- 1Academy of Pediatrics, University of South China, Changsha, China
- 2Hunan Children's Hospital, Changsha, China
- 3Department of Cardiology, The Second Xiangya Hospital of Central South University, Changsha, China
Multifocal atrial tachycardia (MAT) is defined as irregular P-P, R-R, and P-R intervals, isoelectric baseline between P waves, and ventricular rate over 100 beats/min. Although the prognosis of pediatric MAT in most patients is favorable, adverse outcomes of MAT have been reported, such as cardiogenic death (3%), respiratory failure (6%), or persistent arrhythmia (7%), due to delayed diagnosis and poorly controlled MAT. Previous studies demonstrated that pediatric MAT is associated with multiple enhanced automatic lesions located in the atrium or abnormal automaticity of a single lesion located in the pulmonary veins via multiple pathways to trigger electrical activity. Recent studies indicated that pediatric MAT is associated with the formation of a re-entry loop, abnormal automaticity, and triggering activity. The occurrence of pediatric MAT is affected by gestational disease, congenital heart disease, post-cardiac surgery, pulmonary hypertension, and infectious diseases, which promote MAT via inflammation, redistribution of the autonomic nervous system, and abnormal ion channels. However, the pathogenesis of MAT needs to be explored. This review is aimed to summarize and analyze the pathogenesis in pediatric MAT.
Introduction
Multifocal atrial tachycardia (MAT) is defined as the presence of 3 or more P wave morphologies, which is characterized by irregular P-P, R-R, and P-R intervals, isoelectric baseline between the P waves, and ventricular rate over 100 beats/min on the electrocardiogram (1–4). MAT accounts for less than 1% of supraventricular tachycardia in pediatric arrhythmia, and 82% of patients diagnosed with MAT in infancy, whose lungs and heart are both immature (3, 5, 6). In previous studies, the main etiologies of MAT are gestational disease, Costello syndrome, congenital heart disease, post-cardiac surgery, cardiac anatomic abnormality, pulmonary arterial hypertension, and infectious diseases. Although the prognosis of pediatric MAT is favorable in most patients, adverse outcomes of MAT have been reported in some children, particularly the patients who are accompanied by HRAS, RYR2, RAF1, and RAS gene mutation (5, 7). The adverse outcomes of MAT include cardiogenic death (3%), respiratory failure (6%), or persistent arrhythmia (7%). Some recent studies demonstrated that pediatric MAT is associated with multiple enhanced automatic lesions located in the atrium or abnormal automaticity of a single lesion located in the pulmonary veins via multiple pathways to trigger electrical activity (4). However, the pathophysiology of pediatric MAT is needed to be further explored, which needs to further analyze more clinical interrelated information and conduct fundamental experiment studies (8). This review is aimed to summarize and analyze possible pathophysiological mechanisms of MAT. Therefore, this review aims to introduce the etiology of MAT at different periods of growth and the specific etiopathogenesis of MAT.
Etiology of Mat at Different Periods of Growth
Gestational Disease Leads to MAT
Most cases of MAT have been detected in infancy and utero, which suggests that immaturity and vulnerability of the atrial cells are responsible for the infantile-dominated distribution of MAT (4). During pregnancy, many risk factors, such as gestational hypertension and diabetes, result in fetal ischemia, hypoxia, and edema, inducing abnormal development of fetal atrial tissue, cells, and epicardial adipose tissue (9), which in turn results in fetal atrial development abnormalities and changes in signal transduction and contributes to MAT.
Gestational Diabetes Mellitus
Gestational diabetes mellitus (GDM) is the most common metabolic disease during pregnancy, with an overall prevalence of 6%−13% (10, 11). In patients with gestational diabetes, maternal metabolic abnormalities lead to impaired fetal cardiovascular hemodynamics through a variety of mechanisms, including decreased cardiac output and increased aortic isthmus' flow velocity and pulsation (12). Furthermore, abnormal blood glucose variability in mothers with diabetes contributes to the development of cardiac diastolic dysfunction, even when obvious cardiac hypertrophy is not observed. Further studies have shown that chronic fetal hyperinsulinemia, caused by fetal glucose elevation within gestational diabetes, increases fetal oxygen consumption, which results in fetal hypoxemia and directly prohibits the development of the cardiovascular system via inducing an imbalance of oxidative stress and antioxidant (13–15). Oxidative stress and antioxidation can contribute to the development of cardiovascular disease via injury of endothelial cells and imbalance of inflammation factors (14). Hypoxemia and ischemia can lead to atrial fibrosis and atrial remodeling via calcium homeostasis disorder in fibroblasts. Dysfunction of Ca2+ plays a key role in the pathogenesis of MAT through increasing afterdepolarization-mediated triggered activity, conduction block, and Ca2+-driven cardiac alternans. All these changes promote remodeling of the fetal atrium and facilitate the occurrence of atrial arrhythmia in infants (13, 16, 17).
Gestational Hypertension
During pregnancy, about 10% of pregnant women develop hypertension, such as chronic hypertension, gestational hypertension, and pre-eclampsia (10, 18–21). Significant diastolic dysfunction is observed in the fetus's heart in gestational hypertension due to intrauterine growth restriction and pre-eclampsia. Moreover, cardiac dysfunction is exacerbated by the deterioration of placental vascular resistance (22–25). Particularly, fetal cardiac dysfunction in pre-eclampsia is possibly due to increased afterload, which is caused by extensive vasoconstriction, resulting in an imbalance of angiogenesis, and chronic trophoblastic ischemia (22–25). Chronic trophoblastic ischemia is a key factor of fetal hypoxia and changes in the fetal signaling pathway (26), which contributes to the formation of arrhythmogenic substrates and atrial arrhythmia. Moreover, fetal hypoxemia promotes abnormal development of the cardiovascular system, which partially contributes to fatal arrhythmia in pregnancy hypertension (27).
As mentioned above, pregnancy disease, such as GDM and hypertension, impedes the development of fetal atrial tissue and epicardial adipose tissue via decreasing fetal nutrition and blood supply, then promote generation of arrhythmogenic substrates and disorder of signal transduction, and, finally, facilitating the occurrence of part MAT in infants of ≤ 3 months.
Pediatric Diseases Lead to MAT
Costello Syndrome
Costello Syndrome (CS) is a mutation disease of HRAS with unique craniofacial features, cardiac abnormalities (65%−75%), growth retardation, dermatological, orthopedic, ocular, and neurological problems (28–31). Cardiac abnormalities usually occur in infancy and can be detected at any age, including typical hypertrophic cardiomyopathy, congenital heart defects, and arrhythmias (especially MAT or ectopic atrial tachycardia) (29). Previous studies indicated that ~9% of Costello Syndrome is complicated with MAT (3), and every MAT can result in death within the first 2 years of life (56%), but is usually self-limiting with aggressive treatment (31, 32). Another study indicated that patients being diagnosed with CS are also complicated with AT (33%) and the majority of them have MAT (64.5%) (32). The etiology of CS with MAT is dysplasia and degeneration of the conduction system, then, the abnormal conduction pathways of the atrioventricular node (32). However, the pathogenesis of CS is complex and difficult to establish animal models, and the specific pathogenesis of CS is complicated with MAT still needs further exploration.
Congenital Heart Disease and Cardiac Anatomic Abnormality
Previous studies indicated that ~50% of supraventricular tachycardia (SVT) are atrial tachycardia (AT), most of which occurred in children who are <6 months old (33) and are associated with structural heart disease (34). The incidence of AT in patients with congenital heart disease (CHD) is three times that in the general population, and 42% of structural heart disease is complicated with MAT (3). Furthermore, the morbidity of atrial arrhythmia is increased with aging and the complexity of CHD, both of which predict adverse outcomes of AT, such as heart failure, death, and intervention (7). Because of early diagnosis and early surgery, the prognosis of patients with CHD has greatly improved in recent decades. However, the ameliorated survival rate is accompanied by an increase in arrhythmia morbidity (35), especially MAT, which is related to a specific type of CHD, palliative surgery, and radical surgical repair (36–38). Another study demonstrated that 50% of Klippel-Feil syndrome, tetralogy of Fallot, Noonan syndrome, myocarditis, familial hypertrophic obstructive cardiomyopathy, RASopathy syndrome, and Heterotaxy syndrome have a cardiac anatomic abnormality, which facilitates the development of MAT (3, 5).
The AT is typically related to anatomical abnormalities, which are associated with the underlying cardiac defect in children with CHD (39). Firstly, the scar and fibrosis of the atrial tissue increase atrial activation, which promotes automatic disorder and the occurrence of MAT (40). Secondly, the shunt or valve reflux caused by structural abnormalities in CHD leads to ion channel dysfunction via inducing enlargement of the cardiac cavity, then resulting in MAT by enhanced automaticity and triggering activities (41). Thirdly, the enlargement of the cardiac cavity resulting from hemodynamic changes could increase cardiac volume, which will result in pressure overload of each cardiac cavity. The development of arrhythmogenic substrates would be induced by scar tissue, long-term volume overload and/or pressure overload, and abnormal ion channel function, which, will, finally, result in MAT (42, 43).
Post-cardiac Surgery
Patients with CHD surgery are prone to arrhythmia in their lifetime. Particularly, the incidence of postoperative arrhythmia is ≥48% in children who have undergone congenital heart surgery (44), among which atrial arrhythmia is more common. The risk of atrial arrhythmia in patients with CHD is ~50% before 20 years old. Many factors are increasing the risk of tachyarrhythmia after surgical treatment. For example, cardiopulmonary bypass during surgical procedures causes an acute inflammatory state and facilitates the production of pro-inflammatory factors (38). Furthermore, aortic cross forceps increase coronary artery ischemia-reperfusion injury and myocardial conduction system ischemia, both of which cause hemodynamic changes, resulting in ischemia and hypoxemia, promoting the production of arrhythmogenic substrates, and increasing the risk of MAT; over time, owing to surgical incisions, fibrosis, suture lines, baffles (45), conduits, scarring within the walls of the atria (38), and residual cardiac lesions, which induce abnormal cardiac remodeling, hypoxemia (35), and change of areas of slow conduction. All of these lead to the formation of arrhythmogenic substrates occurrence (35), which induces an increased incidence of MAT (46). MAT predominantly arises in areas of abnormal conduction around suture points, surgical incisions, or scar borders, which is commonly caused by re-entry (45, 46). The mechanism is not clear, and follow-up studies are needed to address this issue.
Pulmonary Arterial Hypertension
Pulmonary arterial hypertension (PAH) is a progressive and lethal disease, especially in infants, which mainly affects cardiopulmonary function, and 8%−35% of its complication is supraventricular tachycardia, particularly atrial arrhythmias being more common and severe (47, 48). PAH leads to right atrial enlargement, pulmonary vascular resistance increases, and lower cardiac index values, all of which promote the probability of occurrence of atrial arrhythmia (49–51). Recent studies indicated that legumain activated MMP2/TGF β1 signaling pathway to increase extracellular matrix (ECM) in PAH (52) and activated PI3K/Akt pathway to promote vascular remodeling in atherosclerosis (53). Another recent study has shown that legumain affects the prognosis of acute myocardial infarction by excessive ECM degradation via MMP2 pathway activation (54). Consequently, this review speculates that legumain plays an important role in PAH with atrial arrhythmia, but the pathogenesis is needed to be further investigated.
Infectious Diseases
Infants, especially at age of 3 months old or less, are susceptible to acute infectious diseases, particularly, when it is concomitant with congenital heart disease. It is more prone to immune deficiency, which increase the possibility of infectious diseases, such as respiratory system infection and digestive system infection (55). Inflammation caused by infection diseases, promoting hypercapnia, hypoxia, or adrenergic stimulation, and increases the cardiac load, which may result in right atrium enlargement and development of MAT (3, 5, 56). Chronic inflammation has been caused by sarcoidosis, systemic lupus erythematosus, and scleroderma, which could induce sympathetic nervous overactivation and accelerate myocardial cell necrosis, which triggers electrical and structural remodeling, and, finally, resulting in MAT (57).
Etiopathogenesis of Mat
Inflammation
Inflammation increases the risk of MAT through the following mechanisms. Firstly, disorganized secretion of inflammatory cytokines promotes the release of pro-inflammatory factors, which induces disorderly physiological functions of the myocardial cell by several pathways (Figure 1A): (A) Calcium overload results in abnormal calcium handling and potentially prolonged action potential duration (58); (B) Intracellular calcium levels are increased by reduced sarcoplasmic reticulum ATPase activity; (C) Calcium is leaked from the sarcoplasmic reticulum (59); (D) Myocardial depolarization damage is caused by sodium channel dysfunction (60); (E) Reduction of heart rate variability is observed; and (F) It prolongs the interval between QTc and myocardial repolarization to disrupt the physiological functions of myocardial cells. Secondly, the proinflammatory factor is released by myocardial cell at the inflammatory response, which has a substantial influence on blood coagulation and fibrinolytic system and produces blood coagulation system activation to result in a hypercoagulable state. Part of the studies indicated that coagulation cascade is produced at the systemic inflammatory response, which is via a variety of ways to promote the occurrence of MAT directly or indirectly (61). Thirdly, hemodynamic changes and redistribution of the blood flow in various organs and tissues of the body are produced by an inflammatory response, which induces ischemia and hypoxia in local organs or tissues and ischemic heart diseases occurrence in severe cases. All of these facilitate the formation of arrhythmogenic substrates, which can ultimately result in enhanced automaticity, reentry, and triggered activity (62). Previous research have reported that inflammation could promote the release of inflammatory cytokines, such as TNF-α, IL-6, and IL-8, which also have been used as an important biomarker of acute infection and played a role in the prediction of AT in partial patients (62, 63). Therefore, it is necessary to further explore the role of these biomarkers in MAT and find more suitable biomarkers for predicting MAT.
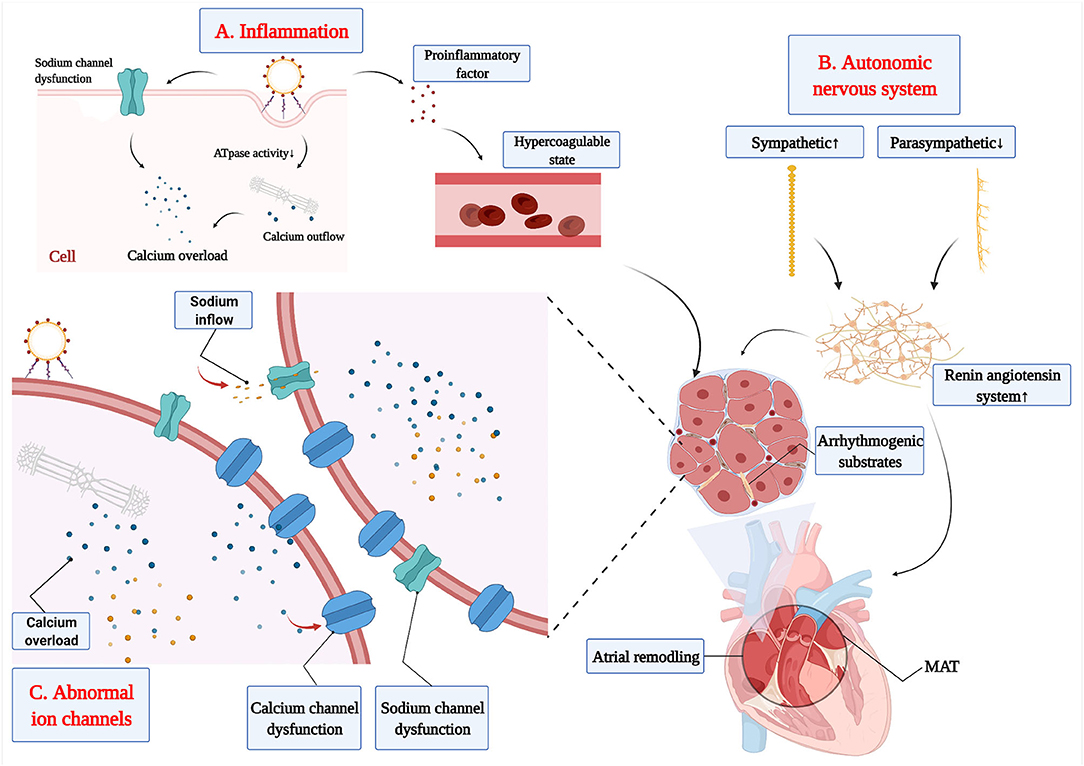
Figure 1. The etiopathogenesis of MAT. (A) The release of proinflammatory factor from inflammation increases the risk of MAT through hypercoagulable state and dysfunction of myocardial cell. (B) The disorder of the autonomic nervous system increases the activation of the renin-angiotensin-aldosterone system to promote the development of arrhythmogenic substrates. (C) The calcium channel and sodium channel dysfunction promote the development of arrhythmogenic substrates.
Autonomic Nervous System
The heart is innervated by the autonomic nervous system, including the sympathetic and parasympathetic nervous systems. The autonomic nervous system plays a key role in the control of heart rate and pathogenesis of multiple types of cardiac arrhythmia (64–66).
In resting state, the vagus exerts an inhibitory modulation in heart rate via increasing vagal output to the sinus node and limiting the influence of the sympathetic nerve, and finally making the heart rate slow and steady. The inhibitory effect of the vagus nerve is disengaged, and the vagus nerve output decreased during stress, then, resulting in increasing in heart rate (64).
Under pathological conditions, such as myocardial infarction and heart failure, neuronal remodeling and activation of the renin-angiotensin system were triggered by the decrease in cardiac output (67). Meanwhile, mechanical stretch of atrial myocardial cells occurred in heart failure (60, 68), which could stimulate the synthesis of nerve growth factors to promote sympathetic germination and over-innervation (69). Sympathetic over-innervation enhances Ca2+ transient and early after depolarization, which could shorten the atrial effective refractory period and increase the effective refractory period dispersion (66, 70, 71). Finally, all of these can promote the production of arrhythmogenic substrates, which is an important factor in the development of MAT. Hypertension is characterized by peripheral vascular resistance increases, sympathetic activation enhancement, and vagus activation reduction, which increases susceptibility to atrial arrhythmia (72). Furthermore, excessive sympathetic enhancement increases the activation of the renin-angiotensin-aldosterone system, which will induce atrial electrical and structural remodeling (73), and, finally, promote the production of arrhythmogenic substrates in MAT (Figure 1B).
Abnormal Ion Channels
The ion channels are critical to maintaining cell excitability and the propagation of electrical impulses spread in cardiac excitable cells (74). Under pathological conditions, ion channel expression and function could be altered by pathogenesis, such as inflammation, electrolyte disturbance, and autonomic nervous system imbalance (71), which then result in the development of MAT.
Although the relationship between abnormal ion channels and MAT has not fully been elucidated, there has been achieved significant progress in the functional involvement of ion channels in MAT. Voltage-gated sodium channels determine the magnitude and slope of action potential rise, which is particularly important in controlling conduction velocity and maintaining appropriate excitability in the myocardium (74). Atrial ischemia and hypoxia enhance the excitability of atrial myocytes and promote the slow inflow of a small number of sodium ions to these cells, which decrease resting membrane potential and enhance the automaticity of fast-responding cells, finally resulting in MAT. Electrolyte disturbances caused by various causes, such as hypokalemia and acidosis, would increase intracellular potassium ion outflows. However, the patients with severe hypokalemia suffer from severe potassium deficiency, both inside and outside the atrial cell. Then, cells inhibit potassium ion outflow to ensure physiological metabolism, which will decrease atrial resting membrane potential, slowing conduction velocity and prolonging repolarization (75, 76). Then, the threshold of myocardial cell excitation decreases with lower atrial resting membrane potential, which will induce AP more easily. Ectopic electrical activity will be a triggered in multiple cardiac myocytes based on existing atrial remodeling, which will trigger multiple pathways to promote MAT. Furthermore, the dysfunction of Ca2+ plays a key role in the pathogenesis of atrial arrhythmia (77) through increasing after depolarization-mediated triggered activity, conduction block, and Ca2+-driven cardiac alternans (78) (Figure 1C). Severe hypokalemia or myocardial injury inhibit the influx of calcium in slow response cells of the sinoatrial node, which relates to enhancement of atrial ectopic excitability and formation of reentry loop, finally resulting in MAT. Inflammation reduces sarcoplasmic reticulum ATPase activity, thereby increasing intracellular calcium levels, which induces calcium leakage from the sarcoplasmic reticulum (59). Moreover, the abnormal handling of calcium generates calcium overload and ICaL downregulation, which can result in MAT via modulating atrial action potential repolarization, action potential duration, and automaticity (58).
Conclusion
From fetal to infants <3 months old, the development of atrial tissue has not been completed, and the atria are immature and vulnerable period. At this stage, the development of atrial is extremely vulnerable, a variety of diseases could promote the development of arrhythmogenic substrates via various causes to the disorder of hemodynamics, ischemia hypoxia for tissues, abnormal cell metabolism, autonomic nervous system dysfunction, and abnormal ion channels, which will result in the formation of reentry loop, abnormal automaticity, and triggering activity. However, fetal atrial tissue has a strong ability to regenerate and differentiate and MAT is self-limited, so most patients with MAT have the favorable outcome. This review makes clinicians more careful about the diagnosis of MAT in pediatric patients, who had the gestational disease, congenital heart disease, cardiac anatomic abnormality, post-cardiac surgery, pulmonary hypertension, or infectious diseases, and give clinical intervention as soon as possible to avoid the occurrence of cardiogenic death, respiratory failure, and persistent arrhythmia. However, the pathogenesis of pediatric MAT, particularly in ion channel, still needs further studies, and explore the pathway to MAT prevention and better therapeutic target.
Author Contributions
HC, YM, and YX contributed to the study. HC wrote the first draft of the manuscript. YM wrote sections of the manuscript. All authors contributed to manuscript revision, read, and approved the submitted version.
Funding
This study was funded by Hunan Clinical Research Center for Children's Cardiovascular Diseases (2021SK4019), Major projects of Hunan Province (2020SK1013, QL), the Hunan Provincial Health Commission Project (No. 20200483), Project of Hunan Provincial Research on Chinese Medicine (No. 201914), and 2019 National Medical Service, and Support Capacity Improvement Project: Children's Difficult Diagnosis and Treatment Center.
Conflict of Interest
The authors declare that the research was conducted in the absence of any commercial or financial relationships that could be construed as a potential conflict of interest.
Publisher's Note
All claims expressed in this article are solely those of the authors and do not necessarily represent those of their affiliated organizations, or those of the publisher, the editors and the reviewers. Any product that may be evaluated in this article, or claim that may be made by its manufacturer, is not guaranteed or endorsed by the publisher.
Acknowledgments
We acknowledge BioRender.com for the generation of cartoon figures.
References
1. Phillips J, Spano J, Burch G. Chaotic atrial mechanism. Am Heart J. (1969) 78:171–9. doi: 10.1016/0002-8703(69)90005-2
2. Bisset GS, Seigel SF, Gaum WE. Chaotic atrial tachycardia in childhood. Am Heart J. (1981) 101:268–72. doi: 10.1016/0002-8703(81)90189-7
3. Baek SM, Chung H, Song MK, Bae EJ, Kim GB. The complexity of pediatric multifocal atrial tachycardia and its prognostic factors. Korean Circ J. (2018) 48:148–58. doi: 10.4070/kcj.2017.0179
4. Huh J. Clinical implication of multifocal atrial tachycardia in children for pediatric cardiologist. Korean Circ J. (2018) 48:173–5. doi: 10.4070/kcj.2018.0037
5. Bradley DJ, Fischbach PS, Law IH, Serwer GA. The clinical course of multifocal atrial tachycardia in infants and children. J Am Coll Cardiol. (2001) 38:401–8. doi: 10.1016/S0735-1097(01)01390-0
6. Liberthson RR, Colan SD. Multifocal or chaotic atrial rhythm: report of nine infants, delineation of clinical course and management, and review of the literature. Pediatr Cardiol. (1982) 2:179–84. doi: 10.1007/BF02332108
7. Guerrier K, Hendrickson B, Waller BR. Diagnostic and therapeutic approach to arrhythmias in adult congenital heart disease. Curr Treat Options Cardiovasc Med. (2019) 21:44. doi: 10.1007/s11936-019-0749-9
8. Custer AM, Yelamanchili VS, Lappin SL. Multifocal Atrial Tachycardia (MAT). Treasure Island, FL: StatPearls Publishing (2021).
9. Yamamoto T, Iwasaki Y-K, Fujimoto Y, Oka E, Hayashi H, Murata H, et al. The characteristics and efficacy of catheter ablation of focal atrial tachycardia arising from an epicardial site. Clin Cardiol. (2021) 44:563–72. doi: 10.1002/clc.23577
10. Ramlakhan KP, Johnson MR, Roos-Hesselink JW. Pregnancy and cardiovascular disease. Nat Rev Cardiol. (2020) 17:718–31. doi: 10.1038/s41569-020-0390-z
11. Zhu Y, Zhang C. Prevalence of gestational diabetes and risk of progression to type 2 diabetes: a global perspective. Curr Diab Rep. (2016) 16:7. doi: 10.1007/s11892-015-0699-x
12. Lehtoranta L, Haapsamo M, Vuolteenaho O, Palo P, Ekholm E. Fetal cardiovascular hemodynamics in type 1 diabetic pregnancies at near-term gestation. Acta Obstet Gynecol Scand. (2021) 100:263–71. doi: 10.1111/aogs.13987
13. Leybovitz-Haleluya N, Wainstock T, Landau D. Maternal gestational diabetes mellitus and the risk of subsequent pediatric cardiovascular diseases of the offspring: a population-based cohort study with up to 18 years of follow up. Acta Diabetol. (2018) 55:1037–42. doi: 10.1007/s00592-018-1176-1
14. Lappas M, Hiden U, Desoye G, Froehlich J. Mouzon SH-d, Jawerbaum A. The role of oxidative stress in the pathophysiology of gestational diabetes mellitus. Antioxid Redox Signal. (2011) 15:3061–100. doi: 10.1089/ars.2010.3765
15. Escobar J, Teramo K, Stefanovic V, Andersson S, Asensi MA, Arduini A, et al. Amniotic fluid oxidative and nitrosative stress biomarkers correlate with fetal chronic hypoxia in diabetic pregnancies. Neonatology. (2013) 103:193–8. doi: 10.1159/000345194
16. Pike JI, Krishnan A, Kaltman J, Donofrio MT. Fetal and neonatal atrial arrhythmias: an association with maternal diabetes and neonatal macrosomia. Prenat Diagn. (2013) 33:1152–7. doi: 10.1002/pd.4210
17. Topcuoglu S, Karatekin G, Yavuz T, Arman D, Kaya A, Gursoy T, et al. The relationship between the oxidative stress and the cardiac hypertrophy in infants of diabetic mothers. Diabetes Res Clin Pract. (2015) 109:104–9. doi: 10.1016/j.diabres.2015.04.022
18. Duley L. The global impact of pre-eclampsia and eclampsia. Semin Perinatol. (2009) 33:130–7. doi: 10.1053/j.semperi.2009.02.010
19. Steegers EAP, Dadelszen PV, Duvekot JJ, Pijnenborg R. Pre-eclampsia. Lancet. (2010) 376:631–44. doi: 10.1016/S0140-6736(10)60279-6
20. World Health Organization. WHO Recommendations for Prevention and Treatment of Pre-Eclampsia and Eclampsia. Geneva: WHO (2011). Available online at: https://www.ncbi.nlm.nih.gov/books/NBK140561 (accessed February 2022).
21. Lawlor DA, Macdonald-Wallis C, Fraser A, Nelson SM, Hingorani A, Smith GD, et al. Cardiovascular biomarkers and vascular function during childhood in the offspring of mothers with hypertensive disorders of pregnancy: findings from the Avon Longitudinal Study of Parents and Children. Eur Heart J. (2012) 33:335–45. doi: 10.1093/eurheartj/ehr300
22. Bhorat IE, Bagratee JS, Pillay M, Reddy T. Determination of the myocardial performance index in deteriorating grades of intrauterine growth restriction and its link to adverse outcomes. Prenat Diagn. (2015) 35:266–73. doi: 10.1002/pd.4537
23. Bhorat IE, Bagratee JS. T Reddy. Assessment of fetal myocardial performance in severe early onset pre-eclampsia (EO-PET) with and without intrauterine growth restriction across deteriorating stages of placental vascular resistance and links to adverse outcomes Eur J Obstet Gynecol Reprod Biol. (2017) 210:325–33. doi: 10.1016/j.ejogrb.2017.01.014
24. Crispi F, Hernandez-Andrade E, Pelsers MMAL, Plasencia W, Benavides-Serralde JA, Eixarch E, et al. Cardiac dysfunction and cell damage across clinical stages of severity in growth-restricted fetuses. Am J Obstet Gynecol. (2008) 199:254.e251–8. doi: 10.1016/j.ajog.2008.06.056
25. Tsutsumi T, Ishii M, Eto G, Hota M, Kato H. Serial evaluation for myocardial performance in fetuses and neonates using a new Doppler index. Pediatr Int. (1999) 41:722–7. doi: 10.1046/j.1442-200x.1999.01155.x
26. Bhorat I. An integrated model of materno-foetal cardiac dysfunction in severe pre-eclampsia. Cardiovasc J Afr. (2019) 30:181–3. doi: 10.5830/CVJA-2018-071
27. Ray JG, Schull MJ, Kingdom JC, Vermeulen MJ. Heart failure and dysrhythmias after maternal placental syndromes: HAD MPS Study. Heart. (2012) 98:1136–41. doi: 10.1136/heartjnl-2011-301548
29. Philip N, Sigaudy S. Costello syndrome. J Med Genet. (1998) 35:238–40. doi: 10.1136/jmg.35.3.238
30. Hennekam RC. Costello syndrome: an overview. Am J Med Genet C Semin Med Genet. (2003) 117C:42–8. doi: 10.1002/ajmg.c.10019
31. Gripp KW, Morse LA, Axelrad M, Chatfield KC, Chidekel A, Dobyns W, et al. Costello syndrome: Clinical phenotype, genotype, and management guidelines. Am J Med Genet A. (2019) 179:1725–44. doi: 10.1002/ajmg.a.61270
32. Lin AE, Grossfeld PD, Hamilton RM, Smoot L, Gripp KW, Proud V, et al. Further delineation of cardiac abnormalities in Costello syndrome. Am J Med Genet. (2002) 111:115–29. doi: 10.1002/ajmg.10558
33. Page RL, Joglar JA, Caldwell MA, Calkins H, Conti JB, Deal BJ, et al. 2015 ACC/AHA/HRS guideline for the management of adult patients with supraventricular tachycardia: a report of the American College of Cardiology/American Heart Association Task Force on Clinical Practice Guidelines and the Heart Rhythm Society. Heart Rhythm. (2016) 13:e136–221. doi: 10.1016/j.hrthm.2015.09.019
34. Boldt T, Eronen M, Andersson S. Long-term outcome in fetuses with cardiac arrhythmias. Obstet Gynecol. (2003) 102:1372–9. doi: 10.1097/00006250-200312000-00026
35. Roca-Luque I, Gándara NR, Subirà LD, Pascual JF, Domenech AP, et al. Intra-atrial re-entrant tachycardia in congenital heart disease: types and relation of isthmus to atrial voltage. Europace. (2018) 20:353–61. doi: 10.1093/europace/eux250
36. Clark BC, Berul CI. Arrhythmia diagnosis and management throughout life in congenital heart disease. Expert Rev Cardiovasc Ther. (2016) 14:301–20. doi: 10.1586/14779072.2016.1128826
37. Kasar T, Tanidir IC, Öztürk E, Gökalp S, Sahin GT, Topkarci MA, et al. Arrhythmia during diagnostic cardiac catheterization in pediatric patients with congenital heart disease. Turk Kardiyol Dern Ars. (2018) 46:675–82. doi: 10.5543/tkda.2018.06025
38. Houck CA, Chandler SF, Bogers AJJC, Triedman JK, Walsh EP, Groot NMSd, et al. Arrhythmia mechanisms and outcomes of ablation in pediatric patients with congenital heart disease. Circ Arrhythm Electrophysiol. (2019) 12:e007663. doi: 10.1161/CIRCEP.119.007663
39. Karbassi A, Nair K, Harris L, Wald RM, Roche SL. Atrial tachyarrhythmia in adult congenital heart disease. World J Cardiol. (2017) 9:496–507. doi: 10.4330/wjc.v9.i6.496
40. Roca-Luque I, Rivas-Gándara N, Subirà LD, Francisco-Pascual J, Pijuan-Domenech A, Pérez-Rodon J, et al. Mechanisms of intra-atrial re-entrant tachycardias in congenital heart disease: types and predictors. Am J Cardiol. (2018) 122:672–82. doi: 10.1016/j.amjcard.2018.04.041
41. Walsh EP, Cecchin F. Arrhythmias in adult patients with congenital heart disease. Circulation. (2007) 115:534–45. doi: 10.1161/CIRCULATIONAHA.105.592410
42. Kharbanda RK, Schie MSv, Leeuwen WJv, Taverne YJHJ, Houck CA, Kammeraad JAE, et al. First-in-children epicardial mapping of the heart: unravelling arrhythmogenesis in congenital heart disease. Interact Cardiovasc Thorac Surg. (2021) 32:137–40. doi: 10.1093/icvts/ivaa233
43. Klehs S, Schneider HE, Backhoff D, Paul T, Krause U. Radiofrequency catheter ablation of atrial tachycardias in congenital heart disease: results with special reference to complexity of underlying anatomy. Circ Arrhythm Electrophysiol. (2017) 10:e005451. doi: 10.1161/CIRCEP.117.005451
44. Sahu MK, Das A, Siddharth B, Talwar S, Singh SP, Abraham A, et al. Arrhythmias in children in early postoperative period after cardiac surgery. World J Pediatr Congenit Heart Surg. (2018) 9:38–46. doi: 10.1177/2150135117737687
45. Labombarda F, Hamilton R, Shohoudi A, Aboulhosn J, Broberg CS, Chaix MA, et al. Increasing prevalence of atrial fibrillation and permanent atrial arrhythmias in congenital heart disease. J Am Coll Cardiol. (2017) 70:857–65. doi: 10.1016/j.jacc.2017.06.034
46. He D, Aggarwal N, Zurakowski D, Jonas RA, Berul CI, Hanumanthaiah S, et al. Lower risk of postoperative arrhythmias in congenital heart surgery following intraoperative administration of magnesium. J Thorac Cardiovasc Surg. (2018) 156:763–70.e761. doi: 10.1016/j.jtcvs.2018.04.044
47. Galiè N, Humbert M, Vachiery J-L, Gibbs S, Lang I, Torbicki A, et al. 2015 ESC/ERS guidelines for the diagnosis and treatment of pulmonary hypertension: the joint task force for the diagnosis and treatment of pulmonary hypertension of the european society of cardiology (ESC) and the european respiratory Society (ERS): Endorsed by: Association for European Paediatric and Congenital Cardiology (AEPC), international society for heart and lung transplantation (ISHLT). Eur Heart J. (2016) 37:67–119. doi: 10.1093/eurheartj/ehv317
48. Lau EMT, Giannoulatou E, Celermajer DS, Humbert M. Epidemiology and treatment of pulmonary arterial hypertension. Nat Rev Cardiol. (2017) 14:603–14. doi: 10.1038/nrcardio.2017.84
49. Wen L, Sun M-L, An P, Jiang X, Sun K, Zheng L, et al. Frequency of supraventricular arrhythmias in patients with idiopathic pulmonary arterial hypertension. Am J Cardiol. (2014) 114:1420–5. doi: 10.1016/j.amjcard.2014.07.079
50. Małaczyńska-Rajpold K, Komosa A, Błaszyk K, Araszkiewicz A, Janus M, Olasińska-Wiśniewska A, et al. The management of supraventricular tachyarrhythmias in patients with pulmonary arterial hypertension. Heart Lung Circ. (2016) 25:442–50. doi: 10.1016/j.hlc.2015.10.008
51. Olsson KM, Nickel NP, Tongers J, Hoeper MM. Atrial flutter and fibrillation in patients with pulmonary hypertension. Int J Cardiol. (2013) 167:2300–5. doi: 10.1016/j.ijcard.2012.06.024
52. Bai P, Lyu L, Yu T, Zuo C, Fu J, He Y, et al. Macrophage-derived legumain promotes pulmonary hypertension by activating the MMP (Matrix metalloproteinase)-2/TGF (Transforming growth factor)-β1 signaling. Arterioscler Thromb Vasc Biol. (2019) 39:e130–45. doi: 10.1161/ATVBAHA.118.312254
53. Ozawa N, Sato Y, Mori Y, Masuda H, Yamane M, Yamamoto Y, et al. Legumain promotes atherosclerotic vascular remodeling. Int J Mol Sci. (2019) 20:2195. doi: 10.3390/ijms20092195
54. Yang H, He Y, Zou P, Hu Y, Li X, Tang L, et al. Legumain is a predictor of all-cause mortality and potential therapeutic target in acute myocardial infarction. Cell Death Dis. (2020) 11:1014. doi: 10.1038/s41419-020-03211-4
55. Banjac L, Nikcević D, Vujosević D, Raonić J, Banjac G. Tachycardia in a newborn with enterovirus infection. Acta Clin Croat. (2014) 53:102–6.
56. Vonderlin N, Siebermair J, Kaya E, Köhler M, Rassaf T, Wakili R. Critical inflammatory mechanisms underlying arrhythmias. Herz. (2019) 44:121–9. doi: 10.1007/s00059-019-4788-5
57. Gawałko M, Balsam P, Lodziński P, Grabowski M, Krzowski B, Opolski G, et al. Cardiac arrhythmias in autoimmune diseases. Circ J. (2020) 84:685–94. doi: 10.1253/circj.CJ-19-0705
58. Qi XY, Yeh Y-H, Xiao L, Burstein B, Maguy A, Chartier D, et al. Cellular signaling underlying atrial tachycardia remodeling of L-type calcium current. Circ Res. (2008) 103:845–54. doi: 10.1161/CIRCRESAHA.108.175463
59. Duncan DJ, Yang Z, Hopkins PM, Steele DS, Harrison SM. TNF-alpha and IL-1beta increase Ca2+ leak from the sarcoplasmic reticulum and susceptibility to arrhythmia in rat ventricular myocytes. Cell Calcium. (2010) 47:378–86. doi: 10.1016/j.ceca.2010.02.002
60. Grandy SA, Brouillette J, Fiset C. Reduction of ventricular sodium current in a mouse model of HIV. J Cardiovasc Electrophysiol. (2010) 21:916–22. doi: 10.1111/j.1540-8167.2009.01713.x
61. Alonso A, Tang W, Agarwal SK, Soliman EZ, Chamberlain AM, Folsom AR. Hemostatic markers are associated with the risk and prognosis of atrial fibrillation: the ARIC study. Int J Cardiol. (2012) 155:217–22. doi: 10.1016/j.ijcard.2010.09.051
62. Yalta T, Yalta K. Systemic inflammation and arrhythmogenesis: a review of mechanistic and clinical perspectives. Angiology. (2018) 69:288–96. doi: 10.1177/0003319717709380
63. Aydin M, Yildiz A, Yüksel M, Polat N, Aktan A, Islamoglu Y. Assessment of the neutrophil/lymphocyte ratio in patients with supraventricular tachycardia. Anatol J Cardiol. (2016) 16:29–33. doi: 10.5152/akd.2015.5927
64. Suurland J. Heijden KBvd, Huijbregts SCJ, Goozen SHMv, Swaab H. Interaction between prenatal risk and infant parasympathetic and sympathetic stress reactivity predicts early aggression. Biol Psychol. (2017) 128:98–104. doi: 10.1016/j.biopsycho.2017.07.005
65. Qin M, Liu X, Liu T, Wang T, Huang CX. Potential role of regulator of G-protein signaling 5 in the protection of vagal-related bradycardia and atrial tachyarrhythmia. J Am Heart Assoc. (2016) 5:e002783. doi: 10.1161/JAHA.115.002783
66. Stavrakis S, Kulkarni K, Singh JP, Katritsis DG, Armoundas AA. Autonomic modulation of cardiac arrhythmias: methods to assess treatment and outcomes. JACC Clin Electrophysiol. (2020) 6:467–83. doi: 10.1016/j.jacep.2020.02.014
67. Zhu C, Hanna P, Rajendran PS, Shivkumar K. Neuromodulation for ventricular tachycardia and atrial fibrillation: a clinical scenario-based review. JACC Clin Electrophysiol. (2019) 5:881–96. doi: 10.1016/j.jacep.2019.06.009
68. Rana OR, Schauerte P, Hommes D, Schwinger RHG, Schröder JW, Hoffmann R, et al. Mechanical stretch induces nerve sprouting in rat sympathetic neurocytes. Auton Neurosci. (2010) 155:25–32. doi: 10.1016/j.autneu.2010.01.003
69. Shangguan W, Shi W, Li G, Wang Y, Li J, Wang X. Angiotensin-(1-7) attenuates atrial tachycardia-induced sympathetic nerve remodeling. J Renin Angiotensin Aldosterone Syst. (2017) 18:1470320317729281. doi: 10.1177/1470320317729281
70. Yu L, Scherlag BJ, Sha Y, Li S, Sharma T, Nakagawa H, et al. Interactions between atrial electrical remodeling and autonomic remodeling: how to break the vicious cycle. Heart Rhythm. (2012) 9:804–9. doi: 10.1016/j.hrthm.2011.12.023
71. Lu Z, Scherlag BJ, Lin J, Niu G, Fung K-M, Zhao L, et al. Atrial fibrillation begets atrial fibrillation: autonomic mechanism for atrial electrical remodeling induced by short-term rapid atrial pacing. Circ Arrhythm Electrophysiol. (2008) 1:184–92. doi: 10.1161/CIRCEP.108.784272
72. Rodrigues JQD, Camara H. Junior EDdS, Godinho RO, Jurkiewicz A. Intrinsic adaptation of SHR right atrium reduces heart rate. J Cardiovasc Pharmacol. (2019) 74:542–8. doi: 10.1097/FJC.0000000000000746
73. Waldron NH, Fudim M, Mathew JP, Piccini JP. Neuromodulation for the treatment of heart rhythm disorders. JACC Basic Transl Sci. (2019) 4:546–62. doi: 10.1016/j.jacbts.2019.02.009
74. Vanninen SUM, Nikus K, Aalto-Setälä K. Electrocardiogram changes and atrial arrhythmias in individuals carrying sodium channel SCN5A D1275N mutation. Ann Med. (2017) 49:496–503. doi: 10.1080/07853890.2017.1307515
75. Bao Y, Willis BC, Frasier CR, Lopez-Santiago LF, Lin X, Ramos-Mondragón R, et al. Scn2b deletion in mice results in ventricular and atrial arrhythmias. Circ Arrhythm Electrophysiol. (2016) 9:e003923. doi: 10.1161/CIRCEP.116.003923
76. Shkryl VM, Maxwell JT, Domeier TL, Blatter LA. Refractoriness of sarcoplasmic reticulum Ca2+ release determines Ca2+ alternans in atrial myocytes. Am J Physiol Heart Circ Physiol. (2012) 302:H2310–20. doi: 10.1152/ajpheart.00079.2012
77. Landstrom AP, Dobrev D, Wehrens XHT. Calcium signaling and cardiac arrhythmias. Circ Res. (2017) 120:1969–93. doi: 10.1161/CIRCRESAHA.117.310083
Keywords: multifocal atrial tachycardia (MAT), inflammation, autonomic nervous, ion channel, pathogenesis, etiology
Citation: Chen H, Ma Y, Wang Y, Luo H, Xiao Z, Chen Z, Liu Q and Xiao Y (2022) Progress of Pathogenesis in Pediatric Multifocal Atrial Tachycardia. Front. Pediatr. 10:922464. doi: 10.3389/fped.2022.922464
Received: 18 April 2022; Accepted: 23 May 2022;
Published: 22 June 2022.
Edited by:
Hongfang Jin, Peking University, ChinaReviewed by:
Tiewei Lv, Children's Hospital of Chongqing Medical University, ChinaPengjun Zhao, Shanghai Jiao Tong University, China
Copyright © 2022 Chen, Ma, Wang, Luo, Xiao, Chen, Liu and Xiao. This is an open-access article distributed under the terms of the Creative Commons Attribution License (CC BY). The use, distribution or reproduction in other forums is permitted, provided the original author(s) and the copyright owner(s) are credited and that the original publication in this journal is cited, in accordance with accepted academic practice. No use, distribution or reproduction is permitted which does not comply with these terms.
*Correspondence: Yunbin Xiao, eGlhb3l1bmJpbnJ1aUAxMjYuY29t