Corrigendum: Craniofacial and upper airway morphological characteristics associated with the presence and severity of obstructive sleep apnea in Chinese children
- 1Engineering Laboratory for Biomaterials and Tissue Regeneration, Ningbo Stomatology Hospital, Ningbo, Zhejiang, China & Savaid Stomatology School of Hangzhou Medical College, Hangzhou, Zhejiang, China
- 2Department of Orthodontics, School and Hospital of Stomatology, Cheeloo College of Medicine, Shandong University & Shandong Key Laboratory of Oral Tissue Regeneration & Shandong Engineering Laboratory for Dental Materials and Oral Tissue Regeneration & Shandong Provincial Clinical Research Center for Oral Diseases, Jinan, China
- 3Department of Stomatology, Beijing Tongren Hospital, Capital Medical University, Beijing, China
- 4Department of Implantology, School and Hospital of Stomatology, Cheeloo College of Medicine, Shandong University & Shandong Key Laboratory of Oral Tissue Regeneration & Shandong Engineering Laboratory for Dental Materials and Oral Tissue Regeneration & Shandong Provincial Clinical Research Center for Oral Diseases, Jinan, China
Objectives: To identify craniofacial and upper airway morphological characteristics associated with the presence and severity of obstructive sleep apnea (OSA) in children.
Methods: This study consisted of 82 OSA children and 77 controls (age 5–10 years). All subjects underwent cephalograms and were divided into a 5–7 age group and an 8–10 age group. Cephalometric variables were compared between OSA children and controls, and hierarchical regression analysis was performed to examine the relationship between cephalometric variables and OSA severity [expressed by the obstructive apnea–hypopnea index (OAHI)] in different age groups.
Results: Increased A/N ratio, narrowed posterior airway space, decreased SNA and SNB angles, and shortened ramus height were observed among OSA children in different age groups. In the 5–7 age group, the A/N ratio and a lower gonial angle explained 40.0% and 14.7% of the variance in the OAHI, respectively. In the 8–10 age group, the BMI z-score and A/N ratio explained 25.2% and 6.6% of the variance in the OAHI, followed by a lower gonial angle and the hyoid-retrognathion distance (19.1% in total).
Conclusions: Adenoid hypertrophy was a major factor associated with OSA in preschool children, whereas obesity replaced adenoid hypertrophy as the main contributor to OSA in late childhood. Several craniofacial skeletal variables such as the SNB angle, ramus height, lower gonial angle, and hyoid position are also associated with the presence and/or severity of OSA, which could be used to help recognize children at a higher risk for OSA.
Introduction
Obstructive sleep apnea (OSA) is characterized by recurrent partial or complete upper airway obstruction during sleep that interrupts normal sleep patterns and ventilation, therefore resulting in intermittent hypoxemia, hypercarbia, and/or sleep fragmentation (1). OSA in children is associated with a number of significant complications, such as neurocognitive impairments, learning deficits, behavioral problems, growth retardation, pulmonary hypertension, and cardiac dysfunction (2–5). The early identification and proper treatment of pediatric OSA are essential to prevent these deleterious complications.
Adenotonsillar hypertrophy has been generally considered the most common risk factor for OSA in otherwise normal healthy children (6). Enlarged upper airway lymphoid tissues will increase pharyngeal resistance when superimposed with other functional factors (e.g., reduced neuromuscular reflexes of the upper airway) and predispose the child to obstructed breathing during sleep (7). Adenotonsillectomy (AT), the first-line therapy recommended for most children by the American Academy of Pediatrics, has been associated with an improvement in behavior, quality of life, and polysomnography parameters (7–9). Even after the performance of a successful AT, OSA persists in a substantial proportion of children, which implies that other risk factors such as craniofacial skeletal abnormalities and childhood obesity are also critical in the development and progression of OSA (6, 10, 11).
Lateral cephalometry radiography remains a suitable screening tool to evaluate the adenoidal tissue size, craniofacial skeletal characteristics, and the site of airway obstruction in children with OSA (12). Cephalometric studies have shown that adenotonsillar hypertrophy and decreased pharyngeal diameters at the levels of the adenoids were highly prevalent in the OSA group (13). Certain craniofacial skeletal abnormalities have been linked to OSA in children, such as maxillary and mandibular retrognathia, maxillary transverse constriction, increased mandibular plane angle, and inferiorly positioned hyoid bone (14–16). Reduced SNB (sella-nasion-B point) angle and increased ANB (A point-nasion-B point) angle were described among children with OSA (15). The development of the cranial base influences the growth of the head and face regions. A shorter cranial base has been associated with a vertical growth patten and may play a role in OSA in children (17). However, some other contradictory studies do not support such relationships (18, 19). At present, there is insufficient evidence to the effect that craniofacial morphology is or is not associated with OSA (20). Identification of craniofacial features that may be associated with OSA in children is important since these features are routinely evaluated in dental and orthodontic practices and may help in the screening, diagnosis, and future management of OSA in children.
Therefore, the aim of this study is to identify cephalometric variables associated with the presence and severity of OSA in children.
Materials and methods
Study design and samples
A total of 82 children with OSA and 77 controls were included in this observational prospective study. This study was approved by the Medical Ethics Committee of Stomatology Hospital of Shandong University (Approval No. 20210405) and the Ethics Committee of Ningbo Stomatology Hospital (Approval No. 330202022-202100001). Informed consent was obtained from the parents of all participants.
Children with OSA were transferred from the Ningbo ENT hospital. Patients were eligible for inclusion if they were 5–10 years of age, diagnosed with OSA on the basis of polysomnography, and if they underwent standardized cephalograms. Patients were excluded if they had craniofacial or growth abnormalities and a history of orthodontic treatment, tonsillectomy, or adenoidectomy. Control subjects were consecutively recruited from first-visit patients in Stomatology Hospital of Shandong University and Ningbo Stomatology Hospital. The exclusion criteria of the control subjects were the presence of snoring and congenital disease. Children were included as control subjects if they were 5–10 years of age, if they underwent standardized cephalograms, and if they were at low risk on the Pediatric Sleep Questionnaire (PSQ). The included samples were divided into two groups according to their age: 5–7 age group (28 OSA children and 30 controls) and 8–10 age group (54 OSA children and 47 controls).
Pediatric Sleep Questionnaire
All 82 OSA children included in this study underwent an overnight PSG recording (SOMNOscreenTM Plus PSG, Randersacker, Germany) at the Ningbo ENT hospital. PSG allows a recording of thoracic and abdominal movements, airflow by nasal cannula, pulse oximetry, electroencephalogram, body position, electrooculograms, leg and chin electromyograms, and an electrocardiogram. The respiratory scoring rules were based on the American Academy of Sleep Medicine manual (21). Subjects with an obstructive apnea–hypopnea index (OAHI) score ≥1 time/h were defined as having OSA, and the severity of OSA was also indexed by using the OAHI score (22).
Questionnaire
To evaluate the risk of OSA, each parent of the control subjects was asked to complete a translated and validated PSQ. The PSQ was specifically developed to calculate the risk of OSA in children with high reliability and good validity (23). This questionnaire consists of four sections with a total of 22 questions focused on three symptoms: snoring, excessive daytime sleepiness, and inattentive/hyperactive behavior (23). Subjects with less than eight positive answers were considered at low risk or no risk for OSA and were regarded as controls in this study.
Cephalogram analysis
All included participants underwent cephalometric radiography with the same digital x-ray unit (ORTHOPHOS XG 3D ready Ceph, Sirona Dental Systems GmbH, Bensheim, Germany). They were in an upright position with their teeth in centric occlusion and their head in natural position. At the time of image analysis, the operator was blinded to the clinical information of the subjects. Cephalometric variables are defined in Table 1 and shown in Figure 1. Cephalometric measurements were repeated on 20 randomly chosen images by an investigator (QX) with a 2-week time interval and were also repeated by another investigator (XW).
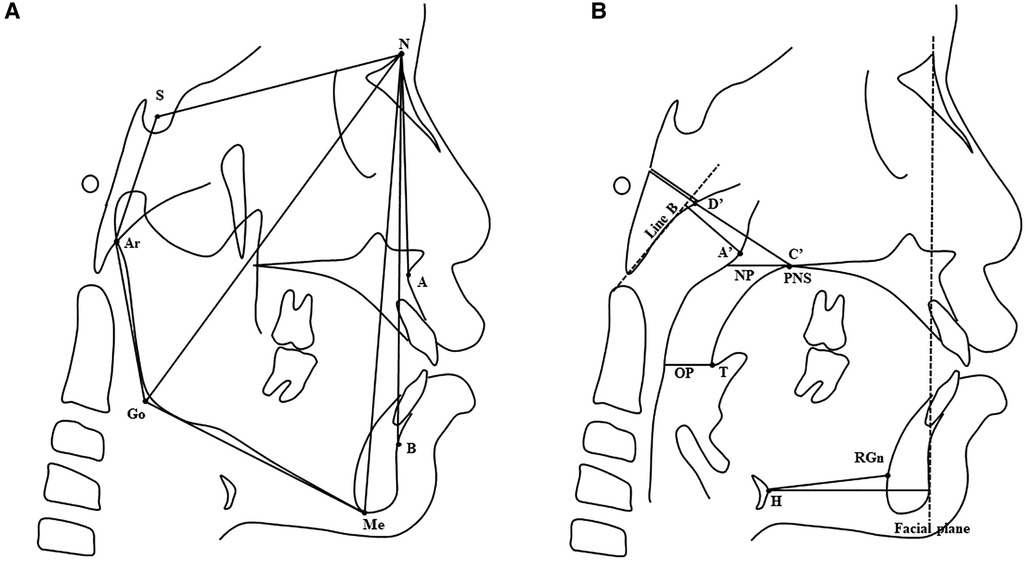
Figure 1. (A) Craniofacial skeletal variables. Landmarks: S, sella; N, nasion; A, A point; B, B point; Ar, articulare; Go, gonion; Me, menton. (B) Upper airway, adenoid, and hyoid bone variables. Landmarks: PNS, posterior nasal spine; T, tip of soft palate; A', A' point (maximal convexity along the inferior margin of adenoid shadow); C’, C’ point (posterior nasal spine); D', D' point (anteroinferior edge of phenobasioccipital synchondrosis); H, hyoid; RGn, retrognathion. Reference line: Line B, the line drawn along the straight part of the anterior margin of the basiocciput; Facial plane, the line formed by the nasion and pogonion. Variables: NP, nasopharyngeal airway space; OP, oropharyngeal airway space. See Table 1 for a detailed description of cephalometric variables.
Statistical analysis
All data were statistically analyzed using SPSS software (version 26.0, Chicago, III). The measurement error was determined by using Dahlberg's formula, which ranged between 0.581 and 1.441 mm for linear measurements and between 0.601° and 0.778° for angular measurements. An intraclass correlation coefficient (ICC) was used for determining the interobserver reliability and intraobserver reliability of the measurements. The ICC ranged from 0.891 to 0.979, showing repeated agreement with regard to all measurements.
Whether the data are normally distributed was examined by using the Shapiro–Wilk test. The independent t-test (for normally distributed variables), non-parametric Mann–Whitney test (for non-normally distributed variables), and χ2 test (for categorical variables) were used to compare the differences in the demographic characteristics between OSA children and the controls in different age groups. Demographic characteristics that were significantly different between OSA children and the controls were used as covariate(s) in the following analysis. One-way multivariate analysis of covariance was used to compare the differences of cephalometric variables between OSA children and control subjects.
Hierarchical regression analysis was performed to examine the relationship between cephalometric variables and OSA severity (expressed by OAHI) in different age groups. In step 1, since the BMI z-score was reported as a significant correlation factor with OSA severity (24), we included it in the model as a control variable to exclude its confounding effect. Then, the A/N ratio was entered into the model in step 2 to calculate its explanatory power in OSA severity. In step 3, we added each cephalometric variable (except for A/N) individually and ran a series of models. Cephalometric variables significantly correlated with OAHI (P < 0.05) after controlling for the BMI z-score, and the A/N ratio was included in the “final” multivariate model.
Results
There was no significance in age, gender, and BMI z-score between OSA children and controls in the 5–7 age group (Table 2). In the 8–10 age group, OSA children and controls approximately matched in age and gender, while OSA children had a higher BMI z-score than controls (Table 1). Therefore, the BMI z-score was entered as a covariate in the comparison of cephalometric variables between OSA children and controls in the 8–10 age group.
In the 5–7 age group, the SNB angle, nasopharynx (NP), oropharynx (OP), and bony nasopharynx (N) of OSA children were all significantly smaller than those of control subjects, and increased adenoid size (A) and A/N ratio were found among OSA children (Table 3). In the 8–10 age group, the SNA angle, SNB angle, and OP were smaller, and the A/N ratio was larger among OSA children compared with controls. In addition, the ramus height (Ar-Go) of OSA children was significantly smaller than that of controls. There was no significant difference in the hyoid position (H-RGn and H-FP) between OSA children and controls in both age groups (Table 3).
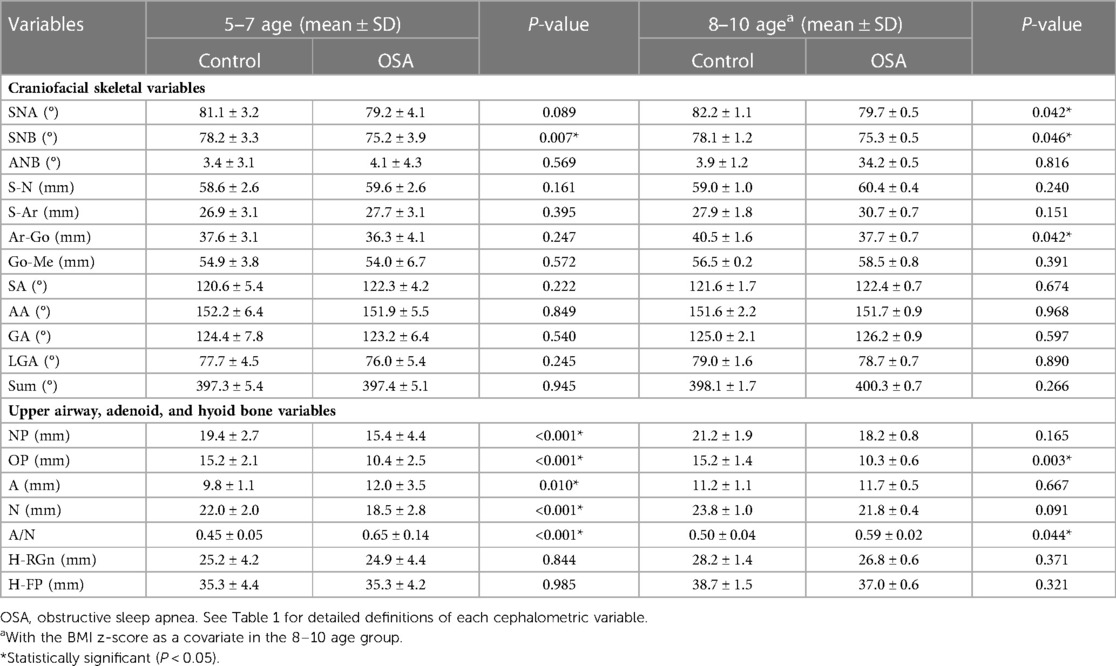
Table 3. Comparison of the cephalometric variables between OSA children and control subjects in different age groups.
The results of hierarchical regression analysis of the 5–7 age group showed that the BMI z-score was not significantly correlated with the OAHI (ΔR2 = 0.010, P = 0.618) (Table 4). Given the widely accepted influence of obesity on the OAHI, we deemed it appropriate to include the BMI z-score in the following models. The A/N ratio accounted for 40.0% of the variance in the OAHI [F(2,25) = 8.679, P = 0.001]. After controlling for the BMI z-score and A/N, it was found that the gonial angle (GA) and lower gonial angle (LGA) had a significant correlation with the OAHI (Table 4). Due to a strong correlation between GA and LGA (r = 0.775, P < 0.001), we chose LGA with more clinical significance to include it in the final multivariate model. The final model consisted of the BMI z-score, and A/N and LGA accounted for 55.6% and 50.1% (expressed by R2 and adjusted R2, respectively) of the variation in the OAHI [F(3,24) = 10.031, P < 0.001] (Table 5).
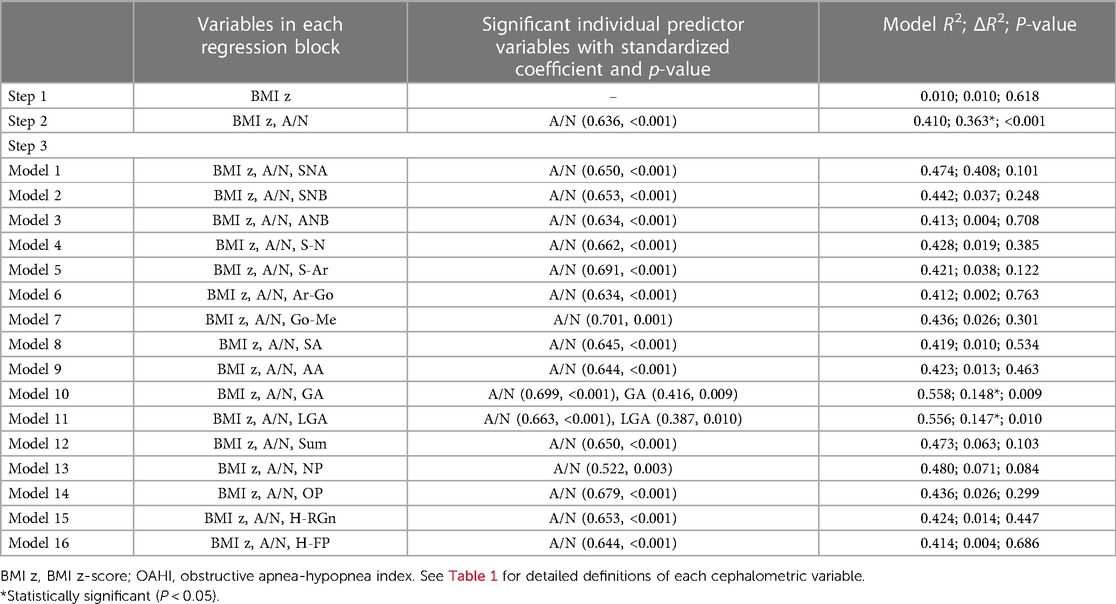
Table 4. Hierarchical regression analysis, with the BMI z-score in step 1, A/N ratio in step 2, and each of the 16 cephalometric variables individually in step 3, used to predict the OAHI in the 5–7 age group.
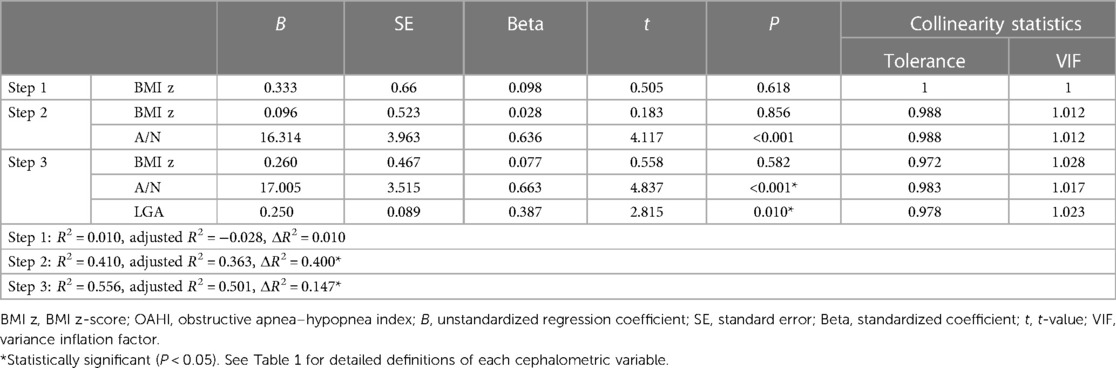
Table 5. Final hierarchical regression analysis with the BMI z-score in step 1, A/N ratio in step 2, and other significant cephalometric variables in step 3, used to predict the OAHI in the 5–7 age group.
The results of the hierarchical regression analysis of the 8–10 age group are shown in Table 6. The BMI z-score accounted for 25.2% of the variance in the OAHI [F(1,51) = 20.441, P < 0.001] and the A/N ratio 6.6% of the variance in the OAHI [F(2,50) = 10.722, P < 0.001]. After controlling for the BMI z-score and A/N, it was found that LGA, H-RGn, and H-NP had a significant correlation with the OAHI. A strong correlation was present between H-RGn and H-NP (r = 0.821, P < 0.001), since both revealed the hyoid position. By comparing the value of ΔR2, H-RGn was chosen to be included in the final multivariate model. The final model consisted of the BMI z-score, A/N, LGA, and H-RGn, and accounted for 50.9% and 46.8% (expressed by R2 and adjusted R2, respectively) of the variation in the OAHI [F(4, 48) = 10.307, P < 0.001] (Table 7).
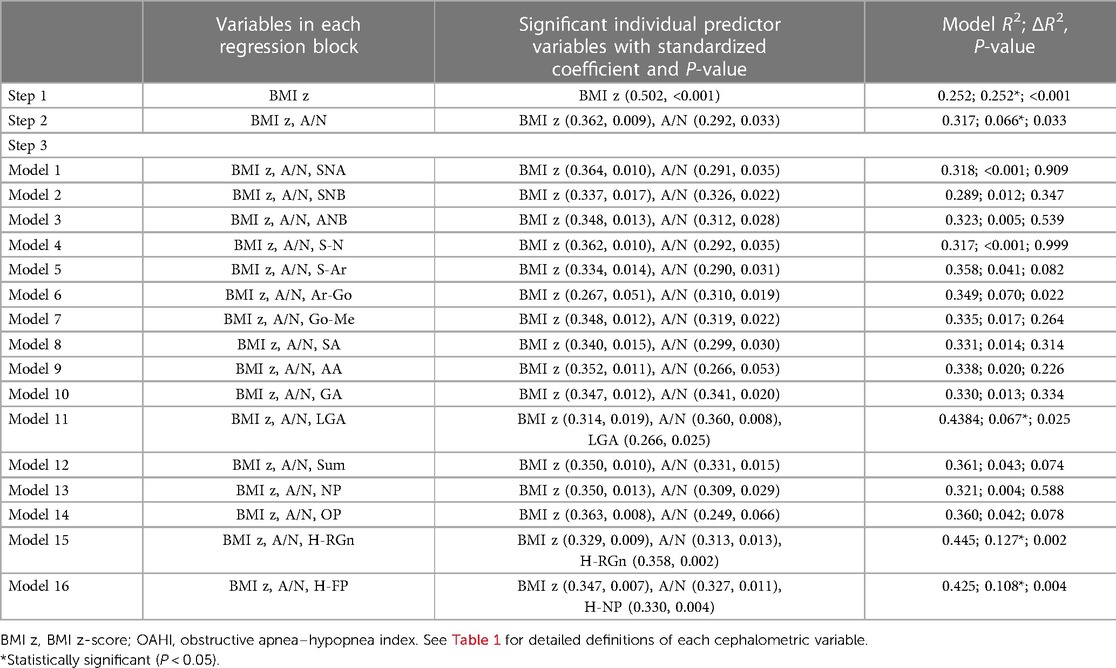
Table 6. Hierarchical regression analysis, with the BMI z-score in step 1, A/N ratio in step 2, and each of the 16 cephalometric variables individually in step 3, used to predict the OAHI in the 8–10 age group.
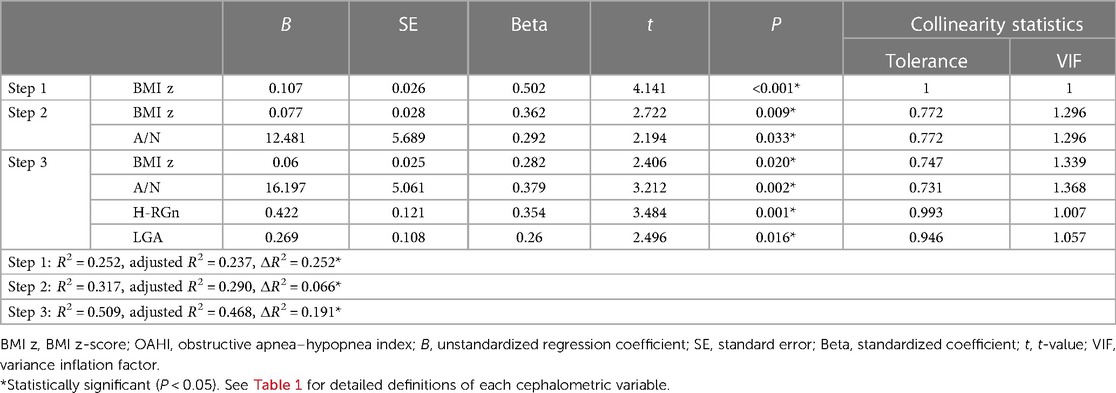
Table 7. Final hierarchical regression analysis with the BMI z-score in step 1, A/N ratio in step 2, and other significant cephalometric variables in step 3, used to predict the OAHI in the 8–10 age group.
Discussion
In this study, the cephalometric variables identified were associated with the presence and severity of OSA in children. OSA occurs in children of all ages. In our study, we focused our attention on children aged between 5 and 10 years, who are the main population group for early orthodontic treatment. The peak prevalence of OSA in children occurred in the preschool age group (at the age of 6–7), in which the increased adenotonsillar growth reached its maximum size in relation to the upper airway (25, 26). Therefore, taking into consideration the variability of OSA in developmental age (27, 28), we divided the samples according to age into two age groups (5–7 age group and 8–10 age group).
It was not surprising to find an increased A/N ratio among OSA children in the 5–7 age group. The lymphoid tissue of Waldeyer’s ring develops at a higher rate between 3 and 7 years of age and is more susceptible to recurrent respiratory infections due to immature immune function (29, 30). In addition, it was noted that children with OSA had a smaller bony nasopharynx compared with controls. According to the theory proposed by Guilleminault and colleagues, adenoids were entrapped in a relatively small space, which may be the inciting event triggering mouth breathing and the eventual adenoid growth (31, 32). Due to the mismatching of soft tissue and skeletal structure, the nasopharynx space (at the level of the posterior nasal spine) was smaller among children with OSA. In this study, the SNB angle of OSA children was significantly smaller than that of controls, which was consistent with the results of previous studies. Deng and Gao found that the SNB angle of OSA children (mean 75.82, SD 4.30) was smaller than that in the control group (mean 78.71, SD 2.61) (14). Lee et al. reported that preschool children with OSA presented a skeletal class II pattern with a retropositioned mandible (33). The mandibular retrognathism may contribute to the narrowing of the oropharynx (also observed among OSA children in this study) and subsequently to the susceptibility to OSA development.
In the 8–10 age group, although the A/N ratio of OSA children was still larger than that of controls, there were no significant differences between OSA children and controls in terms of the adenoid size (A) and the bony nasopharynx (N), due to the spontaneous remission of hypertrophied adenoid tissues and the development of the nasomaxillary complex. The difference in the nasopharynx space (at the level of the posterior nasal spine) was less distinct between OSA children and controls in the 8–10 age group, compared with that in the 5–7 age group. A reduced SNB angle and narrowing oropharynx were also observed among OSA children in the 8–10 age group. In addition, OSA children in the 8–10 age group had a significantly smaller SNA angle. It was noteworthy that OSA children in the 8–10 age group had a significantly shorten ramus height (Ar-Go). The growth of the ramus height is mainly determined by the new bone deposition on the mandibular condyle, which involves highly complicated environmental and genetic factors (34, 35). The insufficient growth of the mandibular ramus is usually associated with a reduced posterior facial height, divergent growth pattern, and skeletal class II relationship (36, 37), whereas significant condylar and ramus growth could prevent backward rotation of the mandible (38).
Due to the significant correlation with OSA severity as reported (24), the BMI z-score was included in the model in step 1 as a control variable. In the 5–7 age group, the BMI z-score was not significantly correlated with OSA severity, whereas the A/N ratio accounted for 40.0% of the variance in the OAHI, suggesting that adenoid hypertrophy was the main cause of OSA in children. Several studies have investigated the relationship between adenoid size and OSA severity. Brooks et al. evaluated 33 OSA children with a mean age of 4.5 years and found that the A/N ratio correlated with the duration of obstructive apneas more than the number of obstructive apneas (39). Jain and Sahni reported a significant correlation between adenoid size and OSA severity by evaluating 40 OSA children aged between 4 and 12 years (40). In this study, the results highlighted the direct etiologic role played by adenoid hypertrophy in the OSA severity of the 5–7 age group and suggested that adenoidectomy may be a priority treatment strategy at this stage of age.
After controlling for the BMI z-score and A/N ratio, LGA was a significant predictor of OSA severity in the 5–7 age group, accounting for 14.7% of the variance in the OAHI. Correlative data between craniofacial skeletal variables and OSA severity in pediatric OSA are limited, especially taking into account the effect of potential confounders. Some investigators have suggested that as adenoid hypertrophy blocks the upper airway, children resort to mouth breathing, resulting in an “adenoid face” such as a steep mandibular plane and a retrusive-tending chin. The supporting evidence indicated that adenoidectomy was associated with an acceleration of mandibular growth and correction of the craniofacial growth pattern (35, 41). However, evidence also shows that children who underwent adenotonsillectomy maintained their original craniofacial abnormalities (26, 42, 43). In this study, LGA was still correlated with OSA severity after controlling for the BMI z-score and A/N ratio, which suggested that the growth pattern played a significant role in the OSA severity in children. There may be other important developmental or genetic determinants that predispose children to abnormal growth patterns and then worsen OSA (26). Therefore, in addition to referral to otolaryngology, appropriate orthodontic treatment, including a correction of growth patterns to open airways, is necessary to alleviate OSA severity in children aged 5–7 years old.
Unlike in the 5–7 age group, the BMI z-score accounted for 25.2% of the variance in the OAHI, whereas the A/N ratio accounted for only 6.6% of the variance in the OAHI in the 8–10 age group. In other words, obesity replaced adenoid hypertrophy as the main factor influencing the severity of OSA in this stage of age. Lam et al. found that obese children had significantly higher AHI values than those of non-obese children and demonstrated a significant, although mild, correlation between OSA severity and the degree of obesity in OSA children ranging from 1 to 15 years old (24). In this study, we focused on OSA children aged 5–7 and 8–10 years separately and found that obesity had a significant and remarkable correlation with OSA severity in children aged 8–10 years. As adenoid hypertrophy plays a minor role in the severity of OSA, adenoidectomy should be considered with caution in this age group.
In addition to LGA, the hyoid position (H-RGn) significantly correlated with the OAHI in the 8–10 age group. The relationship between hyoid position and OSA severity has been reported in adults. Bilici et al. showed that the hyoid-menton distance between patients with severe OSA was longer than that in other OSA groups (44). Stipa et al. also described that the distance between the hyoid and the mandibular plane was a significant determinate in the model for OSA severity (45). However, there is limited literature reporting the relationship in children. In adults, the lower position of the hyoid may influence the tongue position and thus the upper airway patency, since the hyoid bone serves as an anchor for the tongue muscles (46). These alterations may also occur in OSA children aged 8–10 years.
The final model indicated that the increases in OAHI variance accounted for by the BMI z-score and cephalometric variables were equal to 50.1% in the 5–7 age group and 46.8% in the 8–10 age group, which were all statistically significant. The results revealed a significant relationship between cephalometric variables and OSA severity in children. However, the values of variance in the OAHI variables that were accounted for were not very high. It should be noted that pediatric OSA is a dynamic process resulting from a combination of upper airway structural and neuromotor abnormalities. In addition to soft tissue hypertrophy, obesity, and craniofacial disharmony, other risk factors such as an impaired neural response and abnormal central arousal mechanism were also involved in the pathology of pediatric OSA (25, 47). Considering the complex pathology of pediatric OSA, the management of OSA in children requires multidisciplinary collaboration involving the pediatric physician, otolaryngologist, and orthodontist.
Our study has several limitations. Firstly, the size of the tonsils plays an important role in the development and progression of OSA in children (48), the evaluation of which was not performed in the present study. Secondly, due to a relatively small sample size, we could not detect further cephalometric variables that may be relevant to OSA presence and severity. Also, in this study, we used OAHI ≥1 time/h as a cutoff value to determine OSA children, while some studies use different values (>1, >1.5, >2) (49, 50). The different cutoff values may influence the results. However, based on the recommendations of ICSD-3 and most researchers, we deemed it more appropriate to use the current cutoff value (51–55).
Conclusions
Adenoid hypertrophy was a major factor associated with OSA in preschool children, whereas obesity replaced adenoid hypertrophy as the main contributor to OSA in late childhood. Several craniofacial skeletal variables such as the SNB angle, ramus height, lower gonial angle, and hyoid position, were also associated with the presence and/or severity of OSA, which could be used to help recognize children at a higher risk of developing OSA.
Data availability statement
The original contributions presented in the study are included in the article/supplementary material; further inquiries can be directed to the corresponding author/s.
Ethics statement
The studies involving human participants were reviewed and approved by the Medical Ethics Committee of Stomatology Hospital of Shandong University (Approval No. 20210405) and the Ethics Committee of Ningbo Stomatology Hospital (Approval No. 330202022-202100001). Written informed consent to participate in this study was provided by the participants’ legal guardian/next of kin.
Author contributions
QX carried out data measurements, conducted statistical analysis, checked the data and wrote the article. XW carried out data measurements, wrote the article, checked the data, and reviewed the article. NL and YW collected data. JG and XX proposed and conceived the ideas for the article and were also involved in project administration. All authors contributed to the article and approved the submitted version.
Funding
This work was supported by the Key Research and Development Program in Shandong Province (grant no. 2018GSF118240) and 3315 Series Project of Ningbo City (grant no. 2020B-30-G).
Acknowledgments
The authors would like to thank all the reviewers who participated in the review during the preparation of this manuscript.
Conflict of interest
The authors declare that the research was conducted in the absence of any commercial or financial relationships that could be construed as a potential conflict of interest.
Publisher's note
All claims expressed in this article are solely those of the authors and do not necessarily represent those of their affiliated organizations, or those of the publisher, the editors and the reviewers. Any product that may be evaluated in this article, or claim that may be made by its manufacturer, is not guaranteed or endorsed by the publisher.
References
1. Marcus CL, Brooks LJ, Draper KA, Gozal D, Halbower AC, Jones J, et al. Diagnosis and management of childhood obstructive sleep apnea syndrome. Pediatrics. (2012) 130(3):576–84. doi: 10.1542/peds.2012-1671
2. Guilleminault C, Lee JH, Chan A. Pediatric obstructive sleep apnea syndrome. Arch Pediatr Adolesc Med. (2005) 159(8):775–85. doi: 10.1001/archpedi.159.8.775
3. Lamberg L. Sleep-disordered breathing may spur behavioral, learning problems in children. JAMA. (2007) 297(24):2681–3. doi: 10.1001/jama.297.24.2681
4. Fernandez-Mendoza J, He F, Calhoun SL, Vgontzas AN, Liao D, Bixler EO. Association of pediatric obstructive sleep apnea with elevated blood pressure and orthostatic hypertension in adolescence. JAMA Cardiol. (2021) 6(10):1144–51. doi: 10.1001/jamacardio.2021.2003
5. Smith DL, Gozal D, Hunter SJ, Philby MF, Kaylegian J, Kheirandish-Gozal L. Impact of sleep disordered breathing on behaviour among elementary school-aged children: a cross-sectional analysis of a large community-based sample. Eur Respir J. (2016) 48(6):1631–9. doi: 10.1183/13993003.00808-2016
6. Maeda K, Tsuiki S, Nakata S, Suzuki K, Itoh E, Inoue Y. Craniofacial contribution to residual obstructive sleep apnea after adenotonsillectomy in children: a preliminary study. J Clin Sleep Med. (2014) 10(9):973–7. doi: 10.5664/jcsm.4028
7. Bhattacharjee R, Kheirandish-Gozal L, Spruyt K, Mitchell RB, Promchiarak J, Simakajornboon N, et al. Adenotonsillectomy outcomes in treatment of obstructive sleep apnea in children: a multicenter retrospective study. Am J Respir Crit Care Med. (2010) 182(5):676–83. doi: 10.1164/rccm.200912-1930OC
8. Friedman M, Wilson M, Lin HC, Chang HW. Updated systematic review of tonsillectomy and adenoidectomy for treatment of pediatric obstructive sleep apnea/hypopnea syndrome. Otolaryngol Head Neck Surg. (2009) 140(6):800–8. doi: 10.1016/j.otohns.2009.01.043
9. Marcus CL, Moore RH, Rosen CL, Giordani B, Garetz SL, Taylor HG, et al. A randomized trial of adenotonsillectomy for childhood sleep apnea. N Engl J Med. (2013) 368(25):2366–76. doi: 10.1056/NEJMoa1215881
10. Boudewyns A, Abel F, Alexopoulos E, Evangelisti M, Kaditis A, Miano S, et al. Adenotonsillectomy to treat obstructive sleep apnea: is it enough? Pediatr Pulmonol. (2017) 52(5):699–709. doi: 10.1002/ppul.23641
11. Imanguli M, Ulualp SO. Risk factors for residual obstructive sleep apnea after adenotonsillectomy in children. Laryngoscope. (2016) 126(11):2624–9. doi: 10.1002/lary.25979
12. Slaats MA, Van Hoorenbeeck K, Van Eyck A, Vos WG, De Backer JW, Boudewyns A, et al. Upper airway imaging in pediatric obstructive sleep apnea syndrome. Sleep Med Rev. (2015) 21:59–71. doi: 10.1016/j.smrv.2014.08.001
13. Shintani T, Asakura K, Kataura A. Evaluation of the role of adenotonsillar hypertrophy and facial morphology in children with obstructive sleep apnea. ORL J Otorhinolaryngol Relat Spec. (1997) 59(5):286–91. doi: 10.1159/000276955
14. Deng J, Gao X. A case–control study of craniofacial features of children with obstructed sleep apnea. Sleep Breath. (2012) 16(4):1219–27. doi: 10.1007/s11325-011-0636-4
15. Katyal V, Pamula Y, Martin AJ, Daynes CN, Kennedy JD, Sampson WJ. Craniofacial and upper airway morphology in pediatric sleep-disordered breathing: systematic review and meta-analysis. Am J Orthod Dentofacial Orthop. (2013) 143(1):20–30.e3. doi: 10.1016/j.ajodo.2012.08.021
16. Yap B, Kontos A, Pamula Y, Martin J, Kennedy D, Sampson W, et al. Differences in dentofacial morphology in children with sleep disordered breathing are detected with routine orthodontic records. Sleep Med. (2019) 55:109–14. doi: 10.1016/j.sleep.2018.12.019
17. Abtahi S, Phuong A, Major PW, Flores-Mir C. Cranial base length in pediatric populations with sleep disordered breathing: a systematic review. Sleep Med Rev. (2018) 39:164–73. doi: 10.1016/j.smrv.2017.09.002
18. Kawashima S, Peltomaki T, Sakata H, Mori K, Happonen RP, Ronning O. Absence of facial type differences among preschool children with sleep-related breathing disorder. Acta Odontol Scand. (2003) 61(2):65–71. doi: 10.1080/00016350310001406
19. Schiffman PH, Rubin NK, Dominguez T, Mahboubi S, Udupa JK, O'Donnell AR, et al. Mandibular dimensions in children with obstructive sleep apnea syndrome. Sleep. (2004) 27(5):959–65. doi: 10.1093/sleep/27.5.959
20. Fagundes NCF, Gianoni-Capenakas S, Heo G, Flores-Mir C. Craniofacial features in children with obstructive sleep apnea: a systematic review and meta-analysis. J Clin Sleep Med. (2022) 18(7):1865–75. doi: 10.5664/jcsm.9904
21. Berry RB, Budhiraja R, Gottlieb DJ, Gozal D, Iber C, Kapur VK, et al. Rules for scoring respiratory events in sleep: update of the 2007 AASM Manual for the Scoring of Sleep and Associated Events. Deliberations of the Sleep Apnea Definitions Task Force of the American Academy of Sleep Medicine. J Clin Sleep Med. (2012) 8(5):597–619. doi: 10.5664/jcsm.2172
22. Working group of Chinese guidelines for the diagnosis and treatment of childhood OSA; Subspecialty Group of Pediatrics, Society of Otorhinolaryngology Head and Neck Surgery, Chinese Medical Association; Subspecialty Group of Respiratory Diseases, Society of Pediatrics, Chinese Medical Association; Society of Pediatric Surgery, Chinese Medical Association; Editorial Board of Chinese Journal of Otorhinolaryngology Head and Neck Surgery. Chinese Guideline for the diagnosis and treatment of childhood obstructive sleep apnea (2020). Zhonghua Er Bi Yan Hou Tou Jing Wai Ke Za Zhi. (2020) 55(8):729–47. doi: 10.3760/cma.j.cn115330-20200521-00431
23. Chervin RD, Hedger K, Dillon JE, Pituch KJ. Pediatric Sleep Questionnaire (PSQ): validity and reliability of scales for sleep-disordered breathing, snoring, sleepiness, and behavioral problems. Sleep Med. (2000) 1(1):21–32. doi: 10.1016/s1389-9457(99)00009-x
24. Lam YY, Chan EY, Ng DK, Chan CH, Cheung JM, Leung SY, et al. The correlation among obesity, apnea–hypopnea index, and tonsil size in children. Chest. (2006) 130(6):1751–6. doi: 10.1378/chest.130.6.1751
25. Alsubie HS, BaHammam AS. Obstructive sleep apnoea: children are not little adults. Paediatr Respir Rev. (2017) 21:72–9. doi: 10.1016/j.prrv.2016.02.003
26. Garg RK, Afifi AM, Garland CB, Sanchez R, Mount DL. Pediatric obstructive sleep apnea: consensus, controversy, and craniofacial considerations. Plast Reconstr Surg. (2017) 140(5):987–97. doi: 10.1097/PRS.0000000000003752
27. Lo Bue A, Salvaggio A, Insalaco G. Obstructive sleep apnea in developmental age. A narrative review. Eur J Pediatr. (2020) 179(3):357–65. doi: 10.1007/s00431-019-03557-8
28. Songu M, Adibelli ZH, Tuncyurek O, Adibelli H. Age-specific size of the upper airway structures in children during development. Ann Otol Rhinol Laryngol. (2010) 119(8):541–6. doi: 10.1177/000348941011900807
29. Su MS, Zhang HL, Cai XH, Lin Y, Liu PN, Zhang YB, et al. Obesity in children with different risk factors for obstructive sleep apnea: a community-based study. Eur J Pediatr. (2016) 175(2):211–20. doi: 10.1007/s00431-015-2613-6
30. Lu C, Sun C, Xu Y, Chen C, Li Q. Polysomnography findings in preschool children with obstructive sleep apnea are affected by growth and developmental level. Int J Pediatr Otorhinolaryngol. (2022) 162:111310. doi: 10.1016/j.ijporl.2022.111310
31. Stupak HD, Park SY. Gravitational forces, negative pressure and facial structure in the genesis of airway dysfunction during sleep: a review of the paradigm. Sleep Med. (2018) 51:125–32. doi: 10.1016/j.sleep.2018.06.016
32. Guilleminault C, Akhtar F. Pediatric sleep-disordered breathing: new evidence on its development. Sleep Med Rev. (2015) 24:46–56. doi: 10.1016/j.smrv.2014.11.008
33. Lee YH, Huang YS, Chen IC, Lin PY, Chuang LC. Craniofacial, dental arch morphology, and characteristics in preschool children with mild obstructive sleep apnea. J Dent Sci. (2020) 15(2):193–9. doi: 10.1016/j.jds.2019.09.005
34. Maki K, Inou N, Takanishi A, Miller AJ. Modeling of structure, quality, and function in the orthodontic patient. Orthod Craniofac Res. (2003) 6(Suppl 1):52–8; discussion 179–82. doi: 10.1034/j.1600-0544.2003.235.x
35. Peltomaki T. The effect of mode of breathing on craniofacial growth—revisited. Eur J Orthod. (2007) 29(5):426–9. doi: 10.1093/ejo/cjm055
36. Dong Q, Shi HY, Jia Q, Tian YY, Zhi KQ, Zhang L. Analysis of three-dimensional morphological differences in the mandible between skeletal class I and class II with CBCT fixed-point measurement method. Scanning. (2021) 2021:9996857. doi: 10.1155/2021/9996857
37. Al Maaitah EF, Alomari S, Al-Khateeb SN, Abu Alhaija ES. Cranial base measurements in different anteroposterior skeletal relationships using Bjork–Jarabak analysis. Angle Orthod. (2022) 92(5):613–8. doi: 10.2319/111321-838.1
38. Bates WR, Cevidanes LS, Larson BE, Adams D, Ruellas ACDO. Three-dimensional cone-beam computed technology evaluation of skeletal and dental changes in growing patients with class II malocclusion treated with the cervical pull face-bow headgear appliance. Am J Orthod Dentofacial Orthop. (2022) 162(4):491–501. doi: 10.1016/j.ajodo.2021.05.011
39. Brooks LJ, Stephens BM, Bacevice AM. Adenoid size is related to severity but not the number of episodes of obstructive apnea in children. J Pediatr. (1998) 132(4):682–6. doi: 10.1016/s0022-3476(98)70360-9
40. Jain A, Sahni JK. Polysomnographic studies in children undergoing adenoidectomy and/or tonsillectomy. J Laryngol Otol. (2002) 116(9):711–5. doi: 10.1258/002221502760238019
41. Zhao T, Ngan P, Hua F, Zheng J, Zhou S, Zhang M, et al. Impact of pediatric obstructive sleep apnea on the development of class II hyperdivergent patients receiving orthodontic treatment: a pilot study. Angle Orthod. (2018) 88(5):560–6. doi: 10.2319/110617-759.1
42. Pirila-Parkkinen K, Pirttiniemi P, Nieminen P, Tolonen U, Pelttari U, Lopponen H. Dental arch morphology in children with sleep-disordered breathing. Eur J Orthod. (2009) 31(2):160–7. doi: 10.1093/ejo/cjn061
43. Zettergren-Wijk L, Forsberg CM, Linder-Aronson S. Changes in dentofacial morphology after adeno-/tonsillectomy in young children with obstructive sleep apnoea—a 5-year follow-up study. Eur J Orthod. (2006) 28(4):319–26. doi: 10.1093/ejo/cji119
44. Bilici S, Yigit O, Celebi OO, Yasak AG, Yardimci AH. Relations between hyoid-related cephalometric measurements and severity of obstructive sleep apnea. J Craniofac Surg. (2018) 29(5):1276–81. doi: 10.1097/Scs.0000000000004483
45. Stipa C, Cameli M, Sorrenti G, Ippolito DR, Pelligra I, Alessandri-Bonetti G. Relationship between cephalometric parameters and the apnoea-hypopnoea index in OSA patients: a retrospective cohort study. Eur J Orthodont. (2020) 42(1):101–6. doi: 10.1093/ejo/cjz038
46. Lam B, Ooi CG, Peh WC, Lauder I, Tsang KW, Lam WK, et al. Computed tomographic evaluation of the role of craniofacial and upper airway morphology in obstructive sleep apnea in Chinese. Respir Med. (2004) 98(4):301–7. doi: 10.1016/j.rmed.2003.10.009
47. Marcus CL, Fernandes Do Prado LB, Lutz J, Katz ES, Black CA, Galster P, et al. Developmental changes in upper airway dynamics. J Appl Physiol. (2004) 97(1):98–108. doi: 10.1152/japplphysiol.00462.2003
48. Pirila-Parkkinen K, Lopponen H, Nieminen P, Tolonen U, Paakko E, Pirttiniemi P. Validity of upper airway assessment in children: a clinical, cephalometric, and MRI study. Angle Orthodontist. (2011) 81(3):433–9. doi: 10.2319/063010-362.1
49. Galeotti A, Festa P, Viarani V, Pavone M, Sitzia E, Piga S, et al. Correlation between cephalometric variables and obstructive sleep apnoea severity in children. Eur J Paediatr Dent. (2019) 20(1):43–7. doi: 10.23804/ejpd.2019.20.01.09
50. Bokov P, Mauvais FX, Madani A, Matrot B, Lecendreux M, Delanoë C, et al. Cross-sectional case-control study of the relationships between pharyngeal compliance and heart rate variability indices in childhood obstructive sleep apnoea. J Sleep Res. (2021) 30(5):e13337. doi: 10.1111/jsr.13337
51. Sateia MJ. International classification of sleep disorders-third edition: highlights and modifications. Chest. (2014) 146(5):1387–94. doi: 10.1378/chest.14-0970
52. Yuen HM, Chan KC, Chu WCW, Chan JWY, Wing YK, Li AM, et al. Craniofacial phenotyping by photogrammetry in Chinese prepubertal children with obstructive sleep apnea. Sleep. (2023) 46(3):zsac289. doi: 10.1093/sleep/zsac289
53. Xiao L, Su S, Liang J, Jiang Y, Shu Y, Ding L. Analysis of the risk factors associated with obstructive sleep apnea syndrome in Chinese children. Front Pediatr. (2022) 10:900216. doi: 10.3389/fped.2022.900216
54. Xu Z, Wu Y, Tai J, Feng G, Ge W, Zheng L, et al. Risk factors of obstructive sleep apnea syndrome in children. J Otolaryngol Head Neck Surg. (2020) 49(1):11. doi: 10.1186/s40463-020-0404-1
Keywords: orthodontic clamps, dentofacial orthopedics, upper airway, pediatric obstructive sleep apnea, cephalometry, craniofacial abnormalities
Citation: Xu Q, Wang X, Li N, Wang Y, Xu X and Guo J (2023) Craniofacial and upper airway morphological characteristics associated with the presence and severity of obstructive sleep apnea in Chinese children. Front. Pediatr. 11:1124610. doi: 10.3389/fped.2023.1124610
Received: 15 December 2022; Accepted: 8 March 2023;
Published: 31 March 2023.
Edited by:
Mohammad Khursheed Alam, Al Jouf University, Saudi ArabiaReviewed by:
Luana Maria Nosetti, University of Insubria, ItalyGustavo Moreira, Federal University of São Paulo, Brazil
© 2023 Xu, Wang, Li, Wang, Xu and Guo. This is an open-access article distributed under the terms of the Creative Commons Attribution License (CC BY). The use, distribution or reproduction in other forums is permitted, provided the original author(s) and the copyright owner(s) are credited and that the original publication in this journal is cited, in accordance with accepted academic practice. No use, distribution or reproduction is permitted which does not comply with these terms.
*Correspondence: Jing Guo Z3VvamluZ0BzZHUuZWR1LmNu
†These authors have contributed equally to this work
Specialty Section: This article was submitted to Pediatric Otolaryngology, a section of the journal Frontiers in Pediatrics