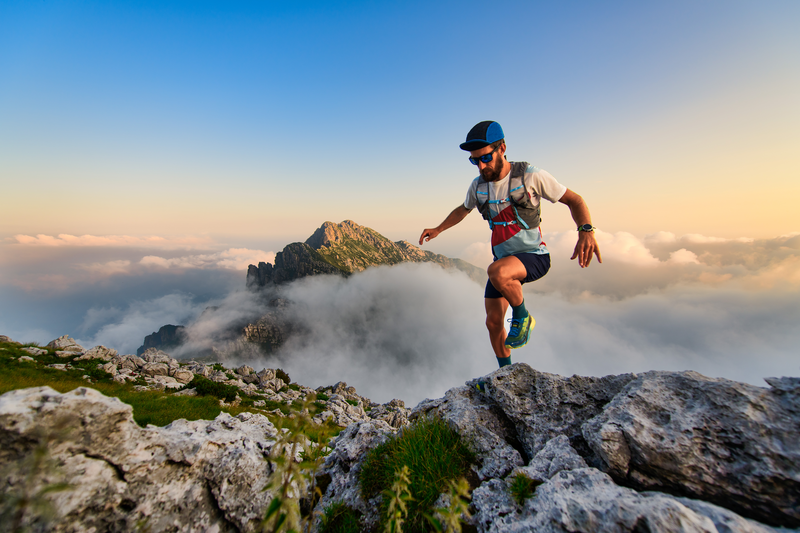
94% of researchers rate our articles as excellent or good
Learn more about the work of our research integrity team to safeguard the quality of each article we publish.
Find out more
ORIGINAL RESEARCH article
Front. Pediatr. , 26 August 2022
Sec. Pediatric Hematology and Hematological Malignancies
Volume 10 - 2022 | https://doi.org/10.3389/fped.2022.914466
Background: Stroke is one of the highest complications of sickle-cell anemia (SCA). The Transcranial Doppler (TCD) has been adopted worldwide as a gold standard method for detecting alterations in the blood velocity in cerebral arteries. In this study, we investigated the association between laboratory parameters and increased cerebral blood flow velocity in Brazilian SCA pediatric patients.
Methods: The study included 159 pediatric patients with SCA, submitted to TCD velocity screening, and the time-averaged maximum mean velocity (TAMMV) was determined in the middle cerebral artery (MCA), anterior cerebral artery (ACA), and distal intracranial internal carotid artery (ICA). We compared cerebral blood flow in patients stratified by the following: TCD1—defined as normal, with TAMMV inferior to 170 cm/s; TCD2—conditional, with TAMMV above 170 cm/s, but less than 199 cm/s; TCD3—altered, with TAMMV greater than or equal to 200 cm/s.
Results: TAMMV was negatively correlated with age and weight (p < 0.05). Moreover, TAMMV was associated or correlated with reductions in HbF, RBC, hemoglobin, hematocrit, HDL, and haptoglobin and, increases in MCV, MCH, RDW, reticulocytes, WBC, lymphocytes, monocytes, eosinophils, total and indirect bilirubin, LDH, AST, ALT, glucose, ferritin, and AAT (p < 0.05).
Conclusion: The current study highlights the importance of the investigation of hemolytic and inflammatory biomarkers for monitoring the clinical outcome of SCA pediatric patients, to avoid acute or chronic stroke. Moreover, glucose and HDL-C appear useful for predicting higher TAMMV.
Sickle-cell anemia (SCA) has an overly complex range of clinical manifestations that depend on many biological and genetic factors, and some are not well-understood. The intravascular hemolysis and chronic anemia that occur in patients SCA play an important role in the disease pathophysiology and clinical event variability. Cerebral vascular accidents (CVA), asymptomatic or symptomatic events, are one of the impairing consequences of SCA and are associated with neurocognitive dysfunction (1–3). Stroke physiopathology can be explained by a microvascular or macrovascular occlusion (4–6).
It frequently occurs during the five (5) first years of life (7), and affects nearly 11% of SCA patients under 20 years of age and, 20–30% will have a silent infarction (4, 8, 9). The same study from the Cooperative Study of Sickle Cell Disease demonstrated that the annual incidence of the first stroke for those with SCA was 0.6% per patient/year with the highest rate of 1.02% per patient/year seen in the group aged 2–5 years. The cumulative risk of stroke was 11% in individuals with age lower than or equal to 20 years, increasing to 24% in those from 20 to 45 years old (8, 9). Many studies have attracted attention to the endothelial dysfunction associated with intravascular hemolysis as an important event in the pathophysiology of stroke in SCA (10–13). Kato et al. (11) made a point about how the hemolytic profile affects the blood flow velocity in the large cerebral vessel, being a risk of stroke event occurrence (11). For decades, stroke has been studied and it happens much progress in the knowledge, especially according to the prevention and early treatment, based on transfusion or/and hydroxyurea (HU) (4, 14, 15). Adams (16) demonstrated that Transcranial Doppler (TCD) velocities can predict stroke risk by detecting that patients with elevated blood flow are more susceptible to a CVA event (9, 16, 17). The TCD has been adopted worldwide as a gold standard method for detecting alteration in the blood velocity in cerebral arteries. Based on the evidence, blood velocity greater than or equal to 200 cm/s is considered a stroke risk condition (17, 18). Currently, some predictors are considered classic markers, such as severe anemia, leukocytosis, nocturnal hypoxemia, and genetic factors (7, 19, 20). Several studies have been conducted to identify risk factors associated with CVA in individuals with SCA (21–24). In this study, we investigated the association between laboratory markers and cerebral blood flow velocity in SCA pediatric patients.
A cross-sectional study was performed between June 2014 and July 2016, and initially included 159 SCA (HbSS) pediatric patients, all seen at the Professor Hosannah de Oliveira Pediatric Center, part of the Professor Edgard Santos University Hospital Complex (CPPHO/HUPES), located in Salvador, and at the Sickle Cell Disease Reference Center of Itabuna. Both the cities are in the state of Bahia, Brazil. We excluded from the study 09 patients who reported a previous stroke event and 13 who were submitted to blood transfusion a few times before enrollment in the study. Of the 137 patients in steady-state (i.e., absence of acute crisis and no use of blood transfusion in the 3 months before blood collection), 31 (22.63%) patients reported HU treatment and were also excluded, due to the association of its use with alterations in laboratory markers (Supplementary Table 1). One hundred and six (106) patients were then considered for the analyses performed in this study, all seen without active infection or inflammatory diseases (Figure 1).
Figure 1. Study design. SCA, sickle cell anemia; HU+, with HU treatment; HU-, without HU treatment; y, years.
This study received approval from the Institutional Review Board of the HUPES (protocol number: 287.768/2013) and was conducted in compliance with the Declaration of Helsinki (1964) and its subsequent amendments. The patient’s legal guardians provided a signed term of informed consent before enrollment in the study.
TCD were examined in all children by the same-trained professional and using the same equipment. Time-averaged maximum mean velocity (TAMMV) was assessed using a 2 MHz probe connected to a Doppler-Box™ X sonography system (Compumedics Germany GmbH, Singen, Hohentwiel, Germany). The TAMMV was recorded in the middle cerebral arteries, anterior cerebral, and distal intracranial internal carotid arteries. Accordingly, 03 outcomes were considered, TCD1–defined as normal, TAMMV inferior to 170 cm/s; TCD2–conditional, with a TAMMV above 170 cm/s and less than 199 cm/s; TCD3—altered, TAMMV greater than or equal to 200 cm/s (9). We did not assess brain imaging during the follow-up and none of the participants of the study had a stroke or started new therapies.
Blood samples were collected by venipuncture, after 12 h of fasting, in an ethylene diamine tetraacetic acid (EDTA) tube for hematological profiling and a dry tube without anticoagulant for biochemical analysis, on the same day on which TCD was performed. Hematological data was obtained using a CELL-DYN Ruby Hematology Analyzer (Abbott Diagnostics, Lake Forest, Illinois, United States), and hemoglobin profiling was performed by high-performance liquid chromatography using an HPLC/Variant II Hemoglobin Testing System (Bio-Rad, Hercules, California, United States). Biochemical analyses, such as lipid and profiles, total proteins and fractions, total bilirubin and fractions, lactate dehydrogenase (LDH), alanine transaminase (ALT), aspartate transaminase (AST), and iron serum levels, were investigated using a spectrophotometer autoanalyzer (Biosystems SA, Barcelona, Spain). Ferritin quantification was performed using an Access 2 Immunochemistry System (Beckman Coulter, Inc., Fullerton, CA, United States). C-reactive protein (C-RP) and alpha-1 antitrypsin (AAT) measurements were performed using an Immage 800 system (Beckman Coulter, Fullerton, CA, United States).
A quantitative variable distribution was developed using the Shapiro-Wilk test. The mean values between the two groups were compared using the Mann-Whitney U test for variables with a non-normal distribution or the independent t-test for variables with a normal distribution. Regarding the comparisons of the mean values between more than two groups, the Kruskal-Wallis or ANOVA was used for variables with a non-normal or normal distribution, respectively. Frequencies of qualitative variables were also calculated. The Spearman correlation test was performed to investigate the relationship between the two quantitative variables. Statistical analyses were performed using Epi Info 7.0, GraphPad Prism 6.0, and SPSS 17.0. P-values less than 0.05 were considered statistically significant. Data were expressed as mean ± standard deviation (M ± SD), fifty percentiles (minimum-maximum), and number or frequency where appropriate.
The analysis of demographic data showed that 45 (42.86%) patients were female. The median age was 6.5 ± 3.6 years (range: 2–17 years), 46 (43.39%) patients had ages ranging between 2 and 5 years. Table 1 and Supplementary Figure 1 present the results of laboratory parameters according to TCD. We observed significantly lower TAMMV values in TCD1 patients compared with those with TCD2 or TCD3. The analysis of biomarkers related to hemolysis and hepatic injury showed that patients with TCD3 had significantly reduced RBC, and hematocrit, as well as increased MCV, MCH, and AST compared with those with TCD1 (p < 0.05). Regarding leukocyte count and inflammatory profiles, increased counts of WBC, lymphocytes, monocytes, and AAT levels were observed in the TCD3 group compared with those with TCD1 (p < 0.05). We also observed an increase in glucose levels in the TCD3 group vs. TCD1 group (p < 0.05).
Table 1. Laboratory parameters of children with sickle-cell anemia (SCA) without stroke and HU treatment according to Transcranial Doppler (TCD).
According to the findings presented in Table 1, which showed similar or elevated values of some parameters in the TCD2 and TCD3 groups, we decided to combine these two groups and compare their laboratory parameters with those in the TCD1 group. Results showed that patients with non-normal TCD (TCD2 + TCD3) had significantly reduced RBC, hemoglobin, and hematocrit, as well as increased MCV, MCH, RDW total, and indirect bilirubin, LDH, AST, and ALT, when compared with those with normal TCD (TCD1). Moreover, they presented increased lymphocyte counts, glucose, and AAT levels, as well as a decrease in HDL-C levels (p < 0.05).
Figure 2 shows the results of the correlation analyses. The analysis of the demographic data showed a negative correlation between TAMMV and age, as well as weight (p < 0.05). TAMMV presented a negative correlation with HbF and a positive correlation with HbA2 (p < 0.05). Regarding the hemolysis and hepatic profile, TAMMV was negatively correlated with hemoglobin, RBC, hematocrit, and haptoglobin, as well as positively correlated with reticulocytes, MCV, MCH, RDW, total and indirect bilirubin, LDH, AST and ALT (p < 0.05). An analysis of the lipid profile showed a negative correlation between TAMMV and HDL-C (p < 0.05). Moreover, we also observed a positive correlation between TAMMV and inflammatory profile (WBC, lymphocyte and eosinophil counts, and ferritin levels).
Figure 2. Correlations between TAMMV and laboratory markers in SCA children. Data were obtained using the Spearman correlation rank test. Bars represent the strength of correlation (r values). Gray and black bars indicate a negative and positive correlation, respectively. TAMMV, time-averaged maximum mean velocity; HBF, Fetal hemoglobin; HBA2, normal hemoglobin A2; RBC, red blood cells; MCV, mean corpuscular volume; MCH, mean corpuscular hemoglobin; RDW, red cell distribution width; TB, total bilirubin; IB, indirect bilirubin; LDH, lactate dehydrogenase; AST, aspartate aminotransferase; ALT, alanine aminotransferase; HDL-C, high-density lipoprotein cholesterol; WBC, white blood cells. *p < 0.05, **p < 0.01, ***p < 0.001 and ****p < 0.0001.
In this study, we investigated the association between laboratory biomarkers and cerebral blood flow velocity in SCA children from Bahia State in Brazil. Reports show higher stroke incidence in young children, specifically between 2 and 5 years old (8). During our study, any patient had no type of stroke. This can be explained as most of our patients were over 5 years old. It could also be an underestimate of silent strokes, given that our patients did not have access to magnetic resonance imaging MRI or MRA, which are more sensitive procedures to detect silent infarction (SCI) (25–27). As expected, the analysis of the TAMMV values between the three different groups investigated showed significantly lower TAMMV values in the TCD1 group compared to those with TCD2 or TCD3. This finding corroborates the results of previous studies (8, 28). The cerebral hemodynamics in SCA is quite complex and has been studied to better understand how it influences the risk of stroke and to consider appropriate therapeutic strategies (29, 30). Elevated cerebral blood flow velocity (CBFV), in patients with SCA, has been related to severe anemia and cerebral vasodilatation (31). Moreover, in the context of cardiovascular disease, anemia is associated with the occurrence of cerebrovascular events (32, 33). Hypoxic conditions are associated with increased CBF velocity and a dilation of the brain vasculature to maintain tissue oxygenation (30, 34).
In this study, laboratory biomarker analysis showed that children with non-normal TCD (TCD2 and/or TCD3 group) had significantly reduced RBC, hemoglobin, and hematocrit when compared with the group with normal TCD (TCD1). These findings corroborate the results from Adams et al., who also demonstrated the association between the reduction in these hemolytic markers and the increase in CBFV (28). Reports suggested anemia as an important risk factor for stroke event occurrence (8, 35, 36). Indeed, the compensatory response to anemia in SCA may lead to an increase in CBFV due to vasodilatation of the cerebral vasculature (29, 37–39). Chan and Ganasekaran explained that anemia can cause alterations in cerebral blood vessels, and change oxygenation in the brain, thereby increasing stroke risk (40). Moreover, children with non-normal TCD exhibited higher MCH, MCV, and RDW, which may be explained by the severe anemia in this group, when compared with those with normal TCD. This finding confirms a previous report, suggesting the lower MCH as a protective marker regarding stroke occurrence (41). When higher MCH is predictive of stroke occurrence, higher MCV was reported as a death risk marker post-stroke, in the short term (42). Regarding RDW, its increase is known to be a predictor of poor stroke outcomes (43, 44). Previous studies conducted in the Brazilian population showed associations between reductions in MCH and MCV levels and alpha thalassemia prevalence (45–47). α-thalassemia was shown to present a protective effect against the risk of abnormal TCD in Nigerian children with SCA (48). Moreover, studies conducted in HbSC and HbSS patients did not observe any association between α-thalassemia and TAMMV (49, 50).
Children with non-normal TCD also had a significant increase in AST levels compared with to those with normal TCD, which may be a risk factor for stroke occurrence as suggested by a previous study (51). Moreover, TCD3 group presented significantly elevated total and indirect bilirubin, ALT, and LDH levels, when compared with the TCD1 group, corroborating the previous data, suggesting a more exacerbated hemolytic profile in the TCD3 group than TCD1 group. Moreover, these data corroborate findings from the study by O’Driscoll et al., which also found a positive correlation between LDH and TAMMV (51).
Analysis of leukocyte and inflammatory profiles showed significantly increased counts of WBC, lymphocytes, and monocytes, and AAT levels in the TCD3 group, when compared with those in TCD1. These findings corroborate a previous study, which found a positive correlation between leukocytes and TAMMV (52). During the cerebral ischemic mechanism, activated leukocytes are highly implicated and are considered a predictor of the outcome (53). Moreover, children with non-normal TCD present increased lymphocyte counts in compared with children with normal TCD. The increase in these inflammatory biomarkers, specifically in the TCD3 group when compared to the TCD1 group, besides to confirming the association of these biomarkers with stroke risk, suggests an association between recruitment of activated-inflammatory cells and acute stroke occurrence (43, 54). Moreover, studies found elevated leukocyte counts in patients who suffered a stroke when compared with controls (43, 53). Indeed, the exacerbated inflammatory condition, involving recruitment, activation, and adhesion of these inflammatory cells to the cerebral vascular endothelial is considered one of the main mechanisms underlying the increase in blood velocity and consequently stroke events (35). Interestingly, we observed an increase in glucose levels in the TCD3 group when compared to TCD1 group. Corroborating this finding, a previous study also demonstrated elevated glucose levels in patients with SCD in the period before and after acute stroke events (55). Although the relationship between glucose and stroke events is unclear, hyperglycemia is known to worsen stroke outcomes due to its association with increases in brain injury (55). Moreover, studies have shown that hyperglycemia increases coagulation, by promoting thrombin production (56), as well as brain tissue damage (57).
Correlation analyses showed a negative correlation between TAMMV and age, corroborating the findings of previous studies, where the TAMMV decreases as the children get older (52, 58). A Nigerian study reported that CBFV peaks during the first 6 years and tends to decline with advancing age (59). Generally, the decline in CBFV with age may be explained by changes in cerebrovascular hemodynamics linked to less metabolic demands (60). According to our findings, children with elevated weight are susceptible to an increase in TAMMV, consequently stroke risk. However, this finding deserves to be better investigated. A study in Jamaica showed a similar correlation but not sustained when they associated TAMV with BMI z-score or height-age z-score (61). We also observed a negative correlation between TAMMV and HbF and, its positive correlation with HbA2. This finding corroborates the association between higher HbF levels and a reduction in the occurrence of silent cerebral infarctions and overt strokes (62, 63). Analyses also demonstrated negative correlations between TAMMV and hemoglobin, RBC, and hematocrit, as well as positive correlations between TAMMV and reticulocytes, MCV, MCH, RDW, total and indirect bilirubin, LDH, AST, and ALT. Previous studies found a negative correlation between TAMMV and hematocrit levels (64, 65); (66, 67) reported that higher serum LDH levels may predict poor outcomes of Aneurysmal Subarachnoid Hemorrhage, while other studies found positive correlation between LDH and TAMM velocity.
Some studies have related the permeability of the Blood-brain barrier by the free bilirubin (BF), then, mechanisms of inflammatory mediators released by astrocytes activated by unconjugated bilirubin (UCB), may contribute to cerebral vasodilation. Also, an increase in CBFV was observed in neonates with hyperbilirubinemia (68, 69).
Serum ALT levels showed significant positive correlations with CBFV in the superior frontal gyrus and negative correlations with CBFV in the middle occipital gyrus, angular gyrus, precuneus, and middle temporal gyrus. But no association with AST (70).
Results also demonstrated a positive correlation between TAMMV and inflammatory biomarkers (WBC, lymphocyte and eosinophil counts, and ferritin levels). Serum ferritin has also been described as a risk factor for stroke in post-menopausal women (71). A previous study demonstrated that increased ferritin levels are correlated with stroke severity and the size of the lesion (72). Furthermore, elevated ferritin levels could be associated with a chronic inflammatory state and chronic hemolytic condition as observed in our population (50). The inflammatory state is evidenced by increases in liver function biomarkers, such as AST, which, in this study, was positively correlated with TAMMV. Regarding the lipid profile, we observed a negative correlation between TAMMV and HDL-C. This finding corroborates a previous study, which suggests an association between higher HDL-C levels and reduced stroke risk (73), which can be explained by the beneficial effects of HDL-C on endothelial function. Previous studies observed significantly increased cerebral CO2 reactivity with increasing levels of serum HDL-C and decreased cerebral CO2 reactivity with an increasing cholesterol/HDL-C ratio (74, 75).
Results of this study highlight the importance of the investigation of hemolytic and inflammatory biomarkers for monitoring the clinical outcome of SCA pediatric patients, to avoid acute or chronic stroke. Moreover, glucose and HDL-C appear to be useful for predicting higher TAMMV i.e., stroke risk. Further studies are needed to understand this relationship and establish a cutoff value, which may be used to identify with precision, in association with hemolytic and inflammatory biomarkers, patients with stroke risk.
The original contributions presented in this study are included in the article/Supplementary material, further inquiries can be directed to the corresponding author.
This study received approval from the Institutional Review Board of the HUPES (protocol number: 287.768/2013). Written informed consent to participate in this study was provided by the participants or their legal guardian/next of kin.
CA and MG conceived and designed the study. CA, SY, RS, SS, JS, TP, MA, and VM collected the samples. CA, SY, RS, SS, DZ, and JS performed the experiments. CA and SY analyzed the data. CA wrote the manuscript. CV, IPL, and IML followed the patients. CV performed the TCD examine. MG supervised the study and critically revised the manuscript. All authors revised and approved the manuscript.
This work was supported by grants from the Fundação de Amparo à Pesquisa do Estado da Bahia (FAPESB) (SUS0034/2013 and 8133/2014 and from the Conselho Nacional de Desenvolvimento Científico e Tecnológico (CNPq) (COSAU 2012. No. 400005/2013-1APQ).
We would like to thank all the children and their legal guardians for their kind participation in this study, as well as personnel at the outpatient of the Professor Edgard Santos University Hospital (HUPES), and personnel of the Departamento de Toxicologia e Análises Clínicas, Faculdade de Farmácia (LACTFAR-UFBA), for their support.
The authors declare that the research was conducted in the absence of any commercial or financial relationships that could be construed as a potential conflict of interest.
All claims expressed in this article are solely those of the authors and do not necessarily represent those of their affiliated organizations, or those of the publisher, the editors and the reviewers. Any product that may be evaluated in this article, or claim that may be made by its manufacturer, is not guaranteed or endorsed by the publisher.
The Supplementary Material for this article can be found online at: https://www.frontiersin.org/articles/10.3389/fped.2022.914466/full#supplementary-material
1. Armstrong FD, Thompson RJ, Wang W, Zimmerman R, Pegelow CH, Miller S, et al. Cognitive functioning and brain magnetic resonance imaging in children with sickle cell disease. Neuropsychology committee of the cooperative study of sickle cell disease. Pediatrics. (1996) 97:864–70.
2. Adams RJ. Stroke prevention and treatment in sickle cell disease. Arch Neurol. (2001) 58:565–8. doi: 10.1001/archneur.58.4.565
3. Bhattacharya P, Sarmah D, Dave KR, Goswami A, Watanabe M, Wang X, et al. Stroke and stroke prevention in sickle cell anemia in developed and selected developing countries. J Neurol Sci. (2021) 427:117510. doi: 10.1016/j.jns.2021.117510
4. Switzer JA, Hess DC, Nichols FT, Adams RJ. Pathophysiology and treatment of stroke in sickle-cell disease: Present and future. Lancet Neurol. (2006) 5:501–12. doi: 10.1016/S1474-4422(06)70469-0
5. Mallon D, Doig D, Dixon L, Gontsarova A, Jan W, Tona F Neuroimaging in sickle cell disease: a review. J Neuroimaging. (2020) 30:725–35. doi: 10.1111/jon.12766
6. Kirkham FJ, Lagunju IA Epidemiology of stroke in sickle cell disease. J Clin Med. (2021) 10:4232. doi: 10.3390/jcm10184232
7. Hoppe C, Klitz W, Cheng S, Apple R, Steiner L, Robles L, et al. Gene interactions and stroke risk in children with sickle cell anemia. Blood. (2004) 103:2391–6. doi: 10.1182/blood-2003-09-3015
8. Ohene-Frempong K, Weiner SJ, Sleeper LA, Miller ST, Embury S, Moohr JW, et al. Cerebrovascular Accidents in sickle cell disease: Rates and risk factors. Blood. (1998) 91:288–94.
9. Schlotman AA, Donahue MJ, Kassim AA, Lee CA, Waddle SL, Pruthi S, et al. Intracranial and extracranial vascular stenosis as risk factors for stroke in sickle cell disease. Pediatr Neurol. (2021) 114:29–34. doi: 10.1016/j.pediatrneurol.2020.10.006
10. Stuart MJ, Nagel RL. Sickle-cell disease. Lancet. (2004) 364:1343–60. doi: 10.1016/S0140-6736(04)17192-4
11. Kato GJ, Gladwin MT, Steinberg MH. Deconstructing sickle cell disease: Reappraisal of the role of hemolysis in the development of clinical subphenotypes. Blood Rev. (2007) 21:37–47. doi: 10.1016/j.blre.2006.07.001
12. Kato GJ, Steinberg MH, Gladwin MT. Intravascular hemolysis and the pathophysiology of sickle cell disease. J Clin Invest. (2017) 127:750–60. doi: 10.1172/JCI89741
13. Sundd P, Gladwin MT, Novelli EM. Pathophysiology of sickle cell disease. Annu Rev Pathol. (2019) 14:263–92. doi: 10.1146/annurev-pathmechdis-012418-012838
14. Ware RE, Davis BR, Schultz WH, Brown RC, Aygun B, Sarnaik S, et al. Hydroxycarbamide versus chronic transfusion for maintenance of transcranial doppler flow velocities in children with sickle cell anaemia—TCD With Transfusions Changing to Hydroxyurea (TWiTCH): A multicentre, open-label, phase 3, non-inferiority trial. Lancet. (2016) 387:661–70. doi: 10.1016/S0140-6736(15)01041-7
15. Hasson C, Veling L, Rico J, Mhaskar R. The role of hydroxyurea to prevent silent stroke in sickle cell disease. Medicine. (2019) 98:e18225. doi: 10.1097/MD.0000000000018225
16. Adams RJ. TCD in sickle cell disease: An important and useful test. Pediatr Radiol. (2005) 35:229–34. doi: 10.1007/s00247-005-1409-7
17. Adams R, McKie V, Nichols F, Carl E, Zhang D-L, McKie K, et al. The use of transcranial ultrasonography to predict stroke in sickle cell disease. N Engl J Med. (1992) 326:605–10. doi: 10.1056/NEJM199202273260905
18. Buchanan ID, James-Herry A, Osunkwo I. The other side of abnormal: A case series of low transcranial Doppler velocities associated with stroke in children with sickle cell disease. J Pediatr Hematol Oncol. (2013) 35:543–6. doi: 10.1097/MPH.0b013e318279caae
19. Kirkham FJ, Hewes DK, Prengler M, Wade A, Lane R, Evans JP. Nocturnal hypoxaemia and central-nervous-system events in sickle-cell disease. Lancet. (2001) 357:1656–9. doi: 10.1016/s0140-6736(00)04821-2
20. Silva M, Vargas S, Coelho A, Ferreira E, Mendonça J, Vieira L, et al. Biomarkers and genetic modulators of cerebral vasculopathy in sub-Saharan ancestry children with sickle cell anemia. Blood Cells Mol Dis. (2020) 83:102436. doi: 10.1016/j.bcmd.2020.102436
21. Hoppe C. Defining stroke risk in children with sickle cell anaemia. Br J Haematol. (2005) 128:751–66. doi: 10.1111/j.1365-2141.2004.05310.x
22. Vizioli L, Muscari S, Muscari A. The relationship of mean platelet volume with the risk and prognosis of cardiovascular diseases. Int J Clin Pract. (2009) 63:1509–15. doi: 10.1111/j.1742-1241.2009.02070.x
23. Estcourt LJ, Fortin PM, Hopewell S, Trivella M, Doree C, Abboud MR. Interventions for preventing silent cerebral infarcts in people with sickle cell disease. Cochrane Database Syst Rev. (2017) 5:CD012389. doi: 10.1002/14651858.CD012389.pub2
24. Belisário AR, Silva CM, Velloso-Rodrigues C, Viana MB, Belisário AR, Silva CM, et al. Genetic, laboratory and clinical risk factors in the development of overt ischemic stroke in children with sickle cell disease. Hematol Transfus Cell Ther. (2018) 40:166–81. doi: 10.1016/j.bjhh.2017.08.008
25. Moser FG, Miller ST, Bello JA, Pegelow CH, Zimmerman RA, Wang WC, et al. The spectrum of brain MR abnormalities in sickle-cell disease: A report from the cooperative study of sickle cell disease. Am J Neuroradiol. (1996) 17:965–72.
26. Bernaudin F, Verlhac S, Arnaud C, Kamdem A, Chevret S, Hau I, et al. Impact of early transcranial Doppler screening and intensive therapy on cerebral vasculopathy outcome in a newborn sickle cell anemia cohort. Blood. (2011) 117:1130–40. doi: 10.1182/blood-2010-06-293514
27. DeBaun MR, Armstrong FD, McKinstry RC, Ware RE, Vichinsky E, Kirkham FJ. Silent cerebral infarcts: A review on a prevalent and progressive cause of neurologic injury in sickle cell anemia. Blood. (2012) 119:4587–96. doi: 10.1182/blood-2011-02-272682
28. Adams RJ, Kutlar A, McKie V, Carl E, Nichols FT, Liu JC, et al. Alpha thalassemia and stroke risk in sickle cell anemia. Am J Hematol. (1994) 45:279–82. doi: 10.1002/ajh.2830450402
29. Guilliams KP, Fields ME, Dowling MM. Advances in understanding ischemic stroke physiology and the impact of vasculopathy in children with sickle cell disease. Stroke. (2019) 50:266–73. doi: 10.1161/STROKEAHA.118.020482
30. Prussien KV, Compas BE, Siciliano RE, Ciriegio AE, Lee CA, Kassim AA, et al. Cerebral hemodynamics and executive function in sickle cell anemia. Stroke. (2021) 52:1830–4. doi: 10.1161/STROKEAHA.120.032741
31. Mozos I. Mechanisms linking red blood cell disorders and cardiovascular diseases. BioMed Res Int. (2015) 2015:682054. doi: 10.1155/2015/682054
32. Sabatine MS, Morrow DA, Giugliano RP, Burton PBJ, Murphy SA, McCabe CH, et al. Association of hemoglobin levels with clinical outcomes in acute coronary syndromes. Circulation. (2005) 111:2042–9. doi: 10.1161/01.CIR.0000162477.70955.5F
33. Lasek-Bal A, Holecki M, Stêposz A, Duława J. The impact of anemia on the course and short-term prognosis in patients with first ever ischemic stroke. Neurol Neurochir Pol. (2015) 49:107–12. doi: 10.1016/j.pjnns.2015.03.001
34. Afzali-Hashemi L, Baas KPA, Schrantee A, Coolen BF, van Osch MJP, Spann SM, et al. Impairment of cerebrovascular hemodynamics in patients with severe and milder forms of sickle cell disease. Front Physiol. (2021) 12:645205. doi: 10.3389/fphys.2021.645205
35. Verduzco LA, Nathan DG. Sickle cell disease and stroke. Blood. (2009) 114:5117–25. doi: 10.1182/blood-2009-05-220921
36. Sico JJ, Concato J, Wells CK, Lo AC, Nadeau SE, Williams LS, et al. Anemia is associated with poor outcomes in patients with less severe ischemic stroke. J Stroke Cerebrovasc Dis. (2013) 22:271–8. doi: 10.1016/j.jstrokecerebrovasdis.2011.09.003
37. van den Tweel XW, Nederveen AJ, Majoie CBLM, van der Lee JH, Wagener-Schimmel L, van Walderveen MAA, et al. Cerebral blood flow measurement in children with sickle cell disease using continuous arterial spin labeling at 3.0-Tesla MRI. Stroke. (2009) 40:795–800. doi: 10.1161/STROKEAHA.108.523308
38. Dowling MM, Quinn CT, Plumb P, Rogers ZR, Rollins NK, Koral K, et al. Acute silent cerebral ischemia and infarction during acute anemia in children with and without sickle cell disease. Blood. (2012) 120:3891–7. doi: 10.1182/blood-2012-01-406314
39. Kosinski PD, Croal PL, Leung J, Williams S, Odame I, Hare GMT, et al. The severity of anaemia depletes cerebrovascular dilatory reserve in children with sickle cell disease: A quantitative magnetic resonance imaging study. Br J Haematol. (2017) 176:280–7. doi: 10.1111/bjh.14424
40. Chan T, Ganasekaran G. The effect of anemia on the functional outcomes of the stroke patients and the efficiency of their stroke rehabilitation. J Stroke Cerebrovasc Dis. (2015) 24:1438–42. doi: 10.1016/j.jstrokecerebrovasdis.2015.03.009
41. Rees DC, Lambert C, Cooper E, Bartram J, Goss D, Deane C, et al. Glucose 6 phosphate dehydrogenase deficiency is not associated with cerebrovascular disease in children with sickle cell anemia. Blood. (2009) 114:742–3. doi: 10.1182/blood-2009-04-216861
42. Hatamian H, Saberi A, Pourghasem M. The relationship between stroke mortality and red blood cell parameters. Iran J Neurol. (2014) 13:237–40.
43. Furlan JC, Vergouwen MDI, Fang J, Silver FL. White blood cell count is an independent predictor of outcomes after acute ischaemic stroke. Eur. J. Neurol. (2014) 21:215–22. doi: 10.1111/ene.12233
44. Feng G-H, Li H-P, Li Q-L, Fu Y, Huang R-B. Red blood cell distribution width and ischaemic stroke. Stroke Vasc Neurol. (2017) 2:172–5. doi: 10.1136/svn-2017-000071
45. Adorno EV, Couto FD, Moura Neto JP, Menezes JF, Rêgo M, Reis MG, et al. Hemoglobinopathies in newborns from Salvador, Bahia, Northeast Brazil. Cad Saude Publica. (2005) 21:292–8. doi: 10.1590/s0102-311x2005000100032
46. Aleluia MM, da Guarda CC, Santiago RP, Fonseca TCC, Neves FI, de Souza RQ, et al. Association of classical markers and establishment of the dyslipidemic sub-phenotype of sickle cell anemia. Lipids Health Dis. (2017) 16:74. doi: 10.1186/s12944-017-0454-1
47. Anselmo FC, Soumanou AG, de Aguiar Ferreira C, Sobrinha FMV, Castro ACS, Brito RO, et al. Detection of common deletional of α-Thalassemia 3.7 Kb from metropolitan region of manaus, amazonas, Brazil. Mediterr J Hematol Infect Dis. (2021) 13:e2021001. doi: 10.4084/MJHID.2021.001
48. Fasola FA, Babalola OA, Brown BJ, Odetunde A, Falusi AG, Olopade O. The effect of alpha thalassemia, HbF and HbC on haematological parameters of sickle cell disease patients in Ibadan, Nigeria. Mediterr J Hematol Infect Dis. (2022) 14:e2022001. doi: 10.4084/MJHID.2022.001
49. Miller ST, Rieder RF, Rao SP, Brown AK. Cerebrovascular accidents in children with sickle-cell disease and alpha-thalassemia. J Pediatr. (1988) 113:847–9. doi: 10.1016/s0022-3476(88)80013-1
50. Santiago RP, Vieira C, Adanho CSA, Santana SS, Guarda CC, Figueiredo CVB, et al. Laboratory and genetic biomarkers associated with cerebral blood flow velocity in hemoglobin SC disease. Dis. Markers. (2017) 2017:6359871. doi: 10.1155/2017/6359871
51. O’Driscoll S, Height SE, Dick MC, Rees DC. Serum lactate dehydrogenase activity as a biomarker in children with sickle cell disease. Br J Haematol. (2008) 140:206–9. doi: 10.1111/j.1365-2141.2007.06894.x
52. Chukwu B, Menezes L, Fukuda T, Filho J, Goncalves M. Timed average mean maximum velocity (TAMMV) of cerebral blood flow of children and adolescents with sickle cell disease: Correlation with clinical and hematological profiles in country. Malawi Med J. (2021) 33:169–77. doi: 10.4314/mmj.v33i3.4
53. Quan K, Wang A, Zhang X, Wang Y. Leukocyte count and adverse clinical outcomes in acute ischemic stroke patients. Front Neurol. (2019) 10:1240. doi: 10.3389/fneur.2019.01240
54. Belisário AR, Sales RR, Toledo NE, Muniz MB, de SR, Velloso-Rodrigues C, et al. Reticulocyte count is the most important predictor of acute cerebral ischemia and high-risk transcranial Doppler in a newborn cohort of 395 children with sickle cell anemia. Ann Hematol. (2016) 95:1869–80. doi: 10.1007/s00277-016-2789-5
55. Lindsberg PJ, Roine RO. Hyperglycemia in acute stroke. Stroke. (2004) 35:363–4. doi: 10.1161/01.STR.0000115297.92132.84
56. Hafez S, Coucha M, Bruno A, Fagan SC, Ergul A. Hyperglycemia, acute ischemic stroke and thrombolytic therapy. Transl Stroke Res. (2014) 5:442–53. doi: 10.1007/s12975-014-0336-z
57. Helgason CM. Blood glucose and stroke. Curr Treat Options Cardiovasc Med. (2012) 14:284–7. doi: 10.1007/s11936-012-0178-5
58. Adekunle MO, Animasahun AB, Diaku-Akinwumi IN, Njokanma OF. Pattern of cerebral blood flow velocity using transcranial Doppler ultrasonography in children with sickle cell disorder in lagos state Nigeria. Mediterr J Hematol Infect Dis. (2017) 9:e2017050. doi: 10.4084/MJHID.2017.050
59. Lagunju I, Sodeinde O, Brown B, Akinbami F, Adedokun B. Transcranial Doppler ultrasonography in children with sickle cell anemia: Clinical and laboratory correlates for elevated blood flow velocities. J Clin Ultrasound. (2014) 42:89–95. doi: 10.1002/jcu.22099
60. Torbey MT, Hauser T-K, Bhardwaj A, Williams MA, Ulatowski JA, Mirski MA, et al. Effect of age on cerebral blood flow velocity and incidence of vasospasm after aneurysmal subarachnoid hemorrhage. Stroke. (2001) 32:2005–11. doi: 10.1161/hs0901.094622
61. Rankine-Mullings AE, Morrison-Levy N, Soares D, Aldred K, King L, Ali S, et al. Transcranial doppler velocity among jamaican children with sickle cell anaemia: determining the significance of haematological values and nutrition. Br J Haematol. (2018) 181:242–51. doi: 10.1111/bjh.15162
62. Adekile AD, Yacoub F, Gupta R, Sinan T, Haider MZ, Habeeb Y, et al. Silent brain infarcts are rare in Kuwaiti children with sickle cell disease and high Hb F. Am J Hematol (2002) 70:228–31. doi: 10.1002/ajh.10143
63. Ismail A, Yusuf AA, Kuliya-Gwarzo A, Ahmed SG, Tabari AM, Abubakar SA. Correlating transcranial arterial Doppler velocities with haematologic parameters and haemolytic indices of Nigerian children with sickle cell anaemia. Ultrasound. (2019) 27:101–10. doi: 10.1177/1742271X19836264
64. Vorstrup S, Lass P, Waldemar G, Brandi L, Schmidt JF, Johnsen A, et al. Increased cerebral blood flow in anemic patients on long-term hemodialytic treatment. J Cereb Blood Flow Metab. (1992) 12:745–9. doi: 10.1038/jcbfm.1992.105
65. Zheng S, Wang H, Chen G, Shangguan H, Yu L, Lin Z, et al. Higher serum levels of lactate dehydrogenase before microsurgery predict poor outcome of aneurysmal subarachnoid hemorrhage. Front Neurol. (2021) 12:720574. doi: 10.3389/fneur.2021.720574
66. Ojewunmi OO, Adeyemo TA, Osuntoki AA, Imaga NA, Oyetunji AI. Haemoglobin oxygen saturation, leucocyte count and lactate dehydrogenase are predictors of elevated cerebral blood flow velocity in Nigerian children with sickle cell anaemia. Paediatr Int Child Health. (2018) 38:34–9. doi: 10.1080/20469047.2017.1311087
67. Apollonsky N, Lerner NB, Zhang F, Raybagkar D, Eng J, Tarazi R. Laboratory biomarkers, cerebral blood flow velocity, and intellectual function in children with sickle cell disease. Adv Hematol. (2020) 2020:e8181425. doi: 10.1155/2020/8181425
68. Saunders NR, Liddelow SA, Dziegielewska KM. Barrier mechanisms in the developing brain. Front Pharmacol. (2012) 3:46. doi: 10.3389/fphar.2012.00046
69. Basu S, De D, Shukla RC, Kumar A. Difference in cerebral blood flow velocity in neonates with and without hyperbilirubinemia. J Epidemiol Glob Health. (2014) 4:97–106. doi: 10.1016/j.jegh.2013.09.008
70. Chen J, Liu S, Wang C, Zhang C, Cai H, Zhang M, et al. Associations of serum liver function markers with brain structure, function, and perfusion in healthy young adults. Front Neurol. (2021) 12:606094. doi: 10.3389/fneur.2021.606094
71. Van der A DL, Grobbee DE, Roest M, Marx JJM, Voorbij HA, van der Schouw YT. Serum ferritin is a risk factor for stroke in postmenopausal women. Stroke. (2005) 36:1637–41. doi: 10.1161/01.STR.0000173172.82880.72
72. Erdemoglu AK, Ozbakir S. Serum ferritin levels and early prognosis of stroke. Eur J Neurol. (2002) 9:633–7. doi: 10.1046/j.1468-1331.2002.00472.x
73. Amarenco P, Labreuche J. Lipid management in the prevention of stroke: Review and updated meta-analysis of statins for stroke prevention. Lancet Neurol. (2009) 8:453–63. doi: 10.1016/S1474-4422(09)70058-4
74. Bakker SL, de Leeuw FE, Koudstaal PJ, Hofman A, Breteler MM. Cerebral CO2 reactivity, cholesterol, and high-density lipoprotein cholesterol in the elderly. Neurology. (2000) 54:987–9. doi: 10.1212/wnl.54.4.987
Keywords: sickle cell anemia, stroke, Transcranial Doppler, laboratory parameters, TAMMV
Citation: Adanho CSA, Yahouédéhou SCMA, Santana SS, Vieira C, Santiago RP, de Santana JM, Pitanga TN, Aleluia MM, Maffili VV, Leite IPR, Zanette DL, Lyra IM and Goncalves MS (2022) Association of laboratory markers and cerebral blood flow among sickle cell anemia children. Front. Pediatr. 10:914466. doi: 10.3389/fped.2022.914466
Received: 06 April 2022; Accepted: 10 August 2022;
Published: 26 August 2022.
Edited by:
Monica Hulbert, Washington University in St. Louis, United StatesReviewed by:
Sivakumar Nuvvula, Narayana Dental College and Hospital, IndiaCopyright © 2022 Adanho, Yahouédéhou, Santana, Vieira, Santiago, de Santana, Pitanga, Aleluia, Maffili, Leite, Zanette, Lyra and Goncalves. This is an open-access article distributed under the terms of the Creative Commons Attribution License (CC BY). The use, distribution or reproduction in other forums is permitted, provided the original author(s) and the copyright owner(s) are credited and that the original publication in this journal is cited, in accordance with accepted academic practice. No use, distribution or reproduction is permitted which does not comply with these terms.
*Correspondence: Marilda Souza Goncalves, bWFyaWxkYS5nb25jYWx2ZXNAZmlvY3J1ei5icg==
Disclaimer: All claims expressed in this article are solely those of the authors and do not necessarily represent those of their affiliated organizations, or those of the publisher, the editors and the reviewers. Any product that may be evaluated in this article or claim that may be made by its manufacturer is not guaranteed or endorsed by the publisher.
Research integrity at Frontiers
Learn more about the work of our research integrity team to safeguard the quality of each article we publish.