wuhan, China. Lancet. (2020) 395:497–506. doi: 10.1016/S0140-6736(20)30183-5
2. Zhu N, Zhang D, Wang W, Li X, Yang B, Song J, et al. A novel coronavirus from patients with pneumonia in China, 2019. N Engl J Med. (2020) 382:727–33. doi: 10.1056/Nejmoa2001017
3. VM M, RW W. Electron micrography of the virus of influenza. Nature. (1946) 157:263. doi: 10.1038/157263a0
4. Cucinotta D, Vanelli M. Who declares COVID-19 A pandemic. Acta Biomed. (2020) 91:157–60. doi: 10.23750/Abm.V91i1.9397
5. Who coronavirus (Covid-19) dashboard | who coronavirus (Covid-19) dashboard with vaccination data (2022). Available at: https://Covid19.Who.Int/?Adgroupsurvey={Adgroupsurvey}&Gclid=Cjwkcaia7vwcbhbueiwaxieitinyj-Atbahkxgn_M5qik55w6hswy2k5fci1mx5pv_G57ppkmdhk2xoc-Zaqavd_Bwe (Accessed December 17, 2022).
6. KP F. Particle sizes of infectious aerosols: implications for infection control. Lancet Respir Med. (2020) 8:914–24. doi: 10.1016/S2213-2600(20)30323-4
7. Van Doremalen N, Bushmaker T, Morris DH, Holbrook MG, Gamble A, Williamson BN, et al. Aerosol and surface stability of sars-cov-2 as compared with sars-cov-1. N Engl J Med. (2020) 382:1564–7. doi: 10.1056/Nejmc2004973
8. Wang B, Zhang A, Sun JL, Liu H, Hu J, Xu LX. Study of sars transmission via liquid droplets in air. J Biomech Eng. (2005) 127:32–8. doi: 10.1115/1.1835350
9. Morawska L, Milton DK. It is time to address airborne transmission of coronavirus disease 2019 (COVID-19). Clin Infect Dis. (2020) 71:2311–3. doi: 10.1093/Cid/Ciaa939
10. Modes of transmission of virus causing Covid-19: implications for IPC precaution recommendations (2022). Available at: https://www.Who.Int/News-Room/Commentaries/Detail/Modes-Of-Transmission-Of-Virus-Causing-Covid-19-Implications-For-Ipc-Precaution-Recommendations (Accessed December 18, 2022).
11. Coronavirus disease (Covid-19) situation reports (2022). Available at: https://www.Who.Int/Emergencies/Diseases/Novel-Coronavirus-2019/Situation-Reports/ (Accessed December 18, 2022).
12. Morawska L, Cao J. Airborne transmission of Sars-Cov-2: the world should face the reality. Environ Int. (2020) 139:105730. doi: 10.1016/J.Envint.2020.105730
13. Miller SL, Nazaroff WW, Jimenez JL, Boerstra A, Buonanno G, Dancer SJ, et al. Transmission of Sars-Cov-2 by inhalation of respiratory aerosol in the skagit valley chorale superspreading event. Indoor Air. (2021) 31:314–23. doi: 10.1111/Ina.12751
14. Li Y, Qian H, Hang J, Chen X, Cheng P, Ling H, et al. Probable airborne transmission of Sars-Cov-2 in A poorly ventilated restaurant. Build Environ. (2021) 196:107788. doi: 10.1016/J.Buildenv.2021.107788
15. Qian H, Miao T, Liu L, Zheng X, Luo D, Li Y. Indoor transmission of Sars-Cov-2. Indoor Air. (2021) 31:639–45. doi: 10.1111/Ina.12766
16. Bake B, Larsson P, Ljungkvist G, Ljungström E, Olin A-C. Exhaled particles and small airways. Respir Res. (2019) 20:8. doi: 10.1186/S12931-019-0970-9
17. Almstrand A-C, Bake B, Ljungström E, Larsson P, Bredberg A, Mirgorodskaya E, et al. Effect of airway opening on production of exhaled particles. J Appl Physiol. (2010) 108:584–8. doi: 10.1152/Japplphysiol.00873.2009
18. Johnson GR, Morawska L. The mechanism of breath aerosol formation. J Aerosol Med Pulm Drug Deliv. (2009) 22:229–37. doi: 10.1089/Jamp.2008.0720
19. Fabian P, Brain J, Houseman EA, Gern J, Milton DK. Origin of exhaled breath particles from healthy and human rhinovirus-infected subjects. J Aerosol Med Pulm Drug Deliv. (2011) 24:137–47. doi: 10.1089/Jamp.2010.0815
20. Scheuch G. Breathing is enough: for the spread of influenza virus and Sars-Cov-2 by breathing only. J Aerosol Med Pulm Drug Deliv. (2020) 33:230–4. doi: 10.1089/Jamp.2020.1616
21. Morawska L, Johnson GR, Ristovski ZD, Hargreaves M, Mengersen K, Corbett S, et al. Size distribution and sites of origin of droplets expelled from the human respiratory tract during expiratory activities. J Aerosol Sci. (2009) 40:256–69. doi: 10.1016/J.Jaerosci.2008.11.002
22. Mürbe D, Kriegel M, Lange J, Rotheudt H, Fleischer M. Aerosol emission in professional singing of classical music. Sci Rep. (2021) 11:14861. doi: 10.1038/S41598-021-93281-X
23. Mürbe D, Kriegel M, Lange J, Schumann L, Hartmann A, Fleischer M. Aerosol emission of adolescents voices during speaking, singing and shouting. PloS One. (2021) 16:E0246819. doi: 10.1371/Journal.Pone.0246819
24. Alsved M, Matamis A, Bohlin R, Richter M, Bengtsson P-E, Fraenkel C-J, et al. Exhaled respiratory particles during singing and talking. Aerosol Sci Technol. (2020) 54:1245–8. doi: 10.1080/02786826.2020.1812502
25. Asadi S, Wexler AS, Cappa CD, Barreda S, Bouvier NM, Ristenpart WD. Aerosol emission and superemission during human speech increase with voice loudness. Sci Rep. (2019) 9:2348. doi: 10.1038/S41598-019-38808-Z
26. Milton DK. A Rosetta stone for understanding infectious drops and aerosols. J Pediatric Infect Dis Soc. (2020) 9:413–5. doi: 10.1093/Jpids/Piaa079
27. Fabian P, Mcdevitt JJ, Dehaan WH, Fung RO, Cowling BJ, Chan KH, et al. Influenza virus in human exhaled breath: an observational study. PloS One. (2008) 3:E2691. doi: 10.1371/Journal.Pone.0002691
28. Tellier R. Aerosol transmission of influenza A virus: a review of new studies. J R Soc Interface. (2009) 6(Suppl 6):S783–90. doi: 10.1098/Rsif.2009.0302.Focus
29. Sznitman J. Revisiting airflow and aerosol transport phenomena in the deep lungs with microfluidics. Chem Rev. (2022) 122:7182–204. doi: 10.1021/Acs.Chemrev.1c00621
30. Wang CC, Prather KA, Sznitman J, Jimenez JL, Lakdawala SS, Tufekci Z, et al. Airborne transmission of respiratory viruses. Science. (2021) 373:2–6. doi: 10.1126/Science.Abd9149
31. Coleman KK, Tay DJ, Sen Tan K, Ong SW, Son TT, Koh MH, et al. Viral load Sars-Cov-2 in respiratory aerosols emitted by COVID-19 patients while breathing, talking, and singing. Clin Infect Dis. (2021) 74(10):1726. doi: 10.1093/Cid/Ciab691
32. Cui X, Zhao Z, Zhang T, Guo W, Guo W, Zheng J, et al. A systematic review and meta-analysis of children with coronavirus disease 2019 (COVID-19). J Med Virol. (2021) 93:1057–69. doi: 10.1002/Jmv.26398
33. Bullard J, Funk D, Dust K, Garnett L, Tran K, Bello A, et al. Infectivity of severe acute respiratory syndrome coronavirus 2 in children compared with adults. Can Med Assoc J. (2021) 193:E601–6. doi: 10.1503/Cmaj.210263
34. Yonker LM, Neilan AM, Bartsch Y, Patel AB, Regan J, Arya P, et al. Pediatric severe acute respiratory syndrome coronavirus 2 (sars-cov-2): clinical presentation, infectivity, and immune responses. J Pediatr. (2020) 227:45–52.E5. doi: 10.1016/J.Jpeds.2020.08.037
35. Biggs HM, Killerby ME, Haynes AK, Dahl RM, Gerber SI, Watson JT. Human coronavirus circulation in the USA, 2014‒2017. Open Forum Infect Dis. (2017) 4:S311–2. doi: 10.1093/Ofid/Ofx163.727
36. Hou YJ, Okuda K, Edwards CE, Martinez DR, Asakura T, Dinnon KH, et al. Sars-Cov-2 reverse genetics reveals A variable infection gradient in the respiratory tract. Cell. (2020) 182:429–446.E14. doi: 10.1016/J.Cell.2020.05.042
37. Dattner I, Goldberg Y, Katriel G, Yaari R, Gal N, Miron Y, et al. The role of children in the spread of COVID-19: using household data from bnei brak, Israel, to estimate the relative susceptibility and infectivity of children. PloS Comput Biol. (2021) 17:E1008559. doi: 10.1371/Journal.Pcbi.1008559
38. Bunyavanich S, Do A, Vicencio A. Nasal gene expression of angiotensin-converting enzyme 2 in children and adults. J Am Med Assoc. (2020) 323:2427–9. doi: 10.1001/Jama.2020.8707
39. Gutmann D, Scheuch G, Lehmkühler T, Herrlich L-S, Landeis A, Hutter M, et al. Aerosol measurement identifies Sars-Cov 2 pcr positive adults compared with healthy controls. Environ Res. (2023) 216:114417. doi: 10.1016/J.Envres.2022.114417
40. Palas Gmbh. Resp-Aer-Meter - Produktlinien - Palas (2022). Available at: https://www.Palas.De/Product/Respaermeter (Accessed December 18, 2022).
41. Moschovis PP, Yonker LM, Shah J, Singh D, Demokritou P, Kinane TB. Aerosol transmission of Sars-Cov-2 by children and adults during the COVID-19 pandemic. Pediatr Pulmonol. (2021) 56:1389–94. doi: 10.1002/Ppul.25330
42. Pavilonis B, Ierardi AM, Levine L, Mirer F, Kelvin EA. Estimating aerosol transmission risk of Sars-Cov-2 in New York city public schools during reopening. Environ Res. (2021) 195:110805. doi: 10.1016/J.Envres.2021.110805
43. Viner RM, Mytton OT, Bonell C, Melendez-Torres GJ, Ward J, Hudson L, et al. Susceptibility to Sars-Cov-2 infection among children and adolescents compared with adults: a systematic review and meta-analysis. JAMA Pediatr. (2021) 175:143–56. doi: 10.1001/Jamapediatrics.2020.4573
44. Rostad CA, Kamidani S, Anderson EJ. Implications of Sars-Cov-2 viral load in children: getting back to school and normal. JAMA Pediatr. (2021) 175:E212022. doi: 10.1001/Jamapediatrics.2021.2022
45. Riediker M, Morawska L. Low exhaled breath droplet formation may explain why children are poor Sars-Cov-2 transmitters. Aerosol Air Qual Res. (2020) 20:1513–5. doi: 10.4209/Aaqr.2020.06.0304
46. Herring MJ, Putney LF, Wyatt G, Finkbeiner WE, Hyde DM. Growth of alveoli during postnatal development in humans based on stereological estimation. Am J Physiol Lung Cell Mol Physiol. (2014) 307:L338–44. doi: 10.1152/Ajplung.00094.2014
47. Edwards DA, Ausiello D, Salzman J, Devlin T, Langer R, Beddingfield BJ, et al. Exhaled aerosol increases with COVID-19 infection, age, and obesity. Proc Natl Acad Sci U S A. (2021) 118:2–3. doi: 10.1073/Pnas.2021830118
48. Gutmann D, Donath H, Herrlich L, Lehmkühler T, Landeis A, Ume ER, et al. Exhaled aerosols in Sars-Cov-2 polymerase chain reaction-positive children and age-matched-negative controls. Front Pediatr. (2022) 10:941785. doi: 10.3389/Fped.2022.941785
49. Veneti L, Bøås H, Bråthen Kristoffersen A, Stålcrantz J, Bragstad K, Hungnes O, et al. Reduced risk of hospitalisation among reported COVID-19 cases infected with the sars-cov-2 omicron ba.1 variant compared with the delta variant, Norway, December 2021 to January 2022. Euro Surveill. (2022) 27:2–4. doi: 10.2807/1560-7917.Es.2022.27.4.2200077
50. Menni C, Valdes AM, Polidori L, Antonelli M, Penamakuri S, Nogal A, et al. Symptom prevalence, duration, and risk of hospital admission in individuals infected with sars-cov-2 during periods of omicron and delta variant dominance: a prospective observational study from the zoe COVID study. Lancet. (2022) 399:1618–24. doi: 10.1016/S0140-6736(22)00327-0
51. Nyberg T, Ferguson NM, Nash SG, Webster HH, Flaxman S, Andrews N, et al. Comparative analysis of the risks of hospitalisation and death associated with sars-cov-2 omicron (B.1.1.529) and delta (B.1.617.2) variants in England: a cohort study. Lancet. (2022) 399:1303–12. doi: 10.1016/S0140-6736(22)00462-7
52. Lewnard JA, Hong VX, Patel MM, Kahn R, Lipsitch M, Tartof SY. Clinical outcomes associated with Sars-Cov-2 omicron (B.1.1.529) variant and ba.1/ba.1.1 or ba.2 subvariant infection in southern California. Nat Med. (2022) 28:1933–43. doi: 10.1038/S41591-022-01887-Z
53. Lim S, Zhang M, Chang TL. Ace2-Independent alternative receptors for Sars-Cov-2. Viruses. (2022) 14:1–2. doi: 10.3390/V14112535
54. Hui KP, Ho JC, Cheung M-C, Ng K-C, Ching RH, Lai K-L, et al. Sars-Cov-2 omicron variant replication in human bronchus and lung ex vivo. Nature. (2022) 603:715–20. doi: 10.1038/S41586-022-04479-6
55. Trunfio M, Portesani F, Vicinanza S, Nespoli P, Traverso F, Cortese G, et al. Real-life evidence of lower lung virulence in COVID-19 inpatients infected with Sars-Cov-2 omicron variant compared to wild-type and delta Sars-Cov-2 pneumonia. Lung. (2022) 200:573–7. doi: 10.1007/S00408-022-00566-7
56. Sinnberg T, Lichtensteiger C, Ali OH, Pop OT, Jochum A-K, Risch L, et al. Pulmonary surfactant proteins are inhibited by immunoglobulin A autoantibodies in severe COVID-19. Am J Respir Crit Care Med. (2023) 207:38–49. doi: 10.1164/Rccm.202201-0011Oc
Keywords: aerosols, SARS-CoV-2 infection, COVID-19 vaccination, omicron, children
Citation: Schuchmann P, Scheuch G, Naumann R, Keute M, Lücke T, Zielen S and Brinkmann F (2023) Exhaled aerosols among PCR-confirmed SARS-CoV-2-infected children. Front. Pediatr. 11:1156366. doi: 10.3389/fped.2023.1156366
Received: 1 February 2023; Accepted: 3 April 2023;
Published: 21 April 2023.
Edited by:
Thomas S. Murray, Yale University, United StatesReviewed by:
Josue Sznitman, Technion Israel Institute of Technology, IsraelRichard Martinello, Yale University, United States
© 2023 Schuchmann, Scheuch, Naumann, Keute, Lücke, Zielen and Brinkmann. This is an open-access article distributed under the terms of the Creative Commons Attribution License (CC BY). The use, distribution or reproduction in other forums is permitted, provided the original author(s) and the copyright owner(s) are credited and that the original publication in this journal is cited, in accordance with accepted academic practice. No use, distribution or reproduction is permitted which does not comply with these terms.
*Correspondence: Pia Schuchmann pia.schuchmann@gmail.com
Specialty Section: This article was submitted to Pediatric Infectious Diseases, a section of the journal Frontiers in Pediatrics