- 1Ritchie Centre, Hudson Institute of Medical Research, Melbourne, VIC, Australia
- 2Department of Paediatrics, Monash University, Melbourne, VIC, Australia
- 3Monash Newborn, Monash Children’s Hospital, Melbourne, VIC, Australia
Preterm birth is defined as delivery at <37 weeks of gestational age (GA) and exposes 15 million infants worldwide to serious early life diseases. Lowering the age of viability to 22 weeks GA entailed provision of intensive care to a greater number of extremely premature infants. Moreover, improved survival, especially at extremes of prematurity, comes with a rising incidence of early life diseases with short- and long-term sequelae. The transition from fetal to neonatal circulation is a substantial and complex physiologic adaptation, which normally happens rapidly and in an orderly sequence. Maternal chorioamnionitis or fetal growth restriction (FGR) are two common causes of preterm birth that are associated with impaired circulatory transition. Among many cytokines contributing to the pathogenesis of chorioamnionitis-related perinatal inflammatory diseases, the potent pro-inflammatory interleukin (IL)-1 has been shown to play a central role. The effects of utero-placental insufficiency-related FGR and in-utero hypoxia may also be mediated, in part, via the inflammatory cascade. In preclinical studies, blocking such inflammation, early and effectively, holds great promise for improving the transition of circulation. In this mini-review, we outline the mechanistic pathways leading to abnormalities in transitional circulation in chorioamnionitis and FGR. In addition, we explore the therapeutic potential of targeting IL-1 and its influence on perinatal transition in the context of chorioamnionitis and FGR.
1. Introduction
Chorioamnionitis and fetal growth restriction (FGR) – also referred to as intrauterine growth restriction (IUGR) – are conditions that complicate the course of pregnancy and may predispose infants to morbidity and mortality in early life. Chorioamnionitis involves inflammation of the placenta and fetal membranes (1, 2), whereas FGR is a condition where a fetus fails to reach its genetic growth potential (3). The pathophysiology of both is multifactorial and heterogenous; however, inflammation is a common underlying factor. In a study of 20,091 births (15,710 term and 4,381 preterm), 50.6% of preterm births were linked to placental mal-perfusion, which was associated with FGR, and 27.3% were linked to inflammation/infection (4). Hence, the growing preclinical and clinical evidence for dysregulation of the pro-inflammatory cytokine interleukin (IL)-1 (5–10) in both conditions is the focus of this mini-review.
2. IL-1 and its receptors – a brief overview
IL-1 comprises two distinct proteins and master regulators of inflammation, IL-1α and IL-1β. Both require protease processing regulated by inflammasomes to activate their pro-forms (11). One such inflammasome is the nucleotide-binding oligomerization domain-like receptor (NLRP)3 inflammasome. Assembly of the NLRP3 inflammasome is key in the activation of caspase-1, which cleaves pro-IL-1β, allowing for the secretion of its biologically active form, IL-1β (12, 13). Thus, production of IL-1α and IL-1β is controlled by transcription, maturation, and release.
IL-1α and IL-1β are expressed in a wide range of tissues and immune cells and activate pro-inflammatory transcription pathways (14–16) by signaling through the same receptor complex IL-1R1:IL-1R3 (17–19).
The IL-1 receptors contain a cytoplasmic Toll-IL-1-Receptor (TIR) domain that is common to Toll-like receptors (TLRs), which are critical for innate host defense (20), including responses to the intrauterine infections driving preterm delivery (21–23). IL-1 receptors are expressed by a wide variety of cells, resulting in a diverse range of responses upon receptor activation, such as expansion of CD4+ T cells (24), increased production of neutrophil chemoattractants (25), and increased permeability of endothelial tissue (26).
Natural counterregulatory mechanisms curtailing IL-1 function comprise IL-1 receptor antagonist (IL-1Ra), which antagonizes the binding of IL-1 to IL-1R1 (27), and the decoy receptor IL-1R2, which transduces no signal upon IL-1α and IL-1β engagement (28).
Blocking IL-1 with its natural adversary IL-1Ra (drug name anakinra) has an excellent safety and efficacy record in inflammatory disease, as established by over two decades of use in adults, children, and infants (29–34). Other trialed strategies of blocking IL-1 [reviewed in (29)] include soluble IL-1 receptor (35), neutralizing IL-1β (36, 37) or IL-1R1-blocking antibodies (38).
3. IL-1 and labor onset
In rodent models, IL-1 signaling is not necessary to ensure fertility and initiate labor, as mice deficient in IL-1β (39), caspase-1 (40), or IL-1R1 were fully fertile and delivered at term (41, 42). However, an association between IL-1 and labor has been established in non-human primate models of preterm labor. Pregnant rhesus macaques given an intra-amniotic (i.a.) infusion of IL-1β developed uterine contractions that resulted in preterm labor. In comparison, only 40% of monkeys infused with TNF had uterine contractions, and infusion of IL-6, IL-8 or saline (43) did not result in preterm labor.
In vitro, IL-1 has been suggested to promote labor by increasing calcium concentrations (44) and prostaglandin production (45) in human myometrial cells. Increased prostaglandin abundance has also been observed in the amniotic fluid of women in preterm labor (correlated with IL-1) (46) and in porcine fetal membranes stimulated with IL-1β (47).
Clinical associations between increased IL-1 production and labor onset have also been observed. At late term pregnancy, cervicovaginal fluid abundance of IL-1α and IL-1β peaked 4–14 days prior to spontaneous labor onset, whilst the anti-inflammatory IL-1Ra decreased (48). IL1B expression was low in gestational tissues from women not in labor but was present in both maternal and fetal tissues during labor, regardless of GA or intrauterine infection (49). Notably, gene expression data on IL1B needs to be interpreted with caution; unless a further activation step triggers IL-1β protein production, IL1B mRNA is rapidly degraded.
Even during a healthy pregnancy, the infiltration of leukocytes such as neutrophils, monocytes and macrophages was observed in placental tissue preceding spontaneous labor (50–52). Biopsies from women undergoing cesarean section after the onset of labor revealed that IL-1β was localized to leukocytes in the myometrium, cervix, and fetal membranes (53). Moreover, IL-1β in the amniotic fluid of women at term pregnancy correlated with the degree of leukocytic infiltration in the chorionic membrane (54). In addition to IL-1, pro-inflammatory cytokines IL-6 and IL-8 were rarely found in reproductive tissues pre-labor, but readily found following labor (49, 53, 54). Notably, IL-6 and IL-8 are both induced by IL-1. This indicates that inflammatory processes, especially those driven by IL-1 and originating in infiltrating leukocytes, play a central role in pregnancy and parturition [reviewed in (55)] (Table 1).
4. Chorioamnionitis
4.1. Clinical association of IL-1 in maternal and fetal chorioamnionitis-affected tissues
In chorioamnionitis, IL-1β abundance was shown to be increased in maternal and fetal tissues (Figure 1 and Table 1), particularly in the amniotic fluid, placenta, maternal blood as well as cord blood in some instances.
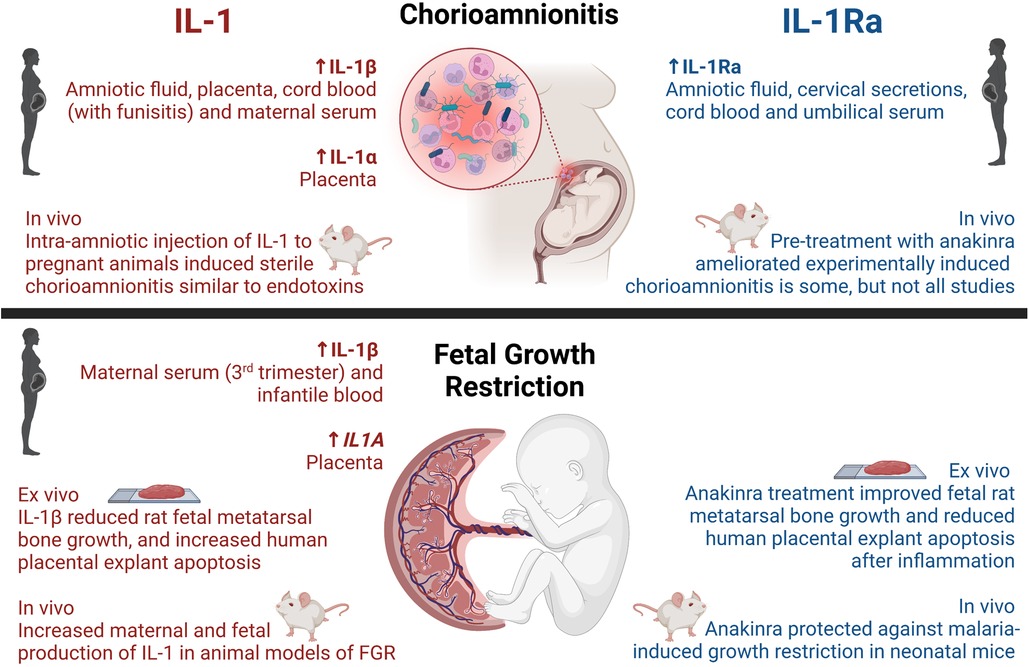
Figure 1. Reported activities of IL-1 and IL-1Ra on chorioamnionitis and fetal growth restriction in humans and disease models. Created with BioRender.com.
It is well-documented that chorioamnionitis, possibly due to the infiltration of leukocytes into the chorion and amnion (1, 64), is associated with an increased abundance of IL-1 in amniotic fluid (5, 46, 64–70), in addition to preterm labor (46, 70) and preterm pre-labor rupture of membranes (PPROM) (5). Additionally, placental tissues from chorioamnionitis-affected deliveries presented with a seventeen-fold increase in IL-1 abundance compared to healthy pregnancies, with a predisposition towards greater placental IL-1β compared to IL-1α (6). In maternal serum, IL-1β was elevated in preterm histological chorioamnionitis (71), term clinical chorioamnionitis (72) and PPROM complicated by chorioamnionitis (73). IL1B mRNA in maternal blood was elevated in women with chorioamnionitis complicated by PPROM (74). There is therefore a strong clinical association between chorioamnionitis and increased abundance of IL-1, which might be related to an increased incidence of PPROM and preterm labor.
This relationship between chorioamnionitis and IL-1 abundance in cord blood is less clear. Increased IL-1β was associated with chorioamnionitis in a select number of studies (75–77), however other studies reported no difference (78–81). This discrepancy may be explained by the concurrent presence of funisitis, an indicator of the fetal inflammatory response, marked by focal aggregation of polymorphonuclear leukocytes at the umbilical cord surface (77).
Additionally, there is now ample evidence from amniotic fluid, cervical secretions, and cord blood (82–85) that IL-1Ra, the natural IL-1 inhibitor, also positively correlates with chorioamnionitis. At first glance, elevations of anti-inflammatory mediators seem counterintuitive in this context; however, increases in the abundance of anti-inflammatory/regulatory mediators are well-recognized as part of the immune system's attempt at regaining homeostasis. Examples include increased IL-1Ra in autoimmune diseases such as rheumatoid arthritis (126) or systemic lupus erythematosus (127). Moreover, as IL-1Ra concentrations increased in maternal serum at 22–24 weeks gestation, so did the risk of preterm birth (56). Polymorphisms in the gene encoding IL-1Ra (IL1RN) have been associated with acute deciduitis (86). These findings are consistent with an involvement of IL-1 in chorioamnionitis and preterm labor.
4.2. IL-1-driven animal models of chorioamnionitis
Animal studies support the key role of IL-1 during chorioamnionitis (Figure 1 and Table 1). Intraperitoneal (i.p.) injection of lipopolysaccharide (LPS; an endotoxin of Gram-negative bacteria) to pregnant dams increased expression of the gene for IL-1β in both sheep chorion (IL1B) (58) and rat placenta (Il1b) (57), and additionally increased IL-6 and IL-1β in the maternal serum and amniotic fluid of guinea pigs (60). Moreover, pregnant rhesus macaques injected with live Group B Streptococcus (GBS) into the amniotic cavity or choriodecidual space demonstrated increased abundance of IL-1β and TNF from 13 h post-injection, and concurrently reduced the abundance of prolactin (7). Very high levels of prolactin in amniotic fluid suggest an important role in fetal development, and has been speculated to be involved in the balance of water and electrolytes, yet there is no consensus on the function of prolactin during pregnancy (128).
In addition to chorioamnionitis triggering IL-1 production, i.a. injection of IL-1 was shown to induce sterile chorioamnionitis similar to endotoxins such as LPS: Rhesus macaques were injected with either IL-1β or saline 24 or 72 h before preterm delivery via cesarean section at 80% gestation, i.e., equivalent to 32 weeks GA for a human (8). Monkeys exposed to IL-1β 24 h before cesarean exhibited infiltration of predominantly neutrophils into the decidua parietalis, and these decidual neutrophils produced increased pro-inflammatory cytokines such as TNF and IL-8, and more frequently expressed anti-inflammatory IDO (indoleamine 2, 3-dioxygenase) mRNA, than vehicle-treated controls (8). These effects were short-lasting however and by 72-hours post-dose the neutrophil infiltration and cytokine production was lower than at 24 h (8). Data on IL-1 blockade in chorioamnionitis are described in Section 7.
5. Fetal growth restriction (FGR)
5.1. The impact of FGR on transitional circulation
FGR is intimately related to issues in the cardiopulmonary transition to postnatal life, as placental insufficiency results in chronic deprivation of oxygen and nutrients, which the fetus responds to with adaptations in circulation. These adaptations can be conceptualized as “brain-sparing”, whereby blood flow is increased to the brain, as measured by middle cerebral artery Doppler studies (129, 130), whereas flow to the lower body is reduced. Due to placental vascular bed resistance, the fetal heart is subject to increased afterload, resulting in bilateral ventricular hypertrophy and reduced compliance (131, 132). Moreover, greater coronary artery blood flow, a local response seen in FGR infants, was not associated with improved cardiac function (133). As the severity of FGR increases, fetal cardiac dysfunction and myocardial cell damage increase, and perinatal morbidity worsens (134–136). Dysmorphic pulmonary vascular growth increases right to left ductal shunting, further contributing to cardiac dysfunction (137). Despite extensive research into FGR and the transitional circulation, little is known of the role of inflammation and IL-1 in this relationship.
5.2. Clinical association of IL-1 with FGR-affected maternal and infantile tissues
Knowledge on the clinical association between FGR and IL-1 in maternal and infantile samples is limited (Figure 1 and Table 1). Maternal serum abundance of IL-1β was elevated in third trimester pregnancies affected by placental insufficiency and FGR, compared to uncomplicated pregnancies (96, 97). Moreover, on day 14 of neonatal life, IL-1β was increased in peripheral blood from growth-restricted or -limited infants (98), and placentas from growth-restricted pregnancies exhibited higher IL1A mRNA when compared to healthy pregnancies (99). Polymorphisms in IL1A, namely −889C/T and +4,845G/T alleles, were associated with altered transcriptional activity and aberrant production of IL-1α. Females carrying these alleles had an elevated risk of preterm birth, and bearing of low birthweight infants, however no significant difference in the risk of FGR was confirmed (100). These findings suggest that aberrant production of IL-1α may increase the risk of preterm birth, and thus low birthweight, but were not directly associated with FGR. No associations between polymorphisms in IL1B and the risk of preterm birth, low birthweight, nor FGR were found (100). When examining amniotic fluid and cord blood, no differences in IL-1 abundance were detected between growth-restricted and appropriately grown infants (101–103).
5.3. Dysregulation of IL-1 in animal models of FGR
FGR has been linked to dysregulated IL-1 and related pathways in vivo (Figure 1 and Table 1). Many animal models of FGR have been used to investigate IL-1, including calorie-restriction (89), uteroplacental ligation (90), maternal ethanol exposure (87), bariatric surgery (10) and administration of uric acid (93). These studies demonstrated increased maternal and fetal protein production and gene expression of IL-1α and IL-1β in FGR-affected pregnancies compared to uncomplicated pregnancies.
In FGR piglets, cellular immune responses were compromised and contributed to an increased incidence of sepsis in the postnatal period (88). Piglets born FGR were associated with a lower serum immunoglobulin A and IL-1 when compared to control offspring (91, 92), potentially via increased expression of HSP70 (heat shock protein 70) (91, 138). HSP70 is a stress-response protein which can be induced by hypoxia-ischaemia (139), and thus may be increased in FGR, as found in one study examining piglet intestines at birth (140). HSP70 is also a danger signal to the innate immune system (141), and if overexpressed, can inhibit cytokine expression (142). Thus, HSP70 overproduction secondary to FGR may compromise cellular immune responses, including IL-1 expression (91).
6. Neonatal outcomes
Perinatal inflammation is inversely related to GA and birthweight, and predisposes infants to cardiopulmonary dysfunction and morbidity, as well as patent ductus arteriosus.
6.1. Cardiac dysfunction
Exposure to IL-1β has been linked to cardiac depression. IL-1β depressed rat cardiac myocyte contractile function in vitro (143, 144), and isolated hearts from IL-1 receptor associated kinase-1 (IRAK1) deficient mice were resistant to LPS-induced contractile dysfunction ex vivo (104).
Whilst a causal relationship between IL-1 in the transitional circulation and cardiac dysfunction has not yet been established, excessive inflammation in utero, to which IL-1 signaling contributes, induced cardiac dysfunction in neonatal rodents and sheep. The intracervical injection of LPS to pregnant rats on embryonic day (E)15 and subsequent serial transabdominal echocardiogram performed on fetuses in utero revealed a blunted increase in gestation related aortic blood flow velocity (BFC), and a decreased middle cerebral artery BFC compared to the vehicle controls (105). Another study administered LPS i.p. to pregnant mice on E14-15 and after 6 h investigated fetal cardiac dysfunction and inflammatory changes in the placenta. LPS exposed animals revealed increased fetal cardiac afterload, reduced fetal cardiac output, and increased placental expression of Il1a, Il6 and Tnf (106).
An association between intrauterine inflammation and long-term vulnerability to cardiac disease is beginning to be explored. Lambs exposed to i.a. injection of LPS 48 h before delivery exhibited impaired cardiomyocyte growth, increased collagen deposition and remodeling of the left ventricular myocardium, when compared to saline-treated controls. Affected lambs also demonstrated increased expression of genes related to cardiac metabolism and calcium handling, however expression of IL1B was not significantly increased (107). In non-human primates, cardiac tissue exposed to intrauterine infection identified reduced gene expression of pathways involved in cardiac morphogenesis and vasculogenesis (108). Abundance of IL-6 and IL-8 in cardiac tissue was increased in intrauterine infection, however IL-1 and TNF abundance was not significantly different between groups (108). These studies highlight the long-term implications of intrauterine inflammation on heart disease, beyond the transition to extrauterine life.
In humans with sepsis, circulating depressing factors, such as IL-1β, are speculated to induce myocardial dysfunction (145), and cardiac dysfunction after inflammation is further evidenced in human fetuses exposed to pre-labor rupture of membranes (PROM) or i.a. infections. Affected infants had increased ventricular compliance and reduced contractile function (109, 110). Cardiotocography during PROM also showed that fetuses exposed to histological chorioamnionitis exhibited a higher baseline heart rate and increased periods of low variability (111). Histological chorioamnionitis has been correlated with increased cord blood IL-1β and IL-6, increased heart rate and decreased blood pressure in the first week after birth (76).
Therefore, inflammation in the transitional circulation, as occurring in chorioamnionitis, precipitates cardiac dysfunction, and is speculated to be mediated in part by IL-1 (Table 1), however this needs to be confirmed in future studies.
6.2. Pulmonary inflammation and maturation
In addition to cardiac complications, chorioamnionitis and increased IL-1 were associated with pulmonary complications (Table 1). Early life inflammation had an initial maturing effect which increased surfactant production from type-2 alveolar epithelial cells. This increased surfactant production increased lung compliance and improved lung functionality, in experimentally-induced chorioamnionitis (112, 113) or i.a. IL-1α injection (114). Clinically, there is little research on the relationship between chorioamnionitis and surfactant production. However, it was reported that infants with respiratory distress from pregnancies complicated by chorioamnionitis presented with an altered lung surfactant lipidome compared to those without chorioamnionitis (119).
Despite the initial maturing effect of chorioamnionitis on the immature lung, the longer-term outcomes are often poor. Chronic pulmonary inflammation in mice, induced with antenatal inflammation and postnatal hyperoxia, disrupted alveolarization and vasculogenesis, manifesting a lung disease known as bronchopulmonary dysplasia (BPD). This BPD phenotype was precipitated by a rise in pulmonary inflammation, to which IL-1β was established as a key pathogenic factor (115–118, 146). In humans, infants from pregnancies complicated by chorioamnionitis have elevated IL-1β in bronchoalveolar lavage fluid (120, 121) and serum (122). Thus, somewhat paradoxically, chorioamnionitis was associated with a reduced incidence of early respiratory distress (147), but an increased incidence of BPD (148).
6.3. Patent ductus arteriosus (PDA)
PDA describes a persistent opening between the aorta and pulmonary artery after birth, affecting up to 55% of infants ≤28 weeks' GA (149) and 31% of infants <1,500 g (150). Patency and closure of the ductus arteriosus (DA) is a complex area of study [as reviewed in (151)]. During early fetal development nitric oxide (NO) is the primary mediator responsible for maintaining patency (152, 153). Closer to term, this role is filled by prostaglandin E2 (PGE2) (152, 153). Importantly IL-1β is a potent inducer of PGE2 expression (154), yet the relationship between IL-1 and PDA remains understudied (Table 1). Infection and inflammation increase the risk of PDA, and is associated with increased cyclooxygenase-1 (155) and 6-keoprostaglandin F1α (156).
After birth, multiple factors contribute to the closure of the DA, a process that is ultimately achieved by smooth muscle constriction (157). Key triggers for this muscular contraction likely include a drop in circulating PGE2 (158, 159), and increased calcium activity after acute oxygenation (as is seen in preterm infants with ventilation) (160–162).
Systematic review and meta-analysis of 23 studies, including over 17,708 infants, revealed that chorioamnionitis predisposed infants to PDA (123). Further, another clinical study found that late-onset sepsis (i.e., sepsis occurring later than ∼72 h of life) was associated with a higher rate of unsuccessful closure after treatment with concomitant diuretic and oral paracetamol treatment (124). Accordingly, inflammation is likely to play an important role in persistence of the DA. There is very little research on the relationship between IL-1 and PDA. However, echocardiography and plasma samples taken on day 3 of life in 53 infants, with a GA at birth below 28 completed weeks, revealed an association between large PDA (>1.5 mm) and increased pro-inflammatory (IL-1β, IL-8) and anti-inflammatory (IL-1Ra, IL-10) cytokines (125).
7. Blockade of IL-1 in chorioamnionitis
Considering the evidence linking chorioamnionitis with maternal and neonatal morbidity, and the negative effects of excessive inflammation and IL-1 production, anakinra has been investigated in a variety of intrauterine inflammation models (Figure 1 and Table 1).
Pregnant rats that received GBS i.p. at E19, and subsequently received three antenatal i.p. doses of anakinra, exhibited improved gestational weight, reduced IL-1β titer in placentae, maternal and fetal sera, and improved neonatal neurobehavioral outcomes when compared with rats exposed to GBS only (9).
Fetal sheep were exposed to i.a. injections of LPS with or without prior i.a. injection of recombinant human IL-1Ra. IL-1Ra pre-treatment decreased LPS-induced inflammation, as assessed by decreased lung neutrophil and monocyte influx, and decreased lung IL6 and IL1B levels, as well as decreased plasma IL-8. Blockade of IL-1 signaling in the amniotic compartment therefore inhibited fetal inflammation in response to chorioamnionitis (61).
Administration of either IL-1α or LPS i.a. to pregnant sheep resulted in placental inflammation, increased IL1B, IL6 and IL8 mRNA and IL-8 protein abundance and infiltration of inflammatory cells into the chorio-amnion (59). However, pretreatment with anakinra did not ameliorate the LPS-induced inflammation; most notably C-C Motif Chemokine Ligand 2 (CCL2)-expressing cells in the chorio-amnion were unchanged (59). In a separate study, i.a. LPS injection to pregnant sheep increased amniotic fluid CCL2 after 24 h (63). After fetal intravenous administration of rytvela, a non-competitive allosteric IL-1Ra, amniotic fluid CCL2 was significantly lower compared to controls (63). The differences in outcomes between these studies could be related to dosing or timing of LPS administration or the mechanism of IL-1 blockade.
Treatment of pregnant rhesus macaques with anakinra prior to i.a. administration of LPS prevented increased neutrophil infiltration and increased IL-6 and PGE2 abundance in the amniotic fluid, as compared to LPS-only controls (163). In a separate study using the same protocol, anakinra did not prevent the increase in pro-inflammatory T cells and decreased anti-inflammatory regulatory T cells, in the spleen of LPS-exposed fetuses (62).
IL-1R blockade shows promise as a potential therapeutic to reduce intrauterine inflammation and neonatal morbidity as seen in chorioamnionitis. However, more research is needed considering the limited literature.
8. Blockade of IL-1 in FGR
FGR is a multifactorial disease, to which inflammation contributes as described. Thus, one could speculate that blocking IL-1 could hold promise as a treatment to improve the outcome or even prevent FGR. However, blockade of IL-1 in preclinical models of FGR remains relatively understudied (Figure 1 and Table 1).
To our knowledge, there are only two ex vivo studies investigating IL-1R blockade in FGR. The first study exposed fetal metatarsal bones from Sprague Dawley rats to IL-1β and TNF, leading to reduced bone growth, which was improved by anakinra in a dose-dependent manner (94). The second study induced apoptosis via uric acid or IL-1β in human placental explants, which could be prevented by caspase-1 inhibition or anakinra treatment (93).
Interestingly, malaria infection during pregnancy led to FGR in infants, which was paralleled by placental activation of the NLRP3 inflammasome and increased IL1B expression (95). Antenatal exposure of pregnant mice to a Plasmodium parasite followed by a 5-day therapeutic treatment with anakinra commenced within 24 h after infection, restored fetal growth and reduced fetal resorption (95). Whilst this provides preliminary evidence for treating malaria-induced FGR with IL-1 blockade, further studies are needed to determine whether anakinra protects against other FGR pathologies, including placental mal-perfusion.
9. Conclusion
In normal pregnancies, IL-1 contributes to normal parturition and birth. However, in inflammation, e.g., in chorioamnionitis, IL-1 is often increased, and associated with preterm labor. Exposure of the fetus to increased IL-1 also contributes to postnatal inflammation, which can negatively affect the neonatal heart (resulting in myocardial depression) and lungs (increased risk of BPD and PDA). Therapeutic or prophylactic blockade of IL-1 signaling pathways in preclinical models of chorioamnionitis have shown to reduce intrauterine inflammation and improve fetal outcomes. The evidence on IL-1 blockade as a treatment for FGR is preliminary, but opens the field for further studies. Overall, there is good evidence to support the concept of IL-1 blockade for treating perinatal inflammation and to improve transitional circulation.
Author contributions
JCO and SPG: retrieved references and wrote the original manuscript. BMP and PJB: helped in drafting the manuscript and language modification. MFN, AS and CANP: conceived and reviewed the manuscript. All authors contributed to the article and approved the submitted version.
Funding
SPG is supported by a Co-funded Monash Graduate Scholarship. JCO and BMP are supported by Australian Government Research Training Program Scholarships. MFN is funded by the Fielding Fellowship 2017 by the Fielding Foundation. CANP is funded by an NHMRC Investigator Grant Leadership 1 (grant no. 1173584). The authors are also supported by the Victorian State Government Operational Infrastructure Scheme.
Conflict of interest
The authors declare that the research was conducted in the absence of any commercial or financial relationships that could be construed as a potential conflict of interest
Publisher's note
All claims expressed in this article are solely those of the authors and do not necessarily represent those of their affiliated organizations, or those of the publisher, the editors and the reviewers. Any product that may be evaluated in this article, or claim that may be made by its manufacturer, is not guaranteed or endorsed by the publisher.
References
1. Redline RW, Faye-Petersen O, Heller D, Qureshi F, Savell V, Vogler C, et al. Amniotic infection syndrome: nosology and reproducibility of placental reaction patterns. Pediatr Dev Pathol. (2003) 6(5):435–48. doi: 10.1007/s10024-003-7070-y
2. Tita AT, Andrews WW. Diagnosis and management of clinical chorioamnionitis. Clin Perinatol. (2010) 37(2):339–54. doi: 10.1016/j.clp.2010.02.003
3. Fenton TR, Kim JH. A systematic review and meta-analysis to revise the Fenton growth chart for preterm infants. BMC Pediatr. (2013) 13:59. doi: 10.1186/1471-2431-13-59
4. Catov JM, Scifres CM, Caritis SN, Bertolet M, Larkin J, Parks WT. Neonatal outcomes following preterm birth classified according to placental features. Am J Obstet Gynecol. (2017) 216(4):411 e1–e14. doi: 10.1016/j.ajog.2016.12.022
5. Shobokshi A, Shaarawy M. Maternal serum and amniotic fluid cytokines in patients with preterm premature rupture of membranes with and without intrauterine infection. Int J Gynaecol Obstet. (2002) 79(3):209–15. doi: 10.1016/S0020-7292(02)00238-2
6. Taniguchi T, Matsuzaki N, Kameda T, Shimoya K, Jo T, Saji F, et al. The enhanced production of placental interleukin-1 during labor and intrauterine infection. Am J Obstet Gynecol. (1991) 165(1):131–7. doi: 10.1016/0002-9378(91)90241-I
7. Bethea CL, Gravett MG, Sadowsky DW, Haluska GJ, Axthelm MK, Novy MJ. Amniotic fluid prolactin is decreased by experimental intrauterine infection or interleukin-1beta infusion but not via prostaglandins in pregnant rhesus macaques. Biol Reprod. (1998) 58(6):1385–93. doi: 10.1095/biolreprod58.6.1385
8. Presicce P, Senthamaraikannan P, Alvarez M, Rueda CM, Cappelletti M, Miller LA, et al. Neutrophil recruitment and activation in decidua with intra-amniotic IL-1beta in the preterm rhesus macaque. Biol Reprod. (2015) 92(2):56. doi: 10.1095/biolreprod.114.124420
9. Ayash TA, Vancolen SY, Segura M, Allard MJ, Sebire G. Protective effects of interleukin-1 blockade on group B streptococcus-induced chorioamnionitis and subsequent neurobehavioral impairments of the offspring. Front Endocrinol. (2022) 13:833121. doi: 10.3389/fendo.2022.833121
10. Spann RA, Lawson WJ, Bidwell GL 3rd, Zamarripa CA, Maranon RO, Bandyopadhyay S, et al. Rodent vertical sleeve gastrectomy alters maternal immune health and fetoplacental development. Clin Sci. (2018) 132(2):295–312. doi: 10.1042/CS20171416
11. Gomez-Lopez N, Romero R, Xu Y, Plazyo O, Unkel R, Than NG, et al. A role for the inflammasome in spontaneous labor at term with acute histologic chorioamnionitis. Reprod Sci. (2017) 24(6):934–53. doi: 10.1177/1933719116675058
12. Thornberry NA, Bull HG, Calaycay JR, Chapman KT, Howard AD, Kostura MJ, et al. A novel heterodimeric cysteine protease is required for interleukin-1 beta processing in monocytes. Nature. (1992) 356(6372):768–74. doi: 10.1038/356768a0
13. Latz E, Xiao TS, Stutz A. Activation and regulation of the inflammasomes. Nat Rev Immunol. (2013) 13(6):397–411. doi: 10.1038/nri3452
14. Mantovani A, Dinarello CA, Molgora M, Garlanda C. Interleukin-1 and related cytokines in the regulation of inflammation and immunity. Immunity. (2019) 50(4):778–95. doi: 10.1016/j.immuni.2019.03.012
15. Netea MG, Balkwill F, Chonchol M, Cominelli F, Donath MY, Giamarellos-Bourboulis EJ, et al. A guiding map for inflammation. Nat Immunol. (2017) 18(8):826–31. doi: 10.1038/ni.3790
16. Dinarello CA. Overview of the IL-1 family in innate inflammation and acquired immunity. Immunol Rev. (2018) 281(1):8–27. doi: 10.1111/imr.12621
17. Korherr C, Hofmeister R, Wesche H, Falk W. A critical role for interleukin-1 receptor accessory protein in interleukin-1 signaling. Eur J Immunol. (1997) 27(1):262–7. doi: 10.1002/eji.1830270139
18. Cullinan EB, Kwee L, Nunes P, Shuster DJ, Ju G, McIntyre KW, et al. IL-1 receptor accessory protein is an essential component of the IL-1 receptor. J Immunol. (1998) 161(10):5614–20. doi: 10.4049/jimmunol.161.10.5614
19. Wang D, Zhang S, Li L, Liu X, Mei K, Wang X. Structural insights into the assembly and activation of IL-1beta with its receptors. Nat Immunol. (2010) 11(10):905–11. doi: 10.1038/ni.1925
20. O’Neill LA. Signal transduction pathways activated by the IL-1 receptor/toll-like receptor superfamily. Curr Top Microbiol Immunol. (2002) 270:47–61. doi: 10.1007/978-3-642-59430-4_3
21. Moroi H, Kotani T, Miki R, Tsuda H, Mizuno M, Ito Y, et al. The expression of toll-like receptor 5 in preterm histologic chorioamnionitis. J Clin Biochem Nutr. (2018) 62(1):63–7. doi: 10.3164/jcbn.16-103
22. Kim YM, Romero R, Chaiworapongsa T, Kim GJ, Kim MR, Kuivaniemi H, et al. Toll-like receptor-2 and -4 in the chorioamniotic membranes in spontaneous labor at term and in preterm parturition that are associated with chorioamnionitis. Am J Obstet Gynecol. (2004) 191(4):1346–55. doi: 10.1016/j.ajog.2004.07.009
23. Adams KM, Lucas J, Kapur RP, Stevens AM. LPS induces translocation of TLR4 in amniotic epithelium. Placenta. (2007) 28(5-6):477–81. doi: 10.1016/j.placenta.2006.08.004
24. Ben-Sasson SZ, Hu-Li J, Quiel J, Cauchetaux S, Ratner M, Shapira I, et al. IL-1 acts directly on CD4 T cells to enhance their antigen-driven expansion and differentiation. Proc Natl Acad Sci U S A. (2009) 106(17):7119–24. doi: 10.1073/pnas.0902745106
25. Chou RC, Kim ND, Sadik CD, Seung E, Lan Y, Byrne MH, et al. Lipid-cytokine-chemokine cascade drives neutrophil recruitment in a murine model of inflammatory arthritis. Immunity. (2010) 33(2):266–78. doi: 10.1016/j.immuni.2010.07.018
26. Puhlmann M, Weinreich DM, Farma JM, Carroll NM, Turner EM, Alexander HR Jr. Interleukin-1beta induced vascular permeability is dependent on induction of endothelial tissue factor (TF) activity. J Transl Med. (2005) 3:37. doi: 10.1186/1479-5876-3-37
27. McIntyre KW, Stepan GJ, Kolinsky KD, Benjamin WR, Plocinski JM, Kaffka KL, et al. Inhibition of interleukin 1 (IL-1) binding and bioactivity in vitro and modulation of acute inflammation in vivo by IL-1 receptor antagonist and anti-IL-1 receptor monoclonal antibody. J Exp Med. (1991) 173(4):931–9. doi: 10.1084/jem.173.4.931
28. Shimizu K, Nakajima A, Sudo K, Liu Y, Mizoroki A, Ikarashi T, et al. IL-1 receptor type 2 suppresses collagen-induced arthritis by inhibiting IL-1 signal on macrophages. J Immunol. (2015) 194(7):3156–68. doi: 10.4049/jimmunol.1402155
29. Dinarello CA, Simon A, van der Meer JW. Treating inflammation by blocking interleukin-1 in a broad spectrum of diseases. Nat Rev Drug Discov. (2012) 11(8):633–52. doi: 10.1038/nrd3800
30. Gratton SB, Scalapino KJ, Fye KH. Case of anakinra as a steroid-sparing agent for gout inflammation. Arthritis Rheum. (2009) 61(9):1268–70. doi: 10.1002/art.24694
31. Committee for Medicinal Products for Human Use extension of indication variation assessment report: 30 Churchill Place, Canary Wharf, London E14 5EU, United Kingdom. European Medicines Agency (2018).
32. Kalliolias GD, Georgiou PE, Antonopoulos IA, Andonopoulos AP, Liossis SN. Anakinra treatment in patients with adult-onset still’s disease is fast, effective, safe and steroid sparing: experience from an uncontrolled trial. Ann Rheum Dis. (2007) 66(6):842–3. doi: 10.1136/ard.2006.066381
33. Mertens M, Singh JA. Anakinra for rheumatoid arthritis. Cochrane Database Syst Rev. (2009) 1:CD005121. doi: 10.1002/14651858.CD005121.pub3
34. Pascual V, Allantaz F, Arce E, Punaro M, Banchereau J. Role of interleukin-1 (IL-1) in the pathogenesis of systemic onset juvenile idiopathic arthritis and clinical response to IL-1 blockade. J Exp Med. (2005) 201(9):1479–86. doi: 10.1084/jem.20050473
35. Takebe N, Paredes J, Pino MC, Lownsbury WH, Agosti J, Krown SE. Phase I/II trial of the type I soluble recombinant human interleukin-1 receptor in HIV-1-infected patients. J Interferon Cytokine Res. (1998) 18(5):321–6. doi: 10.1089/jir.1998.18.321
36. Lachmann HJ, Kone-Paut I, Kuemmerle-Deschner JB, Leslie KS, Hachulla E, Quartier P, et al. Use of canakinumab in the cryopyrin-associated periodic syndrome. N Engl J Med. (2009) 360(23):2416–25. doi: 10.1056/NEJMoa0810787
37. Klein AL, Lin D, Cremer PC, Nasir S, Luis SA, Abbate A, et al. Efficacy and safety of rilonacept for recurrent pericarditis: results from a phase II clinical trial. Heart. (2020) 107(6):488–96. doi: 10.1136/heartjnl-2020-317928
38. Cohen SB, Proudman S, Kivitz AJ, Burch FX, Donohue JP, Burstein D, et al. A randomized, double-blind study of AMG 108 (a fully human monoclonal antibody to IL-1R1) in patients with osteoarthritis of the knee. Arthritis Res Ther. (2011) 13(4):R125. doi: 10.1186/ar3430
39. Zheng H, Fletcher D, Kozak W, Jiang M, Hofmann KJ, Conn CA, et al. Resistance to fever induction and impaired acute-phase response in interleukin-1 beta-deficient mice. Immunity. (1995) 3(1):9–19. doi: 10.1016/1074-7613(95)90154-X
40. Li P, Allen H, Banerjee S, Franklin S, Herzog L, Johnston C, et al. Mice deficient in IL-1 beta-converting enzyme are defective in production of mature IL-1 beta and resistant to endotoxic shock. Cell. (1995) 80(3):401–11. doi: 10.1016/0092-8674(95)90490-5
41. Leon LR, Conn CA, Glaccum M, Kluger MJ. IL-1 type I receptor mediates acute phase response to turpentine, but not lipopolysaccharide, in mice. Am J Physiol. (1996) 271(6 Pt 2):R1668–75. doi: 10.1152/ajpregu.1996.271.6.R1668
42. Abbondanzo SJ, Cullinan EB, McIntyre K, Labow MA, Stewart CL. Reproduction in mice lacking a functional type 1 IL-1 receptor. Endocrinology. (1996) 137(8):3598–601. doi: 10.1210/endo.137.8.8754793
43. Sadowsky DW, Adams KM, Gravett MG, Witkin SS, Novy MJ. Preterm labor is induced by intraamniotic infusions of interleukin-1beta and tumor necrosis factor-alpha but not by interleukin-6 or interleukin-8 in a nonhuman primate model. Am J Obstet Gynecol. (2006) 195(6):1578–89. doi: 10.1016/j.ajog.2006.06.072
44. Tribe RM, Moriarty P, Dalrymple A, Hassoni AA, Poston L. Interleukin-1beta induces calcium transients and enhances basal and store operated calcium entry in human myometrial smooth muscle. Biol Reprod. (2003) 68(5):1842–9. doi: 10.1095/biolreprod.102.011403
45. Pollard JK, Mitchell MD. Intrauterine infection and the effects of inflammatory mediators on prostaglandin production by myometrial cells from pregnant women. Am J Obstet Gynecol. (1996) 174(2):682–6. doi: 10.1016/S0002-9378(96)70450-7
46. Romero R, Brody DT, Oyarzun E, Mazor M, Wu YK, Hobbins JC, et al. Infection and labor. III. Interleukin-1: a signal for the onset of parturition. Am J Obstet Gynecol. (1989) 160(5 Pt 1):1117–23. doi: 10.1016/0002-9378(89)90172-5
47. Jana B, Kozlowska A, Andronowska A, Jedlinska-Krakowska M. The effect of tumor necrosis factor-alpha (TNF-alpha), interleukin (IL)-1 beta and IL-6 on chorioamnion secretion of prostaglandins (PG)F 2 alpha and E2 in pigs. Reprod Biol. (2008) 8(1):57–68. doi: 10.1016/S1642-431X(12)60004-7
48. Heng YJ, Liong S, Permezel M, Rice GE, Di Quinzio MK, Georgiou HM. The interplay of the interleukin 1 system in pregnancy and labor. Reprod Sci. (2014) 21(1):122–30. doi: 10.1177/1933719113492204
49. Dudley DJ, Collmer D, Mitchell MD, Trautman MS. Inflammatory cytokine mRNA in human gestational tissues: implications for term and preterm labor. J Soc Gynecol Investig. (1996) 3(6):328–35. doi: 10.1016/S1071-5576(96)00042-1
50. Osman I, Young A, Ledingham MA, Thomson AJ, Jordan F, Greer IA, et al. Leukocyte density and pro-inflammatory cytokine expression in human fetal membranes, decidua, cervix and myometrium before and during labour at term. Mol Hum Reprod. (2003) 9(1):41–5. doi: 10.1093/molehr/gag001
51. Thomson AJ, Telfer JF, Young A, Campbell S, Stewart CJ, Cameron IT, et al. Leukocytes infiltrate the myometrium during human parturition: further evidence that labour is an inflammatory process. Hum Reprod. (1999) 14(1):229–36. doi: 10.1093/humrep/14.1.229
52. Osman I, Young A, Jordan F, Greer IA, Norman JE. Leukocyte density and proinflammatory mediator expression in regional human fetal membranes and decidua before and during labor at term. J Soc Gynecol Investig. (2006) 13(2):97–103. doi: 10.1016/j.jsgi.2005.12.002
53. Young A, Thomson AJ, Ledingham M, Jordan F, Greer IA, Norman JE. Immunolocalization of proinflammatory cytokines in myometrium, cervix, and fetal membranes during human parturition at term. Biol Reprod. (2002) 66(2):445–9. doi: 10.1095/biolreprod66.2.445
54. Halgunset J, Johnsen H, Kjollesdal AM, Qvigstad E, Espevik T, Austgulen R. Cytokine levels in amniotic fluid and inflammatory changes in the placenta from normal deliveries at term. Eur J Obstet Gynecol Reprod Biol. (1994) 56(3):153–60. doi: 10.1016/0028-2243(94)90162-7
55. Nadeau-Vallee M, Obari D, Quiniou C, Lubell WD, Olson DM, Girard S, et al. A critical role of interleukin-1 in preterm labor. Cytokine Growth Factor Rev. (2016) 28:37–51. doi: 10.1016/j.cytogfr.2015.11.001
56. Ruiz RJ, Jallo N, Murphey C, Marti CN, Godbold E, Pickler RH. Second trimester maternal plasma levels of cytokines IL-1Ra, Il-6 and IL-10 and preterm birth. J Perinatol. (2012) 32(7):483–90. doi: 10.1038/jp.2011.193
57. Gayle DA, Beloosesky R, Desai M, Amidi F, Nunez SE, Ross MG. Maternal LPS induces cytokines in the amniotic fluid and corticotropin releasing hormone in the fetal rat brain. Am J Physiol Regul Integr Comp Physiol. (2004) 286(6):R1024–9. doi: 10.1152/ajpregu.00664.2003
58. Newnham JP, Kallapur SG, Kramer BW, Moss TJ, Nitsos I, Ikegami M, et al. Betamethasone effects on chorioamnionitis induced by intra-amniotic endotoxin in sheep. Am J Obstet Gynecol. (2003) 189(5):1458–66. doi: 10.1067/S0002-9378(03)00758-0
59. Berry CA, Nitsos I, Hillman NH, Pillow JJ, Polglase GR, Kramer BW, et al. Interleukin-1 in lipopolysaccharide induced chorioamnionitis in the fetal sheep. Reprod Sci. (2011) 18(11):1092–102. doi: 10.1177/1933719111404609
60. Dickinson MA, Harnett EL, Venditti CC, Smith GN. Transient lipopolysaccharide-induced cytokine responses in the maternal serum and amniotic fluid of the Guinea pig. Am J Obstet Gynecol. (2009) 200(5):534 e1–6. doi: 10.1016/j.ajog.2008.12.034
61. Kallapur SG, Nitsos I, Moss TJ, Polglase GR, Pillow JJ, Cheah FC, et al. IL-1 mediates pulmonary and systemic inflammatory responses to chorioamnionitis induced by lipopolysaccharide. Am J Respir Crit Care Med. (2009) 179(10):955–61. doi: 10.1164/rccm.200811-1728OC
62. Rueda CM, Presicce P, Jackson CM, Miller LA, Kallapur SG, Jobe AH, et al. Lipopolysaccharide-Induced chorioamnionitis promotes IL-1-dependent inflammatory FOXP3+ CD4+ T cells in the fetal rhesus macaque. J Immunol. (2016) 196(9):3706–15. doi: 10.4049/jimmunol.1502613
63. Takahashi Y, Saito M, Usuda H, Takahashi T, Watanabe S, Hanita T, et al. Direct administration of the non-competitive interleukin-1 receptor antagonist rytvela transiently reduced intrauterine inflammation in an extremely preterm sheep model of chorioamnionitis. PLoS ONE. (2021) 16(9):e0257847. doi: 10.1371/journal.pone.0257847
64. Stallmach T, Hebisch G, Joller-Jemelka HI, Orban P, Schwaller J, Engelmann M. Cytokine production and visualized effects in the feto-maternal unit. Quantitative and topographic data on cytokines during intrauterine disease. Lab Invest. (1995) 73(3):384–92.7564271
65. Steinborn A, Gatje R, Kramer P, Kuhnert M, Halberstadt E. Cytokines in the diagnosis of amniotic infection syndrome. Z Geburtshilfe Perinatol. (1994) 198(1):1–5.8165834
66. Stallmach T, Hebisch G, Joller H, Kolditz P, Engelmann M. Expression pattern of cytokines in the different compartments of the feto-maternal unit under various conditions. Reprod Fertil Dev. (1995) 7(6):1573–80. doi: 10.1071/RD9951573
67. Putz I, Lohbreyer M, Winkler M, Rath W. Appearance of inflammatory cytokines interleukin-1 beta and interleukin-6 in amniotic fluid during labor and in intrauterine pathogen colonization. Z Geburtshilfe Neonatol. (1998) 202(1):14–8.9577917
68. Baud O, Emilie D, Pelletier E, Lacaze-Masmonteil T, Zupan V, Fernandez H, et al. Amniotic fluid concentrations of interleukin-1beta, interleukin-6 and TNF-alpha in chorioamnionitis before 32 weeks of gestation: histological associations and neonatal outcome. Br J Obstet Gynaecol. (1999) 106(1):72–7. doi: 10.1111/j.1471-0528.1999.tb08088.x
69. Gonzalez-Bosquet E, Cerqueira MJ, Dominguez C, Gasser I, Bermejo B, Cabero L. Amniotic fluid glucose and cytokines values in the early diagnosis of amniotic infection in patients with preterm labor and intact membranes. J Matern Fetal Med. (1999) 8(4):155–8. doi: 10.1002/(SICI)1520-6661(199907/08)8:4%3C155::AID-MFM3%3E3.0.CO;2-2
70. Arntzen KJ, Kjollesdal AM, Halgunset J, Vatten L, Austgulen R. TNF, IL-1, IL-6, IL-8 and soluble TNF receptors in relation to chorioamnionitis and premature labor. J Perinat Med. (1998) 26(1):17–26. doi: 10.1515/jpme.1998.26.1.17
71. Torbe A, Czajka R, Kordek A, Rzepka R, Kwiatkowski S, Rudnicki J. Maternal serum proinflammatory cytokines in preterm labor with intact membranes: neonatal outcome and histological associations. Eur Cytokine Netw. (2007) 18(2):102–7. doi: 10.1684/ecn.2007.0092
72. Romero R, Chaemsaithong P, Docheva N, Korzeniewski SJ, Tarca AL, Bhatti G, et al. Clinical chorioamnionitis at term IV: the maternal plasma cytokine profile. J Perinat Med. (2016) 44(1):77–98. doi: 10.1515/jpm-2015-0103
73. Tian CF, Lv FH, Wang M, Gu XS. Serum beta-human chorionic gonadotropin and interleukin-1 as diagnostic biomarkers for the premature rupture of membranes and chorioamnionitis. Biomed Rep. (2014) 2(6):905–9. doi: 10.3892/br.2014.342
74. Stock O, Gordon L, Kapoor J, Walker SP, Whitehead C, Kaitu’u-Lino TJ, et al. Chorioamnionitis occurring in women with preterm rupture of the fetal membranes is associated with a dynamic increase in mRNAs coding cytokines in the maternal circulation. Reprod Sci. (2015) 22(7):852–9. doi: 10.1177/1933719114565034
75. Buscher U, Chen FC, Pitzen A, Menon R, Vogel M, Obladen M, et al. Il-1 beta, Il-6, Il-8 and G-CSF in the diagnosis of early-onset neonatal infections. J Perinat Med. (2000) 28(5):383–8. doi: 10.1515/JPM.2000.049
76. Yanowitz TD, Jordan JA, Gilmour CH, Towbin R, Bowen A, Roberts JM, et al. Hemodynamic disturbances in premature infants born after chorioamnionitis: association with cord blood cytokine concentrations. Pediatr Res. (2002) 51(3):310–6. doi: 10.1203/00006450-200203000-00008
77. D’Alquen D, Kramer BW, Seidenspinner S, Marx A, Berg D, Groneck P, et al. Activation of umbilical cord endothelial cells and fetal inflammatory response in preterm infants with chorioamnionitis and funisitis. Pediatr Res. (2005) 57(2):263–9. doi: 10.1203/01.PDR.0000148713.48218.86
78. Lencki SG, Maciulla MB, Eglinton GS. Maternal and umbilical cord serum interleukin levels in preterm labor with clinical chorioamnionitis. Am J Obstet Gynecol. (1994) 170(5 Pt 1):1345–51. doi: 10.1016/S0002-9378(13)90462-2
79. Shimoya K, Matsuzaki N, Taniguchi T, Okada T, Saji F, Murata Y. Interleukin-8 level in maternal serum as a marker for screening of histological chorioamnionitis at term. Int J Gynaecol Obstet. (1997) 57(2):153–9. doi: 10.1016/S0020-7292(97)02891-9
80. Lagodka S, Petrucci S, Moretti ML, Cabbad M, Lakhi NA. Fetal and maternal inflammatory response in the setting of maternal intrapartum fever with and without clinical and histologic chorioamnionitis. Am J Obstet Gynecol MFM. (2022) 4(2):100539. doi: 10.1016/j.ajogmf.2021.100539
81. Yuce O, Bicer OS, Kavuncuoglu S, Ozelgun B, Ongut C. Prematurity, infection, mortality, morbidity and interleukins: the reason or the result of preterm labor? Minerva Pediatr. (2014) 66(6):563–70.25336099
82. Baergen R, Benirschke K, Ulich TR. Cytokine expression in the placenta. The role of interleukin 1 and interleukin 1 receptor antagonist expression in chorioamnionitis and parturition. Arch Pathol Lab Med. (1994) 118(1):52–5.7506905
83. Romero R, Gomez R, Galasso M, Mazor M, Berry SM, Quintero RA, et al. The natural interleukin-1 receptor antagonist in the fetal, maternal, and amniotic fluid compartments: the effect of gestational age, fetal gender, and intrauterine infection. Am J Obstet Gynecol. (1994) 171(4):912–21. doi: 10.1016/S0002-9378(94)70058-3
84. Rizzo G, Capponi A, Rinaldo D, Tedeschi D, Arduini D, Romanini C. Interleukin-6 concentrations in cervical secretions identify microbial invasion of the amniotic cavity in patients with preterm labor and intact membranes. Am J Obstet Gynecol. (1996) 175(4 Pt 1):812–7. doi: 10.1016/S0002-9378(96)80004-4
85. Dollner H, Vatten L, Halgunset J, Rahimipoor S, Austgulen R. Histologic chorioamnionitis and umbilical serum levels of pro-inflammatory cytokines and cytokine inhibitors. BJOG. (2002) 109(5):534–9. doi: 10.1111/j.1471-0528.2002.01028.x
86. Polydorides AD, Kalish RB, Witkin SS, Baergen RN. Fetal polymorphisms in anti-inflammatory cytokine and beta-adrenergic receptor genes associated with placental pathological lesions. Int J Gynecol Pathol. (2008) 27(1):79–85. doi: 10.1097/pgp.0b013e31809861c5
87. Zheng D, Li Y, He L, Tang Y, Li X, Shen Q, et al. The protective effect of astaxanthin on fetal alcohol spectrum disorder in mice. Neuropharmacology. (2014) 84:13–8. doi: 10.1016/j.neuropharm.2014.04.013
88. Baek O, Ren S, Brunse A, Sangild PT, Nguyen DN. Impaired neonatal immunity and infection resistance following fetal growth restriction in preterm pigs. Front Immunol. (2020) 11:1808. doi: 10.3389/fimmu.2020.01808
89. Equils O, Singh S, Karaburun S, Lu D, Thamotharan M, Devaskar SU. Intra-uterine growth restriction downregulates the hepatic toll like receptor-4 expression and function. Clin Dev Immunol. (2005) 12(1):59–66. doi: 10.1080/17402520400008905
90. Figueroa H, Lozano M, Suazo C, Eixarch E, Illanes SE, Carreno JE, et al. Intrauterine growth restriction modifies the normal gene expression in kidney from rabbit fetuses. Early Hum Dev. (2012) 88(11):899–904. doi: 10.1016/j.earlhumdev.2012.07.010
91. Zhong X, Li W, Huang X, Zhang L, Yimamu M, Raiput N, et al. Impairment of cellular immunity is associated with overexpression of heat shock protein 70 in neonatal pigs with intrauterine growth retardation. Cell Stress Chaperones. (2012) 17(4):495–505. doi: 10.1007/s12192-012-0326-6
92. Che L, Hu L, Liu Y, Yan C, Peng X, Xu Q, et al. Dietary nucleotides supplementation improves the intestinal development and immune function of neonates with intra-uterine growth restriction in a pig model. PLoS ONE. (2016) 11(6):e0157314. doi: 10.1371/journal.pone.0157314
93. Brien ME, Duval C, Palacios J, Boufaied I, Hudon-Thibeault AA, Nadeau-Vallee M, et al. Uric acid crystals induce placental inflammation and alter trophoblast function via an IL-1-dependent pathway: implications for fetal growth restriction. J Immunol. (2017) 198(1):443–51. doi: 10.4049/jimmunol.1601179
94. Fernandez-Vojvodich P, Karimian E, Savendahl L. The biologics anakinra and etanercept prevent cytokine-induced growth retardation in cultured fetal rat metatarsal bones. Horm Res Paediatr. (2011) 76(4):278–85. doi: 10.1159/000330726
95. Reis AS, Barboza R, Murillo O, Barateiro A, Peixoto EPM, Lima FA, et al. Inflammasome activation and IL-1 signaling during placental malaria induce poor pregnancy outcomes. Sci Adv. (2020) 6(10):eaax6346. doi: 10.1126/sciadv.aax6346
96. Berbets A, Koval H, Barbe A, Albota O, Yuzko O. Melatonin decreases and cytokines increase in women with placental insufficiency. J Matern Fetal Neonatal Med. (2021) 34(3):373–8. doi: 10.1080/14767058.2019.1608432
97. Abi Nahed R, Reynaud D, Borg AJ, Traboulsi W, Wetzel A, Sapin V, et al. NLRP7 Is increased in human idiopathic fetal growth restriction and plays a critical role in trophoblast differentiation. J Mol Med. (2019) 97(3):355–67. doi: 10.1007/s00109-018-01737-x
98. McElrath TF, Allred EN, Van Marter L, Fichorova RN, Leviton A, Investigators ES. Perinatal systemic inflammatory responses of growth-restricted preterm newborns. Acta Paediatr. (2013) 102(10):e439–42. doi: 10.1111/apa.12339
99. Heinig J, Wilhelm S, Muller H, Briese V, Bittorf T, Brock J. Determination of cytokine mRNA-expression in term human placenta of patients with gestational hypertension, intrauterine growth retardation and gestational diabetes mellitus using polymerase chain reaction. Zentralbl Gynakol. (2000) 122(8):413–8. doi: 10.1055/s-2000-10606
100. Sata F, Toya S, Yamada H, Suzuki K, Saijo Y, Yamazaki A, et al. Proinflammatory cytokine polymorphisms and the risk of preterm birth and low birthweight in a Japanese population. Mol Hum Reprod. (2009) 15(2):121–30. doi: 10.1093/molehr/gan078
101. Vasco VRL, Cosmi E, Visentin S, Di Raimo T, Salmaso R, Zanardo V, et al. IL-1beta and IL-23 in amniotic fluids of ultrasound-detected aortic intima/media thickness and growth retardation. J Reprod Immunol. (2012) 93(1):64–7. doi: 10.1016/j.jri.2011.10.001
102. Lindner U, Tutdibi E, Binot S, Monz D, Hilgendorff A, Gortner L. Levels of cytokines in umbilical cord blood in small for gestational age preterm infants. Klin Padiatr. (2013) 225(2):70–4. doi: 10.1055/s-0033-1334879
103. Berbets AM, Barbe AM, Andriiets OA, Andriiets AV, Yuzko OM. Melatonin levels decrease in the umbilical cord in case of intrauterine growth restriction. J Med Life. (2020) 13(4):548–53. doi: 10.25122/jml-2020-0128
104. Thomas JA, Haudek SB, Koroglu T, Tsen MF, Bryant DD, White DJ, et al. IRAK1 Deletion disrupts cardiac toll/IL-1 signaling and protects against contractile dysfunction. Am J Physiol Heart Circ Physiol. (2003) 285(2):H597–606. doi: 10.1152/ajpheart.0655.2001
105. Abdulkadir AA, Kimimasa T, Bell MJ, Macpherson TA, Keller BB, Yanowitz TD. Placental inflammation and fetal hemodynamics in a rat model of chorioamnionitis. Pediatr Res. (2010) 68(6):513–8. doi: 10.1203/PDR.0b013e3181f851ed
106. Rounioja S, Rasanen J, Ojaniemi M, Glumoff V, Autio-Harmainen H, Hallman M. Mechanism of acute fetal cardiovascular depression after maternal inflammatory challenge in mouse. Am J Pathol. (2005) 166(6):1585–92. doi: 10.1016/S0002-9440(10)62469-8
107. Vrselja A, Pillow JJ, Bensley JG, Ellery SJ, Ahmadi-Noorbakhsh S, Moss TJ, et al. Intrauterine inflammation exacerbates maladaptive remodeling of the immature myocardium after preterm birth in lambs. Pediatr Res. (2022) 92(6):1555–65. doi: 10.1038/s41390-022-01955-7
108. Mitchell T, MacDonald JW, Srinouanpranchanh S, Bammler TK, Merillat S, Boldenow E, et al. Evidence of cardiac involvement in the fetal inflammatory response syndrome: disruption of gene networks programming cardiac development in nonhuman primates. Am J Obstet Gynecol. (2018) 218(4):438 e1–16. doi: 10.1016/j.ajog.2018.01.009
109. Romero R, Espinoza J, Goncalves LF, Gomez R, Medina L, Silva M, et al. Fetal cardiac dysfunction in preterm premature rupture of membranes. J Matern Fetal Neonatal Med. (2004) 16(3):146–57. doi: 10.1080/jmf.16.3.146.157
110. Di Naro E, Cromi A, Ghezzi F, Giocolano A, Caringella A, Loverro G. Myocardial dysfunction in fetuses exposed to intraamniotic infection: new insights from tissue Doppler and strain imaging. Am J Obstet Gynecol. (2010) 203(5):459 e1–7. doi: 10.1016/j.ajog.2010.06.033
111. Vandenbroucke L, Doyen M, Le Lous M, Beuchee A, Loget P, Carrault G, et al. Chorioamnionitis following preterm premature rupture of membranes and fetal heart rate variability. PLoS One. (2017) 12(9):e0184924. doi: 10.1371/journal.pone.0184924
112. Jobe AH, Newnham JP, Willet KE, Moss TJ, Gore Ervin M, Padbury JF, et al. Endotoxin-induced lung maturation in preterm lambs is not mediated by cortisol. Am J Respir Crit Care Med. (2000) 162(5):1656–61. doi: 10.1164/ajrccm.162.5.2003044
113. Bry K, Lappalainen U. Intra-amniotic endotoxin accelerates lung maturation in fetal rabbits. Acta Paediatr. (2001) 90(1):74–80. doi: 10.1111/j.1651-2227.2001.tb00259.x
114. Bry K, Lappalainen U, Hallman M. Intraamniotic interleukin-1 accelerates surfactant protein synthesis in fetal rabbits and improves lung stability after premature birth. J Clin Invest. (1997) 99(12):2992–9. doi: 10.1172/JCI119494
115. Garrick SP, Berger PJ, Nold MF, Nold-Petry CA. Murine double hit model for neonatal cardiopulmonary diseases: Bronchopulmonary Dysplasia (BPD) and pulmonary hypertension associated with BPD. Bio Protoc. (2022) 12(21):e4669. doi: 10.21769/BioProtoc.4669
116. Nold MF, Mangan NE, Rudloff I, Cho SX, Shariatian N, Samarasinghe TD, et al. Interleukin-1 receptor antagonist prevents murine bronchopulmonary dysplasia induced by perinatal inflammation and hyperoxia. Proc Natl Acad Sci U S A. (2013) 110(35):14384–9. doi: 10.1073/pnas.1306859110
117. Royce SG, Nold MF, Bui C, Donovan C, Lam M, Lamanna E, et al. Airway remodeling and hyperreactivity in a model of bronchopulmonary dysplasia and their modulation by IL-1 receptor antagonist. Am J Respir Cell Mol Biol. (2016) 55(6):858–68. doi: 10.1165/rcmb.2016-0031OC
118. Rudloff I, Cho SX, Bui CB, McLean C, Veldman A, Berger PJ, et al. Refining anti-inflammatory therapy strategies for bronchopulmonary dysplasia. J Cell Mol Med. (2017) 21(6):1128–38. doi: 10.1111/jcmm.13044
119. Giambelluca S, Verlato G, Simonato M, Vedovelli L, Bonadies L, Najdekr L, et al. Chorioamnionitis alters lung surfactant lipidome in newborns with respiratory distress syndrome. Pediatr Res. (2021) 90(5):1039–43. doi: 10.1038/s41390-021-01371-3
120. Watterberg KL, Demers LM, Scott SM, Murphy S. Chorioamnionitis and early lung inflammation in infants in whom bronchopulmonary dysplasia develops. Pediatrics. (1996) 97(2):210–5. doi: 10.1542/peds.97.2.210
121. Lyon AJ, McColm J, Middlemist L, Fergusson S, McIntosh N, Ross PW. Randomised trial of erythromycin on the development of chronic lung disease in preterm infants. Arch Dis Child Fetal Neonatal Ed. (1998) 78(1):F10–4. doi: 10.1136/fn.78.1.F10
122. Viscardi RM, Muhumuza CK, Rodriguez A, Fairchild KD, Sun CC, Gross GW, et al. Inflammatory markers in intrauterine and fetal blood and cerebrospinal fluid compartments are associated with adverse pulmonary and neurologic outcomes in preterm infants. Pediatr Res. (2004) 55(6):1009–17. doi: 10.1203/01.pdr.0000127015.60185.8a
123. Park HW, Choi YS, Kim KS, Kim SN. Chorioamnionitis and patent ductus arteriosus: a systematic review and meta-analysis. PLoS ONE. (2015) 10(9):e0138114. doi: 10.1371/journal.pone.0138114
124. Pharande P, Watson H, Tan K, Sehgal A. Oral paracetamol for patent ductus arteriosus rescue closure. Pediatr Cardiol. (2018) 39(1):183–90. doi: 10.1007/s00246-017-1745-z
125. Sellmer A, Henriksen TB, Palmfeldt J, Bech BH, Astono J, Bennike TB, et al. The patent ductus arteriosus in extremely preterm neonates is more than a hemodynamic challenge: new molecular insights. Biomolecules. (2022) 12(9):1179. doi: 10.3390/biom12091179
126. Mertens M, Singh JA. Anakinra for rheumatoid arthritis: a systematic review. J Rheumatol. (2009) 36(6):1118–25. doi: 10.3899/jrheum.090074
127. Rudloff I, Godsell J, Nold-Petry CA, Harris J, Hoi A, Morand EF, et al. Brief report: interleukin-38 exerts antiinflammatory functions and is associated with disease activity in systemic lupus erythematosus. Arthritis Rheumatol. (2015) 67(12):3219–25. doi: 10.1002/art.39328
128. Rana M, Jain S, Choubey P. Prolactin and its significance in the placenta. Hormones. (2022) 21(2):209–19. doi: 10.1007/s42000-022-00373-y
129. Mari G, Hanif F, Kruger M, Cosmi E, Santolaya-Forgas J, Treadwell MC. Middle cerebral artery peak systolic velocity: a new Doppler parameter in the assessment of growth-restricted fetuses. Ultrasound Obstet Gynecol. (2007) 29(3):310–6. doi: 10.1002/uog.3953
130. Vollgraff Heidweiller-Schreurs CA, De Boer MA, Heymans MW, Schoonmade LJ, Bossuyt PMM, Mol BWJ, et al. Prognostic accuracy of cerebroplacental ratio and middle cerebral artery Doppler for adverse perinatal outcome: systematic review and meta-analysis. Ultrasound Obstet Gynecol. (2018) 51(3):313–22. doi: 10.1002/uog.18809
131. Perez-Cruz M, Cruz-Lemini M, Fernandez MT, Parra JA, Bartrons J, Gomez-Roig MD, et al. Fetal cardiac function in late-onset intrauterine growth restriction vs small-for-gestational age, as defined by estimated fetal weight, cerebroplacental ratio and uterine artery Doppler. Ultrasound Obstet Gynecol. (2015) 46(4):465–71. doi: 10.1002/uog.14930
132. Menendez-Castro C, Fahlbusch F, Cordasic N, Amann K, Munzel K, Plank C, et al. Early and late postnatal myocardial and vascular changes in a protein restriction rat model of intrauterine growth restriction. PLoS One. (2011) 6(5):e20369. doi: 10.1371/journal.pone.0020369
133. Sehgal A, Allison BJ, Miller SL, Polglase GR. Myocardial perfusion and function dichotomy in growth restricted preterm infants. J Dev Orig Health Dis. (2022):1–9. doi: 10.1017/S2040174422000630
134. Crispi F, Hernandez-Andrade E, Pelsers MM, Plasencia W, Benavides-Serralde JA, Eixarch E, et al. Cardiac dysfunction and cell damage across clinical stages of severity in growth-restricted fetuses. Am J Obstet Gynecol. (2008) 199(3):254 e1–8. doi: 10.1016/j.ajog.2008.06.056
135. Nassr AA, Youssef AA, Zakherah MS, Ismail AM, Brost BC. Clinical application of fetal left modified myocardial performance index in the evaluation of fetal growth restriction. J Perinat Med. (2015) 43(6):749–54. doi: 10.1515/jpm-2014-0018
136. Rizzo G, Arduini D. Fetal cardiac function in intrauterine growth retardation. Am J Obstet Gynecol. (1991) 165(4 Pt 1):876–82. doi: 10.1016/0002-9378(91)90431-P
137. Sehgal A, Bhatia R, Roberts CT. Cardiovascular response and sequelae after minimally invasive surfactant therapy in growth-restricted preterm infants. J Perinatol. (2020) 40(8):1178–84. doi: 10.1038/s41372-020-0682-5
138. Zhong X, Li W, Huang X, Wang Y, Zhang L, Zhou Y, et al. Effects of glutamine supplementation on the immune status in weaning piglets with intrauterine growth retardation. Arch Anim Nutr. (2012) 66(5):347–56. doi: 10.1080/1745039X.2012.683325
139. Ferriero DM, Soberano HQ, Simon RP, Sharp FR. Hypoxia-ischemia induces heat shock protein-like (HSP72) immunoreactivity in neonatal rat brain. Brain Res Dev Brain Res. (1990) 53(1):145–50. doi: 10.1016/0165-3806(90)90136-M
140. Zhong X, Wang T, Zhang X, Li W. Heat shock protein 70 is upregulated in the intestine of intrauterine growth retardation piglets. Cell Stress Chaperones. (2010) 15(3):335–42. doi: 10.1007/s12192-009-0148-3
141. Luo X, Zuo X, Zhang B, Song L, Wei X, Zhou Y, et al. Release of heat shock protein 70 and the effects of extracellular heat shock protein 70 on the production of IL-10 in fibroblast-like synoviocytes. Cell Stress Chaperones. (2008) 13(3):365–73. doi: 10.1007/s12192-008-0036-2
142. Dokladny K, Lobb R, Wharton W, Ma TY, Moseley PL. LPS-induced cytokine levels are repressed by elevated expression of HSP70 in rats: possible role of NF-kappaB. Cell Stress Chaperones. (2010) 15(2):153–63. doi: 10.1007/s12192-009-0129-6
143. Kumar A, Thota V, Dee L, Olson J, Uretz E, Parrillo JE. Tumor necrosis factor alpha and interleukin 1beta are responsible for in vitro myocardial cell depression induced by human septic shock serum. J Exp Med. (1996) 183(3):949–58. doi: 10.1084/jem.183.3.949
144. Hosenpud JD, Campbell SM, Mendelson DJ. Interleukin-1-induced myocardial depression in an isolated beating heart preparation. J Heart Transplant. (1989) 8(6):460–4.2614547
145. Court O, Kumar A, Parrillo JE, Kumar A. Clinical review: myocardial depression in sepsis and septic shock. Crit Care. (2002) 6(6):500–8. doi: 10.1186/cc1822
146. Green EA, Garrick SP, Peterson B, Berger PJ, Galinsky R, Hunt RW, et al. The role of the interleukin-1 family in complications of prematurity. Int J Mol Sci. (2023) 24(3):2795. doi: 10.3390/ijms24032795
147. Sarno L, Della Corte L, Saccone G, Sirico A, Raimondi F, Zullo F, et al. Histological chorioamnionitis and risk of pulmonary complications in preterm births: a systematic review and meta-analysis. J Matern Fetal Neonatal Med. (2021) 34(22):3803–12. doi: 10.1080/14767058.2019.1689945
148. Villamor-Martinez E, Alvarez-Fuente M, Ghazi AMT, Degraeuwe P, Zimmermann LJI, Kramer BW, et al. Association of chorioamnionitis with bronchopulmonary dysplasia among preterm infants: a systematic review, meta-analysis, and metaregression. JAMA Netw Open. (2019) 2(11):e1914611. doi: 10.1001/jamanetworkopen.2019.14611
149. Sung SI, Chang YS, Kim J, Choi JH, Ahn SY, Park WS. Natural evolution of ductus arteriosus with noninterventional conservative management in extremely preterm infants born at 23–28 weeks of gestation. PLoS ONE. (2019) 14(2):e0212256. doi: 10.1371/journal.pone.0212256
150. The Vermont-Oxford Trials Network: very low birth weight outcomes for 1990. Investigators of the Vermont-Oxford trials network database project. Pediatrics. (1993) 91(3):540–5. doi: 10.1542/peds.91.3.540
151. Crockett SL, Berger CD, Shelton EL, Reese J. Molecular and mechanical factors contributing to ductus arteriosus patency and closure. Congenit Heart Dis. (2019) 14(1):15–20. doi: 10.1111/chd.12714
152. Momma K, Toyono M. The role of nitric oxide in dilating the fetal ductus arteriosus in rats. Pediatr Res. (1999) 46(3):311–5. doi: 10.1203/00006450-199909000-00010
153. Reese J, O’Mara PW, Poole SD, Brown N, Tolentino C, Eckman DM, et al. Regulation of the fetal mouse ductus arteriosus is dependent on interaction of nitric oxide and COX enzymes in the ductal wall. Prostaglandins Other Lipid Mediat. (2009) 88(3-4):89–96. doi: 10.1016/j.prostaglandins.2008.11.001
154. Dinarello CA. The IL-1 family and inflammatory diseases. Clin Exp Rheumatol. (2002) 20(5 Suppl 27):S1–S13.14989423
155. Kim ES, Kim EK, Choi CW, Kim HS, Kim BI, Choi JH, et al. Intrauterine inflammation as a risk factor for persistent ductus arteriosus patency after cyclooxygenase inhibition in extremely low birth weight infants. J Pediatr. (2010) 157(5):745–50 e1. doi: 10.1016/j.jpeds.2010.05.020
156. Gonzalez A, Sosenko IR, Chandar J, Hummler H, Claure N, Bancalari E. Influence of infection on patent ductus arteriosus and chronic lung disease in premature infants weighing 1000 grams or less. J Pediatr. (1996) 128(4):470–8. doi: 10.1016/S0022-3476(96)70356-6
157. Silver MM, Freedom RM, Silver MD, Olley PM. The morphology of the human newborn ductus arteriosus: a reappraisal of its structure and closure with special reference to prostaglandin E1 therapy. Hum Pathol. (1981) 12(12):1123–36. doi: 10.1016/S0046-8177(81)80333-4
158. Nguyen M, Camenisch T, Snouwaert JN, Hicks E, Coffman TM, Anderson PA, et al. The prostaglandin receptor EP4 triggers remodelling of the cardiovascular system at birth. Nature. (1997) 390(6655):78–81. doi: 10.1038/36342
159. Clyman RI, Mauray F, Roman C, Rudolph AM, Heymann MA. Circulating prostaglandin E2 concentrations and patent ductus arteriosus in fetal and neonatal lambs. J Pediatr. (1980) 97(3):455–61. doi: 10.1016/S0022-3476(80)80205-8
160. Baragatti B, Ciofini E, Scebba F, Angeloni D, Sodini D, Luin S, et al. Cytochrome P-450 3A13 and endothelin jointly mediate ductus arteriosus constriction to oxygen in mice. Am J Physiol Heart Circ Physiol. (2011) 300(3):H892–H901. doi: 10.1152/ajpheart.00907.2010
161. Keck M, Resnik E, Linden B, Anderson F, Sukovich DJ, Herron J, et al. Oxygen increases ductus arteriosus smooth muscle cytosolic calcium via release of calcium from inositol triphosphate-sensitive stores. Am J Physiol Lung Cell Mol Physiol. (2005) 288(5):L917–23. doi: 10.1152/ajplung.00403.2004
162. Fay FS. Guinea Pig ductus arteriosus. I. cellular and metabolic basis for oxygen sensitivity. Am J Physiol. (1971) 221(2):470–9. doi: 10.1152/ajplegacy.1971.221.2.470
Keywords: perinatal inflammation, transitional circulation, interleukin-1, chorioamnionitis, fetal growth restriction
Citation: Owen JC, Garrick SP, Peterson BM, Berger PJ, Nold MF, Sehgal A and Nold-Petry CA (2023) The role of interleukin-1 in perinatal inflammation and its impact on transitional circulation. Front. Pediatr. 11:1130013. doi: 10.3389/fped.2023.1130013
Received: 22 December 2022; Accepted: 13 February 2023;
Published: 13 March 2023.
Edited by:
Laura Mihaela Suciu, George Emil Palade University of Medicine, RomaniaReviewed by:
Dimitrios Angelis, University of Texas Southwestern Medical Center, United States© 2023 Owen, Garrick, Peterson, Berger, Nold, Sehgal and Nold-Petry. This is an open-access article distributed under the terms of the Creative Commons Attribution License (CC BY). The use, distribution or reproduction in other forums is permitted, provided the original author(s) and the copyright owner(s) are credited and that the original publication in this journal is cited, in accordance with accepted academic practice. No use, distribution or reproduction is permitted which does not comply with these terms.
*Correspondence: Claudia A. Nold-Petry Y2xhdWRpYS5ub2xkQG1vbmFzaC5lZHU=
†These authors have contributed equally to this work
Specialty Section: This article was submitted to Neonatology, a section of the journal Frontiers in Pediatrics