- Department of Anesthesiology, National Center for Cardiovascular Diseases, Fuwai Hospital, Chinese Academy of Medical Sciences and Peking Union Medical College, Beijing, China
Background: Chronic hypoxia induces pulmonary microvascular endothelial dysfunction. The left atrial pressure (LAP) represents the hydrostatic pressure of pulmonary microcirculation. The conjunction of the LAP and any abnormal pulmonary microvascular endothelial barrier function will have an impact on pulmonary exudation, resulting in prolonged mechanical ventilation. This study aimed to investigate the tolerance threshold of the pulmonary microcirculation to LAP in children with tetralogy of Fallot (TOF) to avoid prolonged mechanical ventilation after surgery.
Methods: This retrospective study included 297 Chinese patients who underwent TOF correction at Fuwai Hospital. Patients were categorized according to their preoperative oxygen saturation (SpO2) level. One-to-one propensity score matching (PSM) revealed a total of 126 participants in the SpO2 < 90% and SpO2 ≥ 90% groups. Between-group comparisons were conducted to verify the correlation between hypoxia and prolonged mechanical ventilation. A subgroup analysis was performed to reveal the significant role of postoperative LAP stewardship on prolonged mechanical ventilation.
Results: Failure to extubate within the first 48 h (23.81% vs. 9.52%, P = 0.031) and prolonged mechanical ventilation (26.98% vs. 11.11%, P = 0.023) were more commonly observed in children with preoperative SpO2 < 90%. The incidence of prolonged mechanical ventilation consistently increased with LAP in both the SpO2 < 90% and SpO2 ≥ 90% groups, although LAP was still within the normal range (6–12 mmHg). Children in chronic hypoxic conditions tolerated lower LAP well. The tolerance threshold for postoperative LAP in children diagnosed with TOF under chronic hypoxic conditions was identified as 7 mmHg.
Conclusions: Children in a chronic hypoxic state may suffer from a high incidence of prolonged mechanical ventilation after surgical correction of TOF and may not tolerate higher postoperative LAP. To improve pulmonary prognosis, it is better to control and maintain the postoperative LAP at a lower state (≤7 mmHg) in children with chronic hypoxia.
Introduction
Tetralogy of Fallot (TOF) is a common cyanotic congenital heart disease. Due to pulmonary artery (PA) stenosis and chronic pulmonary bloodlessness, the lung compensates by growing collateral circulation to maintain the blood supply (1). The increased number of microcirculation vessels maintains lung function to some extent. However, weak neovascularization may explain pulmonary exudation is more likely to occur.
A large number of studies have shown that pulmonary microvascular endothelial dysfunction occurs under chronic hypoxia (2–4). Meanwhile, cardiopulmonary bypass (CPB) can further damage pulmonary microcirculation barrier function (5). Therefore, children with preoperative chronic hypoxia may be more prone to pulmonary exudation after surgical correction for TOF, resulting in prolonged mechanical ventilation (MV).
In the absence of significant differences in postoperative cardiac function and volume, left atrial pressure (LAP) essentially represents the hydrostatic pressure of the pulmonary microcirculation. Elevated LAP induces hemodynamic pulmonary congestion with high capillary pressure, which leads to increased fluid transfer to the capillary interstitial and alveolar space (6). This can result in edema formation and atelectasis with damaged gas exchange. We hypothesized that children with chronic hypoxia could only tolerate a lower level of postoperative LAP owing to impaired alveolar–capillary barrier function.
However, after a careful literature review, we could not find any clinical evidence of a higher incidence of related adverse pulmonary outcomes and specific LAP management in children with congenital heart disease and chronic hypoxia. Therefore, we retrospectively analyzed the relationship between preoperative oxygen saturation (SpO2) and postoperative prolonged mechanical ventilation in children undergoing TOF correction in our center and accurately confirmed the postoperative pulmonary LAP threshold in children with chronic hypoxia.
Materials and methods
Clinical study design
This observational retrospective study was conducted at Fuwai Hospital, Beijing, China. The study was approved by the Institutional Review Committee, and the requirement for informed consent was waived. We collected the clinical data of 297 patients who underwent corrective operations for TOF (from October 2017 to December 2018). Key criteria for exclusion are as follows: (1) patients aged >6 years old; (2) preoperative use of mechanical ventilation, blood transfusion, and extracorporeal membrane oxygenation; (3) previous palliative surgery or complex abnormality; (4) emergency surgery; (5) left ventricular ejection fraction (LVEF) <50%; (6) incomplete postoperative LAP records as well as a postoperative LAP outside the normal range (6–12 mmHg); and (7) missing data for endpoint diagnosis. After applying the exclusion criteria, 140 patients were included in the analysis (Figure 1). In this study, patients were categorized into two groups according to their preoperative oxygen saturation (SpO2).
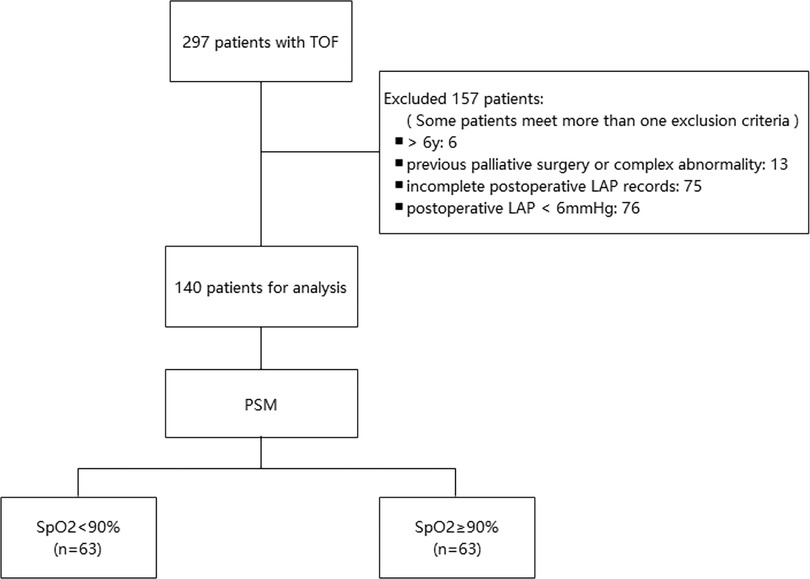
Figure 1. Flowchart of the study. LAP, left atrial pressure; PSM, propensity score matching; SpO2, oxygen saturation; TOF, tetralogy of Fallot.
Anesthetic and procedure
All patients underwent routine anesthetic management and CPB strategies at our institution. They were induced with midazolam (0.05–0.1 mg/kg), sufentanil (0.5–1 μg/kg), cisatracurium (0.2 mg/kg), and phencyclidine (0.02 mg/kg); anesthesia was maintained with inhaled sevoflurane, dexmedetomidine (1 μg/kg/h), cisatracurium (0.2–0.3 mg/kg/h), and sufentanil (2–3 μg/kg). Static inflation with airway pressure at 3–5 cm H2O was applied during CPB. SpO2, ECG, invasive blood pressure (radial artery), temperature (both nasopharynx and rectum), central venous pressure (5.5 F triple-lumen catheter into the right jugular vein), and LAP (20G single-lumen catheter into the right jugular vein and then into the left atrium after CPB through the atrium septum) were monitored routinely (7).
All patients were admitted to the ICU after TOF surgery. Inotropes were administrated by an intensivist. Patients were routinely treated with dopamine and dobutamine (3–8 μg/kg/min) and milrinone (0.2–1 μg/kg/min). Epinephrine and pituitrin were given as needed. Extubation was performed when patients met the following conditions: spontaneous respiration, hemodynamic stabilization, intact airway reflexes, manageable airway secretions, and chest x-ray excluding pulmonary edema and other lung diseases. The intensivist was responsible for the final decision to extubate or reintubate.
Data collection and outcomes
Patient data related to the procedure and anesthetic were obtained from the medical records and checked by two independent medical researchers. The demographic data included age, sex, height, and weight. The body surface area (BSA) was calculated using height and weight (BSA = 0.0061 × height[cm] + 0.0128 × weight[kg] − 0.1529). Preoperative data included the diagnosis, SpO2, LVEF, interventricular septal thickness (IVST), left ventricular end-diastolic volume (LVEDV), and right and left pulmonary artery diameters. Operative data included the operation time, CPB time, aortic cross-clamp (ACC) time, minimum temperature during CPB, intraoperative blood loss, and ultrafiltration volume. The left ventricular end-diastolic volume index (LVEDVI) was calculated using LVEDV and BSA (LVEDVI = LVEDV/BSA), and the Nakata index was calculated using the PA area and BSA [Nakata index = (right PA area + left PA area)/BSA]. Postoperative data included LAP 12 h after the operation, vasoactive-inotropic score (VIS) 12 h after the operation, intake and output volume during the day of surgery, LVEF on the day of surgery, and duration of MV before the first postoperative extubation and reintubation. One of the authors had full access to all the data in this study and took responsibility for their integrity and analysis.
Our study endpoint was prolonged MV, including failure to extubate within the first 48 h after the operation and reintubation. The postoperative LAP was the average value and was recorded as an integer.
Statistical analysis
Statistical analysis was performed using Stata software version 17, and P < 0.05 was considered statistically significant. The participants were first stratified into SpO2 < 90% and SpO2 ≥ 90% groups. Propensity score matching (PSM) was used to balance the potential factors affecting the outcome. To create propensity score-matched pairs, we performed one-to-one matching using the Stata module of psmatch2 (8), in which the control variables in our study were used (LVEDVI, Nakata index, CPB time, and VIS) (9–12). Based on nearest neighbor matching, the 63 SpO2 < 90% cases were matched with 63 SpO2 ≥ 90% cases. Standardized differences were used as indicators of intergroup balance. If the standardized differences were less than 10.0%, the covariates between the two groups were considered well-balanced (13).
For summary statistics, categorical variables were described as numbers and percentages and non-normally distributed continuous variables were described as median and interquartile ranges. The Mann–Whitney U-test and chi-square test were performed to detect differences in outcomes between groups. Subgroup analysis was conducted to investigate the association between postoperative LAP and the occurrence of prolonged MV stratified by SpO2. LAP was categorized based on the cutoff points calculated using receiver-operating characteristic curves. The optimal cutoff point corresponded to the maximum value of the Youden index. The Youden index was calculated by sensitivity and specificity (Youden index = sensitivity + specificity − 1) (14). Finally, the postoperative LAP threshold was obtained by calculating the between-group variation stratified by each level of LAP within the normal range.
Results
Sample characteristics of SpO2 < 90% and SpO2 ≥ 90% groups
A total of 140 patients (54.3% male) were included in this study. The mean age of the patients undergoing corrective surgery for TOF was 12.5 months. Some baseline characteristics showed statistically significant differences between the two groups before PSM. Higher CPB time (112 min vs. 95 min) and ACC time (76 min vs. 66 min) were observed in the SpO2 < 90% group. However, compared with patients in the SpO2 ≥ 90% group, patients in the SpO2 < 90% group showed a lower Nakata index (155.1 vs. 181.5) and LVEDVI (51.1 vs. 54.7). Using one-to-one PSM, 63 cases with SpO2 < 90% cases were matched with 63 cases with SpO2 ≥ 90%. The standardized percentage bias of covariates was close to 10% after PSM, showing a good match. Table 1 shows the demographic and clinical characteristics of the SpO2 < 90% and SpO2 ≥ 90% groups before and after PSM. The demographic and outcome-related characteristics of the SpO2 < 90% and SpO2 ≥ 90% groups were no longer statistically different.
Hypoxia and prolonged mechanical ventilation
Table 2 shows the frequency of prolonged mechanical ventilation-associated events in the SpO2 < 90% and SpO2 ≥ 90% groups. The comparison between the two groups revealed a significant difference in failure to extubate within the first 48 h (x2 = 4.63, P = 0.031) and prolonged MV (x2 = 5.15, P = 0.023). The occurrence of failure to extubate within the first 48 h (23.81% vs. 9.52%) and prolonged MV (26.98% vs. 11.11%) was more commonly observed in children with preoperative SpO2 < 90%.
LAP and prolonged mechanical ventilation
The correlation between MV time and LAP is demonstrated in Figure 2. There was a positive linear correlation between MV time and LAP (Spearman correlation coefficient = 0.41, P < 0.01). In addition, Figure 3 shows the association between LAP and prolonged MV stratified by SpO2. LAP was categorized based on the cutoff points (8 mmHg) calculated using receiver-operating characteristic curves. We found that the incidence of prolonged MV consistently increased with LAP in both the SpO2 < 90% (22.22% vs. 55.56%, P = 0.037) and SpO2 ≥ 90% group (7.55% vs. 30.00%, P = 0.038), although LAP was still within the normal range (6–12 mmHg). Compared to children in the SpO2 ≥ 90% group, a higher occurrence of prolonged MV was observed in children with SpO2 < 90% (22.22% vs. 7.55%, P = 0.033).
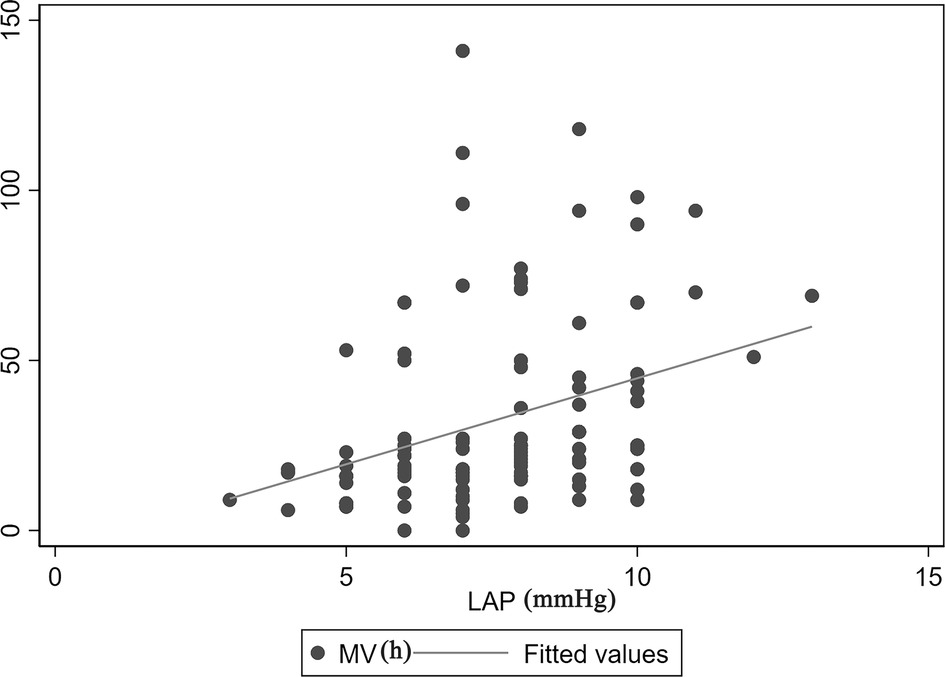
Figure 2. Correlation between MV time and LAP. There was a positive linear correlation between MV time and LAP (Spearman correlation coefficient = 0.41, P < 0.01). LAP, left atrial pressure; MV, mechanical ventilation.
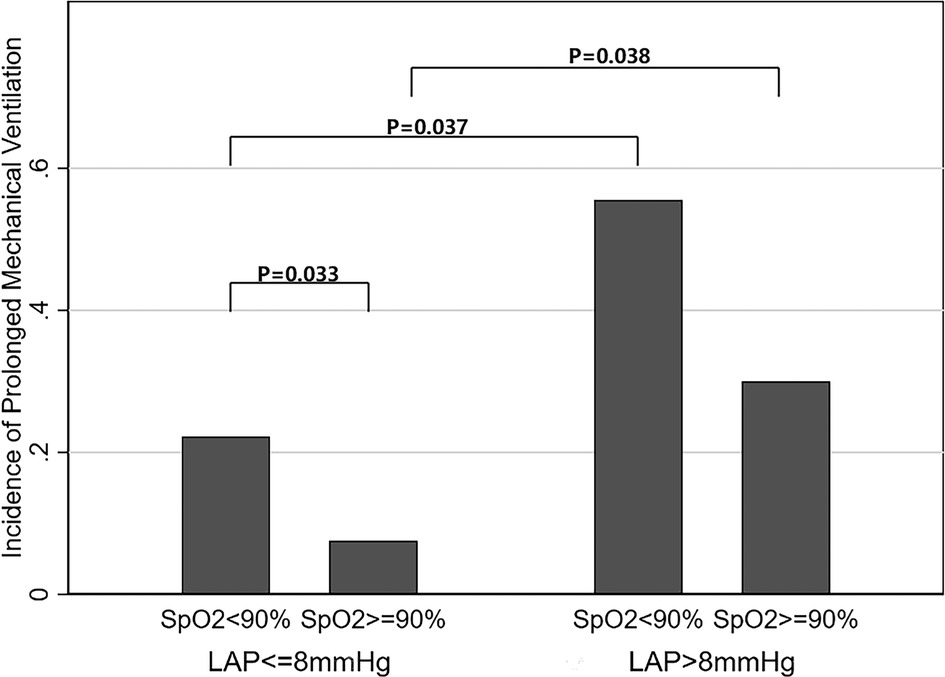
Figure 3. Relationship between LAP within the normal range (6–12 mmHg) and incidence of prolonged mechanical ventilation. The incidence of prolonged MV consistently increased with LAP in both the SpO2 < 90% (22.22% vs. 55.56%, P = 0.037) and SpO2 ≥ 90% (7.55% vs. 30.00%, P = 0.038) groups. Compared to children in the SpO2 ≥ 90% group, a higher occurrence of prolonged MV was observed in children with SpO2 < 90% (22.22% vs. 7.55%, P = 0.033). LAP, left atrial pressure; SpO2, oxygen saturation.
Postoperative LAP threshold
We further compared the variation in prolonged MV between the SpO2 < 90% and SpO2 ≥ 90% groups based on each level of LAP within the normal range (Table 3). When LAP was ≥8 mmHg, a higher occurrence of prolonged MV was observed in the SpO2 < 90% group, with a statistically significant difference. In addition, no significant difference in the incidence of prolonged MV was found between the groups when the LAP was ≤7 mmHg. In other words, if we control LAP to ≤7 mmHg, the occurrence of prolonged MV in children under chronic hypoxia will be close to that in non-hypoxic children.
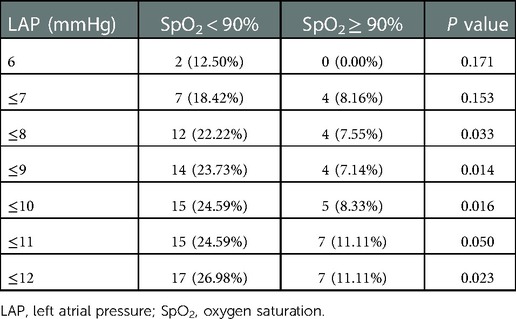
Table 3. Comparison of prolonged mechanical ventilation between groups based on each level of LAP within the normal range.
Discussion
In this study, we grouped patients based on preoperative SpO2 and regarded TOF patients with preoperative SpO2 < 90% as patients with chronic hypoxia (15). By measuring the analysis results above, we found that children with chronic hypoxic conditions were prone to a higher incidence of prolonged MV after cardiac surgery. Furthermore, we discussed the association between postoperative LAP and prolonged MV based on the premise that there were no remarkable differences in postoperative intake and output volume, LVEF, and VIS between the two groups. For pediatric patients with TOF, increasing LAP within the normal range has a substantial association with a high incidence of prolonged MV, and those in chronic hypoxic conditions tolerated lower postoperative LAP (≤7 mmHg) well.
TOF is a cyanotic congenital cardiac defect that was described as having four classical pathological processes: (1) pulmonary outflow tract obstruction, (2) ventricular septal defect, (3) overriding aortic root, and (4) right ventricular hypertrophy (16, 17). The development of the pulmonary vascular bed is strongly dependent on the flow. Pulmonary outflow tract obstruction results in reduced pulmonary blood flow, and the development and function of small pulmonary vessels are directly affected by diminished pulmonary flow in children with TOF (18). Postoperative fluid homeostasis in the lung depends on the integrity of the lung endothelial barrier (19). Breakdown of the barrier causes protein-rich pulmonary edema by increasing the flow of fluid and plasma proteins in the capillaries of the lungs (6). Based on the dysplasia of small pulmonary vessels in children with TOF, the fundamental reason for their susceptibility to postoperative lung exudation may be the disordered pulmonary vascular barrier function induced by chronic hypoxia and CPB surgery.
Many studies have established a paradigm in which hypoxia increases endothelial monolayer permeability (2–4). Other researchers reached the contradictory conclusion that pulmonary microvascular endothelial cells (PMVEC) grow to confluence under hypoxia, and incubation of endothelial monolayers under long-term hypoxic conditions forms a tighter and less permeable endothelial monolayer than similar cells grown under normoxia, as confirmed in both primary human and rat PMVEC (20). Chronic hypoxia before surgery may cause a number of compensatory changes in the lung tissue, particularly remodeling and hyperplasia of the pulmonary vessels (21). Furthermore, children undergoing surgical repair of complex congenital heart diseases tend to require a longer duration of CPB. It was reported that CPB increases pulmonary microvascular permeability and endothelial dysfunction through the Src kinase pathway and degradation of endothelial glycocalyx (5, 22). Therefore, pulmonary vascular barriers in cyanotic patients were weaker and more likely to be damaged after CPB surgery than those in noncyanotic patients, which makes cyanotic patients more likely to experience postoperative pulmonary exudation.
Increasing alveolocapillary barrier permeability or hydrostatic pressure gradients in the pulmonary circulation cause pulmonary edema to form (6). Studies have shown that increased pulmonary vascular pressure induces edema in animal models (23). In the absence of significant differences in postoperative cardiac function and volume, the LAP essentially represents the hydrostatic pressure of the pulmonary microcirculation. Elevated LAP leads to more fluid transfer to the capillary interstitial and alveolar spaces. Meanwhile, alveolar fluid reabsorption is impaired in the presence of elevated LAP (24) because a higher hydrostatic pulmonary circulation pressure inhibits active Na+ transport (25), which can result in more edema formation and pulmonary damage. It has also been reported that in rats with high LAP levels, lung permeability to large and small solutes increases progressively (25). High capillary pressures cause barrier damage, increasing permeability and fluid entry into the interstitium and alveoli. The underlying mechanism might be related to disruption of the endothelial junctional barrier by pressure-induced activation of Piezo1 (6). Accordingly, LAP is closely associated with pulmonary exudation and edema.
In cardiogenic pulmonary edema, excessive hydrostatic pressure across the pulmonary circulation causes swelling. For patients with a fragile pulmonary vascular barrier in chronic hypoxic conditions, it is beneficial to control hydrostatic pressure at a low state to maintain adequate tissue perfusion. LAP is affected by cardiac function, volume, and vasoactive drug intervention, reflecting the left ventricular preload when mitral valve function is normal. Thus, maintaining cardiac function, managing volume, and fluid balance, and monitoring the type and amount of vasoactive drugs are recommended to keep the LAP low. Even so, a very low level of LAP (≤5 mmHg) (26) leads to impairment in lung parenchymal mechanics because the absence of venous pressure may result in an unstable geometry of the alveolar space and deterioration of parenchymal mechanics (27).
Our research was done as rationally as possible. Covariates were selected as potential confounders based on previous studies. The variables associated with MV were age, LVEDVI, Nakata index, previous palliative operations, cardiac dysfunction, emergency status, transfusion, CPB time, and VIS (9, 11). Our exclusion criteria for the study design eliminated the impact of age, previous palliative operations, cardiac dysfunction, emergency status, and transfusion on the endpoint. In addition, we chose LVEDVI, Nakata index, CPB time, and VIS as adjusted covariates in the PSM. In our study, no differences were found in postoperative cardiac function and volume. Therefore, it is rational to discuss the influence of SpO2 and LAP on the study endpoint. This study is the first to provide the scope of postoperative LAP management for children with chronic hypoxia, which has great clinical significance in improving prognosis.
Nevertheless, this study has some limitations. First, our sample size was small; therefore, there was an obvious tendency for the incidence of prolonged MV between the SpO2 < 90% and SpO2 ≥ 90% groups, but no statistically significant variation was found when the postoperative LAP was >8 mmHg. Second, it is unfortunate that not all adjusted variables’ standardized percentage bias was less than 10% after PSM. However, this did not affect baseline consistency between the two groups. The pseudo-R2, an indicator of overall covariate imbalance, was much lower after matching than it was before (after; pseudo-R2 = 0.004, mean bias = 5.9, median bias = 4.4, P = 0.949 vs. before; pseudo-R2 = 0.070, mean bias = 33.8, median bias = 35.7, P = 0.011). These findings suggest an adequate balance of covariate distribution among the matched groups. Third, this was a single-center study, which may have introduced selection bias. Thus, the results may not have a strong ability to be generalized. In the future, performing multicenter prospective studies will help validate our findings.
In conclusion, more importance should be given to pediatric heart disease patients with chronic hypoxic conditions before surgery. Children with chronic preoperative hypoxia were prone to a high occurrence of prolonged mechanical ventilation after correction of TOF. They did not tolerate higher postoperative LAP well. To improve pulmonary prognosis, it is better to control the postoperative LAP at a lower state level (≤7 mmHg).
Data availability statement
The datasets generated for this study will not be made publicly available because of the data protection policy in our hospital. Requests to access the datasets should be directed to the corresponding author.
Ethics statement
The studies involving human participants were reviewed and approved by the Research Ethics Board of Fuwai Hospital. Written informed consent from the participants’ legal guardian/next of kin was not required to participate in this study in accordance with the national legislation and institutional requirements.
Author contributions
FY provided the idea for this study. JH and JD designed the experiments, analyzed the results, and wrote the paper. JH and QL were involved in data collection. XW analyzed the results. YJ and SY were involved in manuscript preparation. All authors contributed to the article and approved the submitted version.
Funding
This study was supported by the National Natural Science Foundation of China (Grant No. 81870070). YF is the principal investigator of the funded project.
Acknowledgments
The authors are grateful to Dongyun Bie, (Department of Anesthesiology, Chinese Academy of Medical Sciences, Fuwai Hospital, China) for her help in retrieving the data.
Conflict of interest
The authors declare that the research was conducted in the absence of any commercial or financial relationships that could be construed as a potential conflict of interest.
Publisher's note
All claims expressed in this article are solely those of the authors and do not necessarily represent those of their affiliated organizations, or those of the publisher, the editors and the reviewers. Any product that may be evaluated in this article, or claim that may be made by its manufacturer, is not guaranteed or endorsed by the publisher.
References
1. Apitz C, Webb GD, Redington AN. Tetralogy of Fallot. Lancet. (2009) 9699:1462–71. doi: 10.1016/s0140-6736(09)60657-7
2. Yegutkin GG, Helenius M, Kaczmarek E, Burns N, Jalkanen S, Stenmark K, et al. Chronic hypoxia impairs extracellular nucleotide metabolism and barrier function in pulmonary artery vasa vasorum endothelial cells. Angiogenesis. (2011) 4:503–13. doi: 10.1007/s10456-011-9234-0
3. Tsai SH, Huang PH, Tsai HY, Hsu YJ, Chen YW, Wang JC, et al. Roles of the hypoximir microRNA-424/322 in acute hypoxia and hypoxia-induced pulmonary vascular leakage. FASEB J. (2019) 11:12565–75. doi: 10.1096/fj.201900564RR
4. Li J, Xia Y, Huang Z, Zhao Y, Xiong R, Li X, et al. Novel HIF-1-target gene isthmin1 contributes to hypoxia-induced hyperpermeability of pulmonary microvascular endothelial cells monolayers. Am J Physiol Cell Physiol. (2021) 4:C671–80. doi: 10.1152/ajpcell.00124.2021
5. Selim J, Hamzaoui M, Boukhalfa I, Djerada Z, Chevalier L, Piton N, et al. Cardiopulmonary bypass increases endothelial dysfunction after pulmonary ischaemia-reperfusion in an animal model. Eur J Cardiothorac Surg. (2021) 5:1037–47. doi: 10.1093/ejcts/ezaa412
6. Friedrich EE, Hong Z, Xiong S, Zhong M, Di A, Rehman J, et al. Endothelial cell Piezo1 mediates pressure-induced lung vascular hyperpermeability via disruption of adherens junctions. Proc Natl Acad Sci U S A. (2019) 26:12980–5. doi: 10.1073/pnas.1902165116
7. Ding J, Zhang Q, Li L, Zhang H, Yan J, Li S, et al. A novel combined-catheter to monitor left and right atrial pressures: a simple and reliable method for pediatric cardiac surgery. Pediatr Crit Care Med. (2016) 3:210–5. doi: 10.1097/PCC.0000000000000641
8. Shin HR, Choi EY, Kim SK, Lee HY, Kim YS. Health literacy and frailty in community-dwelling older adults: evidence from a nationwide cohort study in South Korea. Int J Environ Res Public Health. (2021) 15:7918. doi: 10.3390/ijerph18157918
9. Bartz RR, Ferreira RG, Schroder JN, Davies J, Liu WW, Camara A, et al. Prolonged pulmonary support after cardiac surgery: incidence, risk factors and outcomes: a retrospective cohort study. J Crit Care. (2015) 5:940–4. doi: 10.1016/j.jcrc.2015.04.125
10. Sharma V, Rao V, Manlhiot C, Boruvka A, Fremes S, Wasowicz M. A derived and validated score to predict prolonged mechanical ventilation in patients undergoing cardiac surgery. J Thorac Cardiovasc Surg. (2017) 1:108–15. doi: 10.1016/j.jtcvs.2016.08.020
11. Li S, Zhang Y, Li S, Wang X, Zhang R, Lu Z, et al. Risk factors associated with prolonged mechanical ventilation after corrective surgery for tetralogy of Fallot. Congenit Heart Dis. (2015) 3:254–62. doi: 10.1111/chd.12205
12. Trouillet JL, Combes A, Vaissier E, Luyt CE, Ouattara A, Pavie A, et al. Prolonged mechanical ventilation after cardiac surgery: outcome and predictors. J Thorac Cardiovasc Surg. (2009) 4:948–53. doi: 10.1016/j.jtcvs.2009.05.034
13. Zheng X, Cao C, He Y, Wang X, Wu J, Hu H. Association between nonalcoholic fatty liver disease and incident diabetes mellitus among Japanese: a retrospective cohort study using propensity score matching. Lipids Health Dis. (2021) 1:59. doi: 10.1186/s12944-021-01485-x
14. Ali U, Goldenberg N, Foreman C, Crawford Lynn L, Honjo O, O'Leary J, et al. Association between cyanosis, transfusion, and thrombotic complications in neonates and children undergoing cardiac surgery. J Cardiothorac Vasc Anesth. (2020) 2:349–55. doi: 10.1053/j.jvca.2019.07.123
15. Templeton TW, Miller SA, Lee LK, Kheterpal S, Mathis MR, Goenaga-Díaz EJ, et al. Hypoxemia in young children undergoing one-lung ventilation: a retrospective cohort study. Anesthesiology. (2021) 5:842–53. doi: 10.1097/aln.0000000000003971
16. Van Praagh R. The first Stella van Praagh memorial lecture: the history and anatomy of tetralogy of Fallot. Semin Thorac Cardiovasc Surg Pediatr Card Surg Annu. (2009) 12:19–38. doi: 10.1053/j.pcsu.2009.01.004
17. Starr JP. Tetralogy of Fallot: yesterday and today. World J Surg. (2010) 4:658–68. doi: 10.1007/s00268-009-0296-8
18. Zhang XT, Liu YL, Ruan YM, Yu CT, Liu L. Lung pathology of complex congenital heart defect with diminished pulmonary blood flow but without aortopulmonary collateral artery and patent ductus arteriosus in infants and young children. Zhongguo Dang Dai Er Ke Za Zhi. (2008) 3:311–4.
19. Mehta D, Malik AB. Signaling mechanisms regulating endothelial permeability. Physiol Rev. (2006) 1:279–367. doi: 10.1152/physrev.00012.2005
20. Solodushko V, Parker JC, Fouty B. Pulmonary microvascular endothelial cells form a tighter monolayer when grown in chronic hypoxia. Am J Respir Cell Mol Biol. (2008) 4:491–7. doi: 10.1165/rcmb.2007-0127OC
21. Truog WE, Xu D, Ekekezie II, Mabry S, Rezaiekhaligh M, Svojanovsky S, et al. Chronic hypoxia and rat lung development: analysis by morphometry and directed microarray. Pediatr Res. (2008) 1:56–62. doi: 10.1203/PDR.0b013e31817289f2
22. Zhang J, Jiang Z, Bao C, Mei J, Zhu J. Cardiopulmonary bypass increases pulmonary microvascular permeability through the Src kinase pathway: involvement of caveolin-1 and vascular endothelial cadherin. Mol Med Rep. (2016) 3:2918–24. doi: 10.3892/mmr.2016.4831
23. Azzam ZS, Saldias FJ, Comellas A, Ridge KM, Rutschman DH, Sznajder JI. Catecholamines increase lung edema clearance in rats with increased left atrial pressure. J Appl Physiol. (2001) 3:1088–94. doi: 10.1152/jappl.2001.90.3.1088
24. Dixon DL, Mayne GC, Griggs KM, De Pasquale CG, Bersten AD. Chronic elevation of pulmonary microvascular pressure in chronic heart failure reduces bi-directional pulmonary fluid flux. Eur J Heart Fail. (2013) 4:368–75. doi: 10.1093/eurjhf/hfs201
25. Saldías FJ, Azzam ZS, Ridge KM, Yeldandi A, Rutschman DH, Schraufnagel D, et al. Alveolar fluid reabsorption is impaired by increased left atrial pressures in rats. Am J Physiol Lung Cell Mol Physiol. (2001) 3:L591–7. doi: 10.1152/ajplung.2001.281.3.L591
26. Ritzema J, Troughton R, Melton I, Crozier I, Doughty R, Krum H, et al. Physician-directed patient self-management of left atrial pressure in advanced chronic heart failure. Circulation. (2010) 9:1086–95. doi: 10.1161/CIRCULATIONAHA.108.800490
Keywords: tetralogy of Fallot, child, oxygen saturation, hypoxia, left atrial pressure, mechanical ventilation
Citation: Huang J, Ding J, Wu X, Jia Y, Liu Q, Yuan S and Yan F (2023) Chronic hypoxia prolongs postoperative mechanical ventilation and reduces the left atrial pressure threshold in children with tetralogy of Fallot. Front. Pediatr. 10:965703. doi: 10.3389/fped.2022.965703
Received: 10 June 2022; Accepted: 8 December 2022;
Published: 6 January 2023.
Edited by:
Goverdhan Puri, Post Graduate Institute of Medical Education and Research (PGIMER), IndiaReviewed by:
Pankaj Garg, Mayo Clinic Florida, United StatesAmit Saha, Wake Forest University, United States
© 2023 Huang, Ding, Wu, Jia, Liu, Yuan and Yan. This is an open-access article distributed under the terms of the Creative Commons Attribution License (CC BY). The use, distribution or reproduction in other forums is permitted, provided the original author(s) and the copyright owner(s) are credited and that the original publication in this journal is cited, in accordance with accepted academic practice. No use, distribution or reproduction is permitted which does not comply with these terms.
*Correspondence: Fuxia Yan yanfuxia@sina.com
†These authors have contributed equally to this work and share first authorship
Specialty Section: This article was submitted to Pediatric Critical Care, a section of the journal Frontiers in Pediatrics