- 1Department of Pediatric Surgery, University Medical Center Mannheim, University of Heidelberg, Mannheim, Germany
- 2Department of Pediatrics, The University of Oklahoma Health Sciences Center, Oklahoma, OK, United States
Necrotizing enterocolitis (NEC) continues to be one of the most common causes of mortality and morbidity in preterm infants. Although not fully elucidated, studies suggest that prematurity, formula feeding, imbalanced vascular supply, and altered bacterial colonization play major roles in the pathogenesis of NEC. NEC is characterized by increased cytokine release and leukocyte infiltration. Recent data from preterm infants and animal models of NEC suggest that neutrophil extracellular traps (NETs) are released in intestinal tissue. The contribution of NETs in the pathogenesis and/or prevention/treatment of this disease continues to be controversial. Here, we review the available data on NETs release in NEC in human patients and in different NEC models, highlighting their potential contribution to pathology and resolution of inflammation. Here, we review the available data on NETs release in NEC in human patients and the different NEC models, highlighting their potential contribution to pathology or resolution of inflammation.
Introduction
Necrotizing enterocolitis (NEC) is one of the most devastating diseases in the neonatal intensive care unit. This inflammatory bowel disease primarily affects preterm infants, with an incidence of 7%–12% in neonates born less than 1,500 g (1). Importantly, the incidence is steadily increasing, as improvements in neonatal care lead to enhanced survival of premature infants (2–4.) NEC is associated with mortality rates of up to 30% in very low birth weight infants (5), and up to 80% in the most severe cases (fulminant NEC) (6). Moreover, survivors of NEC are at increased risk of long-term morbidities such as growth failure, short bowel syndrome, and neurodevelopmental delay, all of which increase the physical and psychological burdens for patients and their families (7, 8).
Despite decades of investigations, the pathogenesis of NEC remains inconclusive, perhaps since NEC may not be a single disease, but comprised of several entities (e.g., classic NEC, ischemic intestinal necrosis, food protein intolerance enterocolitis syndrome) (9–11) NEC pathogenesis is multifactorial with prematurity, formula feeding, and dysregulation of perfusion, as well as dysbiosis, playing major roles. In preterm neonates, developmental immaturity of the mucosal barrier and increased expression of toll-like receptor (TLR) 4 in the intestinal epithelium render the gut highly reactive to stimuli (12, 13). The net effect is exaggerated inflammatory cytokine/chemokine release, leukocyte infiltration, epithelial necrosis, altered epithelial barrier, and bacterial translocation across the lumen (14). Of particular interest, TLR4 expression can be reduced by breast milk feeding (15). This excessive TLR4 expression in response to the dysbiotic microbiome can lead to the death of intestinal epithelial cells through apoptosis and necroptosis, as well as impaired mucosal restitution, which in severe cases leads to intestinal perforation, multi-organ failure, and potentially death (16).
Notably, NEC is not solely a disease of the abdomen, rather it is a multisystemic disease that can also affect other organ systems (17–19). Systemic reviews have demonstrated that NEC is an independent risk factor for neurocognitive developmental delay and poor neurocognitive outcomes (20, 21). Moreover, studies suggest that common morbidities of the preterm infant such as bronchopulmonary dysplasia (BPD) and brain damage are affected by the development of NEC necrotizing enterocolitis, through interactions known as the “Gut-Lung-Axis” and “Gut-Brain-Axis”, respectively (18, 19). Recently, it has been reported that the excessive immune response via TLR4 and neutrophil activation are associated with increased damage to not just the intestine but also the lung and brain tissue, suggesting a potential role of neutrophils in distant organ injury in NEC (17–19). Suggesting a potential role of neutrophils in distant organ injury in NEC.
Neutrophils in necrotizing enterocolitis
Neutrophils are the most abundant immune cells and first-line responders of the innate immune system (22, 23). As polymorphonuclear cells, neutrophils are very motile, which enables them to migrate from peripheral vasculature into the tissue of recruitment (23, 24). One of the key functions in NEC pathogenesis is the activation of intestinal epithelial cell toll-like receptor 4 (TLR4) which leads to accelerated apoptosis of enterocytes and reduced rate of healing through impaired intestinal restoration and proliferation. Upregulation in TLR4 expression results in the production of pro-inflammatory cytokines and chemokines leading to the recruitment of neutrophils to the location of inflammation (25). Neutrophil infiltration has been long recognized in NEC tissues. However, the beneficial and detrimental contributions of these cells specifically in this disease remain unclear (11, 26, 27). Neutrophils seem to be critical for mucosal homeostasis as NEC is aggravated by neutrophil depletion in a murine model of NEC (28). However, excessive recruitment and activation of neutrophils could also promote injury and exacerbate disease including mutual upregulation of TLR4 and neutrophil activation (29).
Upon contact with pathogens, neutrophils can react via (1) phagocytosis, (2) production of oxidative bursts like reactive oxygen species (ROS), and (3) degranulation and/or (4) formation of neutrophil extracellular traps (NETs) (30). NETs are large extracellular networks consisting of DNA fibers and spherical proteins. The protein contents of NETs include histones, neutrophil elastase (NE), myeloperoxidase (MPO), defensin, calprotectin, cathepsin G, protease 3, and actin, lactoferrin, gelatinase, lysozyme C, and cathelicidins (24, 31–33). Neutrophils release NETs via multiple mechanisms: (1) NETosis, a programmed cell death pathway distinct from apoptosis, pyroptosis, necroptosis, or ferroptosis, (2) non-lytic discharge of parts or their entire nucleus, and (3) mitochondrial DNA release, providing an additional DNA source for NET formation (34). The main processes involved in NETosis are neutrophil activation, cytoplasmic granule dissolution, neutrophil protease activation, chromatin decondensation, and swelling, followed by plasma membrane rupture. NETs are released after histones are citrullinated by peptidyl arginine deiminase 4 (PAD4) (35). The function of those structures is to capture pathogenic microorganisms and enhance phagocytosis by macrophages thus preventing the spreading of infection (24, 36–38). NETs are normally cleared by plasma DNAse 1 followed by removal by macrophages. Inappropriate or delayed clearance of NETs or NET components specifically the associated histones and proteases contribute to pathological conditions like sepsis (39), thrombosis (40), transfusion-associated acute lung injury (41), cancer development and metastasis (42), autoimmune diseases (23, 43), and impaired wound healing (44) - mostly through induction of INF, proinflammatory cytokines, and the NLRP3 inflammasome (45). A major mechanism for the cytotoxic properties of histones in NETs is through direct binding to the plasma membrane causing calcium influx and loss of membrane barrier function (46–48). Histones also activate TLRs 2, 4, 9 leading to cytokine production, leukocyte recruitment, and tissue injury (49–51). Furthermore, extracellular histones in NETs stimulate platelet adhesion and coagulation (52), which in severe cases can lead to multi-organ failure due to micro thrombosis, decrease microvascular perfusion, and subsequent tissue damage (53). This has been demonstrated by the association of NETs with various thrombo-inflammatory diseases such as stroke, autoimmune diseases, sepsis, lung injury (i.e., COVID-19), diabetes, and ischemia-reperfusion injury of the intestine and testicles (54–60). To further illustrate their double-edged nature, NETs released in the gut have been shown to reduce the translocation of bacteria and support the healing of the intestinal mucosa. On the other hand, excessive NETs formation can damage the barrier function of the intestinal mucosa and thus play a key role in the development of a variety of intestinal diseases (23, 42) (Figure 1).
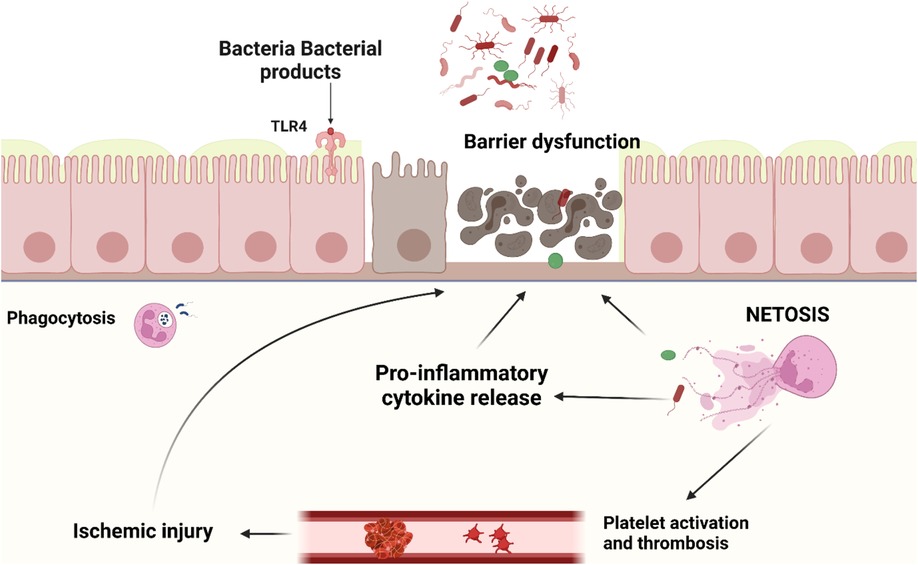
Figure 1. Neutrophil activation and NETs release in intestinal tissue of NEC patients occur as a result of various stimuli such as pathological bacteria, PAMPs and ROS. Though NETs and their components are important to prevent bacterial translocation through the intestine, dysregulated NETs release leads to adjacent epithelial and endothelial injury, cytokine release, platelet activation and thrombus formation. This would result in a vicious cycle of tissue necrosis, further immune activation, ischemia reperfusion injury, uncontrolled inflammation, multi-organ failure, and death.
In neonates, neutrophils exhibit an intrinsic delay in NET formation but are capable of releasing functionally competent NETs (61–64). In a series of elegant experiments, Yost et al. showed that neutrophils isolated from term and preterm infants fail to form NETs in response to ROS, LPS, and bacteria after an hour of incubation. This defect in NET formation was associated with a reduction in extracellular bacterial killing in vitro compared to neutrophils isolated from adults (62). Such differences could explain the increased susceptibility of neonates and preterms in particular to sepsis and infection. To explore the mechanism for this blunted neonatal NET deployment, Yost et al. identified peptides in cord blood from preterm and term infants that inhibit NETosis (63) in vitro and in vivo and appear to be an endogenous regulator of NET generation. Importantly, the authors assessed the ability of neutrophils from preterm neonates longitudinally over the first 28 days after birth for NET formation in response to LPS. NET formation was not demonstrated until day 3 after birth and reached maximum capacity between days 3 and 14. Proteomic analysis identified neonatal NET-inhibitory factor (nNIF) detected in plasma from cord blood of term and preterm infants in the first days after birth and is absent in plasma of adults. Importantly, nNIF use in animal models of inflammation and polymicrobial sepsis improved survival and multiorgan injury, supporting existing evidence that NETs are effectors of collateral vascular and tissue injury in certain pathologies.
Necrotizing enterocolitis and neutrophil extracellular traps
Previous studies showed that NETs release occurs in tissues, serum, and stool of infants and animal models of NEC (26, 65–67). In a prospective pilot study, McQueen et al. showed that infants diagnosed with NEC had increased fecal calprotectin levels compared to infants with NEC “ruled out”. Further analysis using immunohistochemistry, showed an association between calprotectin staining, neutrophil activation markers, and NETs staining in the intestinal tissues of infants with surgical NEC. These data suggest that fecal calprotectin is released, at least in part, as a result of neutrophil infiltration, activation, and potentially NET formation in the intestinal tissue of infants with NEC (67). Other studies later confirmed NETs release in NEC patients and animal models of NEC. Nguyen et showed that preterm infants with NEC and sepsis had higher levels of cell-free DNA (cfDNA), a surrogate marker of circulating NETs, compared to controls (66). Similarly, Chaaban et al. showed increased levels of nucleosomes (histones-DNA), also a surrogate of NETs release, in the serum of infants with NEC stage II and above compared to gestational age-matched controls (65). Analysis of intestinal tissue confirmed neutrophil activation and NET release by immunohistochemical staining of intestinal tissue from preterm infants and a mouse model of NEC. Vincent et al. demonstrated that the pathogenesis of NEC is likely a NET-dependent process (26). They showed that markers of neutrophil activation and NET formation in both serum and histology directly correlate with NEC manifestation, severity, and mortality in a murine model of NEC that utilizes intermittent hypoxia/LSP, and formula feeding. Furthermore, the prevention of NET formation by PAD4 inhibition, using Cl-amidine, significantly reduced NEC histological injury, inflammation, and mortality in the model. The same group further showed that degradation of extracellular DNA in NETs by systemic application of DNase1 leads to a significant reduction in NEC severity, and mortality, suggesting an important role in the pathogenesis of NEC (68). The crucial role of NETs in NEC pathogenesis is further emphasized by the results of Klinke et al. wherein neutrophil concentrations of mice were elevated to match those of human neonates as a method to optimize intestinal injury in the NEC model. Of particular interest is that the NEC severity, tissue damage, and inflammation were significantly reduced, and similar to mice in the control group, in ELANE gene knockout pups, who are incapable of forming NETs (ELANE gene encodes for neutrophil elastase, so knockout results in lack of a key enzyme in NET formation) (69).
These data are in line with the recent studies that suggest, that the degradation of NETs by DNase1 significantly reduces gut-related inflammation, apoptosis of intestinal epithelial cells, and intestinal damage (23). Martinod et al. also showed that suppression of NETs formation by PAD4 inhibition does not impair the ability of neutrophil granulocytes to defend against pathogens and, in particular, does not lead to higher bacteremia or mortality rates in a model of polymicrobial sepsis (70). Moreover, Silva et al. showed that another method of inhibition of NET formation by disulfiram improves organ function and lethality in sepsis (71). Finally, the neonatal NET-inhibitory factor (nNIF) appears to inhibit NET formation in fetuses and neonates in the first days after birth (63). Whether the maturation of NET formation which coincides with the timing for the development of NEC, plays a role in the pathogenesis of NEC, is yet to be determined. It is possible that preterm neonates develop NEC after a period of time, when the protective effects of the nNIF wear off.
In contrast, the use of PAD4 inhibition in another model of NEC characterized by bacteremia, known as the dithizone/klebsiella NEC model was associated with worsened outcomes. NETs inhibition in this model using cl-amidine was associated with increased inflammatory response, increased bacterial translocation, and mortality in the NEC mice (67). Similarly, Saha et al. showed that PAD4-dependent NET generation is indispensable for intestinal clearance of Citrobacter rodentium enteric infection, highlighting the beneficial effects of NETs release in an infectious context (72). These contradicting results strongly suggest that the effects of NET formation may be disease- and model-specific, and in NEC, they depend largely upon the level of intestinal bacterial translocation. They appear to play an integral role in innate defense, especially early on in the clearance of bacterial and bacterial products.
Conclusion
Our current understanding suggests that NETs may be a double-edged sword. They are relevant in the immune defense against pathogenic agents. However, excessive NET ormation induces hyperinflammation, tissue damage, and thrombo-inflammation, contributing to the pathogenesis of a wide variety of diseases such as sepsis, NEC, ARDS, lung injury in COVID-19, ischemia-reperfusion injury, and various oncological diseases. The effect of NETs in pathological conditions is perhaps disease and model specific. In NEC, it is likely dependent on the level of intestinal bacterial translocation. NETs seem crucial in the early phase of the disease to battle bacteremia and reduce bacterial translocation in NEC. However, after the initiation of antibiotic therapy, it may be reasonable to try reducing NET formation through the use of agents like DNases to avert the hyperinflammatory damage caused by NETs. Future studies are needed to further investigate the role of NETs in NEC and other human diseases and explore how best to optimize the beneficial effects and minimize the detrimental effects of NETs for therapy in various human diseases including NEC.
Author contributions
MK and MB performed the literature review, and drafted the first manuscript. HC reviewed the review, and drafted the first manuscript.
Funding
German Research Society (DFG) 446358093 to MB, National Institute of General Medical Sciences (grant nos. K08GM127308 and P20GM134973) to HC.
Conflict of interest
The authors declare that the research was conducted in the absence of any commercial or financial relationships that could be construed as a potential conflict of interest.
Publisher's note
All claims expressed in this article are solely those of the authors and do not necessarily represent those of their affiliated organizations, or those of the publisher, the editors and the reviewers. Any product that may be evaluated in this article, or claim that may be made by its manufacturer, is not guaranteed or endorsed by the publisher.
References
2. Pierro A, Hall N. Surgical treatments of infants with necrotizing enterocolitis. Semin Neonatol. (2003) 8(3):223–32. doi: 10.1016/S1084-2756(03)00025-3
3. Schnabl KL, van Aerde JE, Thomson AB, Clandinin MT. Necrotizing enterocolitis: a multifactorial disease with no cure. World J Gastroenterol. (2008) 14(14):2142–61. doi: 10.3748/wjg.14.2142
4. Pickard SS, Feinstein JA, Popat RA, Huang L, Dutta S. Short- and long-term outcomes of necrotizing enterocolitis in infants with congenital heart disease. Pediatrics. (2009) 123(5):e901–6. doi: 10.1542/peds.2008-3216
5. Lin PW, Stoll BJ. Necrotising enterocolitis. Lancet. (2006) 368(9543):1271–83. doi: 10.1016/S0140-6736(06)69525-1
6. Garg PM, O'fConnor A, Ansari MAY, Vu B, Hobart H, Paschal JL, et al. Hematological predictors of mortality in neonates with fulminant necrotizing enterocolitis. J Perinatol. (2021) 41(5):1110–21. doi: 10.1038/s41372-021-01044-3
7. Ganapathy V, Hay JW, Kim JH, Lee ML, Rechtman DJ. Long term healthcare costs of infants who survived neonatal necrotizing enterocolitis: a retrospective longitudinal study among infants enrolled in Texas medicaid. BMC Pediatr. (2013) 13(1):127. doi: 10.1186/1471-2431-13-127
8. Bazacliu C, Neu J. Necrotizing enterocolitis: long term complications. Curr Pediatr Rev. (2019) 15(2):115–24. doi: 10.2174/1573396315666190312093119
9. Neu J, Modi N, Caplan M. Necrotizing enterocolitis comes in different forms: historical perspectives and defining the disease. Semin Fetal Neonatal Med. (2018) 23(6):370–3. doi: 10.1016/j.siny.2018.07.004
10. Neu J. Necrotizing enterocolitis: the future. Neonatology. (2020) 117(2):240–4. doi: 10.1159/000506866
11. Klinke M, Wiskemann H, Bay B, Schafer HJ, Pagerols Raluy L, Reinshagen K, et al. Cardiac and inflammatory necrotizing enterocolitis in newborns are not the same entity. Front Pediatr. (2020) 8:593926. doi: 10.3389/fped.2020.593926
12. Walker WA. Development of the intestinal mucosal barrier. J Pediatr Gastroenterol Nutr. (2002) 34(Suppl 1); [cited 2022 Dec 10]. Available from: https://pubmed.ncbi.nlm.nih.gov/12082386/.
13. Nanthakumar NN, Fusunyan RD, Sanderson I, Walker WA. Inflammation in the developing human intestine: a possible pathophysiologic contribution to necrotizing enterocolitis. Proc Natl Acad Sci U S A. (2000) 97(11):6043–8. doi: 10.1073/pnas.97.11.6043. [cited 2022 Dec 31 10]. Available from: https://pubmed.ncbi.nlm.nih.gov/10823949/.10823949
14. Lin PW, Nasr TR, Stoll BJ. Necrotizing enterocolitis: recent scientific advances in pathophysiology and prevention. Semin Perinatol. (2008) 32(2):70–82. doi: 10.1053/j.semperi.2008.01.004. [cited 2022 Dec 34 10]. Available from: https://pubmed.ncbi.nlm.nih.gov/18346530/.18346530
15. Good M, Sodhi CP, Egan CE, Afrazi A, Jia H, Yamaguchi Y, et al. Breast milk protects against the development of necrotizing enterocolitis through inhibition of toll-like receptor 4 in the intestinal epithelium via activation of the epidermal growth factor receptor. Mucosal Immunol. (2015) 8(5):1166–79. doi: 10.1038/mi.2015.30
16. Leaphart CL, Cavallo J, Gribar SC, Cetin S, Li J, Branca MF, et al. A critical role for TLR4 in the pathogenesis of necrotizing enterocolitis by modulating intestinal injury and repair. J Immunol. (2007) 179(7):4808–20. doi: 10.4049/jimmunol.179.7.4808
17. Hackam DJ, Sodhi CP. Bench to bedside—new insights into the pathogenesis of necrotizing enterocolitis. Nat Rev Gastroenterol Hepatol. (2022) 19(7):468–79. doi: 10.1038/s41575-022-00594-x
18. Nino DF, Zhou Q, Yamaguchi Y, Martin LY, Wang S, Fulton WB, et al. Cognitive impairments induced by necrotizing enterocolitis can be prevented by inhibiting microglial activation in mouse brain. Sci Transl Med. (2018) 10(471).30541786
19. Willis KA, Ambalavanan N. Necrotizing enterocolitis and the gut-lung axis. Semin Perinatol. (2021) 45(6):151454. doi: 10.1016/j.semperi.2021.151454
20. Jones IH, Hall NJ. Contemporary outcomes for infants with necrotizing enterocolitis-a systematic review. J Pediatr. (2020) 220:86–92.e3. doi: 10.1016/j.jpeds.2019.11.011
21. Vlug LE, Verloop MW, Dierckx B, Bosman L, de Graaf JC, Rings EHHM, et al. Cognitive outcomes in children with conditions affecting the small intestine: a systematic review and meta-analysis. J Pediatr Gastroenterol Nutr. (2022) 74(3):368–76. doi: 10.1097/MPG.0000000000003368
22. Selders GS, Fetz AE, Radic MZ, Bowlin GL. An overview of the role of neutrophils in innate immunity, inflammation and host-biomaterial integration. Regen Biomater. (2017) 4(1):55–68. doi: 10.1093/rb/rbw041
23. Chen F, Liu Y, Shi Y, Zhang J, Liu X, Liu Z, et al. The emerging role of neutrophilic extracellular traps in intestinal disease. Gut Pathog. (2022) 14(1):27. doi: 10.1186/s13099-022-00497-x
24. Brinkmann V, Reichard U, Goosmann C, Fauler B, Uhlemann Y, Weiss DS, et al. Neutrophil extracellular traps kill bacteria. Science. (2004) 303(5663):1532–5. doi: 10.1126/science.1092385. [cited 2022 Dec 96 10]. Available from: https://pubmed.ncbi.nlm.nih.gov/15001782/.15001782
25. Hayashi F, Means TK, Luster AD. Toll-like receptors stimulate human neutrophil function. Blood. (2003) 102(7):2660–9. doi: 10.1182/blood-2003-04-1078
26. Vincent D, Klinke M, Eschenburg G, Trochimiuk M, Appl B, Tiemann B, et al. NEC is likely a NETs dependent process and markers of NETosis are predictive of NEC in mice and humans. Sci Rep. (2018) 8(1):12612. doi: 10.1038/s41598-018-31087-0
27. Olaloye OO, Liu P, Toothaker JM, McCourt BT, McCourt CC, Xiao J, et al. CD16+CD163+monocytes traffic to sites of inflammation during necrotizing enterocolitis in premature infants. J Exp Med. (2021) 218(9). doi: 10.1084/jem.20200344
28. Emami CN, Mittal R, Wang L, Ford HR, Prasadarao Nv. Role of neutrophils and macrophages in the pathogenesis of necrotizing enterocolitis caused by Cronobacter sakazakii. J Surg Res. (2012) 172(1):18–28. doi: 10.1016/j.jss.2011.04.019. [cited 2022 Dec 10]. Available from: https://pubmed.ncbi.nlm.nih.gov/21601887/.21601887
29. Musemeche C, Caplan M, Hsueh W, Sun X, Kelly A. Experimental necrotizing enterocolitis: the role of polymorphonuclear neutrophils. J Pediatr Surg. (1991) 26(9):1047–50. doi: 10.1016/0022-3468(91)90671-F. [cited 2022 Dec 10]. Available from: https://pubmed.ncbi.nlm.nih.gov/1941482/.1941482
30. Kolaczkowska E, Kubes P. Neutrophil recruitment and function in health and inflammation. Nat Rev Immunol. (2013) 13(3):159–75. doi: 10.1038/nri3399
31. Brinkmann V. Neutrophil extracellular traps in the second decade. J Innate Immun. (2018) 10(5–6):414–21. doi: 10.1159/000489829
32. Chen L, Zhao Y, Lai D, Zhang P, Yang Y, Li Y, et al. Neutrophil extracellular traps promote macrophage pyroptosis in sepsis. Cell Death Dis. (2018) 9(6):597. doi: 10.1038/s41419-018-0538-5
33. Papayannopoulos V. Neutrophil extracellular traps in immunity and disease. Nat Rev Immunol. (2018) 18(2):134–47. doi: 10.1038/nri.2017.105
34. Fuchs TA, Abed U, Goosmann C, Hurwitz R, Schulze I, Wahn V, et al. Novel cell death program leads to neutrophil extracellular traps. J Cell Biol. (2007) 176(2):231–41. doi: 10.1083/jcb.200606027
35. Wang Y, Li M, Stadler S, Correll S, Li P, Wang D, et al. Histone hypercitrullination mediates chromatin decondensation and neutrophil extracellular trap formation. J Cell Biol. (2009) 184(2):205–13. doi: 10.1083/jcb.200806072. [cited 2022 Dec 10]. Available from: https://pubmed.ncbi.nlm.nih.gov/19153223/.19153223
36. Jimenez-Alcazar M, Rangaswamy C, Panda R, Bitterling J, Simsek YJ, Long AT, et al. Host DNases prevent vascular occlusion by neutrophil extracellular traps. Science. (2017) 358(6367):1202–6. doi: 10.1126/science.aam8897
37. Clark SR, Ma AC, Tavener SA, McDonald B, Goodarzi Z, Kelly MM, et al. Platelet TLR4 activates neutrophil extracellular traps to ensnare bacteria in septic blood. Nat Med. (2007) 13(4):463–9. doi: 10.1038/nm1565. [cited 2022 Dec 10]. Available from: https://pubmed.ncbi.nlm.nih.gov/17384648/.17384648
38. McDonald B, Urrutia R, Yipp BG, Jenne CN, Kubes P. Intravascular neutrophil extracellular traps capture bacteria from the bloodstream during sepsis. Cell Host Microbe. (2012) 12(3):324–33. doi: 10.1016/j.chom.2012.06.011. [cited 2022 Dec 10]. Available from: https://pubmed.ncbi.nlm.nih.gov/22980329/.22980329
39. Chen Z, Zhang H, Qu M, Nan K, Cao H, Cata JP, et al. Review: the emerging role of neutrophil extracellular traps in sepsis and sepsis-associated thrombosis. Front Cell Infect Microbiol. (2021) 11. [cited 2021 Aug 10]. Available from: /pmc/articles/PMC8010653/.
40. Brill A, Fuchs TA, Savchenko AS, Thomas GM, Martiod K, De Meyer SF, et al. Neutrophil extracellular traps promote deep vein thrombosis in mice. J Thromb Haemost. (2012) 10(1):136–44. doi: 10.1111/j.1538-7836.2011.04544.x. [cited 2022 Dec 10]. Available from: https://pubmed.ncbi.nlm.nih.gov/22044575/.22044575
41. Jorch SK, Kubes P. An emerging role for neutrophil extracellular traps in noninfectious disease. Nat Med. (2017) 23(3):279–87. doi: 10.1038/nm.4294
42. Dominguez-Diaz C, Varela-Trinidad GU, Munoz-Sanchez G, Solorzano-Castanedo K, Avila-Arrezola KE, Iniguez-Gutierrez L, et al. To trap a pathogen: neutrophil extracellular traps and their role in mucosal epithelial and skin diseases. Cells. (2021) 10(6). doi: 10.3390/cells10061469
43. Bruschi M, Moroni G, Sinico RA, Franceschini F, Fredi M, Vaglio A, et al. Neutrophil extracellular traps in the autoimmunity context. Front Med. (2021) 8:614829. doi: 10.3389/fmed.2021.614829
44. Heuer A, Stiel C, Elrod J, Konigs I, Vincent D, Schlegel P, et al. Therapeutic targeting of neutrophil extracellular traps improves primary and secondary intention wound healing in mice. Front Immunol. (2021) 12:614347. doi: 10.3389/fimmu.2021.614347
45. Zhu F, Wang L, Gong Z, Wang Y, Gao Y, Cai W, et al. Blockage of NLRP3 inflammasome activation ameliorates acute inflammatory injury and long-term cognitive impairment induced by necrotizing enterocolitis in mice. J Neuroinflammation. (2021) 18(1):66. doi: 10.1186/s12974-021-02111-4
46. Fuchs TA, Bhandari AA, Wagner DD. Histones induce rapid and profound thrombocytopenia in mice. Blood. (2011) 118(13):3708–14. doi: 10.1182/blood-2011-01-332676
47. Chaaban H, Keshari RS, Silasi-Mansat R, Popescu NI, Mehta-D'LSouza P, Lim Y, et al. Inter-α inhibitor protein and its associated glycosaminoglycans protect against histone-induced injury. Blood. (2015) 125(14):2286–96. doi: 10.1182/blood-2014-06-582759
48. Abrams ST, Zhang N, Manson J, Liu T, Dart C, Baluwa F, et al. Circulating histones are mediators of trauma-associated lung injury. Am J Respir Crit Care Med. (2013) 187(2):160–9. doi: 10.1164/rccm.201206-1037OC
49. Allam R, Scherbaum CR, Darisipudi MN, Mulay SR, Hagele H, Lichtnekert J, et al. Histones from dying renal cells aggravate kidney injury via TLR2 and TLR4. J Am Soc Nephrol. (2012) 23(8):1375–88. doi: 10.1681/ASN.2011111077
50. Semeraro F, Ammollo CT, Morrissey JH, Dale GL, Friese P, Esmon NL, et al. Extracellular histones promote thrombin generation through platelet-dependent mechanisms: involvement of platelet TLR2 and TLR4. Blood. (2011) 118(7):1952–61. doi: 10.1182/blood-2011-03-343061
51. Xu J, Zhang X, Monestier M, Esmon NL, Esmon CT. Extracellular histones are mediators of death through TLR2 and TLR4 in mouse fatal liver injury. J Immunol. (2011) 187(5):2626–31. doi: 10.4049/jimmunol.1003930
52. Yipp BG, Kubes P. NETosis: how vital is it? Blood. (2013) 122(16):2784–94. doi: 10.1182/blood-2013-04-457671
53. Caudrillier A, Kessenbrock K, Gilliss BM, Nguyen JX, Marques MB, Monestier M, et al. Platelets induce neutrophil extracellular traps in transfusion-related acute lung injury. J Clin Invest. (2012) 122(7):2661–71. doi: 10.1172/JCI61303
54. Veras FP, Pontelli MC, Silva CM, Toller-Kawahisa JE, de Lima M, Nascimento DC, et al. SARS-CoV-2-triggered neutrophil extracellular traps mediate COVID-19 pathology. J Exp Med. (2020) 217(12). doi: 10.1084/jem.20201129
55. Liang Y, Wang X, He D, You Q, Zhang T, Dong W, et al. Ameliorating gut microenvironment through staphylococcal nuclease-mediated intestinal NETs degradation for prevention of type 1 diabetes in NOD mice. Life Sci. (2019) 221:301–10. doi: 10.1016/j.lfs.2019.02.034
56. Lood C, Blanco LP, Purmalek MM, Carmona-Rivera C, De Ravin SS, Smith CK, et al. Neutrophil extracellular traps enriched in oxidized mitochondrial DNA are interferogenic and contribute to lupus-like disease. Nat Med. (2016) 22(2):146–53. doi: 10.1038/nm.4027
57. Boettcher M, Eschenburg G, Mietzsch S, Jimenez-Alcazar M, Klinke M, Vincent D, et al. Therapeutic targeting of extracellular DNA improves the outcome of intestinal ischemic reperfusion injury in neonatal rats. Sci Rep. (2017) 7(1):15377. doi: 10.1038/s41598-017-15807-6
58. Boettcher M, Meier D, Jimenez-Alcazar M, Eschenburg G, Mietzsch S, Vincent D, et al. Degradation of extracellular DNA by DNase1 significantly reduces testicular damage after testicular torsion in rats. Urology. (2017) 109:223.e1–7. doi: 10.1016/j.urology.2017.07.031
59. Xu J, Zhang X, Pelayo R, Monestier M, Ammollo CT, Semeraro F, et al. Extracellular histones are major mediators of death in sepsis. Nat Med. (2009) 15(11):1318–21. doi: 10.1038/nm.2053
60. Laridan E, Denorme F, Desender L, Francois O, Andersson T, Deckmyn H, et al. Neutrophil extracellular traps in ischemic stroke thrombi. Ann Neurol. (2017) 82(2):223–32. doi: 10.1002/ana.24993. [cited 2022 Dec 10]. Available from: https://pubmed.ncbi.nlm.nih.gov/28696508/.28696508
61. Marcos V, Nussbaum C, Vitkov L, Hector A, Wiedenbauer EM, Roos D, et al. Delayed but functional neutrophil extracellular trap formation in neonates. Blood. (2009) 114(23):4908–11. doi: 10.1182/blood-2009-09-242388
62. Yost CC, Cody MJ, Harris ES, Thornton NL, McInturff AM, Martinez ML, et al. Impaired neutrophil extracellular trap (NET) formation: a novel innate immune deficiency of human neonates. Blood. (2009) 113(25):6419–27. doi: 10.1182/blood-2008-07-171629
63. Yost CC, Schwertz H, Cody MJ, Wallace JA, Campbell RA, Vieira-de-Abreu A, et al. Neonatal NET-inhibitory factor and related peptides inhibit neutrophil extracellular trap formation. J Clin Invest. (2016) 126(10):3783–98. doi: 10.1172/JCI83873
64. Yost CC, Zimmerman GA. Response: gestational age as a factor in neutrophil extracellular trap formation. Blood. (2009) 114(23):4911–2. doi: 10.1182/blood-2009-10-243048
65. Chaaban H, Burge K, Eckert J, Keshari RS, Silasi R, Lupu C, et al. Neutrophil extracellular trap inhibition increases inflammation, bacteraemia and mortality in murine necrotizing enterocolitis. J Cell Mol Med. (2021) 25(23):10814–24. doi: 10.1111/jcmm.15338. [cited 2022 Dec 10]. Available from: /pmc/articles/PMC8642694/.32515131
66. Nguyen DN, Stensballe A, Lai JC, Jiang P, Brunse A, Li Y, et al. Elevated levels of circulating cell-free DNA and neutrophil proteins are associated with neonatal sepsis and necrotizing enterocolitis in immature mice, pigs and infants. Innate Immun. (2017) 23(6):524–36. doi: 10.1177/1753425917719995. [cited 2022 Dec 10]. Available from: https://pubmed.ncbi.nlm.nih.gov/28714327/.28714327
67. MacQueen BC, Christensen RD, Yost CC, Lambert DK, Baer VL, Sheffield MJ, et al. Elevated fecal calprotectin levels during necrotizing enterocolitis are associated with activated neutrophils extruding neutrophil extracellular traps. J Perinatol. (2016) 36(10):862–9. doi: 10.1038/jp.2016.105
68. Klinke M, Vincent D, Trochimiuk M, Appl B, Tiemann B, Bergholz R, et al. Degradation of extracellular DNA significantly ameliorates necrotizing enterocolitis severity in mice. J Surg Res. (2019) 235:513–20. doi: 10.1016/j.jss.2018.10.041
69. Klinke M, Vincent D, Trochimiuk M, Appl B, Tiemann B, Reinshagen K, et al. Development of an improved murine model of necrotizing enterocolitis shows the importance of neutrophils in NEC pathogenesis. Sci Rep. (2020) 10(1):8049. doi: 10.1038/s41598-020-65120-y
70. Martinod K, Fuchs TA, Zitomersky NL, Wong SL, Demers M, Gallant M, et al. PAD4-deficiency does not affect bacteremia in polymicrobial sepsis and ameliorates endotoxemic shock. Blood. (2015) 125(12):1948–56. doi: 10.1182/blood-2014-07-587709
71. Silva CMS, Wanderley CWS, Veras FP, Sonego F, Nascimento DC, Goncalves AV, et al. Gasdermin D inhibition prevents multiple organ dysfunction during sepsis by blocking NET formation. Blood. (2021) 138(25):2702–13. doi: 10.1182/blood.2021011525
72. Saha P, Yeoh BS, Xiao X, Golonka RM, Singh V, Wang Y, et al. PAD4-dependent NETs generation are indispensable for intestinal clearance of Citrobacter rodentium. Mucosal Immunol. (2019) 12(3):761–71. doi: 10.1038/s41385-019-0139-3. [cited 2022 Dec 10]. Available from: https://pubmed.ncbi.nlm.nih.gov/30710097/.30710097
Keywords: neutrophils, NETs (neutrophil extracellular traps), NEC (necrotizing enterocolitis), intestinal disease
Citation: Klinke M, Chaaban H and Boettcher M (2023) The role of neutrophil extracellular traps in necrotizing enterocolitis. Front. Pediatr. 11:1121193. doi: 10.3389/fped.2023.1121193
Received: 11 December 2022; Accepted: 27 February 2023;
Published: 15 March 2023.
Edited by:
Minesh Khashu, University Hospitals Dorset NHS Foundation Trust, United KingdomReviewed by:
Dhirendra Kumar Singh, University of North Carolina at Chapel Hill, United StatesChristian Con Yost, The University of Utah, United States
© 2023 Klinke, Chaaban and Boettcher. This is an open-access article distributed under the terms of the Creative Commons Attribution License (CC BY). The use, distribution or reproduction in other forums is permitted, provided the original author(s) and the copyright owner(s) are credited and that the original publication in this journal is cited, in accordance with accepted academic practice. No use, distribution or reproduction is permitted which does not comply with these terms.
*Correspondence: Michael Boettcher michboettcher@gmail.com
Specialty Section: This article was submitted to Neonatology, a section of the journal Frontiers in Pediatrics