- 1Department of Congenital Heart Disease – Pediatric Cardiology, German Heart Center Berlin, Berlin, Germany
- 2Institute of Computer-assisted Cardiovascular Medicine, Charité – Universitätsmedizin Berlin, corporate member of Freie Universität Berlin, Humboldt-Universität zu Berlin, and Berlin Institute of Health, Berlin, Germany
- 3DZHK (German Centre for Cardiovascular Research), partner site Berlin, Berlin, Germany
- 4Department of Pediatrics, Division of Cardiology, Charité – Universitätsmedizin Berlin, corporate member of Freie Universität Berlin, Humboldt-Universität zu Berlin, and Berlin Institute of Health, Berlin, Germany
- 5Department of Internal Medicine – Cardiology, German Heart Center Berlin, Berlin, Germany
- 6Department of Cardiology, Charité – Universitätsmedizin Berlin, corporate member of Freie Universität Berlin, Humboldt-Universität zu Berlin, and Berlin Institute of Health, Berlin, Germany
Background: Focal myocardial fibrosis in the systemic right ventricle (RV) is related to ventricular dysfunction and adverse outcome in patients with d-transposition of the great arteries (dTGA) post atrial redirection and those with congenitally corrected TGA (ccTGA). The role of diffuse fibrotic lesions in these conditions remains poorly understood. Our study aimed to investigate diffuse myocardial fibrosis by measuring extracellular volume (ECV) with cardiovascular magnetic resonance (CMR) and to explore correlations between ECV and clinical as well as functional markers of heart failure in patients with TGA and systemic RV.
Methods: We prospectively included dTGA and ccTGA patients aged ≥14 years and compared them to healthy controls. Standardized CMR included modified Look-Locker Inversion recovery T1 mapping to quantify diffuse myocardial fibrosis in the systemic RV and the subpulmonary left ventricle (LV). The centerline of RV and LV myocardium was marked with a line of interest tool to determine native and post-contrast T1 for quantification of ECV.
Results: In total, 13 patients (dTGA: n = 8, ccTGA: n = 5) with a median age of 30.3 years were enrolled. LV ECV was higher in patients than in controls [34% (30%–41%) vs. 26% (23%–27%), p < 0.001], with values increased above the upper limit of normal in 10/13 patients (77%). RV ECV tended to be higher in patients than in controls, albeit without statistical significance [29% (27%–32%) vs. 28% (26%–29%), p = 0.316]. Patients with elevated LV ECV had lower LV ejection fraction than those with normal ECV (52 ± 5% vs. 65 ± 4%, p = 0.007). Correlations with clinical parameters were not observed. LV ECV was significantly higher than RV ECV (p = 0.016) in the patient group.
Conclusions: In this study, LV ECV was significantly increased in TGA patients compared to controls, and was associated with LV dysfunction. Our data suggest that ECV may serve as a non-invasive tissue marker of heart failure in TGA with systemic RV. Further research is necessary to evaluate the prognostic implications and the potential role of ECV in monitoring disease progression and guiding therapy, aiming to maintain LV function or train the LV for subaortic location in TGA patients from infancy to adulthood.
Introduction
Given the advances in care for patients with congenital heart disease (CHD), the majority of children born with CHD reach adulthood (1, 2). However, increased mortality has been described in adult patients with CHD, particularly in the young, with heart failure as the leading cause of death (3). Heart failure signs and symptoms are seen in 22% of patients with d-loop transposition of the great arteries (dTGA) post atrial redirection, and in 32% of patients with congenitally corrected transposition of the great arteries (ccTGA) (4).
Myocardial fibrosis contributes to the development of heart failure in CHD (5, 6). Cardiovascular magnetic resonance (CMR) studies have identified focal myocardial fibrosis in the systemic right ventricle (RV) as assessed by late gadolinium enhancement (LGE) imaging to be associated with ventricular dysfunction and adverse outcome in dTGA post atrial redirection and in ccTGA (7–10). Data on diffuse myocardial fibrosis from CMR T1 mapping in TGA with a systemic RV are limited and somewhat controversial, pointing to an increased fibrotic burden in the systemic RV (11), the subpulmonary left ventricle (LV) (12), the interventricular septum (13), or both RV and LV (14).
With the present study, we aimed to determine biventricular extracellular volume (ECV) as a non-invasive measure of diffuse myocardial fibrosis by applying CMR T1 mapping and to evaluate the correlation of ECV with clinical and functional markers of heart failure in patients with TGA and systemic RV.
Materials and methods
Study population
We prospectively enrolled patients of ages ≥14 years with a diagnosis of dTGA post atrial redirection or ccTGA who were referred for clinically indicated CMR. Exclusion criteria were arrhythmias, cardiac decompensation, cardiomyopathies, chronic or acute infection up to four weeks prior to CMR, chronic systemic diseases, significant renal impairment, devices non-compatible with CMR, and claustrophobia. Patients further underwent blood sampling for hematocrit and N-terminal pro brain natriuretic peptide (NT-proBNP) immediately before CMR, and cardiopulmonary exercise testing (CPET).
Healthy subjects with normal 12-lead electrocardiogram (ECG), blood pressure, and renal function, and no history or symptoms of cardiovascular disease or contraindications for CMR were included as controls.
CMR protocol
All participants received standardized CMR at 1.5 T (Philips Healthcare, Best, The Netherlands). Blood samples for hematocrit and NT-proBNP were collected immediately before CMR. Cine images were acquired with a steady-state free precision sequence in short axis (SAX) and long axis planes as well as in axial orientation covering the entire ventricles. LGE imaging in SAX was conducted using a T1-weighted inversion-recovery three-dimensional spoiled gradient echo sequence 5 min after intravenous bolus application of gadolinium-DOTA (Dotarem®, Guerbet) at a dose of 0.1 mmol/kg. An individually adapted prepulse delay Look-Locker sequence was applied to determine inversion times for nulling myocardium.
For T1 mapping, an ECG-gated single-shot modified Look-Locker inversion-recovery (MOLLI) sequence was used (15) with sequence parameters as follows: MOLLI scheme 3b(3b)3b(3b)5b, slice thickness 8.0 mm, repetition time 2.4 ms, echo time 1.2 ms, flip angle 35°. Native and post-contrast T1 images were acquired in midventricular SAX and axial four-chamber orientation in breath-hold at end-expiration before and 15 min after contrast bolus application (Figure 1).
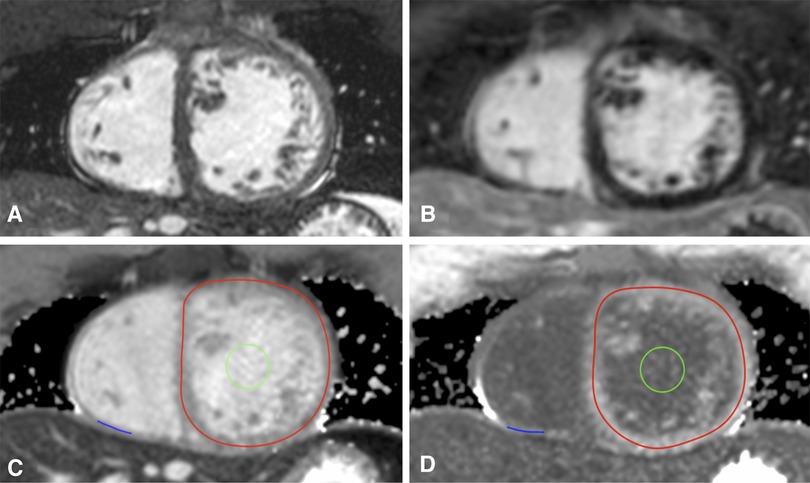
Figure 1. T1 mapping in congenitally corrected transposition of the great arteries. Corresponding cine (A) and late gadolinium enhancement images (B) as well as T1 maps in midventricular short axis before (C) and after contrast application (D) are shown. Lines of interest (LOIs) are drawn into the myocardium of the systemic right ventricle (red LOI) and the subpulmonary left ventricle (blue LOI). The blood pool is marked with a green region of interest.
CMR analysis
A commercially available workstation (Philips Viewforum, Best, The Netherlands) was used to analyze LV and RV volumes [end-diastolic volume (EDV), end-systolic volume (ESV), stroke volume (SV)] and ejection fraction (EF) from cine image stacks in axial orientation. Volumetric parameters were indexed to body surface area (BSA). In TGA patients, the ventricle in the subpulmonary position was defined as the LV, and the ventricle in the subaortic position as the (systemic) RV.
T1 maps were generated with the open-source image reconstruction tool MRmap after manual correction for body motion (16), stored as DICOM files, and analyzed with OsiriX Lite. Native and post-contrast blood T1 values were gained from a region of interest placed in the blood pool of the systemic ventricle in subaortic location with exclusion of the papillary muscles and the trabeculae, respectively. For measurements of myocardial T1, a line of interest (LOI) was manually drawn in the center of the RV and LV myocardium as previously described (17). LOIs marked the myocardial circumference of the subaortic ventricle and the thickest part of the free, anterior or inferior wall of the subpulmonary ventricle on T1 maps in midventricular SAX (Figure 1). In the case of better delineation of myocardial walls, T1 maps in four-chamber-view were alternatively used. Native and post-contrast T1 values of myocardium and blood as well as hematocrit were then used to calculate RV ECV and LV ECV. ECV was considered to be elevated at values above the mean plus two standard deviations (SD) of the control ECV (18).
Follow-up
Medical records of TGA patients were reviewed for adverse events since the date of CMR, including supraventricular and ventricular arrhythmias, implantation of a pacemaker or implantable cardioverter defibrillator (ICD), heart failure-related hospitalization, heart transplantation (HTx), and cardiovascular death.
Statistical analysis
Statistical analyses were carried out with SPSS version 25.0 (IBM Corp., Armonk, NY, United States), considering p-values <0.05 to be statistically significant. Data between groups were compared with the Mann–Whitney U test, and within groups using Wilcoxon signed rank test with Bonferroni correction, respectively. For comparisons between categorical variables, Fisher’s exact test was applied. Spearman's correlation coefficient was used to analyze associations between continuous variables. Results are presented as median (range), mean ± SD, or numbers (n) and percentages (%), as appropriate.
Results
Subject characteristics
In total, 13 patients with a systemic RV and a median age of 30.3 (25.2–37.9) years were enrolled. Of those, eight patients had dTGA post atrial redirection (Senning procedure: n = 7, Mustard procedure: n = 1), and five had ccTGA. A summary of patient characteristics is given in Table 1. Healthy controls (n = 15; female: n = 7) had a median age of 24.4 (23.8–29.0) years and were younger than the patient group (p = 0.004). Differences in gender distribution were not seen.
Cardiovascular magnetic resonance
Late gadolinium enhancement
Mild LGE was visible in 75% of the patients (dTGA post Senning: n = 7, ccTGA: n = 2), and was located at the insertion points (n = 5), the inferior (n = 2) or anterior RV wall (n = 1), and the septum (n = 1). Transmural LGE was not observed.
Extracellular volume
LV ECV was higher in patients than in controls [34% (30%–41%) vs. 26% (23%–27%), p < 0.001; Table 2]. Values were increased above the upper limit of normal (>31.5%) in 10/13 patients (77%). In two out of three dTGA patients with normal LV ECV, (sub-) pulmonary stenosis was present, and the remaining patient had ccTGA without stenosis. RV ECV tended to be higher in patients than in controls, yet without statistical significance [29% (27%–32%) vs. 28% (26%–29%), p = 0.316]. Elevated RV ECV (>29.8%) was detected in four patients (31%). LV ECV was significantly higher than RV ECV (p = 0.016) in the patient group. There were no differences in LV ECV and RV ECV between dTGA and ccTGA patients.
ECV and clinical data
LV ECV and RV ECV of patients did not correlate with clinical characteristics including age at CMR, BSA, systolic or diastolic blood pressure, NT-proBNP, maximum oxygen consumption from CPET, and gender distribution.
ECV and CMR measures
Patients with elevated LV ECV had significantly lower LVEF than those with values within the normal range (52 ± 5% vs. 65 ± 4%, p = 0.007; Figure 2). Both LVEF and RVEF were reduced in patients with elevated RV ECV as compared to those with normal values, but these differences were not statistically significant (51 ± 2% vs. 56 ± 3%, and 39 ± 5% vs. 46 ± 4%, p = 0.371, respectively). LV ECV and RV ECV did not differ between patients with and those without LGE (p = 0.482 and 0.145, respectively). Among patients with positive LGE in the systemic RV, 67% (n = 6) had elevated LV ECV (p = 0.509). There were no differences in the presence of LGE between those with elevated RV ECV and those without (p = 0.509).
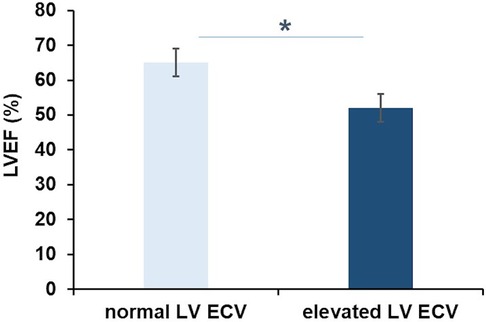
Figure 2. Extracellular volume and left ventricular function in transposition of the great arteries. Patients with elevated left ventricular extracellular volume (LV ECV) had significantly lower LV ejection fraction (LVEF) than patients with normal LV ECV (52 ± 5 vs. 65 ± 4%; *p = 0.007).
Follow-up
Median follow-up time was 5.1 (2.5–8.2) years. One patient was lost to follow-up. Adverse events were observed in 6/12 patients (50%). Of those, four patients (67%) had elevated LV ECV, with additionally elevated RV ECV in two patients. Among those with dTGA post Senning and elevated LV ECV, one patient had isolated non-sustained ventricular tachycardia documented during Holter-ECG. The two patients with elevated LV ECV and RV ECV developed severe dysfunction of the systemic RV and underwent ICD implantation, in one followed by recurrent atrial and ventricular arrhythmias and progressing heart failure, hospitalization, and cardiovascular death 4.0 years after CMR. One patient with ccTGA and elevated LV ECV developed severe heart failure leading to hospitalization and HTx. In those with normal LV ECV, adverse events included hospitalization due to cardiac decompensation in one patient with dTGA post Senning, and complete heart block requiring pacemaker implantation in one patient with ccTGA.
Discussion
In our study, increased LV ECV in TGA patients as compared to controls indicates the presence of diffuse myocardial fibrosis in the subpulmonary LV in the setting of a systemic RV. LV ECV was elevated above the upper limit of normal in the majority of TGA patients and was associated with a reduction in LV function. LV ECV was higher than RV ECV in this cohort.
It has been shown that focal myocardial fibrosis visualized with LGE imaging in the systemic RV correlates with ventricular dysfunction, cardiac arrhythmias, exercise intolerance, and clinical deterioration in patients with dTGA post atrial redirection (7–10) and ccTGA (8). However, the ability of LGE to assess diffuse fibrotic lesions is restricted, and only a few studies have reported on CMR T1 mapping-derived measures of diffuse myocardial fibrosis in TGA (11–14). In the study by Plymen et al., septal ECV was significantly higher in 14 dTGA patients post Senning or Mustard procedure than in age- and gender-matched controls, and was positively related to NT-proBNP and negatively to the chronotropic index during CPET. ECV of the RV wall was not measured for technical reasons (13). Broberg et al. found significantly higher levels of diffuse myocardial fibrosis in association with NT-proBNP in the systemic RV of 53 patients with dTGA post atrial redirection or ccTGA. In that cohort, elevated RV ECV was associated with adverse clinical outcomes, suggesting a role of fibrosis in the development of heart failure (11). Both studies did not report ECV of the subpulmonary LV (11, 13). We evaluated diffuse myocardial fibrosis in the systemic RV as well as in the subpulmonary LV and found significantly higher LV ECV in TGA patients as compared to controls. The majority of patients (77%) had elevated LV ECV and had significantly lower LVEF than those with normal ECV values. Given that conventional heart failure therapies generally fail to improve RV function significantly (19), we anticipate that our findings will stimulate further research on the impact of the LV on morbidity and mortality, and on its role as a potential therapeutic target. The assessment of diffuse myocardial fibrosis by ECV may be useful in selecting patients who may potentially benefit from pulmonary artery banding by improving RV function and tricuspid regurgitation, thus bridging to transplantation or delaying listing for transplantation (20), or by training the LV of ccTGA patients for double switch operation. Furthermore, in our patient group, LV ECV was significantly higher than RV ECV. This is in line with Shehu et al. and Cheung et al. describing greater LV ECV than RV ECV in 10 and 31 dTGA patients post Senning or Mustard, respectively (12, 14). We agree with Cheung et al. that the finding of a higher fibrotic burden in the subpulmonary LV than in the systemic RV is intriguing and that the cause is not immediately apparent. Chronic volume unloading of the eccentrically compressed LV in relation to septal shift and LV diastolic dysfunction, and postcapillary pulmonary hypertension have been discussed as potential contributors, yet the role of these factors in the development of LV fibrotic remodeling remains to be studied further (14).
Cheung et al. in addition to elevated LV ECV also detected higher RV ECV values in the TGA cohort, although with greater involvement of the subpulmonary LV (14). In our study, a trend toward higher RV ECV in patients than in controls was seen, albeit without statistical significance. We found RV ECV to be elevated in almost one-third of the patients (31%). Both RVEF and LVEF were lower than in those with normal RV ECV values, but again these differences were not statistically significant. Apart from technical-methodological differences, the younger age of our TGA cohort in comparison to the abovementioned work by Broberg et al. and Cheung et al. may be contributive to the discrepancies in RV ECV between the studies. Furthermore, we speculate that due to the relatively small sample size, our study may have been underpowered to achieve statistical significance with regard to the differences in RV ECV between patients and controls and the association with impaired biventricular function. Future long-term studies on larger cohorts are required to substantiate our speculations and explore the role of age in the development of diffuse myocardial fibrosis in TGA.
Interestingly, we saw both LV ECV and RV ECV to be elevated in the two dTGA patients requiring ICD implantation due to a severe decline in RV function during follow-up, followed by cardiovascular death in one patient. This points to the importance of interventricular interactions also on the extracellular matrix level, and in turn underlines the potential value of both systemic and subpulmonary myocardial ECV as non-invasive markers for prognosis, risk stratification, and monitoring of disease and therapy in TGA. Moreover, we suspect that the mild, but frequently present LGE in 75% of the TGA patients reflects fibrosis burden that may contribute to the RV dysfunction seen in our cohort. Among patients with positive LGE in the systemic RV, 67% had elevated LV ECV, again pointing to interactions between systemic and subpulmonary ventricles on the tissue level. However, our data in relation to LGE were not statistically significant, which may be explained by the limited number of patients included and the consequent lack of power. Therefore, further research to support our results and their prognostic implications is warranted. The importance of ventriculo-ventricular-interactions and the role of the subpulmonary LV not only as a contributor to morbidity and mortality but also as a potential therapeutic target in right-sided CHD has been well recognized (21). Further investigations need to address the use of ECV measurements for the management of disease and treatment with the goal of preserving LV function or training the LV for systemic pressure in subaortic position.
Limitations
Our study is limited by the small sample size. Due to the lack of clinical indication for regular CMR examinations with contrast enhancement, longitudinal ECV data were unfortunately not available. Future long-term studies on larger cohorts to confirm our findings are required, and may potentially elucidate further differences between groups and associations between ECV and markers of heart failure. Healthy controls were younger compared to the patient group. However, an age-related increase in ECV is seen in individuals significantly older than the patients included in our study (22) and, thus, should not affect comparability between the two groups. Despite the application of the previously described LOI tool, especially helpful for T1 measurements in thin structures (17), and particular care at placing the LOI in the center of the myocardium of the respective subpulmonary ventricle, partial voluming with blood or fat may have caused potential overestimation of ECV. Given the lack of clinical indication for biopsy, we did not perform histological validation of our results.
Conclusions
In this study, LV ECV was significantly higher in TGA patients than in controls and was associated with LV dysfunction. Our data suggest that ECV measurements may serve as an imaging marker of heart failure in the setting of TGA with a systemic RV. Further research is necessary to evaluate the prognostic implications and the potential role of ECV in non-invasively monitoring disease progression and guiding therapy, aiming to maintain LV function or train the LV for subaortic location in TGA patients from infancy to adulthood.
Data availability statement
The raw data supporting the conclusions of this article will be made available by the authors, without undue reservation.
Ethics statement
The studies involving human participants were reviewed and approved by the Ethics Committee of the Charité—Universitätsmedizin Berlin. The patients/participants provided their written informed consent to participate in this study. Written informed consent was obtained from the individual(s) for the publication of any potentially identifiable images or data included in this article.
Author contributions
NA-W-M: conception and design of the study, acquisition, analysis and interpretation of data, and drafting the manuscript. TFdS: implementation of LOI tool. TK and FB: conception and design of the study. DM: conception and design of the study, interpretation of data. All authors contributed to manuscript revision, read, and approved the final manuscript.
Funding
This study has received funding from the German Research Foundation (DFG ME 3508/4). NA-W-M was supported by a DZHK (German Centre for Cardiovascular Research) Excellence Grant (FKZ 81X3100302).
Acknowledgments
We thank Manuela Bauer for patient care, Alireza Khasheei for assistance in performing high-quality CMR examinations, and Sanaz Rastin, Frédéric Muench, and Sevim Yilmaz for support in data acquisition and analysis.
Conflict of interest
The authors declare that the research was conducted in the absence of any commercial or financial relationships that could be construed as a potential conflict of interest.
Publisher's note
All claims expressed in this article are solely those of the authors and do not necessarily represent those of their affiliated organizations, or those of the publisher, the editors and the reviewers. Any product that may be evaluated in this article, or claim that may be made by its manufacturer, is not guaranteed or endorsed by the publisher.
References
1. Marelli AJ, Mackie AS, Ionescu-Ittu R, Rahme E, Pilote L. Congenital heart disease in the general population: changing prevalence and age distribution. Circulation. (2007) 115(2):163–72. doi: 10.1161/CIRCULATIONAHA.106.627224
2. Warnes CA, Liberthson R, Danielson GK, Dore A, Harris L, Hoffman JI, et al. Task force 1: the changing profile of congenital heart disease in adult life. J Am Coll Cardiol. (2001) 37(5):1170–5. doi: 10.1016/s0735-1097(01)01272-4
3. Verheugt CL, Uiterwaal CS, van der Velde ET, Meijboom FJ, Pieper PG, van Dijk AP, et al. Mortality in adult congenital heart disease. Eur Heart J. (2010) 31(10):1220–9. doi: 10.1093/eurheartj/ehq032
4. Budts W, Roos-Hesselink J, Radle-Hurst T, Eicken A, McDonagh TA, Lambrinou E, et al. Treatment of heart failure in adult congenital heart disease: a position paper of the working group of grown-up congenital heart disease and the Heart Failure Association of the European Society of Cardiology. Eur Heart J. (2016) 37(18):1419–27. doi: 10.1093/eurheartj/ehv741
5. Broberg CS, Burchill LJ. Myocardial factor revisited: the importance of myocardial fibrosis in adults with congenital heart disease. Int J Cardiol. (2015) 189:204–10. doi: 10.1016/j.ijcard.2015.04.064
6. Krieger EV, Valente AM. Heart failure treatment in adults with congenital heart disease: where do we stand in 2014? Heart (British Cardiac Society). (2014) 100(17):1329–34. doi: 10.1136/heartjnl-2014-305667
7. Babu-Narayan SV, Goktekin O, Moon JC, Broberg CS, Pantely GA, Pennell DJ, et al. Late gadolinium enhancement cardiovascular magnetic resonance of the systemic right ventricle in adults with previous atrial redirection surgery for transposition of the great arteries. Circulation. (2005) 111(16):2091–8. doi: 10.1161/01.CIR.0000162463.61626.3B
8. Giardini A, Lovato L, Donti A, Formigari R, Oppido G, Gargiulo G, et al. Relation between right ventricular structural alterations and markers of adverse clinical outcome in adults with systemic right ventricle and either congenital complete (after Senning operation) or congenitally corrected transposition of the great arteries. Am J Cardiol. (2006) 98(9):1277–82. doi: 10.1016/j.amjcard.2006.05.062
9. Ladouceur M, Baron S, Nivet-Antoine V, Maruani G, Soulat G, Pereira H, et al. Role of myocardial collagen degradation and fibrosis in right ventricle dysfunction in transposition of the great arteries after atrial switch. Int J Cardiol. (2018) 258:76–82. doi: 10.1016/j.ijcard.2018.01.100
10. Rydman R, Gatzoulis MA, Ho SY, Ernst S, Swan L, Li W, et al. Systemic right ventricular fibrosis detected by cardiovascular magnetic resonance is associated with clinical outcome, mainly new-onset atrial arrhythmia, in patients after atrial redirection surgery for transposition of the great arteries. Circ Cardiovasc Imaging. (2015) 8(5):e002628. doi: 10.1161/CIRCIMAGING.114.002628
11. Broberg CS, Valente AM, Huang J, Burchill LJ, Holt J, Van Woerkom R, et al. Myocardial fibrosis and its relation to adverse outcome in transposition of the great arteries with a systemic right ventricle. Int J Cardiol. (2018) 271:60–5. doi: 10.1016/j.ijcard.2018.04.089
12. Shehu N, Meierhofer C, Messroghli D, Mkrtchyan N, Martinoff S, Ewert P, et al. Diffuse fibrosis is common in the left, but not in the right ventricle in patients with transposition of the great arteries late after atrial switch operation. Int J Cardiovasc Imaging. (2018) 34(8):1241–8. doi: 10.1007/s10554-018-1338-9
13. Plymen CM, Sado DM, Taylor AM, Bolger AP, Lambiase PD, Hughes M, et al. Diffuse myocardial fibrosis in the systemic right ventricle of patients late after Mustard or Senning surgery: an equilibrium contrast cardiovascular magnetic resonance study. Eur Heart J Cardiovasc Imaging. (2013) 14(10):963–8. doi: 10.1093/ehjci/jet014
14. Cheung YF, Lam WWM, So EKF, Chow PC. Differential myocardial fibrosis of the systemic right ventricle and subpulmonary left ventricle after atrial switch operation for complete transposition of the great arteries. Int J Cardiol Heart Vasc. (2020) 30:100612. doi: 10.1016/j.ijcha.2020.100612
15. Messroghli DR, Greiser A, Frohlich M, Dietz R, Schulz-Menger J. Optimization and validation of a fully-integrated pulse sequence for modified look-locker inversion-recovery (MOLLI) T1 mapping of the heart. J Magn Reson Imaging. (2007) 26(4):1081–6. doi: 10.1002/jmri.21119
16. Messroghli DR, Rudolph A, Abdel-Aty H, Wassmuth R, Kuhne T, Dietz R, et al. An open-source software tool for the generation of relaxation time maps in magnetic resonance imaging. BMC Med Imaging. (2010) 10:16. doi: 10.1186/1471-2342-10-16
17. Al-Wakeel-Marquard N, Ferreira da Silva T, Jeuthe S, Rastin S, Muench F, O H-Ici D, et al. Measuring myocardial extracellular volume of the right ventricle in patients with congenital heart disease. Sci Rep. (2021) 11(1):2679. doi: 10.1038/s41598-021-81440-z
18. Messroghli DR, Moon JC, Ferreira VM, Grosse-Wortmann L, He T, Kellman P, et al. Clinical recommendations for cardiovascular magnetic resonance mapping of T1, T2, T2* and extracellular volume: a consensus statement by the society for cardiovascular magnetic resonance (SCMR) endorsed by the European association for cardiovascular imaging (EACVI). J Cardiovasc Magn Reson. (2017) 19(1):75. doi: 10.1186/s12968-017-0389-8
19. Roche SL, Redington AN. Right ventricle: wrong targets? Another blow for pharmacotherapy in congenital heart diseases. Circulation. (2013) 127(3):314–6. doi: 10.1161/CIRCULATIONAHA.112.155887
20. Poirier NC, Yu JH, Brizard CP, Mee RB. Long-term results of left ventricular reconditioning and anatomic correction for systemic right ventricular dysfunction after atrial switch procedures. J Thorac Cardiovasc Surg. (2004) 127(4):975–81. doi: 10.1016/j.jtcvs.2003.10.024
21. Tretter JT, Redington AN. The forgotten ventricle? The left ventricle in right-sided congenital heart disease. Circ Cardiovasc Imaging. (2018) 11(3):e007410. doi: 10.1161/CIRCIMAGING.117.007410
22. Liu CY, Liu YC, Wu C, Armstrong A, Volpe GJ, van der Geest RJ, et al., Evaluation of age-related interstitial myocardial fibrosis with cardiac magnetic resonance contrast-enhanced T1 mapping: MESA (multi-ethnic study of atherosclerosis). J Am Coll Cardiol. (2013) 62(14):1280–7. doi: 10.1016/j.jacc.2013.05.078
Keywords: transposition of the great arteries, systemic right ventricle, cardiovascular magnetic resonance, extracellular volume, myocardial fibrosis, heart failure
Citation: Al-Wakeel-Marquard N, Ferreira da Silva T, Berger F, Kuehne T and Messroghli DR (2022) Myocardial extracellular volume is a non-invasive tissue marker of heart failure in patients with transposition of the great arteries and systemic right ventricle. Front. Pediatr. 10:949078. doi: 10.3389/fped.2022.949078
Received: 20 May 2022; Accepted: 26 September 2022;
Published: 7 November 2022.
Edited by:
Laura Muiño Mosquera, Ghent University Hospital, BelgiumReviewed by:
Bing Jia, Fudan University, ChinaGianfranco Butera, Bambino Gesù Children's Hospital (IRCCS), Italy
© 2022 Al-Wakeel-Marquard, Ferreira da Silva, Berger, Kuehne and Messroghli. This is an open-access article distributed under the terms of the Creative Commons Attribution License (CC BY). The use, distribution or reproduction in other forums is permitted, provided the original author(s) and the copyright owner(s) are credited and that the original publication in this journal is cited, in accordance with accepted academic practice. No use, distribution or reproduction is permitted which does not comply with these terms.
*Correspondence: Nadya Al-Wakeel-Marquard alwakeel@dhzb.de
Specialty Section: This article was submitted to Pediatric Cardiology, a section of the journal Frontiers in Pediatrics