- 1Department of Pediatrics, Third Xiangya Hospital, Central South University, Changsha, China
- 2School of Public Health, Central South University, Changsha, China
- 3Hunan Academy of Agricultural Sciences, Changsha, China
Background: Attention-deficit/hyperactivity disorder (ADHD) is one of the most common neurodevelopmental disorders in childhood and is caused by both genetic and environmental factors. As genetic factors are nonmodifiable, environmental factors have attracted increasing attention.
Objective: To investigate the relationships between urinary chlorpyrifos (CPF) levels, blood micronutrient levels, and ADHD prevalence in children living in rural areas of China.
Methods: This cross-sectional study collected data on CPF exposure (according to urinary levels), blood micronutrient levels, and ADHD prevalence in children aged 1–6 years in rural China. The CPF levels were determined by mass spectrometry. Blood levels of micronutrients, including zinc, iron, calcium, copper, magnesium, and vitamin D, were measured by professional detection kits. ADHD was diagnosed according to the Diagnostic and Statistical Manual of Mental Disorders, 4th Edition. Descriptive statistics and univariate analysis were conducted using SPSS 21.0, and path analysis was conducted using Mplus 8.0.
Results: Of the 738 children who met the eligibility criteria, 673 children (673/738, 91.2%) were included in the final analysis. Baseline questionnaires and urine samples were collected from all 673 subjects. A total of 672 children provided blood samples for micronutrient testing, and 651 completed the ADHD assessment. Approximately one-fifth of children (144/673, 21.4%) had detectable levels of CPF in their urine, and 6.9% (45/651) were diagnosed with ADHD. Path analysis showed that the total effect of CPF exposure on ADHD risk was 0.166 (P < 0.05), with a direct effect of 0.197 (P < 0.05) and an indirect effect of −0.031 (P < 0.05) via vitamin D. The mediating effect of urinary CPF levels on ADHD risk via vitamin D was 18.67%.
Conclusion: Higher levels of CPF exposure are associated with higher risk of ADHD. Additionally, increasing vitamin D levels may have a beneficial effect on the relationship between CPF exposure and ADHD risk. Our findings highlight the importance of modifying environmental factors to reduce ADHD risk and provide insight into future ADHD interventions.
Introduction
Attention-deficit/hyperactivity disorder (ADHD) is a neurological disorder characterized by persistent difficulty in regulating attention and affects 3.4 to 7.1% of school-aged children (1). Although ADHD is one of the most common developmental disorders, little is known about its underlying causes. A large number of studies have shown that the etiology of ADHD is multifaceted and encompasses both genetic susceptibility and environmental factors (2). Empirical research has suggested that exposure to heavy metals, dietary factors (3), and chemical pollutants such as bisphenol A (4), polycyclic aromatic hydrocarbons (5), and pesticides may increase the risk of ADHD. There may also be an interaction between environmental factors and genes that increases the risk of ADHD (6).
Chlorpyrifos (CPF) is one of the most widely used organophosphorus pesticides and an important environmental pollutant. Due to its persistence and bioaccumulation in the environment, exposure to CPF is unavoidable. Recently, the neurotoxicity of CPF has attracted increasing attention. The classic manifestation of CPF-induced damage to the nervous system is cholinergic syndrome, which is caused by acute poisoning and is characterized by the inhibition of cholinesterase activity. Chronic exposure to low-level CPF may have long-term adverse effects on the structure and function of the nervous system, increasing the risk of neurodegenerative diseases and neurodevelopmental disorders (7). Most previous studies have focused on the relationship between prenatal CPF exposure and offspring neurodevelopment rather than on children's direct exposure to CPF (8, 9). Although two recent studies have explored the correlation between CPF exposure and adolescent neurodevelopment, research on CPF exposure levels and ADHD in children is scarce (10, 11). As increasing evidence shows that exposure to low levels of CPF may increase the risk of ADHD, we aimed to explore this relationship in a younger group of children aged 1–6 years.
Nutrition and diet are also important environmental factors that influence the risk of ADHD (12). Recently, studies have found that increased consumption of food dyes and processed foods as well as decreased consumption of fruits and vegetables are associated with the severity of ADHD symptoms (13, 14). Follow-up studies found that many nutritional deficiencies, such as those of magnesium, zinc, iron, copper and vitamin D, are associated with ADHD symptoms (15–19). While diet therapy and nutritional supplement therapy have been explored, the results are inconsistent (20–22). More importantly, data on the relationship between micronutrient levels in children aged 1–6 years and the risk of ADHD are lacking.
To address the above two research gaps, this study aimed to investigate the relationships among urinary CPF levels, blood micronutrient levels, and ADHD risk in children aged 1–6 years. Based on the findings of previous studies, we made the following hypotheses: (1) exposure to CPF is a risk factor for ADHD; (2) micronutrients are protective factors against ADHD; and (3) micronutrients mediate the relationship between CPF exposure and ADHD risk.
Methods
Study population
Using cluster sampling, we selected Xinhua County in Hunan Province, Sansui County in Guizhou Province, and Gong’an County in Hubei Province to represent mountain, hill, and plains regions, respectively. In each of the three counties, three villages were randomly selected. All children aged 1–6 years in these villages were observed, except for those with the following conditions: (1) abnormal birth history, such as premature birth, multiple pregnancy, or birth asphyxia; (2) a history of head trauma; or (3) a history of serious diseases, such as genetic metabolic diseases, congenital abnormalities, feeding difficulties, severe malnutrition, recurrent respiratory diseases, or severe abnormalities of liver or kidney function. Based on the sample size recommendation for path analysis (23) and the Medical Statistics textbook, we determined that the minimum sample size required in each of the three areas was 200 children. We administered questionnaires, assessments of urinary CPF levels, blood micronutrient assessments, and ADHD diagnosis to all eligible children.
Prior to the field test, we verbally explained the purpose and process of the study to the parents of all children and emphasized the confidentiality of the data. The parents of all included children provided written informed consent. This study was strictly conducted according to international guidelines for the protection of human subjects and was approved by the Ethics Committee of the Third Xiangya Hospital of Central South University in Hunan, China. The ethics review number is 2014S162.
Parameters
Assessment of CPF levels in children's urine
Collection and pretreatment of urine samples
The urine samples were collected and analyzed as follows: 20–30 ml of morning urine was collected using disposable sterile urine cups; this sample was further divided into 5-ml polypropylene bottles. Each sample was numbered and recorded. All urine specimens were sent to the laboratory within 24 h of collection and stored at −80 °C until analysis.
Gas chromatography–mass spectrometry analysis (GC–MS) of CPF levels
Before the gas chromatography–mass spectrometry (GC‒MS) analysis, acetonitrile was added to the urine samples, and they were vortexed for approximately five minutes. NaCl was then added, and the mixture was vortexed for approximately one minute. The supernatant was pipetted into a rotary steaming flask, evaporated until nearly dry, and then diluted to a volume of 1 ml with ethyl acetate. This solution was transferred to an Eppendorf (EP) tube with 50 mg of primary secondary amine (PSA), vortexed for 30 s, and centrifuged at 8,000 r/min for four minutes. Finally, the supernatant was passed through a 0.22-μm filter, and GC‒MS analysis was performed.
Measurements of the CPF levels were performed as described elsewhere using GC‒MS analysis (Agilent GC7890A-5975). Laboratory quality control parameters for CPF levels were verified with reference to NY/T 788-2004 Pesticide Residue Test guidelines, which is the agricultural industry standard in China.
Micronutrient assessment
We collected venous whole blood samples from children in the morning after an overnight fast; these samples were refrigerated, transported, and stored until analysis. Measurements of micronutrients were conducted within 48 h after collection.
Vitamin D measurements were carried out using an enzyme-linked immunosorbent assay (ELISA). Monoclonal antibodies were used to detect 25-OH-D in whole blood samples with kits provided by the Oumeng Company (Germany). Calcium, iron, zinc, copper, and magnesium levels were all determined by atomic absorption spectrometry using Beijing Bohui Company's innovative photoelectric technology BH7100S. A total of 40 μl of whole blood was added to the instrument, and the samples were atomized by flame combustion, producing numerous ground-state free atoms that absorbed the characteristic spectrum of the measured elements emitted through a hollow cathode lamp. The levels of vitamin D (25-OH-D), calcium, iron, zinc, copper, and magnesium were treated as continuous variables. The blood level of lead was treated as a categorical variable (>10 µg/dl or ≤ 10 µg/dl), according to the levels of blood lead toxicity proposed by the Chinese Centers for Disease Control and Prevention (CDC). Laboratory quality control parameters for micronutrient assessment were conducted between laboratories in China every year and acquired the certificare from the national center for clinical laboratories.
ADHD assessment
The Diagnostic and Statistical Manual of Mental Disorders, 4th edition (DSM-IV) was used to evaluate ADHD in children aged 1–6 years. The evaluation consists of two parts, including nine symptoms of attention deficits and nine symptoms of hyperactive/impulsive behavior. An individual is diagnosed with ADHD if they exhibit six or more of the nine symptoms in one of the two dimensions and if these symptoms persist for at least six months. The ADHD outcome (with or without an ADHD diagnosis) was treated as a categorical variable.
Questionnaires
We used questionnaires to collect demographic characteristics and medical information from the children, such as age, sex, date of birth, primary caregiver and their educational level, annual family income per capita, maternal age at pregnancy, weight at birth, and breastfeeding history. The questionnaire consisted of a one-on-one survey with the children's primary caregivers. The investigators were uniformly trained before administering the survey.
Statistical analysis
All data analyses were conducted using SPSS version 21.0 for Windows (IBM Corp., Armonk, NY, USA) and Mplus 8.0 (Muthén / Muthén, Los Angeles, CA, USA). The mean (standard deviation [SD]) or the median (25th and 75th percentiles [P25 and P75]) were used to describe the distribution of continuous variables based on the normality of their distribution; n (%) were used to describe categorical variables. Nonparametric tests, t tests, nonparametric tests, or chi-square tests were used for univariate analysis. Pearson or Spearman correlation analyses were then conducted to examine the correlation among all study variables. Prior to the path analysis, we used logistic regression analysis to examine whether there were significant interactions between micronutrients (i.e., calcium, iron, zinc, copper, and magnesium) and CPF levels. As shown in Supplementary Appendix 2, no significant interactions were found; therefore, no interaction terms were included in the path analysis. Significant variables (i.e., P < 0.05) identified in the univariate and correlation analyses and demographic characteristics related to ADHD risk (i.e., age, sex, primary caregivers, and the education of primary caregivers) were included in the next step of the path analysis (24, 25). The path analysis was conducted using the maximum likelihood method. Model fit was examined with the relative χ2 goodness-of-fit statistic (χ2/degrees of freedom [df]), comparative fit index (CFI), Tucker and Lewis's index of fit (TLI), and root mean square error of approximation (RMSEA). A model was considered to exhibit acceptable fit if it met the following criteria: χ2/df <5, RMSEA <0.08, TLI >0.90, and CFI >0.90.
Results
Study population and participant characteristics
Of the 738 children who met the eligibility criteria, 673 (91.2%) were included in the final analysis. Baseline questionnaire data and urine samples were collected from these 673 subjects. A total of 672 children provided blood samples for micronutrient testing, and 651 completed the ADHD assessment (Figure 1). The mean age of the participants was 41.58 ± 15.4 months. The distributions and descriptive statistics of each variable, including sex, primary caregiver, district, education of primary caregiver, annual family income per capita, birth weight, maternal age at pregnancy, and age at weaning, are shown in Table 1.
Blood micronutrient levels and urinary CPF levels
The distributions of lead levels and descriptive statistics of each variable, including levels of zinc, iron, calcium, copper, magnesium, and 25-(OH)-D, are shown in Table 1.
Approximately one-fifth of children (144/673, 21.4%) had detectable levels of CPF in their urine. The number of positive samples and their corresponding CPF concentrations are shown in a histogram (Figure 2).
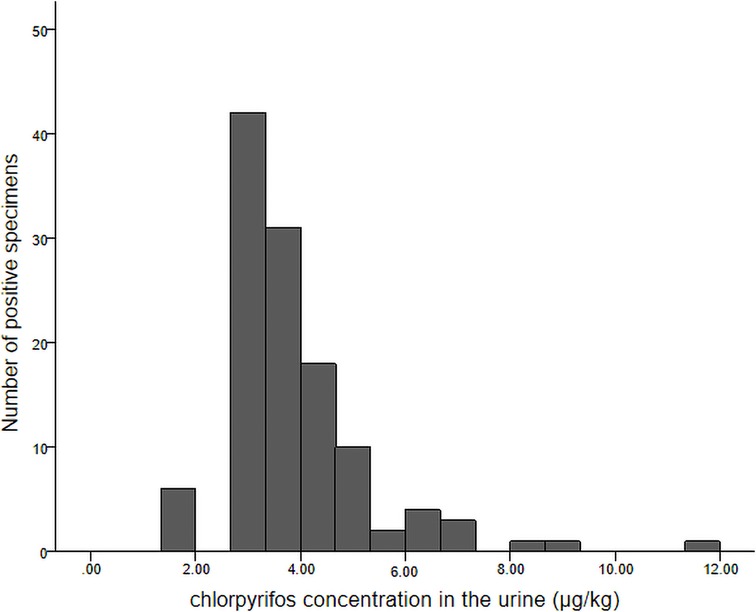
Figure 2. Histogram of chlorpyrifos concentrations detected in urine samples and the corresponding number of positive samples.
Results of ADHD assessment and univariate analyses
In total, 45 children were categorized as having ADHD, a positive rate of 6.9% (45/651). As shown in Table 1, there were significant differences in annual family income per capita and vitamin D levels between children with ADHD and those without ADHD. Specifically, children had a higher risk of ADHD if they had lower vitamin D levels and came from lower income families.
Results of the correlation and path analyses
The correlation results showed that vitamin D levels and age in months were positively correlated with CPF exposure, that annual family income per capita and vitamin D levels were positively correlated with ADHD risk, and that primary caregiver education was positively correlated with vitamin D levels (P < 0.05; see Supplementary Appendix 3).
The path model showed good fit, as shown by the normed chi-square value of 2.37, RMSEA of 0.048, TLI of 0.901, and CFI of 0.984. As shown in Table 2 and Figure 3, CPF had a direct effect on ADHD risk (estimate = 0.197, P < 0.05) as well as an indirect effect via vitamin D levels (estimate = −0.031, P < 0.05). The mediating effect of urinary CPF levels on ADHD risk via vitamin D was 18.67%.
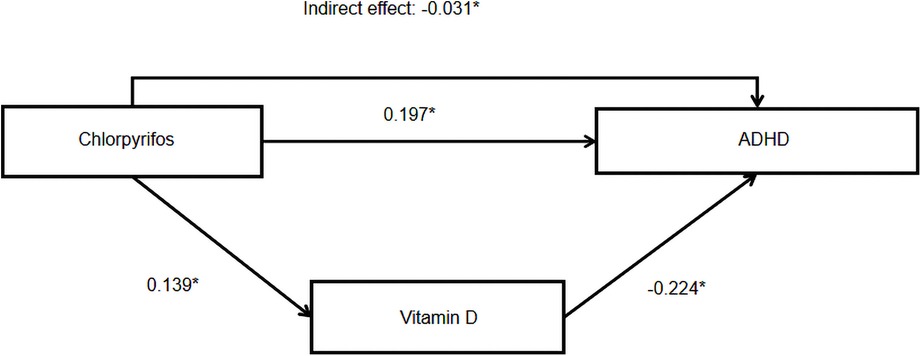
Figure 3. Model linking chlorpyrifos exposure to ADHD. Note: * indicates P < 0.05, statistically significant effects are indicated by solid lines.
Discussion
In the present study, we investigated the relationships among urinary CPF levels, blood micronutrient levels, and ADHD risk in rural Chinese children aged 1–6 years. Consistent with our hypotheses, we found that (1) CPF exposure was a risk factor for ADHD; (2) higher levels of vitamin D may reduce the risk of ADHD; and (3) vitamin D levels mediated the relationship between CPF exposure and ADHD risk.
This cross-sectional study investigated CPF levels in children's urine and found that 21.4% of children had detectable CPF levels. In 2016, a cross-sectional study of 140 children living near a banana plantation in Talamanca, Costa Rica reported that 40.7% (57/140) of children had urine samples positive for the CPF metabolite 3,5,6-trichloro-2-pyridinol (TCPy) (11). In addition, a cross-sectional survey of 60 children (aged 1–6 years) of North Carolina farm workers found that 83.3% of the children had detectable levels of CPF or CPF-methyl in their urine (26). Furthermore, 230 residents of Yunmou County, China underwent testing for urinary CPF; the positive rate was 10%. These results indicate that CPF exposure is related to the environment in which the participants live as well as the methods used to assess their exposure. Furthermore, knowledge of pesticide use influences children's pesticide exposure, indicating the importance of caregiver awareness (27).
The ADHD assessment revealed that 6.9% of children in rural China had ADHD in our study, a rate similar to that in previous studies of children in mainland China (6.3%) (28). Our study indicated that exposure to low-level CPF in the environment may increase the risk of ADHD in children aged 1–6 years. Previous studies showed that maternal exposure to CPF are related with ADHD in children (8, 9). Yu et al. reported that a dose-response relationship was found between urinary concentrations of organophosphate pesticide in Taiwanese children aged 4–15 and ADHD (29). Moreover, mouse experiments have also shown that prenatal CPF exposure impacts the motor activity and memory of offspring (30). But data on the relationship between CPF residual levels in children aged 1–6 and the risk of ADHD are lacking. As a multi-center cross-sectional study, our study is more conducive to early detection, diagnosis, and interventions of ADHD by screening ADHD among preschool children. The mechanism underlying neurotoxicity induced by exposure to low-level CPF remains unclear. Some studies have suggested that exposure to low levels of CPF affects motor coordination and function by influencing extracellular GABA concentrations in the cerebellum as well as NMDA receptor subunits in the hippocampus (30). Perinatal exposure to pesticides, such as CPF, is also correlated with alterations of synaptic plasticity that impair brain development and synaptic signaling (31). In addition, CPF exposure can interfere with transglutaminase activity in tissue as well as transient potential channels (32, 33). CPF-induced oxidative stress and its concomitant damage, including oxidative injury, metabolic imbalance, and lysosomal damage, are also likely correlated with its neurotoxic effects (34). However, counter to the mounting evidence of the neurotoxicity of CPF, researchers reported that early exposure to CPF in rats does not inhibit AChE activity in the blood or brain (35). Thus, further research is needed to elucidate the mechanism underlying neurotoxicity induced by exposure to low-level CPF.
This study also investigated micronutrient levels and their relationships with CPF exposure and ADHD risk. This design introduces biological samples (urine and blood samples), which are more direct and consequential for verifying the impact of environmental factors. Univariate and multivariate analyses revealed that vitamin D levels have a protective effect against the risk of ADHD, a finding consistent with the results of previous studies. Accumulated data suggest that decreased levels of vitamin D may also be a significant risk factor for ADHD (36–38) and that increased prenatal levels of vitamin D may have a protective effect against ADHD (39). However, a Danish cohort study and a Swedish case‒control study found no association between vitamin D levels and ADHD in older children (40, 41) Further research is necessary to determine whether an adequate supply of vitamin D can protect against ADHD. Regarding the underlying mechanism, vitamin D is a steroid hormone and can freely cross the blood‒brain barrier, thereby influencing neurons and glial cells. Research has indicated that microglia produce calcitriol in situ, upregulating the synthesis of nitric oxide synthase by glutathione; nitric oxide synthase has neuroprotective and neuromodulatory functions (42–44). Additionally, studies have suggested that vitamin D protects against many pathophysiological processes of neurons, such as oxidative stress as well as impaired neuronal calcium homeostasis, nerve conduction, detoxification, and immune regulation (45). Furthermore, vitamin D interacts with the promoters of various receptors, exerting epigenetic effects and thus altering their function (46–48). Therefore, vitamin D deficiency has the potential to promote neurodevelopmental disorders such as ADHD.
Furthermore, path analysis showed that CPF exposure was significantly correlated with vitamin D levels. The interaction between CPF and Vitamin D was studied in lab experimental setting. Krzysztof et al. reported that CPF can interfere with vitamin D3 metabolism in skin cells by altering the expression of the vitamin D3 receptor and enzymes such as CYP27A1, CYP27B1, and CYP24A1 (49, 50). As to the relationship of CPF exposure in children with vitamin D, little studies can be found on. This is the first cross sectional study that found the possible correlation between them. The skin is regularly exposed to environmental pollutants, including CPF; it is also the organ responsible for vitamin D3 synthesis. Thus, the interaction between CPF and vitamin D also warrants attention from the perspective of epidermal cells. However, studies on the interplay between CPF and vitamin D are scarce, and further research is needed.
This study has several limitations. First, our study was based on cross-sectional data. Further research using a longitudinal design is needed to confirm the causal relationship between CPF exposure and ADHD risk. Second, there may be self-report bias regarding ADHD. Future research should consider using different forms of data collection to verify whether such measurement bias exists. The third limitation of our study is the possibility of residual confounding, which can not be fully eliminated in an observational study. For example, in this study we did not include children's allergy history and eating behaviors, which may result in biases in our analyses. These variables should be taken into consideration in future studies (51, 52). Finally, using a one-time measurement of urinary CPF levels may not be the best method for estimating long-term CPF exposure; thus, a follow-up study is necessary.
In conclusion, we investigated the relationships between CPF exposure, ADHD risk, and micronutrient levels in rural Chinese children aged 1–6 years. We found that exposure to CPF was a risk factor for ADHD in children and that higher levels of vitamin D protected against ADHD. Supplemental vitamin D for children may thus help protect against ADHD risk in children exposed to CPF. Our findings provide insights for future ADHD interventions that involve modifying environmental risk factors, such as reducing CPF exposure and increasing vitamin D intake, to reduce ADHD risk.
Data availability statement
The original contributions presented in the study are included in the article/Supplementary Material, further inquiries can be directed to the corresponding author/s.
Ethics statement
The studies involving human participants were reviewed and approved by The study was approved by the Medical Ethics Committee of the Third Xiangya Hospital. Written informed consent to participate in this study was provided by the participants’ legal guardian/next of kin.
Author contributions
YD and LZ designed this research. WZ, YD, CZ, HD, LG, XL, WH, JT, and LZ performed the suvey and collected the data. WZ and YD analyzed the data. WZ and LZ wrote the manuscript. All authors contributed to the article and approved the submitted version.
Funding
This work was supported by the UBS Optimus Foundation (No. 6102).
Acknowledgments
The authors thank the study participants for their contribution to the research as well as current and past investigators and staff. We are grateful to the staff of the Medical Center Laboratory at the Third Xiangya Hospital for their excellent technical assistance.
Conflict of interest
The authors declare that the research was conducted in the absence of any commercial or financial relationships that could be construed as a potential conflict of interest.
Publisher's note
All claims expressed in this article are solely those of the authors and do not necessarily represent those of their affiliated organizations, or those of the publisher, the editors and the reviewers. Any product that may be evaluated in this article, or claim that may be made by its manufacturer, is not guaranteed or endorsed by the publisher.
Supplementary material
The Supplementary Material for this article can be found online at https://www.frontiersin.org/articles/10.3389/fped.2022.952559/full#supplementary-material.
References
1. Arruda MA, Querido CN, Bigal ME, Polanczyk GV. ADHD And mental health status in Brazilian school-age children. J Atten Disord. (2015) 19(1):11–7. doi: 10.1177/1087054712446811
2. Thapar A, Cooper M, Eyre O, Langley K. What have we learnt about the causes of ADHD? J Child Psychol Psychiatry. (2013) 54(1):3–16. doi: 10.1111/j.1469-7610.2012.02611.x
3. Del-Ponte B, Quinte GC, Cruz S, Grellert M, Santos IS. Dietary patterns and attention deficit/hyperactivity disorder (ADHD): a systematic review and meta-analysis. J Affect Disord. (2019) 252:160–73. doi: 10.1016/j.jad.2019.04.061
4. Li Y, Zhang H, Kuang H, Fan R, Cha C, Li G, et al. Relationship between bisphenol A exposure and attention-deficit/ hyperactivity disorder: a case-control study for primary school children in Guangzhou, China. Environ Pollut. (2018) 235:141–9. doi: 10.1016/j.envpol.2017.12.056
5. Perera FP, Chang HW, Tang D, Roen EL, Herbstman J, Margolis A, et al. Early-life exposure to polycyclic aromatic hydrocarbons and ADHD behavior problems. Plos One. (2014) 9(11):e111670. doi: 10.1371/journal.pone.0111670
6. Cortese S. The neurobiology and genetics of attention-deficit/hyperactivity disorder (ADHD): what every clinician should know. Eur J Paediatr Neurol. (2012) 16(5):422–33. doi: 10.1016/j.ejpn.2012.01.009
7. Mostafalou S, Abdollahi M. The link of organophosphorus pesticides with neurodegenerative and neurodevelopmental diseases based on evidence and mechanisms. Toxicology. (2018) 409:44–52. doi: 10.1016/j.tox.2018.07.014
8. Dalsager L, Fage-Larsen B, Bilenberg N, Jensen TK, Nielsen F, Kyhl HB, et al. Maternal urinary concentrations of pyrethroid and chlorpyrifos metabolites and attention deficit hyperactivity disorder (ADHD) symptoms in 2-4-year-old children from the odense child cohort. Environ Res. (2019) 176:108533. doi: 10.1016/j.envres.2019.108533
9. Fortenberry GZ, Meeker JD, Sanchez BN, Barr DB, Panuwet P, Bellinger D, et al. Urinary 3,5,6-trichloro-2-pyridinol (TCPY) in pregnant women from Mexico city: distribution, temporal variability, and relationship with child attention and hyperactivity. Int J Hyg Environ Health. (2014) 217(2-3):405–12. doi: 10.1016/j.ijheh.2013.07.018
10. Rohlman DS, Ismail A, Bonner MR, Abdel RG, Hendy O, Ortega DL, et al. Occupational pesticide exposure and symptoms of attention deficit hyperactivity disorder in adolescent pesticide applicators in Egypt. Neurotoxicology. (2019) 74:1–6. doi: 10.1016/j.neuro.2019.05.002
11. van Wendel DJB, Mora AM, Lindh CH, Hernandez-Bonilla D, Cordoba L, Wesseling C, et al. Pesticide exposure and neurodevelopment in children aged 6-9 years from Talamanca, Costa Rica. Cortex. (2016) 85:137–50. doi: 10.1016/j.cortex.2016.09.003
12. Millichap JG, Yee MM. The diet factor in attention-deficit/hyperactivity disorder. Pediatrics. (2012) 129(2):330–7. doi: 10.1542/peds.2011-2199
13. Rios-Hernandez A, Alda JA, Farran-Codina A, Ferreira-Garcia E, Izquierdo-Pulido M. The mediterranean diet and ADHD in children and adolescents. Pediatrics. (2017) 139(2):e20162027. doi: 10.1542/peds.2016-2027
14. Pelsser LM, Frankena K, Toorman J, Savelkoul HF, Dubois AE, Pereira RR, et al. Effects of a restricted elimination diet on the behaviour of children with attention-deficit hyperactivity disorder (INCA study): a randomised controlled trial. Lancet. (2011) 377(9764):494–503. doi: 10.1016/S0140-6736(10)62227-1
15. Hariri M, Azadbakht L. Magnesium, iron, and zinc supplementation for the treatment of attention deficit hyperactivity disorder: a systematic review on the recent literature. Int J Prev Med. (2015) 6:83. doi: 10.4103/2008-7802.164313
16. Viktorinova A, Ursinyova M, Trebaticka J, Uhnakova I, Durackova Z, Masanova V. Changed plasma levels of zinc and copper to zinc ratio and their possible associations with parent- and teacher-rated symptoms in children with attention-deficit hyperactivity disorder. Biol Trace Elem Res. (2016) 169(1):1–7. doi: 10.1007/s12011-015-0395-3
17. Skalny AV, Mazaletskaya AL, Ajsuvakova OP, Bjorklund G, Skalnaya MG, Chao JC, et al. Serum zinc, copper, zinc-to-copper ratio, and other essential elements and minerals in children with attention deficit/hyperactivity disorder (ADHD). J Trace Elem Med Biol. (2020) 58:126445. doi: 10.1016/j.jtemb.2019.126445
18. Yang R, Gao W, Li R, Zhao Z. Were plasma trace element levels changed in the children with ADHD? Biol Trace Elem Res. (2015) 168(2):516–7. doi: 10.1007/s12011-015-0524-z
19. Sucksdorff M, Brown AS, Chudal R, Surcel HM, Hinkka-Yli-Salomaki S, Cheslack-Postava K, et al. Maternal vitamin D levels and the risk of offspring attention-deficit/hyperactivity disorder. J Am Acad Child Adolesc Psychiatry. (2021) 60(1):142–51. doi: 10.1016/j.jaac.2019.11.021
20. Sarris J, Kean J, Schweitzer I, Lake J. Complementary medicines (herbal and nutritional products) in the treatment of attention deficit hyperactivity disorder (ADHD): a systematic review of the evidence. Complement Ther Med. (2011) 19(4):216–27. doi: 10.1016/j.ctim.2011.06.007
21. Heilskov RM, Andersen LB, Houmann T, Bilenberg N, Hvolby A, Molgaard C, et al. Diet in the treatment of ADHD in children - a systematic review of the literature. Nord J Psychiatry. (2015) 69(1):1–18. doi: 10.3109/08039488.2014.921933
22. Rucklidge JJ, Eggleston M, Darling KA, Stevens AJ, Kennedy MA, Frampton CM. Can we predict treatment response in children with ADHD to a vitamin-mineral supplement? An investigation into pre-treatment nutrient serum levels, MTHFR status, clinical correlates and demographic variables. Prog Neuropsychopharmacol Biol Psychiatry. (2019) 89:181–92. doi: 10.1016/j.pnpbp.2018.09.007
23. Golob TF. Structural equation modeling for travel behavior research. Kidlington: Elsevier Ltd (2003). 1–25.
24. Posner J, Polanczyk GV, Sonuga-Barke E. Attention-deficit hyperactivity disorder. The Lancet. (2020) 395(10222):450–62. doi: 10.1016/S0140-6736(19)33004-1
25. Cortese S, Coghill D. Twenty years of research on attention-deficit/hyperactivity disorder (ADHD): looking back, looking forward. Evid Based Ment Health. (2018) 21(4):173–6. doi: 10.1136/ebmental-2018-300050
26. Kamel F, Engel LS, Gladen BC, Hoppin JA, Alavanja MC, Sandler DP. Neurologic symptoms in licensed pesticide applicators in the agricultural health study. Hum Exp Toxicol. (2007) 26(3):243–50. doi: 10.1177/0960327107070582
27. Deng Y, Dai H, Zeng M, Guan L, Luo X, Zhang C, et al. Knowledge and behavior regarding pesticide use: a survey among caregivers of children aged 1-6 years from rural China. Environ Sci Pollut Res Int. (2019) 26(22):23037–43. doi: 10.1007/s11356-019-05560-w
28. Liu A, Xu Y, Yan Q, Tong L. The prevalence of attention deficit/hyperactivity disorder among Chinese children and adolescents. Sci Rep. (2018) 8(1):11169. doi: 10.1038/s41598-018-29488-2
29. Yu CJ, Du JC, Chiou HC, Chung MY, Yang W, Chen YS, et al. Increased risk of attention-deficit/hyperactivity disorder associated with exposure to organophosphate pesticide in Taiwanese children. Andrology-Us. (2016) 4(4):695–705. doi: 10.1111/andr.12183
30. Gomez-Gimenez B, Felipo V, Cabrera-Pastor A, Agusti A, Hernandez-Rabaza V, Llansola M. Developmental exposure to pesticides alters motor activity and coordination in rats: sex differences and underlying mechanisms. Neurotox Res. (2018) 33(2):247–58. doi: 10.1007/s12640-017-9823-9
31. Lopez-Merino E, Cuartero MI, Esteban JA, Briz V. Perinatal exposure to pesticides alters synaptic plasticity signaling and induces behavioral deficits associated with neurodevelopmental disorders. Cell Biol Toxicol. (2022). doi: 10.1007/s10565-022-09697-2. [Epub ahead of print]
32. Li W, Ehrich M. Effects of chlorpyrifos on transient receptor potential channels. Toxicol Lett. (2022) 358:100–4. doi: 10.1016/j.toxlet.2022.01.016
33. Aldubayan MA, Almami IS, Felemban SG, Alhowail AH, Bonner P, Hargreaves AJ. Organophosphates modulate tissue transglutaminase activity in differentiated C6 neural cells. Eur Rev Med Pharmacol Sci. (2022) 26(1):168–82. doi: 10.26355/eurrev_202201_27766
34. Falfushynska H, Khatib I, Kasianchuk N, Lushchak O, Horyn O, Sokolova IM. Toxic effects and mechanisms of common pesticides (roundup and chlorpyrifos) and their mixtures in a zebrafish model (danio rerio). Sci Total Environ. (2022) 833:155236. doi: 10.1016/j.scitotenv.2022.155236
35. Berg EL, Ching TM, Bruun DA, Rivera JK, Careaga M, Ellegood J, et al. Translational outcomes relevant to neurodevelopmental disorders following early life exposure of rats to chlorpyrifos. J Neurodev Disord. (2020) 12(1):40. doi: 10.1186/s11689-020-09342-1
36. Kamal M, Bener A, Ehlayel MS. Is high prevalence of vitamin D deficiency a correlate for attention deficit hyperactivity disorder? Atten Defic Hyperact Disord. (2014) 6(2):73–8. doi: 10.1007/s12402-014-0130-5
37. Goksugur SB, Tufan AE, Semiz M, Gunes C, Bekdas M, Tosun M, et al. Vitamin D status in children with attention-deficit-hyperactivity disorder. Pediatr Int. (2014) 56(4):515–9. doi: 10.1111/ped.12286
38. Shang-Guan LL, Zhao YR. Serum levels of 25-hydroxyvitamin D in children with attention deficit hyperactivity disorder. Zhongguo Dang Dai Er Ke Za Zhi. (2015) 17(8):837–40. PMID: 26287349
39. Mossin MH, Aaby JB, Dalgard C, Lykkedegn S, Christesen HT, Bilenberg N. Inverse associations between cord vitamin D and attention deficit hyperactivity disorder symptoms: a child cohort study. Aust N Z J Psychiatry. (2017) 51(7):703–10. doi: 10.1177/0004867416670013
40. Gustafsson P, Rylander L, Lindh CH, Jonsson BA, Ode A, Olofsson P, et al. Vitamin D status at birth and future risk of attention deficit/hyperactivity disorder (ADHD). Plos One. (2015) 10(10):e140164. doi: 10.1371/journal.pone.0140164
41. Strom M, Halldorsson TI, Hansen S, Granstrom C, Maslova E, Petersen SB, et al. Vitamin D measured in maternal serum and offspring neurodevelopmental outcomes: a prospective study with long-term follow-up. Ann Nutr Metab. (2014) 64(3-4):254–61. doi: 10.1159/000365030
42. DeLuca HF. Vitamin D: historical overview. Vitam Horm. (2016) 100:1–20. doi: 10.1016/bs.vh.2015.11.001
43. Patrick RP, Ames BN. Vitamin D and the omega-3 fatty acids control serotonin synthesis and action, part 2: relevance for ADHD, bipolar disorder, schizophrenia, and impulsive behavior. Faseb J. (2015) 29(6):2207–22. doi: 10.1096/fj.14-268342
44. Garcion E, Wion-Barbot N, Montero-Menei CN, Berger F, Wion D. New clues about vitamin D functions in the nervous system. Trends Endocrinol Metab. (2002) 13(3):100–5. doi: 10.1016/s1043-2760(01)00547-1
45. Buell JS, Dawson-Hughes B. Vitamin D and neurocognitive dysfunction: preventing “D"ecline? Mol Aspects Med. (2008) 29(6):415–22. doi: 10.1016/j.mam.2008.05.001
46. Currenti SA. Understanding and determining the etiology of autism. Cell Mol Neurobiol. (2010) 30(2):161–71. doi: 10.1007/s10571-009-9453-8
47. Landel V, Millet P, Baranger K, Loriod B, Feron F. Vitamin D interacts with Esr1 and Igf1 to regulate molecular pathways relevant to Alzheimer's disease. Mol Neurodegener. (2016) 11:22. doi: 10.1186/s13024-016-0087-2
48. Obradovic D, Zanca C, Vogl A, Trumbach D, Deussing J, Condorelli G, et al. Vitamin D3 signalling in the brain enhances the function of phosphoprotein enriched in astrocytes–15 kD (PEA-15). J Cell Mol Med. (2009) 13(9B):3315–28. doi: 10.1111/j.1582-4934.2009.00753.x
49. Sawicki K, Czajka M, Matysiak-Kucharek M, Kurzepa J, Wojtyla-Buciora P, Zygo K, et al. Chlorpyrifos alters expression of enzymes involved in vitamin D3 synthesis in skin cells. Pestic Biochem Physiol. (2021) 174:104812. doi: 10.1016/j.pestbp.2021.104812
50. Sawicki K, Czajka M, Matysiak-Kucharek M, Kruszewski M, Skawinski W, Brzoska K, et al. Chlorpyrifos stimulates expression of vitamin D3 receptor in skin cells irradiated with UVB. Pestic Biochem Physiol. (2019) 154:17–22. doi: 10.1016/j.pestbp.2018.12.003
51. Kaas TH, Vinding RK, Stokholm J, Bonnelykke K, Bisgaard H, Chawes BL. Association between childhood asthma and attention deficit hyperactivity or autism spectrum disorders: a systematic review with meta-analysis. Clin Exp Allergy. (2021) 51(2):228–52. doi: 10.1111/cea.13750
Keywords: chlorpyrifos, ADHD, micronutrients, vitamin D, cross-sectional study, children
Citation: Zhou W, Deng Y, Zhang C, Dai H, Guan L, Luo X, He W, Tian J and Zhao L (2022) Chlorpyrifos residue level and ADHD among children aged 1–6 years in rural China: A cross-sectional study. Front. Pediatr. 10:952559. doi: 10.3389/fped.2022.952559
Received: 25 May 2022; Accepted: 27 September 2022;
Published: 14 October 2022.
Edited by:
Fangbiao Tao, Anhui Medical University, ChinaReviewed by:
Xin Zhang, Tianjin Medical University, ChinaGuangbo Qu, Anhui Medical University, China
Honglv Xu, Kunming University, China
© 2022 Zhou, Deng, Zhang, Dai, Guan, Luo, He, Tian and Zhao. This is an open-access article distributed under the terms of the Creative Commons Attribution License (CC BY). The use, distribution or reproduction in other forums is permitted, provided the original author(s) and the copyright owner(s) are credited and that the original publication in this journal is cited, in accordance with accepted academic practice. No use, distribution or reproduction is permitted which does not comply with these terms.
*Correspondence: Lingling Zhao bGx6aGFvMjAxMUBxcS5jb20=
Specialty Section: This article was submitted to Children and Health, a section of the journal Frontiers in Pediatrics