- 1Department of Surgery, Ann and Robert H. Lurie Children's Hospital, Chicago Institute for Fetal Health, Northwestern University Feinberg School of Medicine, Chicago, IL, United States
- 2Cincinnati Fetal Care Center, Cincinnati Children's Hospital, University of Cincinnati College of Medicine, Cincinnati, OH, United States
- 3Neonatal Surgical Unit, Medical and Surgical Department of the Fetus, Newborn and Infant, Bambino Gesù Children's Hospital, Rome, Italy
Objective: Liver herniation is a known risk factor for increased severity in CDH and is associated with clinically significant pulmonary hypoplasia and pulmonary hypertension. Better studies are needed to understand the growth of the herniated liver compared to the liver that remains in the abdomen and how this liver growth then affects lung development. Serial hi-resolution fetal MRI enables characterization of liver growth throughout gestation and examination of macroscopic features that may regulate liver growth. Here, we hypothesized that the nature of liver herniation affects liver growth and, in turn, affects lung growth.
Methods: Clinical data were retrospectively collected from consecutive cases of prenatally diagnosed isolated left-sided or right-sided CDH from June 2006 to August 2021. Only those cases with MRI lung volumetry for both mid-gestation and late-gestation time points were recruited for analysis. Cases with fetal chromosomal abnormalities and other major structural abnormalities were excluded. Fractional liver volume and liver growth was indexed to estimated fetal weight and compared to lung growth.
Results: Data was collected from 28 fetuses with a left liver-down CDH (LLD), 37 left liver-up CDH (LLU) and 9 right liver-up CDH (RLU). Overall, RLU fetuses had greater overall and fractional (intra-thoracic vs. intra-abdominal) liver growth when compared to LLD and LLU fetuses. Additionally, intra-thoracic liver growth was consistently slower than intra-abdominal liver growth for either right- or left-sided CDH. When the liver was not herniated, a positive correlation was seen between liver growth and lung growth. However, when the liver was herniated above the diaphragm, this positive correlation was lost.
Conclusion: Right-sided CDH fetuses exhibit greater liver growth compared to left-sided CDH. Liver herniation disrupts the normal positive correlation between liver and lung growth that is seen when the liver is entirely within the abdomen.
Introduction
Congenital diaphragmatic hernia (CDH) occurs in 1 in 2,500 to 1 in 5,000 live births and is associated with significant neonatal morbidity and mortality (1–4). Advances in prenatal imaging permit risk-stratification for affected fetuses using multiple static indices including o/e LHR, o/e TLV, PPLV, %LH and LiTR (5–14). An assessment of these parameters facilitates prenatal counseling, delivery planning and, more recently, determines eligibility for prenatal intervention (15, 16). There is an inherent lack of precision in these indices as they are static, rather than trended throughout gestation. Dynamic variables are needed to better understand outcomes to the fetus, particularly late in gestation when liver growth above the diaphragm may adversely affect lung growth. Understanding factors that affect lung development throughout gestation in CDH will aid in the development of more precise dynamic predictors of patient outcomes.
Liver herniation carries a worse prognosis in CDH and is associated with pulmonary hypoplasia and pulmonary hypertension (17–20). In the experimental nitrofen-induced CDH rat models, larger liver volumes were seen in mice with CDH compared to controls (21). Additionally, larger intrathoracic liver volumes were seen in mice with larger diaphragmatic defects which then correlated with more severe pulmonary hypoplasia (21). In humans, liver herniation into the chest is an indicator of worse prognosis (8–10). The fraction of liver herniation has been shown to predict need for ECMO support and overall survival (6–13, 17, 22). Additionally, the ratio of the intrathoracic liver volume to total thoracic volume (LiTR) predicted postnatal survival independently from o/e TLV (11). When looking at multiple modes of liver and lung assessment, a combination of o/e TLV and %LH provided the best predictive measure for ECMO use and mortality (9). Given the rigid structure of the chest, growth of the intrathoracic liver throughout gestation likely has a negative impact on fetal lung development. Yet, no study in CDH fetuses exists to directly challenge this postulate. Serial hi-resolution fetal MRI enables simultaneous characterization of liver and lung growth throughout gestation and illuminates the consequences of these seemingly reciprocal developmental processes in the CDH fetus. In this study, serial MRI is used to define the relationship of liver growth to lung growth in both left and right sided CDH in utero.
Materials and methods
Study population
Clinical data was collected from cases of both prenatally diagnosed left-sided and right-sided CDH at the Cincinnati Fetal Center and the Chicago Institute for Fetal Health from June 2006 to August 2021 with institutional review board approval (IRB # 2011-2626 and IRB #2020-3050). Only those with complete information on MRI-derived lung volume for both mid-gestation (18–30 completed weeks gestation) and late-gestation (after 30 completed weeks gestation) time points were included in the analysis. Exclusion criteria were fetal chromosomal abnormalities and other major structural abnormalities. No patients in the study population underwent prenatal intervention. Patients were divided into either left-sided CDH with liver entirely down in the abdomen (LLD), left- or right-sided CDH with liver up (LLU or RLU). All patients with a right-sided CDH had some degree of liver herniation into the chest. Gestational age was determined from a well-defined last menstrual period and/or by sonographic biometry in the first trimester of pregnancy.
Prenatal MRI evaluation
Fetal Ultrafast MRI study was performed using a 1.5-T scanner (Horizon; General Electric Medical System, Milwaukee, WI and Ingenia; Phillips Healthcare, Holland) without maternal or fetal sedation. A torso-phased array coil was used to optimize all studies. The MRI protocol included two sequences: a single-shot fast-spin echo T2-weighted sequence with matrix of 224 × 192, a field of view of 30 or less, and a gradient-echo T1-weighted sequence with a matrix of 256 × 192, a field of view of 30–35. The T2 weighted imaging was performed at a slice thickness of 3 or 4 mm and T1 sequences at 5 mm. The corresponding areas of liver down and liver up were determined on each MRI section by using a free-form region of interest on a Vitrea workstation (Vital Images Inc, Minnetonka, MN). Delineation of the liver up and liver down on the ipsilateral site of defect was determined by mirroring the contour of the contralateral diaphragm. Liver volumes were then calculated from T1-weighted images acquired in the coronal plane, which were sequential and without motion, and the summation of the liver areas from all coronal images were multiplied by the thickness of the section. All MRI scans were reviewed and reported by 2 independent fetal radiologists with combined 40 years of experience. Using this methodology, intra- and inter-reviewer reproducibility has been demonstrated to be moderate-to-good for liver volumes above the diaphragm and good-to-excellent for liver volumes below the diaphragm after standardization of the technique (23).
The following MRI findings were collected for analysis: LU (liver up), LD (liver down), and LT (liver total) in milliliters (ml). Examples of T1 MRI images from a left sided CDH fetus with liver herniation are shown in Figure 1 both before (A.) and after (B.) tracing of the liver. Figure 1C. shows a three dimensional representation of the liver volumes from the same patient. The presence of intrathoracic liver was defined as any portion of liver detected in the thoracic cavity, identified by MRI study. LT was calculated by the summation of the LD and LU (measured in mL). To track liver growth relative to fetal size, fractional and total liver volumes and liver growth were also normalized to estimated fetal weight (EFW) measured late in gestation (EFW2) to normalize the liver growth against the growth of the fetus overall. MRI lung volumes were calculated from coronal T2 weighted non-motion sequences and also obtained by the 2-D areas multiplied by the thickness of the slice. Total lung volume was then obtained via the summation of the right and left lung volumes (in ml). The o/e TLV volumes were calculated according to the method outlined by Rypens et al. (24). Lung growth was measured as ΔTLV/EFW2/ΔT.
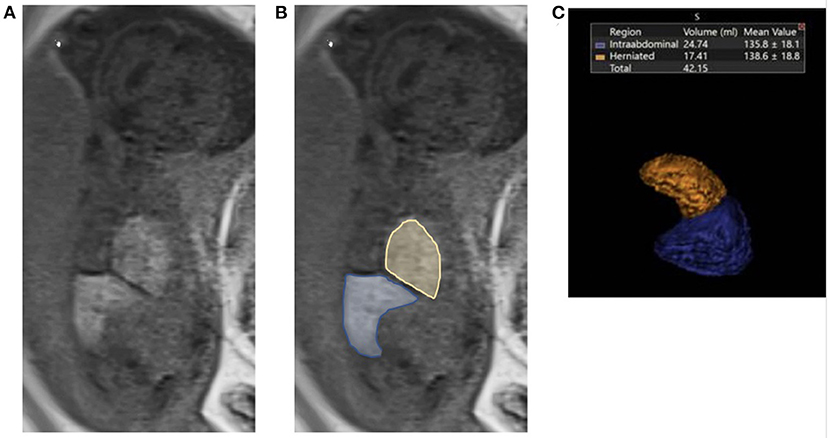
Figure 1. Fetal liver volumetry using T1 MRI images. (A) Liver down (blue) and liver up (yellow) volumes were determined on each MRI section by using a free-form region of interest on a Vitrea workstation (Vital Images Inc, Minnetonka, MN). (B) Delineation of the liver up and liver down on the ipsilateral site of defect was determined by mirroring the contour of the contralateral diaphragm. Liver volumes were then calculated in the T1-weighted coronal plane by using sequences that allowed complete imaging of the liver and without artifacts from motion and the summation. (C) Three dimensional representation of the liver volumes from the same patient.
Statistical analysis
Graphs were created in Graphpad Software Version 9.4.0 (San Diego, CA) to characterize liver volumes and liver growth. Linear regression was calculated assessing liver growth and lung growth. Statistical analyses were performed with IBM Corp. © SPSS Statistics, Version 28.0.1.0 (Armonk, NY).
Results
Cohort characteristics
Baseline maternal and fetal characteristics, growth parameters and o/e LHR are presented in Table 1. Maternal age at delivery, birthweight, and gestational age at delivery were similar between groups. Mid gestational age at time of first MRI and late gestational age at time of second MRI were also similar between groups. Estimated fetal weight was measured for all groups at both mid and late gestation. There was no significant difference in baseline growth parameters between the groups. In order to measure disease severity, o/e LHR was measured. o/e LHR was higher in the liver down group compared to the liver up groups. There was no significant difference between the liver up groups.
Liver volumes in right-sided CDH lower in mid-gestation and higher in late-gestation
A comparison of static liver volumes normalized to estimated fetal weight between the groups (LLD, LLU, and RLU) is summarized in Table 2. When normalized to EFW, RLU fetuses had lower mid-gestation and higher late-gestation liver down volumes when compared to LLD and LLU fetuses, as shown in Table 2. LLU fetuses also had lower liver-down volumes compared to LLD fetuses at both time points. RLU fetuses had higher late-gestation liver up volumes when normalized to EFW compared to LLU fetuses. Taken together, these findings show that RLU fetuses had a higher intrathoracic liver volume and a higher total liver volume when compared to both LLU and LLD fetuses despite having a lower total liver volume in mid-gestation.
Faster liver growth in right sided CDH compared to left
Table 3 compares liver growth among the groups. When normalized to EFW, RLU fetuses had faster liver growth above the diaphragm than LLU fetuses. RLU fetuses had significantly more intrathoracic liver growth compared to LLU fetuses. RLU fetuses also had more liver down growth compared to LLU fetuses but similar liver down growth when compared to LLD. Total liver growth was also greater in the RLU fetuses when compared to both the LLU and LLD fetuses. Figure 2 shows that liver growth below the diaphragm was greater than liver growth above the diaphragm when normalized to EFW for both right- and left-sided CDH fetuses.
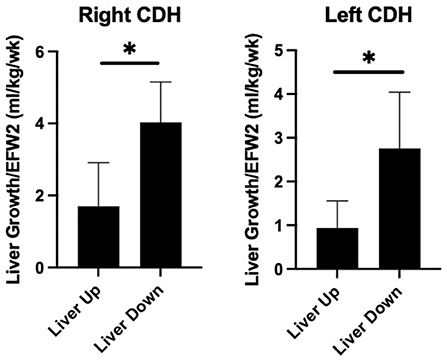
Figure 2. Greater liver growth below the diaphragm in prenatal CDH. Comparison of fractional liver growth (liver up and liver down) normalized to late gestation estimated fetal weight in right and left sided CDH fetuses. As shown, liver growth below the diaphragm is greater than the growth above the diaphragm in both right and left sided CDH (*p < 0.05).
Intrathoracic liver growth adversely affects lung growth
Figure 3 shows the relationship between liver growth and lung growth in fetuses with and without liver herniation. Lung growth correlated positively with liver growth for fetuses without liver herniation (R2 = 0.38; p < 0.05). However, the positive correlation between lung growth and liver growth was lost when the liver was herniated above the diaphragm. Liver growth was not associated with o/e LHR in the liver down group. Liver growth was correlated with lower o/e LHR in the RLU group (R2 = 0.42) however in the small sample size this association is not significant (Figure 4). o/e LHR also down trended with increasing liver growth in the LLU group but this correlation was also not significant.
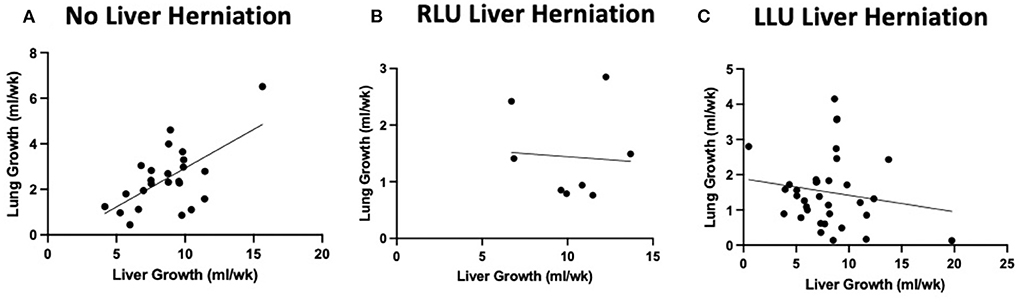
Figure 3. Liver herniation alters normal positive correlation between liver growth and lung growth. Graphical relationship between total liver growth and total lung growth in cases without liver herniation (LLD) and with liver herniation (RLU and LLU). (A) Positive correlation between total lung growth and total liver growth when the liver is not herniated above the diaphragm (R2 = 0.38). (B,C) Loss of positive correlation between lung growth and liver growth when liver is herniated above the diaphragm in both RLU and LLU groups.
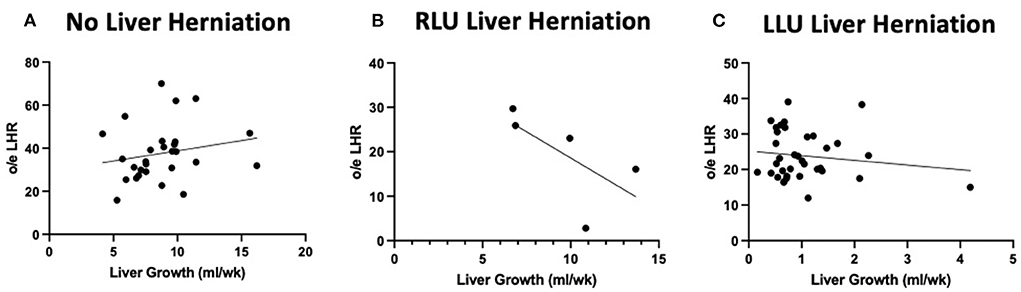
Figure 4. Liver growth correlations to o/e LHR in both herniated and non herniated groups. Graphical relationship between total liver growth and o/e LHR in cases without liver herniation (LLD) and with liver herniation (RLU and LLU). (A) No correlation between total o/e LHR and total liver growth when the liver is not herniated above the diaphragm. (B) A negative correlation between o/e LHR and liver growth when liver is herniated above the diaphragm in RLU group (R2 = 0.42). (C) No correlation between o/e LHR and liver growth when liver is herniated above the diaphragm in LLU group.
Discussion
In the current study, utilizing serial MRI, the relationship between liver and lung growth in utero is described. The presence of a right-sided diaphragmatic defect was associated with faster intrathoracic and overall fetal liver growth albeit in a small sample size. Liver growth below the diaphragm was greater than liver growth above the diaphragm independent of the side of the defect. Liver growth in CDH fetuses without liver herniation was correlated with increasing lung growth but this association was lost in cases with liver herniation above the diaphragm.
An understanding of how the liver grows in utero is limited despite many efforts both in animal models and humans. Under normal conditions, the total liver mass is limited by total body mass suggesting that liver size is determined physiologically by the size of the abdominal cavity (25). This relationship to body mass is also supported with the observation that liver ceases to regenerate after resection once the normal liver volume is achieved (26). Changes in the extracellular matrix changes and growth factor release also play a role in determining timing and degree of liver growth (27–29). The mechanisms regulating liver development in normal gestation are not well understood. Similarly, the regulation of liver growth under abnormal conditions such as in CDH has not been studied.
The current findings reveal that the side of the CDH affects the total and intrathoracic liver growth suggesting that the physical relationship to the diaphragm is a key determinant of liver growth. Additionally, liver growth is greater below when compared to above the diaphragm. This finding parallels findings from a recent study in a fetal rabbit model of CDH (30). The authors found that the intra-thoracic liver weight was higher in right-sided CDH (30). They also showed that there was corresponding lower lung weight in both left- and right-sided CDH when the liver was growing in the chest (30). The authors postulated that these differences could be due to lower compartmental pressures when the defect is directly over the liver allowing for increasing liver growth. Additionally, studies in the nitrofen rat model of CDH suggest that the liver does not simply herniate into the chest but rather the liver grows into the thorax due to lack of compression by the diaphragm (31). Lastly, fetal ultrasound studies of clinical CDH cases reveal that the herniated liver molds to the surrounding chest wall and contains a unique vasculature to support the altered form (32, 33).
The greater liver growth seen in right vs. left sided CDH may have resulted from slower growth of the intrathoracic liver in left-sided CDH due to kinking of the hepatic artery and portal vein. The decreased blood flow to the liver above the diaphragm could result in less liver growth over time (34–36). Fetal liver blood flow affects fetal growth and body composition (37, 38). The fetal liver is oxygenated via the hepatic artery, umbilical vein and the portal vein. Prior studies have demonstrated that increasing blood flow from the umbilical vein leads to higher cell proliferation in the liver, heart, skeletal muscle and kidneys in fetal lamb (39). In addition, studies of human low-risk pregnancies have shown that larger liver size is associated with higher umbilical vein flow to the fetal liver (40). Additionally, the ductus venosus seems to be mechanically compressed when the liver is in the chest which is more severe in right sided CDH and may result in increased blood flow to the liver in these cases (41, 42). Differences in compartmental pressures as well as blood flow between right vs. left CDH may impact both intrathoracic and total liver growth.
In this study, CDH fetuses without liver herniation exhibited a positive correlation with lung growth. However, with liver herniation, this relationship was lost and lung growth was poor throughout gestation in liver up hernias. The severity of lung hypoplasia is the critical parameter affecting survival in cases of prenatally diagnosed CDH (43–45). Several static variables for liver growth and lung growth have been correlated. The current findings extend prior observations and illustrate the relationship between the dynamic changes in the liver and lung throughout development in CDH patients (5, 46). When the liver is not growing in the chest, liver and lung growth parallel fetal growth. When the liver is herniated and growing in the chest, this positive correlation is lost and lung growth decreases as liver growth increases.
The interpretation of the current findings must account for a number of limitations. First, this is a retrospective review of clinical MRI exams taken from a research database. As such, only correlative assessments are possible. Second, the rarity of right-sided CDH cases limits the number of patients in the current analysis and thus limits conclusions resulting from comparisons including this group. Third, MRI-derived volumetric measurements may overestimate tissue volume during fetal development when compared to direct measures such as experimental tissue displacement. However, relative volumes for perfused tissue in situ may not be adequately captured by analysis after animal necropsy and may be better estimated with live cross-sectional imaging (47). Fourth, liver growth was correlated with lung growth alone. There are arguably several other factors involved including the stomach position. Additionally, other outcomes could be evaluated including growth of the pulmonary vasculature, the trachea and bronchi, the left ventricle and the aortic arch. These disease manifestations are critical outcomes and will be important areas for future study. Finally, inter-reviewer reproducibility was not verified in this study, but has been demonstrated to be moderate-to-good for liver volumes above the diaphragm and good-to-excellent for liver volumes below the diaphragm after standardization (23).
More research is needed to better understand the complex interplay between liver and lung growth in CDH patients. Previous studies in rat embryos have shown that the volume of liver herniated into the chest is associated with reduced lung volume (48). In humans, presence of the liver above the diaphragm is a marker of increased severity; however, herniation of the liver in right sided CDH is almost always present and may carry less prognostic significance (19, 22, 49).
The current findings illustrate the potential for liver growth to influence lung growth in CDH fetuses. Future studies incorporating liver and stomach growth in relation to MRI-measured growth of: (1) the pulmonary vasculature; (2) the trachea and bronchi; (3) the left ventricle; and (3) the aortic arch will better characterize the broader impact of liver growth and stomach position on the severity of pulmonary hypoplasia, pulmonary hypertension, tracheobronchomalacia, small left-sided heart structures and aortic arch hypoplasia. Ultimately, these disease manifestations are the critical outcomes that may be affected by growth of the abdominal viscera in the fetal chest.
Conclusion
The current study utilized serial MRI volumetrics to demonstrate that the side of the hernia results in different liver growth trajectories above the diaphragm as well as overall changes in total liver growth. Additionally, when the liver is not growing in the chest, the liver and lung growth correlate throughout gestation as the fetus grows. But, when the liver is herniated and growing in the chest, this correlation is lost and lung growth actually decreases as liver growth increases. The greater significance of the interplay between liver and lung growth will guide future studies to explain how the side of the diaphragmatic hernia as well as altered compartmental pressure and liver blood flow regulate the molecular and cellular mechanisms of liver and lung growth in CDH fetuses.
Data availability statement
The raw data supporting the conclusions of this article will be made available by the authors, without undue reservation.
Ethics statement
The studies involving human participants were reviewed and approved by the institutional review board at Cincinnati Children's Hospital Medical Center as well as Ann and Robert H. Lurie Children's Hospital. The patients/participants provided their written informed consent to participate in this study.
Author contributions
All persons who meet authorship criteria are listed as authors, and certify that they have participated sufficiently in the work to take public responsibility for the content, including participation in the concept, design, analysis, writing, or revision of the manuscript. All authors contributed to the article and approved the submitted version.
Funding
The authors declare that the research was conducted in the absence of any commercial or financial relationships that could be construed as a potential conflict of interest.
Conflict of interest
The authors declare that the research was conducted in the absence of any commercial or financial relationships that could be construed as a potential conflict of interest.
Publisher's note
All claims expressed in this article are solely those of the authors and do not necessarily represent those of their affiliated organizations, or those of the publisher, the editors and the reviewers. Any product that may be evaluated in this article, or claim that may be made by its manufacturer, is not guaranteed or endorsed by the publisher.
References
1. Gallot D, Boda C, Ughetto S, Perthus I, Robert-Gnansia E, Francannet C, et al. Prenatal detection and outcome of congenital diaphragmatic hernia: a French registry-based study. Ultrasound Obstet Gynecol Off J Int Soc Ultrasound Obstet Gynecol. (2007) 29:276–83. doi: 10.1002/uog.3863
2. Hedrick HL. Evaluation and management of congenital diaphragmatic hernia. Pediatr Case Rev. (2001) 1:25–36. doi: 10.1097/00132584-200110000-00004
3. Stege G, Fenton A, Jaffray B. Nihilism in the 1990s: the true mortality of congenital diaphragmatic hernia. Pediatrics. (2003) 112:532–5. doi: 10.1542/peds.112.3.532
4. Coughlin MA, Gupta VS, Ebanks AH, Harting MT, Lally KP. Congenital diaphragmatic hernia study group. Incidence and outcomes of patients with congenital diaphragmatic hernia and pulmonary sequestration. J Pediatr Surg. (2021) 56:1126–9. doi: 10.1016/j.jpedsurg.2021.02.032
5. Cannie M, Jani J, Meersschaert J, Allegaert K. Done' E, Marchal G, Deprest J, Dymarkowski S. Prenatal prediction of survival in isolated diaphragmatic hernia using observed to expected total fetal lung volume determined by magnetic resonance imaging based on either gestational age or fetal body volume. Ultrasound Obstetr Gynecol Off J Int Soc Ultrasound Obstet Gynecol. (2008) 32:633–9. doi: 10.1002/uog.6139
6. Phithakwatchara N, Coleman A, Peiro JL, Lee AE, Keswani SG, Kline-Fath B, et al. Differential patterns of prenatal ipsilateral and contralateral lung growth in cases of isolated left-sided congenital diaphragmatic hernia. Prenat Diagn. (2015) 35:769–76. doi: 10.1002/pd.4605
7. Phithakwatchara N, Coleman A, Peiro JL, Lee AE, Keswani SG, Kline-Fath B, et al. Expanded intrathoracic space in fetal cases of isolated congenital diaphragmatic hernia contributes to disparity between percent predicted lung volume and observed to expected total lung volume. Prenat Diagn. (2015) 35:154–9. doi: 10.1002/pd.4508
8. Werneck Britto IS, Olutoye OO, Cass DL, Zamora IJ, Lee TC, Cassady CI, et al. Quantification of liver herniation in fetuses with isolated congenital diaphragmatic hernia using two-dimensional ultrasonography. Ultrasound Obstet Gynecol. (2015) 46:150–4. doi: 10.1002/uog.14718
9. Ruano R, Lazar DA, Cass DL, Zamora IJ, Lee TC, Cassady CI, et al. Fetal lung volume and quantification of liver herniation by magnetic resonance imaging in isolated congenital diaphragmatic hernia. Ultrasound Obstet Gynecol. (2014) 43:662–9. doi: 10.1002/uog.13223
10. Lazar DA, Ruano R, Cass DL, Moise KJ Jr, Johnson A, Lee TC, et al. Defining “liver-up”: does the volume of liver herniation predict outcome for fetuses with isolated left-sided congenital diaphragmatic hernia? J Pediatr Surg. (2012) 47:1058–62. doi: 10.1016/j.jpedsurg.2012.03.003
11. Cannie M, Jani J, Chaffiotte C, Vaast P, Deruelle P, Houfflin-Debarge V, et al. Quantification of intrathoracic liver herniation by magnetic resonance imaging and prediction of postnatal survival in fetuses with congenital diaphragmatic hernia. Ultrasound Obstet Gynecol. (2008) 32:627–32. doi: 10.1002/uog.6146
12. Cordier AG, Cannie MM, Guilbaud L, De Laveaucoupet J, Martinovic J, Nowakowska D, et al. Lesegno B, Votino C, Senat MV, Jani JC, Benachi A. Stomach position versus liver-to-thoracic volume ratio in left-sided congenital diaphragmatic hernia. J Matern Fetal Neonatal Med. (2015) 28:190–5. doi: 10.3109/14767058.2014.906576
13. Cannie MM, Cordier AG, De Laveaucoupet J, Franchi-Abella S, Cagneaux M, Prodhomme O, et al. Liver-to-thoracic volume ratio: use at MR imaging to predict postnatal survival in fetuses with isolated congenital diaphragmatic hernia with or without prenatal tracheal occlusion. Eur Radiol. (2013) 23:1299–305. doi: 10.1007/s00330-012-2709-6
14. Coleman A, Phithakwatchara N, Shaaban A, Keswani S, Kline-Fath B, Kingma P, et al. Fetal lung growth represented by longitudinal changes in MRI-derived fetal lung volume parameters predicts survival in isolated left-sided congenital diaphragmatic hernia. Prenat Diagn. (2015) 35:160–6. doi: 10.1002/pd.4510
15. Deprest JA, Benachi A, Gratacos E, Nicolaides KH, Berg C, Persico N, et al. Total trial for moderate hypoplasia investigators. Randomized trial of fetal surgery for moderate left diaphragmatic hernia. N Engl J Med. (2021) 385:119–29. doi: 10.1056/NEJMoa2026983
16. Deprest JA, Nicolaides KH, Benachi A, Gratacos E, Ryan G, Persico N, et al. Total trial for severe hypoplasia investigators. Randomized trial of fetal surgery for severe left diaphragmatic hernia. N Engl J Med. (2021) 385:107–18. doi: 10.1056/NEJMoa2027030
17. Hedrick HL, Danzer E, Merchant A, Bebbington MW, Zhao H, Flake AW, et al. Liver position and lung-to-head ratio for prediction of extracorporeal membrane oxygenation and survival in isolated left congenital diaphragmatic hernia. Am J Obstet Gynecol. (2007) 197:422–e1. doi: 10.1016/j.ajog.2007.07.001
18. Kitano Y, Nakagawa S, Kuroda T, Honna T, Itoh Y, Nakamura T, et al. Liver position in fetal congenital diaphragmatic hernia retains a prognostic value in the era of lung-protective strategy. J Pediatr Surg. (2005) 40:1827–32. doi: 10.1016/j.jpedsurg.2005.08.020
19. Albanese CT, Lopoo J, Goldstein RB, Filly RA, Feldstein VA, Calen PW, Jennings RW, Farrell JA, Harrison MR. Fetal liver position and perinatal outcome for congenital diaphragmatic hernia. Prenat Diagn Publ Aff Int Soc Prenat Diagn. (1998) 18:1138-42. doi: 10.1002/(sici)1097-0223(199811)18:11<1138::aid-pd416>3.0.co;2-a
20. Seetharamaiah R, Younger JG, Bartlett RH, Hirschl RB. Congenital diaphragmatic hernia study group. factors associated with survival in infants with congenital diaphragmatic hernia requiring extracorporeal membrane oxygenation: a report from the congenital diaphragmatic hernia study group. J Pediatr Surg. (2009) 44:1315–21. doi: 10.1016/j.jpedsurg.2008.12.021
21. Langwieler T, Fiegel HC, Alaamian M, Mann O, Beshir I, Izbicki JR, et al. The relationship of diaphragmatic defect, liver growth, and lung hypoplasia in nitrofen-induced congenital diaphragmatic hernia in the rat. Pediatr Surg Int. (2004) 20:509–14. doi: 10.1007/s00383-004-1226-3
22. Mullassery D. Ba'Ath ME, Jesudason EC, Losty PD. Value of liver herniation in prediction of outcome in fetal congenital diaphragmatic hernia: a systematic review and meta-analysis. Ultrasound Obstetr Gynecol. (2010) 35:609–14. doi: 10.1002/uog.7586
23. Kolbe AB, Ibirogba ER, Thomas KB, Hull NC, Thacker PG, Hathcock M, et al. Reproducibility of lung and liver volume measurements on fetal magnetic resonance imaging in left-sided congenital diaphragmatic hernia. Fetal Diagn Ther. (2021) 48:258–64. doi: 10.1159/000512491
24. Rypens F, Metens T, Rocourt N, Sonigo P, Brunelle F, Quere MP, et al. Fetal lung volume: estimation at MR imaging—initial results. Radiology. (2001) 219:236–41. doi: 10.1148/radiology.219.1.r01ap18236
25. Columbano A, Shinozuka H. Liver regeneration versus direct hyperplasia. FASEB J. (1996) 10:1118–28. doi: 10.1096/fasebj.10.10.8751714
26. Michalopoulos GK. Liver regeneration. Liver Biol Pathobiol. (2020) 12:566–84. doi: 10.1002/9781119436812.ch45
27. Andez AM, Amenta PS. The extracellular matrix in hepatic regeneration. FASEB J. (1995) 9:1401–10. doi: 10.1096/fasebj.9.14.7589981
28. Diehl AM, Rai RM. Regulation of signal transduction during liver regeneration. FASEB J. (1996) 10:215–27. doi: 10.1096/fasebj.10.2.8641555
29. Fausto N, Laird AD, Webber EM. Role of growth factors and cytokines in hepatic regeneration. FASEB J. (1995) 9:1527–36. doi: 10.1096/fasebj.9.15.8529831
30. Pelizzo G, Peiro JL, Villanacci V, Sbragia L, Oria M, De Silvestri A, Mazzon E, Calcaterra V. Liver pathological alterations in fetal rabbit model of congenital diaphragmatic hernia. Congenit Anom. (2022) 17:105–12. doi: 10.1111/cga.12462
31. Kluth D, Keijzer R, Hertl M, Tibboel D. “Embryology of congenital diaphragmatic hernia”. In Seminars in Pediatric Surgery, Vol. 5, Philadelphia, PA: WB Saunders Co. (1996). pp. 224–33.
32. Sherer DM, Eglinton GS, Gonçalves LE, Lewis KM, Queenan JT. Prenatal color and pulsed Doppler sonographic documentation of intrathoracic umbilical vein and ductus venosus, confirming extensive hepatic herniation in left congenital diaphragmatic hernia. Am J Perinatol. (1996) 13:159–62. doi: 10.1055/s-2007-994316
33. Strouse PJ, Di Pietro MA, Barr Jr M. Pitfall: anomalous umbilical vein and absent ductus venosus in association with right congenital diaphragmatic hernia. Pediatr Radiol. (1997) 27:651–3. doi: 10.1007/s002470050205
34. Pelizzo G, Calcaterra V, Lombardi C, et al. Fetal cardiac impairment in nitrofen-induced congenital diaphragmatic hernia: postmortem microcomputed tomography imaging study. Fetal Pediatr Pathol. (2017) 36:282–93. doi: 10.1080/15513815.2017.1315198
35. Pelizzo G, Bussani R, Zandonà L, et al. Cardiac adaptation to severe congenital diaphragmatic hernia. Fetal Pediatr Pathol. (2016) 35:10–20. doi: 10.3109/15513815.2015.1122125
36. Pelizzo G, Finazzo F, Vitaliti MS, Bellieni CV, Calcaterra V. Left-sided congenital diaphragmatic hernia and liver-up: time to revise simplistic views? J Matern Fetal Neonatal Med. (2019) 25:1–3. doi: 10.1080/14767058.2019.1702948
37. Kessler J, Rasmussen S, Godfrey K, Hanson M, Kiserud T. Venous liver blood flow and regulation of human fetal growth: evidence from macrosomic fetuses. Am J Obstet Gynecol. (2011) 204:429. doi: 10.1016/j.ajog.2010.12.038
38. Tchirikov M, Kertschanska S, Schroder HJ. Obstruction of ductus venosus stimulates cell proliferation in organs of fetal sheep. Placenta. (2001) 22:24–31. doi: 10.1053/plac.2000.0585
39. Tchirikov M, Kertschanska S, Sturenberg HJ, Schroder HJ. Liver blood perfusion as a possible instrument for fetal growth regulation. Placenta. (2002) 23:S153–8. doi: 10.1053/plac.2002.0810
40. Haugen G, Bollerslev J, Henriksen T. Human fetoplacental and fetal liver blood flow after maternal glucose loading: a cross-sectional observational study. Acta Obstet Gynecol Scand. (2014) 93:778–85. doi: 10.1111/aogs.12419
41. Bellotti M, Pennati G, De Gasperi C, Battaglia FC, Ferrazzi E. Role of ductus venosus in distribution of umbilical blood flow in human fetuses during second half of pregnancy. Am J Physiol Heart Circ Physiol. (2000) 279:H1256–63. doi: 10.1152/ajpheart.2000.279.3.H1256
42. Ali AF, Balaha MH, El Halwagy AE, El Namori MM. Fetal liver size, hepatic artery doppler study in late intra uterine growth restriction. J Adv Med Med Res. 33:167–76. doi: 10.9734/jammr/2021/v33i1731041
43. Adzick NS, Harrison MR, Glick PL, Nakayama DK, Manning FA. deLorimier AA. Diaphragmatic hernia in the fetus: prenatal diagnosis and outcome in 94 cases. J. Pediatr Surg. (1985) 20:357–61. doi: 10.1016/S0022-3468(85)80219-0
44. Areechon W, Reid L. Hypoplasia of lung with congenital diaphragmatic hernia. Br Med J. (1963) 1:230. doi: 10.1136/bmj.1.5325.230
45. Harrison MR, Bjordal RI, Langmark F, Knutrud O. Congenital diaphragmatic hernia: the hidden mortality. J Pediatr Surg. (1978) 13:227–30. doi: 10.1016/S0022-3468(78)80391-1
46. Lee TC, Lim FY, Keswani SG, Frischer JS, Haberman B, Kingma PS, et al. Late gestation fetal magnetic resonance imaging—derived total lung volume predicts postnatal survival and need for extracorporeal membrane oxygenation support in isolated congenital diaphragmatic hernia. J Pediatr Surg. (2011) 46:1165–71. doi: 10.1016/j.jpedsurg.2011.03.046
47. Jani J, Breysem L, Maes F, Boulvain M, Roubliova X, Lewi L, et al. Accuracy of magnetic resonance imaging for measuring fetal sheep lungs and other organs. Ultrasound Obstet Gynecol. (2005) 25:270–6. doi: 10.1002/uog.1866
48. Kluth D, Tenbrinck R, von Ekesparre M, Kangah R, Reich P, Brandsma A, et al. The natural history of congenital diaphragmatic hernia and pulmonary hypoplasia in the embryo. J Pediatr Surg. (1993) 28:456–63. doi: 10.1016/0022-3468(93)90248-J
Keywords: congenital diaphragm hernia, liver development, in utero imaging, liver growth, pulmonary hypoplasia, pulmonary hypertension
Citation: Ott KC, Bi M, Scorletti F, Ranginwala SA, Marriott WS, Peiro JL, Kline-Fath BM, Alhajjat AM and Shaaban AF (2022) The interplay between prenatal liver growth and lung development in congenital diaphragmatic hernia. Front. Pediatr. 10:983492. doi: 10.3389/fped.2022.983492
Received: 01 July 2022; Accepted: 07 September 2022;
Published: 26 September 2022.
Edited by:
Dick Tibboel, Erasmus Medical Center, NetherlandsReviewed by:
Irene Maria Borzani, IRCCS Ca 'Granda Foundation Maggiore Policlinico Hospital, ItalyThomas Schaible, University of Heidelberg, Germany
Copyright © 2022 Ott, Bi, Scorletti, Ranginwala, Marriott, Peiro, Kline-Fath, Alhajjat and Shaaban. This is an open-access article distributed under the terms of the Creative Commons Attribution License (CC BY). The use, distribution or reproduction in other forums is permitted, provided the original author(s) and the copyright owner(s) are credited and that the original publication in this journal is cited, in accordance with accepted academic practice. No use, distribution or reproduction is permitted which does not comply with these terms.
*Correspondence: Aimen F. Shaaban, ashaaban@luriechildrens.org