- 1Department of Medicine, Vanderbilt University Medical Center, Nashville, TN, United States
- 2Department of Biostatistics, Vanderbilt University Medical Center, Nashville, TN, United States
- 3Department of Statistics, University of Pittsburgh, Pittsburgh, PA, United States
- 4Department of Public Health Sciences, Henry Ford Health System, Detroit, MI, United States
- 5Department of Pediatrics, University of Wisconsin, Madison, WI, United States
- 6Department of Human Genetics, University of Chicago, Chicago, IL, United States
- 7Department of Medicine, University of California, San Francisco, CA, United States
- 8Department of Internal Medicine, Henry Ford Health System, Detroit, MI, United States
- 9Division of Asthma Research, Cincinnati Children's Hospital Medical Center, Cincinnati, OH, United States
- 10Department of Pediatrics, University of Cincinnati College of Medicine, Cincinnati, OH, United States
- 11Department of Medicine, Northwestern University, Chicago, IL, United States
- 12Department of Genetics and Metabolism, Children’s National Medical Center, Washington, DC, United States
- 13Department of Pediatrics, Vanderbilt University Medical Center, Nashville, TN, United States
Assessing the association of the newborn metabolic state with severity of subsequent respiratory tract infection may provide important insights on infection pathogenesis. In this multi-site birth cohort study, we identified newborn metabolites associated with lower respiratory tract infection (LRTI) in the first year of life in a discovery cohort and assessed for replication in two independent cohorts. Increased citrulline concentration was associated with decreased odds of LRTI (discovery cohort: aOR 0.83 [95% CI 0.70–0.99], p = 0.04; replication cohorts: aOR 0.58 [95% CI 0.28–1.22], p = 0.15). While our findings require further replication and investigation of mechanisms of action, they identify a novel target for LRTI prevention and treatment.
Introduction
Lower respiratory tract infections (LRTIs) are the leading cause of mortality in young children worldwide (1). While children with underlying conditions, such as prematurity, congenital heart disease, and chronic lung disease, are at highest risk of severe LRTI, the majority of these events, including hospitalizations, occur in previously healthy children (2, 3). In the first year of life, LRTIs are primarily caused by respiratory viral pathogens, most commonly respiratory syncytial virus (RSV) (4). With the exception of antiviral agents for influenza and SARS-CoV-2, most infections are not currently vaccine preventable and treatment is only supportive care (5). A very small subset of premature infants with significant pulmonary and cardiac conditions may qualify for RSV immunoprophylaxis (6). Despite the significant public health burden of LRTIs, effective treatment and prevention strategies are lacking for most infants (5).
Energy metabolism is a highly regulated process which plays an important role in susceptibility to viral infections (7). As viruses rely upon energy produced by host cell metabolism to replicate, metabolic pathways influence virulence (7, 8). Immune cells also depend upon nutrients produced through cellular metabolism to recognize and respond to pathogens (9). Immune responses are impaired in individuals with metabolic disorders, allowing viruses to further compromise the immune system and enhance metabolic dysfunction (10, 11). Therefore, assessing the association between the variability in normal metabolism and viral infection severity may provide important insights on LRTI pathogenesis, may aid in the identification of those at risk, and identify new pathways that may modify immune regulation that could be targeted for prevention and treatment. Our objective was to test the association of metabolites measured at birth with risk of LRTI during the first year of life using targeted metabolic data from newborn screening (NBS) programs.
Materials and methods
Study design, populations, and data collection
This multi-site cohort study included three birth cohorts from the NIH Environmental influences on Child Health Outcomes (ECHO) Children's Respiratory and Environmental Workgroup (CREW) consortium (12). We utilized a population-based birth cohort which was the largest of the three cohorts (INSPIRE, n = 1949) for the discovery phase and the smaller cohorts (MAAP, n = 141; WISC, n = 270) to replicate findings. We included enrolled infants with at least one year of follow-up whom we linked with NBS blood metabolic data, including targeted measurement of amino acids, free carnitine, and acylcarnitines. Each state's public health department is responsible for deciding which conditions, and corresponding metabolites, are included on the NBS panel based on evidence of net benefit of screening, availability of effective treatments, and screening capability of the state. Most states screen for the panel of conditions recommended by the U.S. Health Resources / Services Administration, using the same methodology (13), while some states additionally screen for newer conditions (14, 15).
Our primary exposures were metabolite concentrations at birth. Metabolic data for newborns were obtained from the NBS programs at the Tennessee Department of Health, Michigan Department of Health and Human Services, and Wisconsin State Laboratory of Hygiene. Data were provided for infants who did not screen positive for any inherited disorder [i.e., metabolite concentrations were within the normal range, representing >99% of infants in the US (16)] to reduce the risk of potential participant identification and remove skewed metabolite profiles due to inborn errors of metabolism. These data were then linked with demographic and clinical data from each of the cohorts. Metabolites measured in each cohort are listed in Supplementary Table S1. Our primary outcome, LRTI, was ascertained by parental report, physician diagnosis, or medical record documentation of bronchiolitis or pneumonia at any time during the first year of life and defined at age one-year dichotomously as LRTI yes or no. Data collection for ascertainment of the primary outcome within each cohort is summarized in Supplementary Table S2. Demographic and clinical characteristics were ascertained from questionnaires administered during the first year of life.
Patient consent statement
The protocol and informed consent documents were approved by the Vanderbilt University Medical Center, Henry Ford Health System, University of Wisconsin, Tennessee Department of Health, Michigan Department of Health and Human Services, and Wisconsin Department of Health Services Institutional Review Boards. Written informed consent or parent's/guardian's permission was obtained, along with child assent as appropriate, for CREW participation and for participation in specific cohorts.
Statistical analysis
We compared demographic and clinical characteristics between the cohorts using Kruskal-Wallis or Pearson χ2 test, as appropriate. Our a priori statistical plan utilized a common, pre-specified two-stage procedure (17) to identify LRTI-related metabolites in the discovery cohort (Supplementary Figure S1). In the first stage, we used elastic net to identify leading metabolites associated with LRTI in the first year of life. Elastic net is a penalized regression method for selecting groups of correlated metabolites while performing variable selection and continuous shrinkage (18). We included all metabolites in one logistic regression model, which helped reduce the multiple metabolite testing burden. Although there was some skewness and zero values, metabolite concentrations were generally well distributed (normally) (Supplementary Figure S2). As having normal distributions is not an assumption of logistic regression, we utilized raw, untransformed metabolite concentrations in the analyses.
In the second stage, we evaluated the selected metabolites in a multivariable logistic regression model simultaneously, while adjusting for a priori selected demographic and clinical characteristics previously shown to be associated with metabolism or infant LRTI. We calculated each metabolite's adjusted odds ratio for an interquartile range [IQR] difference increase in its concentration. For each metabolite that remained significantly associated with LRTI after the second stage (p < 0.05), we tested its association with LRTI in the replication cohorts using meta-analyzed logistic regression. In the replication analysis, our regression power was limited by sparse LRTI events. To avoid overfitting, we decided a priori to limit covariate adjustment to infant sex (estimated power in replication cohorts: 6%–25%; see Supplementary Material for details on power calculation).
To provide additional insight into pathways involved in severity of RSV infection, we performed a sub-analysis assessing the association between each metabolite identified in the primary analysis and severity of RSV infection. We performed this analysis among a subset of infants in the discovery cohort with either RSV upper respiratory tract infection (URTI, less severe) or RSV LRTI (more severe) identified through biweekly surveillance during RSV season and serology at one year. We used multivariable logistic regression, adjusting for the same covariates included in stage two of the primary analysis, to assess the association. Data analyses were performed using R software, versions 3.6.1 and 4.0.4 (R Foundation for Statistical Computing, Vienna, Austria). Additional information on methodology can be found in the Supplementary Material.
Results
Our final study populations included 1,746 (INSPIRE, discovery cohort), 134 (MAAP, replication cohort), and 222 (WISC, replication cohort) infants after linking NBS metabolic data to >90% of infants (Figure 1). Demographic and clinical characteristics and NBS metabolite concentrations for the study populations are shown in Table 1 and Supplementary Table S3.
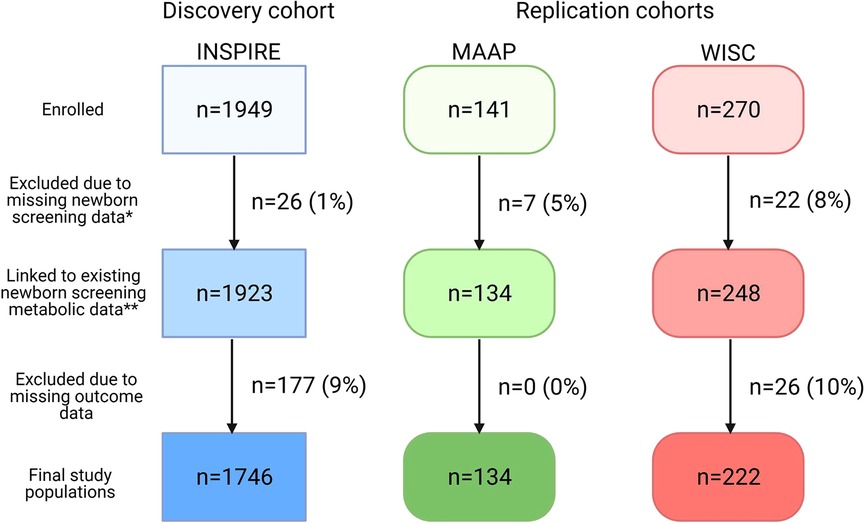
Figure 1. Flow diagram of study populations. Discovery cohort: INSPIRE, Infant Susceptibility to Pulmonary Infections and Asthma following RSV Exposure; Replication cohorts: MAAP, Microbes, Allergy, Asthma and Pets study and WISC, Wisconsin Infant Study Cohort. *Newborn screening data may have been missing due to refusal of newborn screening, metabolite concentrations outside the normal range, or incomplete linkage. **The study population included in the RSV sub-analysis (n = 912 INSPIRE infants) stemmed from this box. This figure was created with BioRender.com.
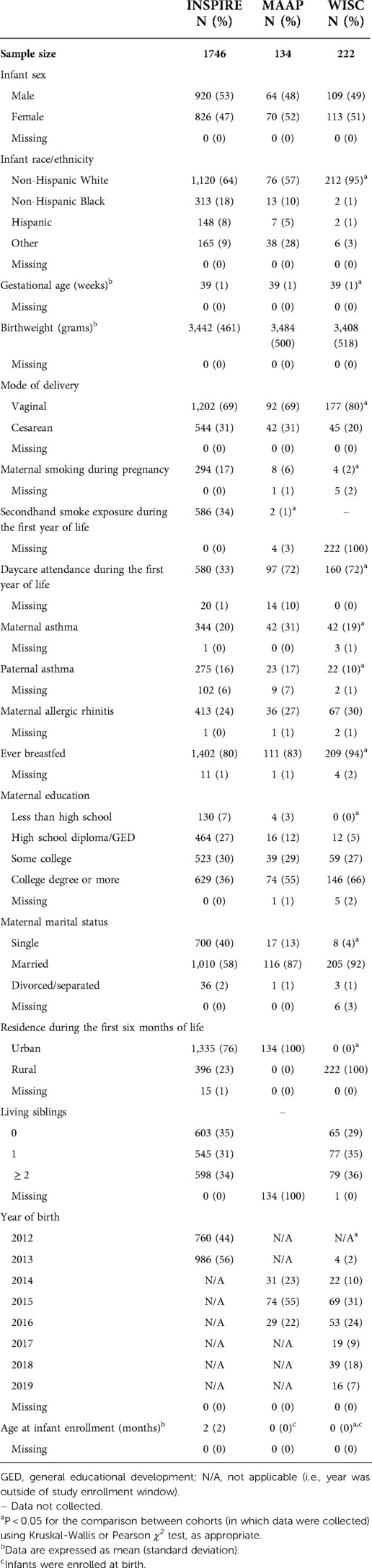
Table 1. Demographic and clinical characteristics of the study populations with linked newborn screening data and non-missing outcome data.
During the first year of life, 25%, 5%, and 3% of INSPIRE, MAAP, and WISC infants reported having an LRTI, respectively. The relationships between the identified set of 15 leading metabolites and covariates and the log odds of LRTI in infancy and the pairwise correlations between the leading metabolites are depicted in Supplementary Figure S3, Supplementary Table S4, and Supplementary Figure S4. While several metabolites were moderately associated with LRTI in infancy in adjusted analyses (C2, C3, C6-DC, and SUAC; p < 0.1), citrulline was the only statistically significant metabolite (Supplementary Figure S5). A 5 umol/l (IQR) increase of citrulline concentration at birth was associated with decreased odds of LRTI in infancy in the discovery cohort (adjusted odds ratio [aOR] 0.83 [95% confidence interval (CI) 0.70–0.99], p = 0.04) (Figure 2, panel A). When assessed in the replication cohorts, the meta-analyzed effect also showed an inverse relationship but was not statistically significant [aOR 0.58 (95% CI 0.28–1.22), p = 0.15] (Supplementary Table S5).
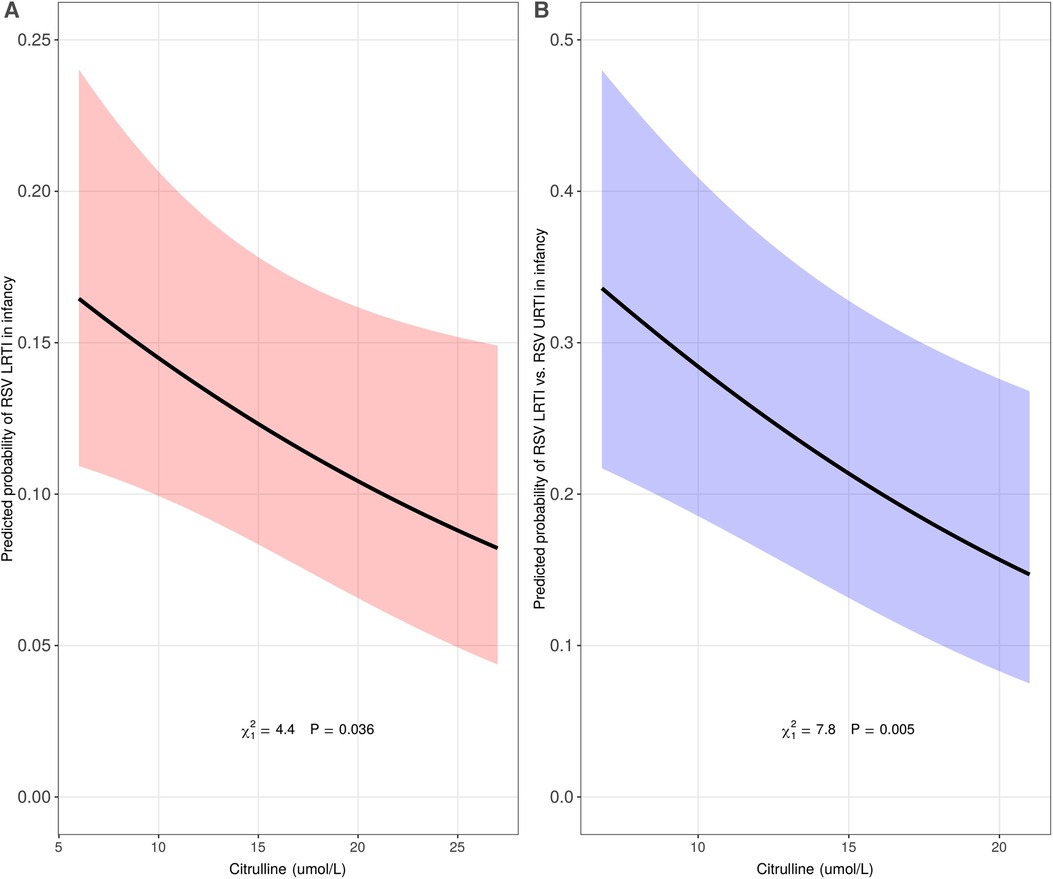
Figure 2. Association of citrulline concentration at birth with lower respiratory tract infection (LRTI) of all etiologies (panel A, n = 1746) and respiratory syncytial virus (RSV) LRTI (panel B, n = 912) in infancy in the discovery cohort. The predicted probability (y-axis) in panel A was calculated using a multivariable logistic regression model in the discovery cohort, INSPIRE, adjusting for infant sex, infant race/ethnicity, mode of delivery, maternal smoking during pregnancy, ever breastfed, maternal asthma, living siblings, daycare attendance during the first year of life, and all other identified metabolites (C2, C3, C5, C5:1, C6-DC, C10:1, C10:2, C16, C18:2, ASA, GLY, ORN, SUAC, and VAL). The predicted probability (y-axis) in panel B was calculated using a multivariable logistic regression model in a subset of infants in INSPIRE with either RSV upper respiratory tract infection (URTI, less severe) or RSV LRTI (more severe), adjusting for infant sex, infant race/ethnicity, mode of delivery, maternal smoking during pregnancy, ever breastfed, maternal asthma, living siblings, and daycare attendance during the first year of life.
In the analysis restricted to INSPIRE infants who were infected with RSV during infancy [n = 912 (176 with RSV LRTI, 736 with RSV URTI)], we consistently observed an inverse relationship between citrulline concentration at birth and odds of LRTI in infancy [aOR 0.74 (95% CI 0.60–0.91), p = 0.005] (Figure 2, panel B).
Discussion
We observed a protective association of citrulline concentration at birth on risk of LRTI in infancy, where increased concentration (within the normal range) was associated with decreased risk. This association persisted when assessed among a subset of infants who were infected with RSV during the first year of life, identifying novel metabolic pathways in early life that may be involved in susceptibility to severe respiratory viral infection, including RSV infection.
Energy metabolism is highly regulated to meet the demands of the cell. Alterations in metabolic pathways have been linked to cell death and dysfunction, reactive oxygen species production, altered immune response, and enhanced inflammation (19). Severe forms of metabolic dysregulation (i.e., inborn errors of metabolism) are identified at birth through NBS. These disorders are caused by genetic mutations leading to defective enzymes, cofactors, or transporters in metabolic pathways, often resulting in toxic accumulation of metabolite intermediates (20, 21). Respiratory manifestations, particularly airway infections, are common among children with inherited metabolic diseases (22), indicating that dysregulation of energy metabolism may predispose children to respiratory illness. Milder forms of metabolic dysregulation have also been implicated in susceptibility to pulmonary infections through impaired immune responses and increased airway inflammation (23). For this reason, we assessed the association between the variability in normal infant metabolism and viral infection severity to provide novel insights on LRTI pathogenesis, to aid in the identification of those at risk, and identify new pathways that may modify immune regulation that could be targeted for prevention and treatment.
LRTIs are a major public health issue (1), and with the exception of antiviral agents for influenza and SARS-CoV-2, the only available infant treatment is supportive care (5). RSV is the most common respiratory viral etiology of severe LRTIs (4). RSV prevention products (i.e., RSV immunoprophylaxis) are currently primarily available in high income countries for a small subset of high-risk infants (24). However, most infant RSV-related deaths occur in low and middle income countries (25), and over 70% of RSV-related hospitalizations occur in term infants without known comorbidities (26). There are no maternal or infant RSV vaccines currently approved, although many are in clinical trials (27, 28). Citrulline is a readily available dietary supplement and supplementation has been shown to be both safe and well tolerated in infants (29). Although citrulline is primarily used for treating urea cycle defects, there is accumulating evidence to suggest that citrulline supplementation may have a wider therapeutic role (30), particularly for lung diseases (31). Our findings may have important clinical application and provide sufficient evidence to further understand the protective effect of citrulline on LRTI in experimental models. While we focused on citrulline (per our a priori statistical plan), the metabolites C2, C3, C6-DC, and SUAC showed modest associations with LRTI, and therefore, may also be of interest in future studies.
The role of citrulline in the regulation of immune function is increasingly being recognized. Citrulline is a nutritionally non-essential amino acid which plays an important role in arginine biosynthesis, the nitric oxide cycle, and the urea cycle (32). The metabolism of citrulline is unique in that it is not used by the intestine or taken up by the liver and, thus, bypasses splanchnic extraction (33). Impaired conversion of citrulline to arginine in argininosuccinate synthetase deficiency has been shown to result in impaired immunity, increased infection susceptibility, and decreased nitric oxide production in experimental models (34–36). Children who are critically ill have low plasma citrulline concentration, which is strongly correlated with severity of inflammation (37). L-citrulline supplementation has been shown to enhance T-cell function by promoting IL-10 and TGF-β1 production in infant rats (36). Citrulline and Lactobacillus probiotic supplementation have been found to have a synergistic, protective effect against pathogen adhesion (i.e., the first step of pathogen infection) in the intestinal tract, and, thus, may be beneficial for improving immunity (38). Citrulline-generating and utilizing enzymes have also been reported to increase in lung myeloid populations during infection, enhancing host defense (39). It is plausible that citrulline's role in the development of the host immune response is one pathway through which increased citrulline concentration at birth could protect against risk of LRTI in infancy. While citrulline is a precursor for arginine, we did not observe a significant association between arginine and LRTI in infancy. This may be due to the distinct role of citrulline in immune modulation and nitric oxide synthesis (36, 40).
Our study has many strengths, including our use of routinely collected and uniformly measured newborn metabolic data and birth cohorts in the ECHO-CREW consortium, including a population-based birth cohort of term, healthy infants for discovery and two additional study cohorts for replication. Although our replication analyses were not statistically significant, they were very small in comparison with the discovery cohort. Despite this, we demonstrated consistent effect sizes and directionality in independent cohorts from diverse populations, which increases the validity of our findings. As our replication cohorts were small, the non-significant finding may be due to low power.
There are some limitations to our study. Replication cohorts were challenging to find, and LRTI was not uniformly ascertained among the three study populations, which likely accounted for the large difference in LRTI incidence. As the INSPIRE cohort was designed to include surveillance for acute respiratory illnesses and respiratory tract infections during infancy, the incidence of LRTI in this population was similar to previously reported population-based estimates (41). LRTI was likely rare among infants in our two replication cohorts because there was no protocol to track these illnesses. However, this would result in misclassification among those considered as having no LRTI and would drive findings towards the null, which suggests our replication results are conservative. Additionally, while the discovery cohort was a population-based birth cohort representative of the region from which participants were recruited, overall, the cohorts included in this study were primarily of non-Hispanic ethnicity, which may limit the generalizability of our findings. As the etiologic viral agent of acute respiratory illnesses was not captured in our replication cohorts, we were unable to replicate the association between citrulline concentration at birth and LRTI during the first year of life among infants with RSV infection in these cohorts.
Conclusions
We identified a protective association of newborn citrulline concentration on risk of LRTI during the first year of life. Our findings allow us to speculate on this amino acid's mechanistic role and importance during pregnancy or early infancy on later LRTI risk and identify an available novel target for LRTI prevention and possibly treatment, where none currently exist for most infants. These data provide support for further investigation of the mechanisms underlying this relationship with a goal toward clinical translation.
Data availability statement
The data analyzed in this study is subject to the following licenses/restrictions: The dataset includes confidential newborn screening data that cannot be shared without individual approvals from the Michigan Department of Health and Human Services, Tennessee Department of Health, and Wisconsin Laboratory of Hygiene Institutional Review Boards. These data were linked to potentially identifying and sensitive patient information of participants in 3 CREW birth cohorts. Access to confidential CREW data requires written authorization from the CREW study sponsor, The National Institutes of Health Environmental Influences on Child Health Outcomes Program, and a data request submitted to the CREW PI. Requests to access these datasets should be directed to: Tina Hartert, tina.hartert@vumc.org.
Ethics statement
The studies involving human participants were reviewed and approved by Vanderbilt University Medical Center, Henry Ford Health System, University of Wisconsin, Tennessee Department of Health, Michigan Department of Health and Human Services, and Wisconsin Department of Health Services Institutional Review Boards. Written informed consent to participate in this study was provided by the participants’ legal guardian/next of kin.
Author contributions
BS, TG, KT, CM, and TH contributed to conception and design of the study. BS, TG, and GC contributed to data curation. TG performed the statistical analysis. BS wrote the first draft of the manuscript. All authors contributed to the article and approved the submitted version.
Funding
This work was supported by the National Institutes of Health (grant numbers UG3/UH3 OD023282 and U19 AI104317 to JEG, UH3 OD023282 and U19 AI095227 to TVH, P01 AI089473 to EMZ, and K01 HL161257 which supports BMS) (https://www.nih.gov/). The content is solely the responsibility of the authors and does not necessarily represent the official views of the National Institutes of Health. The funders had no role in study design, data collection and analysis, decision to publish, or preparation of the manuscript.
Acknowledgments
We are indebted to Yinmei Li (Tennessee Department of Health), Chris Dorley (Tennessee Department of Health), Isabel Hurden (Michigan Department of Health and Human Services), Mary Kleyn (Michigan Department of Health and Human Services), Mary Seeterlin (Michigan Department of Health and Human Services), Mei Baker (Wisconsin State Laboratory of Hygiene), and Sam Dawe (Wisconsin State Laboratory of Hygiene) for their assistance in this investigation.
CREW Cohorts
INSPIRE: Infant Susceptibility to Pulmonary Infections and Asthma Following RSV
Exposure
Tina V. Hartert*, Vanderbilt University Medical Center, Nashville, TN
Donald H. Arnold, Vanderbilt University Medical Center, Nashville, TN
Suman Das, Vanderbilt University Medical Center, Nashville, TN
William Dupont, Vanderbilt University Medical Center, Nashville, TN
Tebeb Gebretsadik, Vanderbilt University Medical Center, Nashville, TN
R. Stokes Peebles, Vanderbilt University Medical Center, Nashville, TN
Christian Rosas-Salazar, Vanderbilt University Medical Center, Nashville, TN
Brittney Snyder, Vanderbilt University Medical Center, Nashville, TN
Kedir Turi, Vanderbilt University Medical Center, Nashville, TN
Martin Moore, Emory University, Atlanta, GA
Larry Anderson, Emory University, Atlanta, GA
Tania Chirkova, Emory University, Atlanta, GA
Teresa M. Chipps, Vanderbilt University Medical Center, Nashville, TN
Wais Folad, Vanderbilt University Medical Center, Nashville, TN
Jessica Levine, Vanderbilt University Medical Center, Nashville, TN
Zhouwen Liu, Vanderbilt University Medical Center, Nashville, TN
Christopher Sutton, Vanderbilt University Medical Center, Nashville, TN
Loren Kreider, Vanderbilt University Medical Center, Nashville, TN
Sara Reiss, Vanderbilt University Medical Center, Nashville, TN
Derek Wiggins, Vanderbilt University Medical Center, Nashville, TN
Kadijah Poleon, Vanderbilt University Medical Center, Nashville, TN
Lisa Martin, Vanderbilt University Medical Center, Nashville, TN
Patty Russell, Vanderbilt University Medical Center, Nashville, TN
Talissa Blackshere, Vanderbilt University Medical Center, Nashville, TN
Gretchen Walter, Vanderbilt University Medical Center, Nashville, TN
Kayla Goodman, Vanderbilt University Medical Center, Nashville, TN
Christina Vietmeyer, Vanderbilt University Medical Center, Nashville, TN
Nina Rohrig, Vanderbilt University Medical Center, Nashville, TN
MAAP: Microbes, Allergy, Asthma and Pets
Edward M. Zoratti*, Henry Ford Health System, Detroit, MI
Christine C. Johnson*, Henry Ford Health System, Detroit, MI
Stacey Bellemore, Henry Ford Health System, Detroit, MI
Kevin Bobbitt, Henry Ford Health System, Detroit, MI
Homer Boushey, University of California, San Francisco, CA
Brent Davidson, Henry Ford Health System, Detroit, MI
Salvatore Finazzo, Henry Ford Health System, Detroit, MI
Suzanne Havstad, Henry Ford Health System, Detroit, MI
Kyra Jones, Henry Ford Health System, Detroit, MI
Albert M. Levin, Henry Ford Health System, Detroit, MI
Nicholas Lukacs, University of Michigan, Ann Arbor, MI
Susan Lynch, University of California, San Francisco, CA
Erik Mann, Henry Ford Health System, Detroit, MI
Dennis Ownby, Augusta University, Augusta, GA
Alexandra Sitarik, Henry Ford Health System, Detroit, MI
Anthony Wahlman, Henry Ford Health System, Detroit, MI
Ganesa Wegienka, Henry Ford Health System, Detroit, MI
Kimberley Woodcroft, Henry Ford Health System, Detroit, MI
Shirley Zhang, Henry Ford Health System, Detroit, MI
WISC: Wisconsin Infant Study Cohort
Christine M. Seroogy*, University of Wisconsin-Madison, Madison, WI
James E. Gern*, University of Wisconsin-Madison, Madison, WI
Elizabeth Armagost, Marshfield Clinic Research Institute, Marshfield, WI
Kathrine L. Barnes, Marshfield Clinic Research Institute, Marshfield, WI
Casper G. Bendixsen, Marshfield Clinic Research Institute, Marshfield, WI
Yury Bochkov, University of Wisconsin-Madison, Madison, WI
Deborah Chasman, University of Wisconsin-Madison, Madison, WI
Deanna Cole, Marshfield Clinic Research Institute, Marshfield, WI
Erin Donnerbauer, Marshfield Clinic Research Institute, Marshfield, WI
Amy Dresen, University of Wisconsin-Madison, Madison, WI
Michael D. Evans, University of Wisconsin-Madison, Madison, WI
Terry Foss, Marshfield Clinic Research Institute, Marshfield, WI
Heather Floerke, University of Wisconsin-Madison, Madison, WI
Wayne Frome, Marshfield Clinic Research Institute, Marshfield, WI
Samantha Fye, University of Wisconsin-Madison, Madison, WI
Ronald E. Gangnon, University of Wisconsin-Madison, Madison, WI
Kristine Grindle, University of Wisconsin-Madison, Madison, WI
Tara Johnson, Marshfield Clinic Research Institute, Marshfield, WI
Matthew C. Keifer, Marshfield Clinic Research Institute, Marshfield, WI
Tammy Kronenwetter Koepel, Marshfield Clinic Research Institute, Marshfield, WI
Jennifer Meece, Marshfield Clinic Research Institute, Marshfield, WI
Vicki Moon, Marshfield Clinic Research Institute, Marshfield, WI
Brent F. Olson, Marshfield Clinic Research Institute, Marshfield, WI
Irene Ong, University of Wisconsin-Madison, Madison, WI
Tressa Pappas, University of Wisconsin-Madison, Madison, WI
Steve Sigelman, National Institute of Allergy and Infectious Diseases, Bethesda, MD
Jeffrey J. VanWormer, Marshfield Clinic Research Institute, Marshfield, WI
Rose Vrtis, University of Wisconsin-Madison, Madison, WI
CREW Science Centers and Cores
Administrative Center
James E. Gern*, University of Wisconsin, Madison, WI
Chris M. Reyes, University of Wisconsin, Madison, WI
Gina Crisafi, University of Wisconsin, Madison, WI
Melissa Yaeger, University of Wisconsin, Madison, WI
Dorothy Floerke, University of Wisconsin, Madison, WI
Rick Kelley, University of Wisconsin, Madison, WI
Biomedical Informatics and Biostatistical Core
Umberto Tachinardi*, University of Wisconsin, Madison, WI
Mark Craven*, University of Wisconsin, Madison, WI
Eneida Mendonca, University of Wisconsin, Madison, WI
Lisa Gress, University of Wisconsin, Madison, WI
Laura Ladick, University of Wisconsin, Madison, WI
Adam Nunez, University of Wisconsin, Madison, WI
Coordinating Center
Cynthia M. Visness*, Rho, Inc, Durham, NC
Rachel Lisi, Rho, Inc, Durham, NC
Caitlin Suddeuth, Rho, Inc, Durham, NC
Jena Tate, Rho, Inc, Durham, NC
*Principal Investigator
Conflict of interest
The authors declare that the research was conducted in the absence of any commercial or financial relationships that could be construed as a potential conflict of interest.
Publisher's note
All claims expressed in this article are solely those of the authors and do not necessarily represent those of their affiliated organizations, or those of the publisher, the editors and the reviewers. Any product that may be evaluated in this article, or claim that may be made by its manufacturer, is not guaranteed or endorsed by the publisher.
Supplementary material
The Supplementary Material for this article can be found online at: https://www.frontiersin.org/articles/10.3389/fped.2022.979777/full#supplementary-material.
References
1. GBD 2016 Lower Respiratory Infections Collaborators. Estimates of the global, regional, and national morbidity, mortality, and aetiologies of lower respiratory infections in 195 countries, 1990–2016: a systematic analysis for the global burden of disease study 2016. Lancet Infect Dis. (2018) 18(11):1191–210. doi: 10.1016/s1473-3099(18)30310-4
2. Scheltema NM, Gentile A, Lucion F, Nokes DJ, Munywoki PK, Madhi SA, et al. Global respiratory syncytial virus-associated mortality in young children (rsv gold): a retrospective case series. Lancet Glob Health. (2017) 5(10):e984–e91. doi: 10.1016/S2214-109X(17)30344-3
3. Hall CB, Weinberg GA, Blumkin AK, Edwards KM, Staat MA, Schultz AF, et al. Respiratory syncytial virus–associated hospitalizations among children less than 24 months of age. Pediatrics. (2013) 132(2):e341–e8. doi: 10.1542/peds.2013-0303
4. Piedra PA, Stark AR. Bronchiolitis in Infants and Children: Clinical Features and Diagnosis. In Uptodate. Waltham, Ma: Uptodate. Available at: https://www.uptodate.com/contents/bronchiolitis-in-infants-and-children-clinical-features-and-diagnosis/print (Accessed September 12, 2022).
5. Piedra PA, Stark AR. Bronchiolitis in Infants and Children: Treatment, Outcome, and Prevention. (Accessed August 31, 2022. In: UpToDate. Waltham, MA: UpToDate. Available from: https://www.uptodate.com/contents/bronchiolitis-in-infants-and-children-treatment-outcome-and-prevention#H162663605
6. COMMITTEE ON INFECTIOUS DISEASES AND BRONCHIOLITIS GUIDELINES COMMITTEE, Brady MT, Byington CL, Davies HD, Edwards KM, Jackson MA, Maldonado YA, et al. Updated guidance for palivizumab prophylaxis among infants and young children at increased risk of hospitalization for respiratory syncytial virus infection. Pediatrics. (2014) 134(2):415–20. doi: 10.1542/peds.2014-1665
7. Eisenreich W, Rudel T, Heesemann J, Goebel W. How viral and intracellular bacterial pathogens reprogram the metabolism of host cells to allow their intracellular replication. Front Cell Infect Microbiol. (2019) 9:42. doi: 10.3389/fcimb.2019.00042
8. Mayer KA, Stöckl J, Zlabinger GJ, Gualdoni GA. Hijacking the supplies: metabolism as a novel facet of virus-host interaction. Front Immunol. (2019) 10:1533. doi: 10.3389/fimmu.2019.01533
9. Ganeshan K, Chawla A. Metabolic regulation of immune responses. Annu Rev Immunol. (2014) 32:609–34. doi: 10.1146/annurev-immunol-032713-120236
10. Honce R, Schultz-Cherry S. Impact of obesity on influenza a virus pathogenesis, immune response, and evolution. Front Immunol. (2019) 10:1071. doi: 10.3389/fimmu.2019.01071
11. Smith M, Honce R, Schultz-Cherry S. Metabolic syndrome and viral pathogenesis: lessons from influenza and coronaviruses. J Virol. (2020) 94(18):e00665-20. doi: 10.1128/jvi.00665-20. PMID 32661141
12. Gern JE, Jackson DJ, Lemanske RF Jr, Seroogy CM, Tachinardi U, Craven M, et al. The children's respiratory and environmental workgroup (crew) birth cohort consortium: design, methods, and study population. Respir Res. (2019) 20(1):115. doi: 10.1186/s12931-019-1088-9
13. CLSI. Newborn screening by tandem mass spectrometry. 2nd ed. CLSI Guideline NBS04. Wayne, Pa: Clinical and Laboratory Standards Institute (2017).
14. Health Resources / Services Administration. Recommended Uniform Screening Panel. Available at: https://www.hrsa.gov/advisory-committees/heritable-disorders/rusp.html (Accessed August 31, 2022).
15. Baby's First Test. Available at: https://www.babysfirsttest.org/ (Accessed August 29, 2022).
16. Feuchtbaum L, Carter J, Dowray S, Currier RJ, Lorey F. Birth prevalence of disorders detectable through newborn screening by race/ethnicity. Genet Med. (2012) 14(11):937–45. doi: 10.1038/gim.2012.76
17. van de Geer S, Bühlmann P, Zhou S. The adaptive and the thresholded lasso for potentially misspecified models (and a lower bound for the lasso). Electron J Stat. (2011) 5(none):688–749. 62. doi: 10.1214/11-EJS624
18. Zou H, Hastie T. Regularization and Variable selection via the elastic net. J R Stat Soc Series B Stat Methodol. (2005) 67(2):301–20. doi: 10.1111/j.1467-9868.2005.00503.x
19. Liu G, Summer R. Cellular metabolism in lung health and disease. Annu Rev Physiol. (2019) 81:403–28. doi: 10.1146/annurev-physiol-020518-114640
20. Mak CM, Lee HC, Chan AY, Lam CW. Inborn errors of metabolism and expanded newborn screening: review and update. Crit Rev Clin Lab Sci. (2013) 50(6):142–62. doi: 10.3109/10408363.2013.847896
21. National Institutes of Health (NIH). About Inborn Errors of Metabolism. Available at: https://www.genome.gov/genetic-disorders/inborn-errors-of-metabolism (Accessed August 20, 2022).
22. Santamaria F, Montella S, Mirra V, De Stefano S, Andria G, Parenti G. Respiratory manifestations in patients with inherited metabolic diseases. Eur Respir Rev. (2013) 22(130):437. doi: 10.1183/09059180.00008012
23. Rao M, Dodoo E, Zumla A, Maeurer M. Immunometabolism and pulmonary infections: implications for protective immune responses and host-directed therapies. Front Microbiol. (2019) 10:962. doi: 10.3389/fmicb.2019.00962
24. Hodgson D, Pebody R, Panovska-Griffiths J, Baguelin M, Atkins KE. Evaluating the next generation of rsv intervention strategies: a mathematical modelling study and cost-effectiveness analysis. BMC Med. (2020) 18(1):348. doi: 10.1186/s12916-020-01802-8
25. Li Y, Wang X, Blau DM, Caballero MT, Feikin DR, Gill CJ, et al. Global, regional, and national disease burden estimates of acute lower respiratory infections due to respiratory syncytial virus in children younger than 5 years in 2019: a systematic analysis. Lancet (London, England). (2022) 399(10340):2047–64. doi: 10.1016/s0140-6736(22)00478-0
26. Gantenberg JR, van Aalst R, Zimmerman N, Limone B, Chaves SS, La Via WV, et al. Medically attended illness due to respiratory syncytial virus infection among infants born in the United States between 2016 and 2020. J Infect Dis. (2022) 226(Supplement_2):S164–S74. doi: 10.1093/infdis/jiac185
27. Srikantiah P, Vora P, Klugman KP. Assessing the full burden of respiratory syncytial virus in young infants in low- and middle-income countries: the importance of community mortality studies. Clin Infect Dis. (2021) 73(Suppl_3):S177–s9. doi: 10.1093/cid/ciab486
28. Ginsburg AS, Srikantiah P. Respiratory syncytial virus: promising progress against a leading cause of pneumonia. Lancet Glob Health. (2021) 9(12):e1644–e5. doi: 10.1016/s2214-109x(21)00455-1
29. Barr FE, Tirona RG, Taylor MB, Rice G, Arnold J, Cunningham G, et al. Pharmacokinetics and safety of intravenously administered citrulline in children undergoing congenital heart surgery: potential therapy for postoperative pulmonary hypertension. J Thorac Cardiovasc Surg. (2007) 134(2):319–26. doi: 10.1016/j.jtcvs.2007.02.043
30. Papadia C, Osowska S, Cynober L, Forbes A. Citrulline in health and disease. Review on human studies. Clin Nutr. (2018) 37(6, Part A):1823–8. doi: 10.1016/j.clnu.2017.10.009
31. Scott JA, Maarsingh H, Holguin F, Grasemann H. Arginine therapy for lung diseases. Front Pharmacol. (2021) 12:627503. doi: 10.3389/fphar.2021.627503
32. Aguayo E, Martínez-Sánchez A, Fernández-Lobato B, Alacid F. L-Citrulline: a non-essential amino acid with important roles in human health. Applied Sciences. (2021) 11(7):3293. doi: 10.3390/app11073293
33. Bahri S, Zerrouk N, Aussel C, Moinard C, Crenn P, Curis E, et al. Citrulline: from metabolism to therapeutic use. Nutrition. (2013) 29(3):479–84. doi: 10.1016/j.nut.2012.07.002
34. Qualls JE, Subramanian C, Rafi W, Smith AM, Balouzian L, DeFreitas AA, et al. Sustained generation of nitric oxide and control of mycobacterial infection requires argininosuccinate synthase 1. Cell Host Microbe. (2012) 12(3):313–23. doi: 10.1016/j.chom.2012.07.012
35. Tarasenko TN, Gomez-Rodriguez J, McGuire PJ. Impaired T cell function in argininosuccinate synthetase deficiency. J Leukoc Biol. (2015) 97(2):273–8. doi: 10.1189/jlb.1AB0714-365R
36. Lee Y-C, Su Y-T, Liu T-Y, Tsai C-M, Chang C-H, Yu H-R. L-Arginine and L-citrulline supplementation have different programming effect on regulatory T-cells function of infantile rats. Front Immunol. (2018) 9:2911. doi: 10.3389/fimmu.2018.02911
37. van Waardenburg DA, de Betue CT, Luiking YC, Engel M, Deutz NE. Plasma arginine and citrulline concentrations in critically ill children: strong relation with inflammation. Am J Clin Nutr. (2007) 86(5):1438–44. doi: 10.1093/ajcn/86.5.1438
38. Ho SW, El-Nezami H, Shah NP. Effects of supplementation of citrulline and Lactobacillus Helveticus ascc 511 on intestinal epithelial cell integrity. J Funct Foods. (2020) 64:103571. doi: 10.1016/j.jff.2019.103571
39. Lange SM, McKell MC, Schmidt SM, Zhao J, Crowther RR, Green LC, et al. L-Arginine synthesis from L-citrulline in myeloid cells drives host defense against mycobacteria in vivo. J Immunol. (2019) 202(6):1747–54. doi: 10.4049/jimmunol.1801569
40. Ware LB, Magarik JA, Wickersham N, Cunningham G, Rice TW, Christman BW, et al. Low plasma citrulline levels are associated with acute respiratory distress syndrome in patients with severe sepsis. Crit Care (London, England). (2013) 17(1):R10. doi: 10.1186/cc11934
Keywords: lower respiratory tract infection, infancy, newborn screening (NBS), metabolites, citrulline
Citation: Snyder BM, Gebretsadik T, Turi KN, McKennan C, Havstad S, Jackson DJ, Ober C, Lynch S, McCauley K, Seroogy CM, Zoratti EM, Khurana Hershey GK, Berdnikovs S, Cunningham G, Summar ML, Gern JE, Hartert TV and on behalf of the ECHO-CREW investigators (2022) Association of citrulline concentration at birth with lower respiratory tract infection in infancy: Findings from a multi-site birth cohort study. Front. Pediatr. 10:979777. doi: 10.3389/fped.2022.979777
Received: 27 June 2022; Accepted: 28 September 2022;
Published: 17 October 2022.
Edited by:
Juan Manuel Mejia-Arangure, Universidad Nacional Autonoma de Mexico, MexicoReviewed by:
Patrizia Zaramella, Università degli Studi di Padova, ItalyPaola Garcia-delaTorre, Mexican Social Security Institute (IMSS), Mexico
© 2022 Snyder, Gebretsadik, Turi, McKennan, Havstad, Jackson, Ober, Lynch, McCauley, Seroogy, Zoratti, Khurana Hershey, Berdnikovs, Cunningham, Summar, Gern and Hartert. This is an open-access article distributed under the terms of the Creative Commons Attribution License (CC BY). The use, distribution or reproduction in other forums is permitted, provided the original author(s) and the copyright owner(s) are credited and that the original publication in this journal is cited, in accordance with accepted academic practice. No use, distribution or reproduction is permitted which does not comply with these terms.
*Correspondence: Tina V. Hartert tina.hartert@vumc.org
Specialty Section: This article was submitted to Pediatric Infectious Diseases, a section of the journal Frontiers in Pediatrics