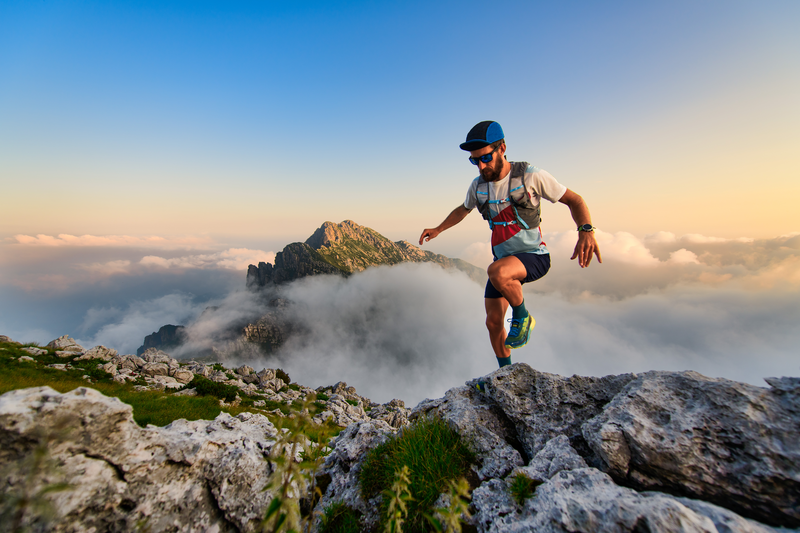
95% of researchers rate our articles as excellent or good
Learn more about the work of our research integrity team to safeguard the quality of each article we publish.
Find out more
ORIGINAL RESEARCH article
Front. Pediatr. , 25 October 2022
Sec. Children and Health
Volume 10 - 2022 | https://doi.org/10.3389/fped.2022.941651
As a heavy metal, lead is a common toxic agent. Its accumulation in the body is harmful to physical health, particularly in children and adolescents. Studies have reported that folate may play a protective role in lead exposure. An association between serum folate concentrations (SFC) and blood lead levels (BLL) has been documented in adults, but studies in adolescents are limited. This study investigated the relationship between SFC and BLL in American adolescents. This cross-sectional study collected relevant data on both SFC and BLL of 5,195 adolescents in the NHANES database from 2007 to 2018. Multivariable linear regressions and smooth curve fittings were adopted to evaluate the correlation between BLL and SFC. After adjusting potential confounders, we found negative relationships between BLL and SFC [β = −0.0041 (−0.0063, −0.0019)], and the associations were significant in non-Hispanic Whites, Mexican Americans, and other races but not significant in non-Hispanic blacks (P = 0.139). Furthermore, the negative trends were significant in adolescents aged 16–19 years and females aged 12–15 years but insignificant in males aged 12–15 years (P = 0.172). Therefore, these findings provide a basis for future research on the mechanism of folate in regulating blood lead levels.
With the rapid development of industrial globalization and technology, heavy metal pollution caused by these processes has become a global public health concern. Heavy metals have bioaccumulation effects and persist in the biosphere (1). Therefore, almost all organisms will be threatened by their toxicity. Lead, as one of the common heavy metals, widely exists in our living environment and can enter the human body in many forms, such as drinking water, air, food, and daily necessities (2). Lead can also cause damage to many systems of the human body, among which hematopoietic, nervous, circulatory, and urinary systems are more sensitive to lead toxicity (3–5). Children and adolescents are at the stage of growth and development, with strong absorption capacity and low elimination rates of lead, and are more vulnerable to lead toxicity during these ages (6). In the past 50 years, the United States has significantly reduced childhood exposure to lead by not using leaded gasoline and paint (7). However, many children are still at risk of exposure and lead poisoning. At present, no blood lead level is considered safe. According to the latest data from the 2011–2014 national health and Nutrition Survey (NHANES), the CDC has the reference value of children's blood lead from 5 μg/dl decreased to 3.5 μg/dl (8). Unfortunately, low blood lead concentrations (mean 1.7 ± 0.3 μg/dl) can still harm children's intellectual development and overall blood function (9).
Folic acid is an essential water-soluble vitamin for the human body. It can not be synthesized by the human body and is obtained through food and vitamin/mineral consumption. Common foods rich in folic acid include leafy vegetables, beans, nuts, fruits, and berries (10). Studies have shown that a certain amount of folic acid intake by adolescents has a positive effect on improving cognition, promoting the development of the nervous system, and reducing anemia (11–13). Epidemiological studies show that the serum unsaturated fatty acids and folic acids of adolescents with depression are lower than those of adolescents with normal development (14). In another study, after long-term oral administration of 3.5 mg folic acid and 60 or 120 mg iron every week, the average hemoglobin in adolescent girls continued to increase with added treatment time (15).
The harm of heavy metals to the human body is primarily caused by oxidative stress (16). Previous studies have found that glutathione (GSH) can alleviate the oxidative stress induced by heavy metals and combine with heavy metals to form a complex, increase the excretion of heavy metals, and alleviate the harm of heavy metals to the human body (17). GSH is the precursor of cysteine. Interestingly, folic acid can directly pass through the trans-sulfur pathway and participate in the anabolism of cysteine and that in turn might increase GSH levels (18). Therefore, folic acid may participate in the detoxification process of heavy metals. A previous cross-sectional study has found negative relationship between lead exposure and serum folate in American adults (19). However, the research on the relationship between serum folate concentrations (SFC) and blood lead levels (BLL) in adolescents is limited. Therefore, exploring the relationship between BLL and SFC in adolescents is necessary, which may be significant in preventing lead poisoning in adolescents. This study used a nationally representative cross-sectional sample to explore the relationship between SFC and BLL in American adolescents. Furthermore, we further explored the relationship between SFC and BLL in the grouping of race, sex, and age to evaluate potential interactions between these factors.
In this study, data were collected from the National Health and Nutrition Examination Survey (NHANES) public database, which was part of the Centers for Disease Control and Prevention (CDC) in the United States. This database used a complex, stratified, multistage sample design to provide nationally representative data. Sources of data included interviews, medical examinations, and laboratory tests. Further methodological details about the NHANES survey were available at www.cdc.gov/nchs/nhanes/.
A total of 59,842 subjects from six continuous cycles of the NHANES database were collected from 2007 to 2018. According to the inclusion criteria (Figure 1), 5,271 participants aged 12–19 years with complete data on SFC and BLL were included. Of the remaining 5,271 participants, we further excluded the population with missing laboratory data (n = 76). Finally, data from 5,195 participants were included in the study.
Figure 1. Flow chart of participants from NHANES included in the study. AST, aspartate aminotransferase, ALT, alanine aminotransferase.
The NCHS Research Ethics Review Board approved all the procedures (20), and all the participants provided written informed consent. For participants under 18 years old, their parents/guardians provided informed consent.
Whole blood specimens were processed, stored, and shipped to the Division of Laboratory Sciences, National Center for Environmental Health, and Centers for Disease Control and Prevention for analysis.
SFC were measured using a microbiological assay (MBA) in NHANES from 2007 to 2010 (21).
From 2011 to 2018, SFC were measured by isotope-dilution high-performance liquid chromatography coupled to tandem mass spectrometry (LC-MS/MS). For total serum folate, the weighted distributions of the NHANES 2011–2012 data measured by LC-MS/MS were similar to the NHANES 2007–2008 and 2009–2010 data measured by MBA (22).
The method directly measured the lead content of whole blood specimens using inductively coupled plasma dynamic reaction cell mass spectrometry (ICP-DRC-MS) after a simple dilution sample preparation step (23). The lower detection limit for blood lead in NHANES from 2013 to 2018 was 0.07 µg/dl, and the lower detection limit for blood lead in NHANES from 2011 to 2012 was 0.25 µg/dl. (In cases where the result was below the detection limit, the value for that variable was the detection limit divided by the square root of 2.)
The covariates were selected based on established associations (19, 24) and/or plausible biological relations to the variables examined. The following covariates were included in our study: age, sex, race, BMI, PIR (Ratio of family income to poverty), AST (aspartate aminotransferase), ALT (alanine aminotransferase), total cholesterol, blood urea nitrogen, serum creatinine, serum cotinine, and folate supplements. Details of these covariate acquisition processes were available at www.cdc.gov/nchs/nhanes/.
The body mass index (BMI) was categorized into three groups according to WHO standards: (i) <25 kg/m2, (ii) 25–30 kg/m2, and (iii) ≥30 kg/m2. The age of the participants was categorized into two groups: (i) 12–15 years and (ii) 16–19 years. Race was classified as “non-Hispanic Black”, “non-Hispanic White”, “Mexican American”, or “Other race”. PIR(Ratio of family income to poverty), AST, ALT, total cholesterol, blood urea nitrogen, serum creatinine, serum cotinine, and folate supplements were designed as continuous variables. In stratified analyses, these continuous variables were divided into three subgroups on average.
In this study, all continuous variables were expressed as mean ± standard deviation, and the difference test between groups was calculated by a weighted linear regression model. Meanwhile, categorical variables were expressed as percentages, and the difference test of groups was calculated by weighted chi-square. Logistic regression models were applied to estimate the independent correlation between BLL and SFC before or after the adjustment of confounders. In each model, a weight suggested by the CDC was used to consider the oversampling of minorities and thus provide an accurate estimate of effects on the population. Weighted multivariate logistic regression models were used to explore the relationships between BLL and SFC. For this study, three sequential models (model 1: non-adjusted model; model 2: adjusted for age, sex, and race; model 3: adjusted for all covariates) were used to control potential confounders. A generalized additive model and smooth curve fitting were used to show the linear and non-linear relationship between the BLL and SFC. A P-value (P) ≤ 0.05 indicated a difference achieving statistical significance. The investigations were carried out using Empower software (www.empowerstats.com; X/Y Solutions, Inc., Boston, MA) and R version 3.4.3 (http://www.R-project.org, The R Foundation).
Five thousand one hundred ninety-five adolescents aged 12–19 were included in this study, including 48.63% males and 51.37% females. Among them, 56.30% were non-Hispanic Whites, 13.47% were non-Hispanic Blacks, 14.33% were Mexican Americans, and 15.90% were other races. The average BLL and SFC were 0.70 μg/dl and 18.88 ng/ml, respectively. Adolescents with lower serum total folate concentrations were more likely to be male, non-Hispanic Black, had higher serum cotinine levels, and had a lower PIR. As expected, participants who took folate supplements had higher folate levels. The lead levels in the blood decreased with the increase in serum folate concentrations (Table 1). Furthermore, blood lead concentrations were higher in non-Hispanic Black populations, those with higher serum cotinine levels, higher serum creatinine, and those from lower PIR (Supplementary Table S1).
As shown in Table 2, in multivariate-adjusted linear regression analysis, the SFC was negatively associated with BLL across the three models (Model 1: β = −0.0040, 95% CI: −0.0061, −0.0019; Model 2: β = −0.0039, 95% CI: −0.0060, −0.0017; and Model 3: β = −0.0041, 95% CI: −0.0063, −0.0019). When folate concentration was classified as a categorical variable, the highest tertile was significantly negatively associated with the BLL in three models.
Table 2. Regression coefficient (95% confidence interval) for BLL by serum SFC in adolescents aged 12–19 years in the 2007–2018 NHANES survey (n = 5,195).
When stratifying by age, sex or race, the negative correlation between SFC and BLL was significant in each subgroup of age and sex. However, in race subgroups, the relationship between SFC and BLL was significant in non-Hispanic Whites [−0.0037 (−0.0073, −0.0002)], Mexican Americans [−0.0065 (−0.0116, −0.0014)] and other races [−0.0076 (−0.0137, −0.0015)], but not significant in non-Hispanic black [−0.0034 (−0.0078, 0.0011)] (Table 3). Additionally, the negative trends were significant (P-trends <0.05) in adolescents aged 16–19 and female adolescents aged 12–15, while no associations were found between SFC and BLL in male adolescents aged 12–15 (Table 4). Furthermore, weighted generalized additive models and smooth curve fittings were used to visually assess the relationships between BLL and SFC, which were presented in Figure 2. The results showed that BLL was negatively associated with the SFC in the adjusted models.
Figure 2. Smooth curve fitting of serum folate concentrations and blood lead levels. (A) Each black point represents a sample. (B) The area between two blue dotted lined is expressed as a 95% CI. The solid red line represents the smooth curve fit between the variables.Age, sex, race, ratio of family income to poverty, body mass index, aspartate aminotransferase, alanine aminotransferase, total cholesterol, blood urea nitrogen, serum creatinine, serum cotinine, folate supplements were adjusted.
Table 3. Association between SFC and BLL for stratified analyses according to baseline characteristics.
The main objective of this study was to investigate whether SFC is independently associated with BLL in adolescents. This study suggests a negative association between serum folate concentrations and blood lead levels among adolescent participants aged 12–19 years from NHANES 2007–2018 survey. The association became more robust when the highest serum folate tertile group was compared with the lowest tertile group. To the best of our knowledge, our research is the first report investigating the relationship between lead levels and serum folate status in adolescents.
Previous studies have explored the association between lead and folate. A prospective birth cohort study suggests that adequate maternal folate status may mitigate the obesity risk associated with maternal lead exposure (25). Additionally, in vitro studies found folate deficiency to enhance DNA damage caused by exposure to heavy metals such as lead and chromium (26). Although the mechanism between lead and folate status was unclear, these findings may be attributed to the oxidative stress of lead and antioxidant properties of folate. Lead can easily cross the cell membrane, induce oxidative stress by disrupting the antioxidant system, and deplete intracellular glutathione (an important antioxidant) (27, 28). In contrast, folate can participate in glutathione anabolism through the transsulfuration pathway (29), which may reduce the toxicity caused by lead. Moreover, folate has also been reported to improve lead excretion and make it more difficult for lead to bind to blood elements, thereby reducing lead toxicity (30). Furthermore, animal experiments suggest that folate may antagonize the testicular toxicity of lead by increasing relative testicular weight and testosterone secretion, reducing oxidative stress and inflammatory responses, and down-regulating the NF-KB signaling pathway (31). Therefore, the above studies show that folate may participate in the metabolism of lead in the blood or reduce the harmful consequences of lead exposure. However, the underlying mechanism in detail requires further investigation.
In our study, the average BLL of the adolescents was 0.70 ug/dl, which was far below the safe levels used by the WHO and CDC (32). However, studies have shown that even relatively low levels of lead exposure are still associated with public health problems. Notably, a large body of literature has examined the effects of low levels of exposure of lead on the health of children and young adolescents. R.G. Lucchini et al. first demonstrated significant adverse cognitive effects of lead at low BPb levels (average BPb was 1.71 mg/dl) in adolescents aged 11–14 years (33). Low blood lead concentrations (1.8 ug/dl) are also associated with more autistic behaviors in school-age children (34). Meanwhile, Cho et al. found that children with a mean blood lead level of 1.9 μg/dl had symptoms of attention deficit hyperactivity disorder (ADHD) (35). An N-shaped curve was found between BLL and total BMD(bone mineral density), subtotal BMD and limb BMD for males and females at low-dose lead exposure (0.84 ug/dl) (36). In addition, when studying the relationship between blood lead and cardiovascular risk factors, Xu (24) found a positive correlation between BLL (geometric mean of 1.17 ug/dl) and serum LDL-C (low-density lipoprotein cholesterol, a biomarker associated with cardiovascular disease) in American adolescents. Therefore, the health effects of low levels of lead exposure cannot be ignored. Notably, further reducing BLL and preventing lead exposure is of great significance to the health of adolescents.
Currently, chelation therapy is the primary treatment that can aid in acute Pb poisoning (37). However, chelating agents can increase essential metals' excretion during treatment and have other potential side effects for children and adolescents (38). Therefore, it is essential to identify non-invasive natural treatments or compounds that might reduce BLL in children and adolescents. Our study showed a negative correlation between SFC and BLL, which indicates that folate may be involved in the detoxification of lead and may prevent low-dose chronic lead poisoning. Further prospective cohort studies are needed to confirm these findings.
Our study found that non-Hispanic blacks and subjects with low PIR have higher BLL and are more vulnerable to lead exposure. The possible explanation for these findings is due to social inequities that place ethnic minority adolescents at a higher risk for lead exposure (39, 40). On the other hand, poverty is another risk factor for lead exposure. Adolescents living in poverty are more likely to be exposed to lead-contaminated soil and to live near industrial facilities that emit lead pollutants (38). We also found that non-Hispanic blacks and subjects with low PIR have lower SFC, which is consistent with the conclusion in another NHANES cross-sectional study (41) that found Non-Hispanic blacks and subjects with lower socioeconomic status were accounted for the poor nutritional status of most biomarkers, including folate.
In subgroup analysis, although the negative association between BLL and SFC was insignificant in non-Hispanic blacks, we still found that non-Hispanic blacks with lower serum folate concentrations were likelier to have higher BLL. Previous cross-sectional studies found a negative association between BLL and SFC in different populations (19, 30). However, few studies have analyzed whether this relationship exists in different ethnic groups. This finding in the study suggests that the relationship may differ between races. We speculate that the variability may be due to other potentially confounding factors which are difficult to assess (e.g., exercise and dietary patterns). Among adolescents aged 12–15, we found that the negative trends were significant between BLL and SFC in females but not males. The sources of the sex differences between BLL and SFC are not known. It could be that hormonal differences between males and females mediated these findings. Alternatively, this could be a chance effect. Future studies should attempt to replicate these findings and if significant further explore these possibilities.
This is the first study to analyze adolescents' relationship between SFC and BLL. In this study, we selected a representative American adolescent population and conducted further stratified analyses, which is the advantage of our research. However, this study also has some noted limitations. Firstly, the current study is a cross-sectional study, and it cannot explain causal relationships. Further prospective research is needed in the future. Secondly, as in all observational studies, residual confounding is still possible despite our adjustment and stratification for various potential confounders. Thirdly, our study did not include the indicators used to measure the protective role of folate in lead exposure. For example, the increase in blood lead concentration can lead to anemia (42), and the hemoglobin content can reflect whether there is anemia. Therefore, it is unknown whether serum folate exerts a protective effect on the anemia caused by lead. Further research is needed in order to better understand whether and how serum folate decreases BLLs in adolescents and its impact on other important health conditions that have been associated with chronic lead exposure such as anemia.
In conclusion, we found a negative correlation between SFC and BLL in American adolescents.
This association was insignificant in non-Hispanic blacks and males aged 12–15 years. These findings may provide new insights into the treatment of lead poisoning and the study of reducing blood lead levels in adolescents. Furthermore, potential differences in sex and races need more studies in the future to better explain these relationships and apply preventative measures.
The datasets presented in this study can be found in online repositories. The names of the repository/repositories and accession number(s) can be found below: www.cdc.gov/nchs/nhanes/.
Written informed consent was obtained from the individual(s), and minor(s)' legal guardian/next of kin, for the publication of any potentially identifiable images or data included in this article.
HH and ZZ designed research; ML conducted research; HH analyzed data and wrote the paper. All authors contributed to the article and approved the submitted version.
The authors thank AiMi Academic Services (www.aimieditor.com) for English language editing and review services.
The authors declare that the research was conducted in the absence of any commercial or financial relationships that could be construed as a potential conflict of interest.
All claims expressed in this article are solely those of the authors and do not necessarily represent those of their affiliated organizations, or those of the publisher, the editors and the reviewers. Any product that may be evaluated in this article, or claim that may be made by its manufacturer, is not guaranteed or endorsed by the publisher.
The Supplementary Material for this article can be found online at: https://www.frontiersin.org/articles/10.3389/fped.2022.941651/full#supplementary-material.
1. Long X, Chen R, Tan J, Lu Y, Wang J, Huang T, et al. Electrochemical recovery of cobalt using nanoparticles film of copper hexacyanoferrates from aqueous solution. J Hazard Mater. (2020) 384:121252. doi: 10.1016/j.jhazmat.2019.121252
2. Jomova K, Valko M. Advances in metal-induced oxidative stress and human disease. Toxicology. (2011) 283(2–3):65–87. doi: 10.1016/j.tox.2011.03.001
3. Reuben A. Childhood lead exposure and adult neurodegenerative disease. J Alzheimers Dis. (2018) 64(1):17–42. doi: 10.3233/JAD-180267
4. Gundacker C, Forsthuber M, Szigeti T, Kakucs R, Mustieles V, Fernandez MF, et al. Lead (pb) and neurodevelopment: a review on exposure and biomarkers of effect (BDNF, HDL) and susceptibility. Int J Hyg Environ Health. (2021) 238:113855. doi: 10.1016/j.ijheh.2021.113855
5. Abadin H, Ashizawa A, Stevens YW, Llados F, Diamond G, Sage G, et al. Toxicological profile for lead. Atlanta, GA: Agency for Toxic Substances and Disease Registry (US) (2007).
6. Bellinger DC. Assessing environmental neurotoxicant exposures and child neurobehavior: confounded by confounding? Epidemiology. (2004) 15(4):383–4. doi: 10.1097/01.ede.0000129525.15064.a4
7. Lanphear B. Still treating lead poisoning after all these years. Pediatrics. (2017) 140(2):e20171400. doi: 10.1542/peds.2017-1400
8. Tsoi MF, Cheung CL, Cheung TT, Cheung BM. Continual decrease in blood lead level in Americans: united States national health nutrition and examination survey 1999–2014. Am J Med. (2016) 129(11):1213–8. doi: 10.1016/j.amjmed.2016.05.042
9. Alvarez-Ortega N, Caballero-Gallardo K, Olivero-Verbel J. Low blood lead levels impair intellectual and hematological function in children from cartagena, Caribbean coast of Colombia. J Trace Elem Med Biol. (2017) 44:233–40. doi: 10.1016/j.jtemb.2017.08.006
10. Htet MK, Fahmida U, Thurnham DI, Hlaing LM, Akib A, Utomo B, et al. Folate and vitamin B12 status and dietary intake of anaemic adolescent schoolgirls in the delta region of Myanmar. Br J Nutr. (2016) 116(Suppl 1):S36–41. doi: 10.1017/S0007114515001609
11. Balashova OA, Visina O, Borodinsky LN. Folate action in nervous system development and disease. Dev Neurobiol. (2018) 78(4):391–402. doi: 10.1002/dneu.22579
12. Roche ML, Samson KLI, Green TJ, Karakochuk CD, Martinez H. Perspective: weekly iron and folic acid supplementation (WIFAS): a critical review and rationale for inclusion in the essential medicines list to accelerate Anemia and neural tube defects reduction. Adv Nutr. (2021) 12(2):334–42. doi: 10.1093/advances/nmaa169
13. Lohner S, Fekete K, Berti C, Hermoso M, Cetin I, Koletzko B, et al. Effect of folate supplementation on folate status and health outcomes in infants, children and adolescents: a systematic review. Int J Food Sci Nutr. (2012) 63(8):1014–20. doi: 10.3109/09637486.2012.683779
14. Tsuchimine S, Saito M, Kaneko S, Yasui-Furukori N. Decreased serum levels of polyunsaturated fatty acids and folate, but not brain-derived neurotrophic factor, in childhood and adolescent females with depression. Psychiatry Res. (2015) 225(1–2):187–90. doi: 10.1016/j.psychres.2014.11.018
15. Tee ES, Kandiah M, Awin N, Chong SM, Satgunasingam N, Kamarudin L, et al. School-administered weekly iron-folate supplements improve hemoglobin and ferritin concentrations in Malaysian adolescent girls. Am J Clin Nutr. (1999) 69(6):1249–56. doi: 10.1093/ajcn/69.6.1249
16. Paithankar JG, Saini S, Dwivedi S, Sharma A, Chowdhuri DK. Heavy metal associated health hazards: an interplay of oxidative stress and signal transduction. Chemosphere. (2021) 262:128350. doi: 10.1016/j.chemosphere.2020.128350
17. Huang S, Ye J, Yu J, Chen L, Zhou L, Wang H, et al. The accumulation and efflux of lead partly depend on ATP-dependent efflux pump-multidrug resistance protein 1 and glutathione in testis sertoli cells. Toxicol Lett. (2014) 226(3):277–84. doi: 10.1016/j.toxlet.2014.02.017
18. Mason JB. Biomarkers of nutrient exposure and status in one-carbon (methyl) metabolism. J Nutr. (2003) 133(Suppl 3(3)):941S–7S. doi: 10.1093/jn/133.3.941S
19. Wu Z, Hu H, Wang C, Wu J, Xiong Y, Fu Y, et al. Association between serum folate levels and blood concentrations of cadmium and lead in US adults. Environ Sci Pollut Res Int. (2022) 29(3):3565–74. doi: 10.1007/s11356-021-15767-5
20. Zipf G, Chiappa M, Porter KS, Ostchega Y, Lewis BG, Dostal J. National health and nutrition examination survey: plan and operations, 1999–2010. Vital Health Stat 1. (2013)(56):1–37.25078429
21. NCHS. National center for health statistics. National health and nutrition examination survey (2011). Available at: https://wwwn.cdc.gov/Nchs/Nhanes/2009-2010/FOLATE_F.htm (Accessed April 26, 2022).
22. NCHS. National center for health statistics.National health and nutrition examination survey (2019). Available at: https://wwwn.cdc.gov/Nchs/Nhanes/2015-2016/FOLFMS_I.htm (Accessed April 26, 2022).
23. CDC. Cadmium, Lead, Manganese, Mercury, and Selenium Lab Procedure Manual. (2018). Available at: https://wwwn.cdc.gov/nchs/data/nhanes/2017-2018/labmethods/PBCD-J-PBY-J-R-MET-508.pdf (Accessed April 26, 2022).
24. Xu C, Shu Y, Fu Z, Hu Y, Mo X. Associations between lead concentrations and cardiovascular risk factors in U.S. Adolescents. Sci Rep. (2017) 7:9121. doi: 10.1038/s41598-017-09701-4
25. Wang G, DiBari J, Bind E, Steffens AM, Mukherjee J, Azuine RE, et al. Association between maternal exposure to lead, Maternal folate Status, and intergenerational risk of childhood overweight and obesity. JAMA Netw Open. (2019) 2(10):e1912343. doi: 10.1001/jamanetworkopen.2019.12343
26. Alimba CG, Dhillon V, Bakare AA, Fenech M. Genotoxicity and cytotoxicity of chromium, copper, manganese and lead, and their mixture in WIL2-NS human B lymphoblastoid cells is enhanced by folate depletion. Mutat Res Genet Toxicol Environ Mutagen. (2016) 798–799:35–47. doi: 10.1016/j.mrgentox.2016.02.002
27. Wyparło-Wszelaki M, Machoń-Grecka A, Wąsik M, Dobrakowski M. Critical aspects of the physiological interactions between lead and magnesium. J Biochem Mol Toxicol. (2022) 36(2):e22964. doi: 10.1002/jbt.22964
28. Naranjo VI, Hendricks M, Jones KS. Lead toxicity in children: an unremitting public health problem. Pediatr Neurol. (2020) 113:51–5. doi: 10.1016/j.pediatrneurol.2020.08.005
29. Morellato AE, Umansky C, Pontel LB. The toxic side of one-carbon metabolism and epigenetics. Redox Biol. (2021) 40:101850. doi: 10.1016/j.redox.2020.101850
30. Solon O, Riddell TJ, Quimbo SA, Butrick E, Aylward GP, Lou Bacate M, et al. Associations between cognitive function, blood lead concentration, and nutrition among children in the central Philippines. J Pediatr. (2008) 152(2):237–43. doi: 10.1016/j.jpeds.2007.09.008
31. Gomaa AM, Abou Khalil NS, Abdel-Ghani MA. The protective role of folic acid against testicular dysfunction in lead-intoxicated rat model. Gen Physiol Biophys. (2017) 36(3):297–308. doi: 10.4149/gpb_2016048
32. Centers for Disease Control and Prevention (CDC). Adult blood lead epidemiology and surveillance–United States, 2005–2007. MMWR Morb Mortal Wkly Rep. (2009) 58(14):365–9.19373197
33. Lucchini RG, Zoni S, Guazzetti S, Bontempi E, Micheletti S, Broberg K, et al. Inverse association of intellectual function with very low blood lead but not with manganese exposure in Italian adolescents. Environ Res. (2012) 118:65–71. doi: 10.1016/j.envres.2012.08.003
34. Kim KN, Kwon HJ, Hong YC. Low-level lead exposure and autistic behaviors in school-age children. Neurotoxicology. (2016) 53:193–200. doi: 10.1016/j.neuro.2016.02.004
35. Cho SC, Kim BN, Hong YC, Shin MS, Yoo HJ, Kim JW, et al. Effect of environmental exposure to lead and tobacco smoke on inattentive and hyperactive symptoms and neurocognitive performance in children. J Child Psychol Psychiatry. (2010) 51(9):1050–7. doi: 10.1111/j.1469-7610.2010.02250.x
36. Li T, Xie Y, Wang L, Huang G, Cheng Y, Hou D, et al. The association between lead exposure and bone mineral density in childhood and adolescence: results from NHANES 1999–2006 and 2011–2018. Nutrients. (2022) 14(7):1523. doi: 10.3390/nu14071523
38. Mohammed TA, Meier CM, Kalvoda T, Kalt M, Rulíšek L, Shoshan MS. Potent cyclic tetrapeptide for lead detoxification. Angew Chem Int Ed Engl. (2021) 60(22):12381–5. doi: 10.1002/anie.202103217
39. Dilworth-Bart JE, Moore CF. Mercy mercy me: social injustice and the prevention of environmental pollutant exposures among ethnic minority and poor children. Child Dev. (2006) 77(2):247–65. doi: 10.1111/j.1467-8624.2006.00868.x
40. Tong S, von Schirnding YE, Prapamontol T. Environmental lead exposure: a public health problem of global dimensions. Bull World Health Organ. (2000) 78(9):1068–77.11019456
41. Wang W, Zhu F, Wu L, Han S, Wu X. Trends in nutritional biomarkers by demographic characteristics across 14 years among US adults. Front Nutr. (2022) 8:737102. doi: 10.3389/fnut.2021.737102
Keywords: serum folate, blood lead, adolescents, NHANES, a cross sectional study
Citation: He H, Zhang Z and Li M (2022) Association between serum folate concentrations and blood lead levels in adolescents: A cross-sectional study. Front. Pediatr. 10:941651. doi: 10.3389/fped.2022.941651
Received: 11 May 2022; Accepted: 5 October 2022;
Published: 25 October 2022.
Edited by:
Nicholas P. Hays, Nestle, SwitzerlandReviewed by:
Mayra Gisel Flores-Montoya, Carleton College, United States© 2022 He, Zhang and Li. This is an open-access article distributed under the terms of the Creative Commons Attribution License (CC BY). The use, distribution or reproduction in other forums is permitted, provided the original author(s) and the copyright owner(s) are credited and that the original publication in this journal is cited, in accordance with accepted academic practice. No use, distribution or reproduction is permitted which does not comply with these terms.
*Correspondence: Huan He aHVhbmhlXzAzMTlAMTYzLmNvbQ==
Specialty Section: This article was submitted to Children and Health, a section of the journal Frontiers in Pediatrics
Disclaimer: All claims expressed in this article are solely those of the authors and do not necessarily represent those of their affiliated organizations, or those of the publisher, the editors and the reviewers. Any product that may be evaluated in this article or claim that may be made by its manufacturer is not guaranteed or endorsed by the publisher.
Research integrity at Frontiers
Learn more about the work of our research integrity team to safeguard the quality of each article we publish.