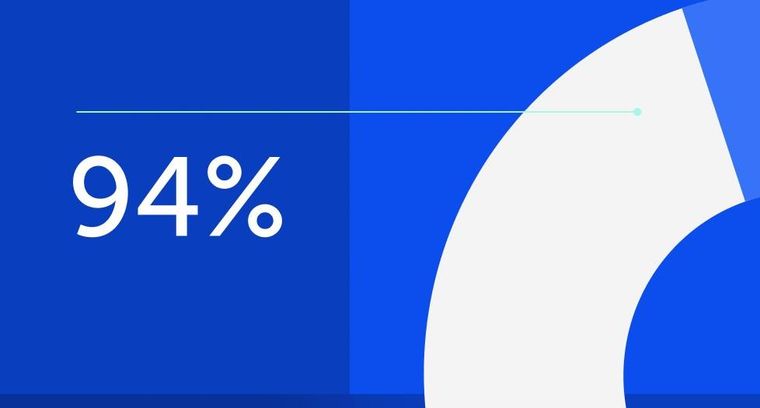
94% of researchers rate our articles as excellent or good
Learn more about the work of our research integrity team to safeguard the quality of each article we publish.
Find out more
MINI REVIEW article
Front. Pediatr., 06 June 2022
Sec. Pediatric Pulmonology
Volume 10 - 2022 | https://doi.org/10.3389/fped.2022.908607
This article is part of the Research TopicInsights in Pediatric Pulmonology: 2021View all 15 articles
Background: The burden of respiratory disease is high in low-middle income countries (LMIC). Pulmonary function tests are useful as an objective measure of lung health and to track progression. Spirometry is the commonest test, but its use is limited in preschool children. Other lung function methods have been developed but their use in LMIC has not been well described.
Aim: To review the use of preschool lung function testing in children in LMIC, with particular reference to feasibility and clinical applications.
Methods: Electronic databases “PubMed”, “Scopus”,” Web of Science”, and “EBSCO host” were searched for publications in low and middle income countries on preschool lung function testing, including spirometry, fractional exhaled nitric oxide (FeNO), oscillometry, interrupter technique, tidal breathing and multiple breath washout (MBW), from 1 January 2011 to 31 January 2022. Papers in English were included and those including only children ≥6 years were excluded.
Result: A total of 61 papers from LMIC in Asia, South America, Africa, Eurasia or the Middle East were included. Of these, 40 included spirometry, 7 FeNO, 15 oscillometry, 2 interrupter technique, and 2 tidal breathing. The papers covered test feasibility (19/61), clinical application (46/61) or epidemiological studies (13/61). Lung function testing was successful in preschool children from LMIC. Spirometry was the most technically demanding and success gradually increased with age.
Conclusion: Preschool lung function testing is under-represented in LMIC for the burden of respiratory disease. These tests have the potential to strengthen respiratory care in LMIC, however access needs to be improved.
Childhood respiratory disease is a common cause of morbidity and mortality globally (1). The burden of acute and chronic respiratory disease is especially high in low-middle income countries (LMIC) (2), may result in impaired lung function and set a trajectory for chronic illness into adulthood (3, 4). However, access to respiratory diagnostic and management tools such as lung function are limited in many LMIC (5).
Lung function attained in early life is important for respiratory health, with low lung function associated with subsequent risk of respiratory disease (6). Pulmonary function tests are an objective measure of lung health which can be used to diagnose and track lung disease and assess response to treatment. In recent years non-invasive tests have been developed and guidelines produced for preschool children, facilitating its use in assessing respiratory health in early life (7, 8).
Lung function tests used in preschool children include spirometry, bronchial response testing, multiple breath washout (MBW), fractional exhaled nitric oxide (FeNO), oscillometry and other tests which measure resistance, including the interrupter technique (Rint). and plethysmography. With increased recognition of the importance of maximizing early life respiratory health and the growing availability of tools to do so, their use in LMIC is of particular interest.
Of the preschool lung function tests, spirometry is the most commonly used and most widely available. Even though use is limited in very young or uncooperative children, it is feasible in children as young as 3 years (9). Spirometry measures lung volumes at maximal expiration and is able to assess airflow obstruction, response to bronchodilator therapy and lung volumes on forced expiration. The commonly reported measures are the forced expiratory volume in the first second (FEV1), forced vital capacity (FVC), FEV1/FVC and forced expiratory flow between 25 and 75% of FVC (FEF25−75). (10). Spirometry predominantly reflects airflow in large and medium sized airways, and is a poor measure of peripheral small airways or early lung disease (11). Current international recommendations for spirometry collection and interpretation in young children are available (10, 12).
Oscillometry (or the forced oscillation technique, FOT). is a simple, non-invasive technique which is performed during tidal breathing. Minimal co-operation is required thereby making this a popular measurement in preschool children. This measures the impedance of the respiratory system, which includes resistance and reactance across a range of frequencies reflecting the entire respiratory system including the small airways (13). The novel intra-breath measurement may be a more sensitive measure of small airway disease, thus allowing early detection of disease (14, 15). Recent international recommendations for oscillometry have been published on methodology, technical standards and future developments for use in children (7, 16, 17).
The interrupter technique measures the resistance of the respiratory system requiring minimal cooperation. The technique involves a sudden interruption of flow during tidal breathing, this allows for alveolar and pressure at the mouth to equilibrate therefore alveolar pressure can be estimated (18, 19). Different methods have been used to perform the test making the comparison of results difficult thus highlighting the need for test standardization. Furthermore, research is still required to determine the best algorithm to calculate pressure at the mouth during occlusion and the cut-off value for Rint post bronchodilator needs to be established (7).
Multiple breath washout (MBW) is used to assess ventilation homogeneity. It measures the functional residual capacity (FRC) and the lung clearance index (LCI) in preschool children (8). It is more sensitive than spirometry for the detection of peripheral airway disease and has been successfully used in the monitoring of children with cystic fibrosis (CF) and primary ciliary dyskinesia (PCD) (7, 20). It correlates with high resolution CT scan in CF patients as well as in children with asthma, bronchiolitis obliterans or chronic lung disease of prematurity (8, 21).
Fractional exhaled nitric oxide (FeNO) is a non-invasive marker of T-helper cell type 2 (TH2). eosinophilic airway inflammation. Tests can be performed with high repeatability and accuracy (22–24). Its main use is an adjunct to the diagnosis of TH2 type asthma and guiding the use of inhaled corticosteroid (ICS) treatment. In addition, FeNO can also assist in the differential diagnosis of other conditions such as cystic fibrosis (CF), PCD, scleroderma, obstructive sleep apnoea syndrome and hepatopulmonary syndrome. Fractional exhaled nitric oxide levels are low in PCD; however measurement of nasal NO improves the diagnostic accuracy and is a useful screening tool for PCD (23, 25). Normal values for FeNO are published for children from 4 years of age (24), as well as international applications and the use of FeNO (23, 24, 26). However, there are limited data on the use and feasibility of such measures in LMIC, despite the high burden of lung disease and need for objective tools to diagnose and monitor these.
We aimed to review the use of preschool lung function testing in children in LMIC, with reference to feasibility and clinical applications, to identify opportunities for optimizing diagnosis and management of childhood respiratory disease in these settings.
We reviewed published literature of preschool lung function testing in LMIC, which included children between the ages of 3 to 5 years. We included published papers from 1 January 2011 to 31 January 2022 that included lung function testing in the preschool age group from a World Bank defined LMIC.
The search was conducted on the following electronic databases: PubMed, Scopus, EBSCOhost (Cinahl, Africa wide information, Health source- Nursing/Academic Edition). and Web of Science including the search terms: Respiratory Function Test*” OR “Lung function test*” OR “pulmonary function test*” OR “respiratory function test*” OR “multiple-breath washout” OR “Forced oscillation technique” OR” tidal breathing” OR “fractional excretion of nitric oxide” OR Spirometry OR Oscillometry OR “impulse oscillometry” OR “interrupter technique” OR “interrupter resistance” AND “preschool child*”. Full search strategy can be seen in Supplementary Table S1. Reviews, editorials, case reports and conference proceedings were excluded. Any papers including only children ≥ 6 years were excluded. Abstracts of identified documents were reviewed and screened by SC, with second author DG assisting with inclusion queries. All included papers were reviewed by SC.
A total of 626 papers were screened of which 61 were eligible for inclusion, Supplementary Figure S1. They included papers from 4 regions: 30 (49.2%) from Asia, 17 (27.9%) from South America, 9 (14.7%) from Africa and 5 (8.2%) from Europe and the Middle East. Five lung function tests were most commonly reported: 40 (65.6%) spirometry, 7 (11.5%) FeNO, 15 (24.6%) oscillometry, 2 (3.3%) interrupter technique and 2 (3.3%) tidal breathing measurements. There were no papers including MBW. The papers covered test feasibility (19, 31.1%), clinical applications (46, 75.4%) and epidemiological studies (13, 21.3%). Clinical studies focused on development of reference tools (19/46, 41.3%). and on specific diseases: asthma (15/46, 32.6%), of which 4 papers in addition to asthma included allergic rhinitis, air pollution, obesity and allergic bronchopulmonary aspergillosis (ABPA); CF (5/46, 10.9%); 1 bronchiolitis obliterans, 1 recurrent wheeze, 1 systemic sclerosis. Epidemiological studies assessed the impact of air pollution (10/13, 77%); electronic waste (e-waste) (2/13, 15.4%) (27, 28) and the effect of antenatal omega 3 fatty acid supplementation (1/13, 7.7%) on lung function. E-waste is accumulated discarded or broken electronic devices which is becoming the largest amount of waste in the world (27, 28). As spirometry, FeNO, oscillometry or tidal breathing were most commonly used they were the focus of this review.
The majority (40, 68%) of papers included spirometry, (Table 1). Spirometry was used to develop reference equations, diagnose and manage respiratory diseases including asthma or CF, assess the impact of air pollution, electronic exposures (e-exposures) or socio-economic status on lung function. Twenty (50%) studies were from Asia, 9 (22.5%) from South America, 7 (17.5%). from Africa and 4 (10%) from Europe and the Middle East.
Success rates for spirometry in preschool children increased with age (29). Children between 4–6 years of age achieved a success rate between 82–85%, (30–32). while children between 3–5 years and 3–6 years of age, success rates were 68.4% and 42% respectively (29, 33).
A number of reference ranges were generated for individual population groups in LMIC, which were compared to either local reference ranges and/or The Global Lung Function Initiative (GLI) 2012 equation (32–39). Many resulted in an over estimation/underestimation of lung function for the population assessed, highlighting the complexity of population differences in lung function. A Nigerian study determined reference equations for children with sickle cell anemia (40). Published international equations also used arm span to determine height for spirometry (41). The GLI 2012 “Caucasian” provided a reasonable fit for Jordanian children (29). When assessing spirometry in two groups of healthy children with Indian ancestry, one living in UK and the other in India, the GLI equations for best fit differed: the “GLI-Black” equation was most useful for interpreting the South-Asian data and “GLI-Other” for North Indian data (42). Similarly in a South Africa population the GLI 2012 “GLI-Caucasian” provided a good fit for the Caucasian population, ”GLI-Black “ and “GLI-Southeast Asian” was a good fit for the Indian population and “GLI-Other” fit the Black African and Mixed ancestry populations well (43). These findings highlight the importance of multiple factors, including environment and socioeconomic exposures that impact population differences (42, 43).
Numerous environmental factors were reported to impact on lung function. Children living in rural areas or exposed to poorer socio-economic circumstances had lower lung function compared to those in urban areas (42, 44). Exposures to volatile organic compounds, particulate matter 10 (PM10) or carbon monoxide (CO) were associated with a decrease in FEV1 and FVC in exposed preschool children (45–47).
In a study in Guatemala, where CO was used as a proxy for PM2.5, timing of chimney stove installation was compared to cooking over open fires, and showed a decrease in PEF of 173 ml/min/year (95% CI −341 to −7). with chimney stove installation at 18 months compared to installation at birth (48). A Chinese study failed to demonstrate any significant association between PM2.5 exposure and any of the spirometry lung function measures, however an increase in oscillometry resistance was noted suggesting that oscillometry may be a more sensitive measure (49). In addition to air pollution, e-waste is a growing concern. Chinese children living in e-waste exposed areas had significantly lower birth weight, chest circumference and spirometry lung function compared to those in unexposed areas (27, 28).
The majority of included papers used spirometry in the clinical diagnosis and management of pediatric obstructive lung disease including asthma and CF. The studies investigated genetic predisposition to asthma, and management of acute, poorly controlled and cough-variant asthma (CVA). and the impact of obesity (50–53). Genes associated with increased susceptibility to asthma and lower spirometry indices were identified in Chinese and Egyptian children (54, 55). One study assessed current definitions of BDR, suggesting that a BDR of >7.5% may be more valuable in young children rather than the adult defined 12% (56). Another study included assessing impact of ABPA on spirometry of asthmatic children (57). In a Chinese study acute asthma did not respond to adding montelukast to the regular regimen (58), however it was noted that in children with CVA, cough associated with chronic airway allergic inflammation without wheeze, had a significant improvement in FEV1, FVC, and PEF (p <0.001). with montelukast and budesonide compared to budesonide alone, while another study noted that FEV1/FVC was normal in CVA compared to patients with asthma (p <0.001) (51, 52).
Spirometry was also used in South American, Turkish and South African children to monitor lung function in cystic fibrosis (59), and showed good correlation between CT scan Bhalla score and FEV1, FVC, FEF25−75 (60).
Of the 7 papers using FeNO, 4 (57%) clinical studies measured FeNO to assess risk and treatment response of asthma or recurrent wheeze; and 3 epidemiological studies assessed the impact of IAP on airway inflammation in children. These included studies mainly from Asia (5 from China, 1 from Thailand) and 1 from Ecuador, South America. There were no published preschool studies from Africa. Included studies are summarized in Table 2.
Table 2. Detail of included studies that assessed fractional exhaled nitric oxide (n = 7), oscillometry (n = 15), interrupter technique (n = 2). and tidal breathing (n = 2).
The success rate for performing FeNO in preschool children ranged between 86–99%. Thai children living in a metropolitan area attending day care, average age of 50.1 months (range 29–72 months), achieved a success rate between 86–93% with data collected over 3 time points (61). A cohort of 507 Chinese children aged 5 years achieved a success rate of 99% (62). Over half of studies (57%) used a Niox Mino analyzer (Aerocrine, Solna, Sweden). to measure FeNO using the single breath technique. Most studies used the recommended normal standards (26). No studies have explored population differences in FeNO.
All 3 studies assessing impact of air quality on respiratory health found that environmental pollution, including benzene and PM2.5, were associated with high FeNO (49, 61, 62).
Studies from China and one from South America, used FeNO measurement in the management of preschool asthma (63–65), noting the strong association with IgE mediated inflammation and ICS efficacy (63). However, it was also suggested that FeNO may be less useful in the preschool age group for detecting ICS response, as the mean FeNO level was significantly higher only in the “older” age group than the cut off values reported in other studies for the diagnosis of asthma (63). FeNO was not able to predict recurrence (64).
Oscillometry has been successfully used in LMIC to assess the impact of early life exposures and for clinical management of children with respiratory disease. These include: 6 studies from Asia, 6 from South America, 2 from West Africa and 1 from the Middle East summarized in Table 2. The studies used a range of commercially available equipment including both impulse oscillometry (IOS). and airwave oscillometry (AOS).
Oscillometry has proven to be effective in assessing the impact of early life exposures on preschool lung function (49, 66). An increase in household PM2.5 exposure increased airway reactance in Nigerian children, similarly high personal exposure to PM2.5 was associated with an increase in small airway resistance (R5−20), total airway resistance (R5). and resistance frequency dependence (R5−20), representing small airway disease, in asthmatic Chinese children living outside Shanghai (49, 66).
In a Ghanaian longitudinal cohort, infants exposed to a less diverse nasopharyngeal microbiome had a higher small airway resistance compared to a more diverse microbiome at 4 years of age (67). Further, in a Mexican birth cohort study, prenatal omega 3 fatty acid supplementation in pregnancy did not influence preschool lung function at 36, 48 or 60 months (68).
Oscillometry was easily performed by preschool children with the overall success rate ranging between 74–98% in studies making it a particularly attractive option (69–73). As a clinical tool oscillometry for preschool children is supported by studies that are able to detect differences in prebronchodilator lung function of preschool children with respiratory symptoms compared to those without respiratory symptoms (74).
Reference data for Mexican, Thai, Turkish and Colombian children have been collected (70–72, 75–77), facilitating the use in diagnosis of respiratory disease. It is also a useful tool to assess airway reversibility in asthmatic children and cut off values for bronchodilator response were proposed (69, 73). A Chinese study in children with obstructive sleep apnea hypopnea syndrome (OSAHS). demonstrated an increase in total airway resistance in children with OSAHS compared to children with snoring but without OSAHS (78).
The interrupter technique was used in 2 South American studies, one of which looked at the development of reference values for newborn, infants and preschool children, while the other measured Rint in children with cystic fibrosis and found that Rint correlated well to spirometry FeV1 and FEF25−75, but not accurate in determining bronchodilator response (79, 80).
Tidal breathing was used in 2 studies, one an Indian birth cohort and the other a Chinese retrospective cohort study (81, 82), (Table 2). Tidal breathing measurements were in keeping with an obstructive pattern in patients with bronchiolitis obliterans (82). Indian children who had an acute respiratory tract infection in infancy had increased ratios of tidal expiratory flow (TEF) at 25 or 50% of tidal expiratory volume to peak TEF (TEF50 or TEF25/peak TEF) at 3 years suggesting increased airway resistance (81).
Preschool lung function tests used in LMIC were feasible with high success rates. Success with spirometry increased with age (29), and oscillometry had higher feasibility compared to spirometry. All tests were useful for clinical application and epidemiological studies. Comprehensive preschool testing including spirometry, FeNO, oscillometry and tidal breathing were only reported from China, and only 19 countries of 137 registered LMIC (14%) were represented in the review, a large discrepancy as the majority (80%) of children live in LMIC, where the burden of early life respiratory disease and exposures known to cause illness are high. These include air pollution, maternal smoking, a high infectious load including tuberculosis, and high rates of preterm birth (2, 83–86). Lung functions tests assessing these vulnerable groups are lacking. A number of studies explored the effects of air pollution; and the studies suggest that air pollutants increase airway inflammation and may in part explain the association between household air pollution and recurrent wheeze (83). Given the burden of early life exposures and the need to identify preventative measure, priority should be given to strengthening access to lung function assessment tools.
The majority of clinical studies focussed on asthma diagnosis and management, including defining BDR in young children. The prevalence of asthma in LMIC is high, with a temporal increase in severe asthma (87). Of particular concern is that up to 40% of children in LMIC with severe symptoms are undiagnosed (87, 88). Oscillometry was useful, and more sensitive than spirometry, in measuring airway resistance and cut off values for BDR response was also determined. FeNO has been identified as a useful adjunct in diagnosis and informing treatment in asthmatic patients (89). Access to these tests to improve diagnosis and management is needed for the many children living in LMIC.
Other clinical uses have included management of chronic lung conditions like CF, bronchiolitis obliterans, OSAHS, obesity and systemic sclerosis all of which are associated with respiratory complications. Lung function tests assessing vulnerable groups such as children born preterm, those living with HIV or exposed in utero and those with a history of pulmonary TB and other early life lower respiratory tract infections are lacking in children from LMIC.
The importance of reference range equations were highlighted in these studies. A healthy standard is needed for lung function tests to distinguish between health and disease in different populations. The GLI reference equations attempt to address this, however unique environmental exposures including in-utero exposures influence lung development and this needs to be considered when developing reference equations (90). Research studies assessing impact of exposures require appropriate patient samples and statistical modeling and should include both exposed and unexposed control groups.
There are currently no published data for MBW in preschool children in LMIC settings, however data on infant testing have been published in South Africa (3, 91). There is limited data on tidal breathing and the interrupter technique. Impulse and airway oscillometry use different input signals and this may impact results especially at high impedance, which is associated with disease. Furthermore, FeNO single breath and tidal breathing measures have different interpretation of normal ranges, and the results are not interchangeable. These factors may affect the interpretation and comparison of data.
Challenges to accessing lung function testing need to be addressed, these include lack of trained personnel as each lung function test requires specialized training skills and lack of financial resources to support development and implementation of these tests. Spirometry and oscillometry are relatively inexpensive, whereas FeNO and MBW are currently more expensive further limiting access. Equipment maintenance and poor access locally to consumables and technical support incur further costs. This reduces research outputs and lack of robust data to better diagnose, prevent and manage respiratory disease in these settings (5).
In conclusion, preschool testing in LMIC is feasible, both epidemiologically and clinically. It has the potential to be useful in strengthening the diagnosis, management and prevention of respiratory diseases in younger children but is underutilized. Spirometry still remains a key clinical and epidemiological tool in LMIC, however has limitations especially in young children. Understanding and addressing the challenges for improving access to these tools is needed in order to strengthen the prevention, early diagnosis and management of childhood respiratory disease in LMIC.
SC, DG, and HZ conceived the idea. SC and DG reviewed the literature and drafted the manuscript. HZ provided further input into the manuscript. All authors reviewed, contributed, and approved the final manuscript.
DG was funded by the Wellcome Trust (204755/z/16/z). HZ was funded by the SA-Medical Research Council.
The authors declare that the research was conducted in the absence of any commercial or financial relationships that could be construed as a potential conflict of interest.
All claims expressed in this article are solely those of the authors and do not necessarily represent those of their affiliated organizations, or those of the publisher, the editors and the reviewers. Any product that may be evaluated in this article, or claim that may be made by its manufacturer, is not guaranteed or endorsed by the publisher.
The Supplementary Material for this article can be found online at: https://www.frontiersin.org/articles/10.3389/fped.2022.908607/full#supplementary-material
1. Liu L, Villavicencio F, Yeung D, Perin J, Lopez G, Strong KL, et al. National, regional, and global causes of mortality in 5–19-year-olds from 2000 to 2019: a systematic analysis. Lancet Glob Health. (2022) 10:e337–e47. doi: 10.1016/S2214-109X(21)00566-0
2. Zar HJ, Ferkol TW. The global burden of respiratory disease—impact on child health. Pediatr Pulmonol. (2014) 49:430–4. doi: 10.1002/ppul.23030
3. Gray DM, Turkovic L, Willemse L, Visagie A, Vanker A, Stein DJ, et al. Lung function in african infants in the drakenstein child health study. impact of lower respiratory tract illness. Am J Respir Crit Care Med. (2017) 195:212–20. doi: 10.1164/rccm.201601-0188OC
4. Chan JY, Stern DA, Guerra S, Wright AL, Morgan WJ, Martinez FD. Pneumonia in childhood and impaired lung function in adults: a longitudinal study. Pediatrics. (2015) 135:607–16. doi: 10.1542/peds.2014-3060
5. Masekela R, Zurba L, Gray D. Dealing with access to spirometry in Africa: a commentary on challenges and solutions. Int J Environ Res Public Health. (2019) 16:62. doi: 10.3390/ijerph16010062
6. Belgrave DCM, Granell R, Turner SW, Curtin JA, Buchan IE, Le Souef PN, et al. Lung function trajectories from pre-school age to adulthood and their associations with early life factors: a retrospective analysis of three population-based birth cohort studies. Lancet Respir Med. (2018) 6:526–34. doi: 10.1016/S2213-2600(18)30099-7
7. Beydon N, Davis SD, Lombardi E, Allen JL, Arets HG, Aurora P, et al. An official american thoracic society/european respiratory society statement: pulmonary function testing in preschool children. Am J Respir Crit Care Med. (2007) 175:1304–45. doi: 10.1164/rccm.200605-642ST
8. Robinson PD, Latzin P, Ramsey KA, Stanojevic S, Aurora P, Davis SD, et al. Preschool multiple-breath washout testing. an official american thoracic society technical statement. Am J Respir Crit Care Med. (2018) 197:e1–e19. doi: 10.1164/rccm.201801-0074ST
9. Nystad W, Samuelsen S, Nafstad P, Edvardsen E, Stensrud T, Jaakkola J. Feasibility of measuring lung function in preschool children. Thorax. (2002) 57:1021–7. doi: 10.1136/thorax.57.12.1021
10. Graham BL, Steenbruggen I, Miller MR, Barjaktarevic IZ, Cooper BG, Hall GL, et al. Standardization of Spirometry 2019 Update. an official american thoracic society and european respiratory society technical statement. Am J Respir Crit Care Med. (2019) 200:e70–88. doi: 10.1164/rccm.201908-1590ST
11. Knihtila H, Kotaniemi-Syrjanen A, Makela MJ, Bondestam J, Pelkonen AS, Malmberg LP. Preschool oscillometry and lung function at adolescence in asthmatic children. Pediatr Pulmonol. (2015) 50:1205–13. doi: 10.1002/ppul.23188
12. Quanjer PH, Stanojevic S, Cole TJ, Baur X, Hall GL, Culver BH, et al. Multi-ethnic reference values for spirometry for the 3-95-yr age range: the global lung function 2012 equations. Eur Respir J. (2012) 40:1324–43. doi: 10.1183/09031936.00080312
13. Bates JH, Irvin CG, Farré R, Hantos Z. Oscillation mechanics of the respiratory system. Compr Physiol. (2011) 1:1233–72. doi: 10.1002/cphy.c100058
14. Gray DM, Czovek D, McMillan L, Turkovic L, Stadler JAM, Vanker A, et al. Intra-breath measures of respiratory mechanics in healthy African infants detect risk of respiratory illness in early life. Eur Respir J. (2019) 53:2. doi: 10.1183/13993003.00998-2018
15. Hantos Z. Intra-breath oscillometry for assessing respiratory outcomes. Curr Opin Physiol. (2021). 10:1445. doi: 10.1016/j.cophys.2021.05.004
16. Oostveen E, MacLeod D, Lorino H, Farre R, Hantos Z, Desager K, et al. The forced oscillation technique in clinical practice: methodology, recommendations and future developments. Eur Respir J. (2003) 22:1026–41. doi: 10.1183/09031936.03.00089403
17. King GG, Bates J, Berger KI, Calverley P, de Melo PL, Dellacà RL, et al. Technical standards for respiratory oscillometry. Eur Respir J. (2020) 55:1–20. doi: 10.1183/13993003.00753-2019
18. Fenu G, Calogero C, Lombardi E. Lung function tests in preschool children. Turkish thoracic J. (2015) 16:185–8. doi: 10.5152/ttd.2015.5038
19. Beydon N. Interrupter resistance: what's feasible? Paediatr Respir Rev. (2006) 7:S5–7. doi: 10.1016/j.prrv.2006.04.022
20. Kinghorn B, McNamara S, Genatossio A, Sullivan E, Siegel M, Bauer I, et al. Comparison of multiple breath washout and spirometry in children with primary ciliary dyskinesia and cystic fibrosis and healthy controls. Ann Am Thorac Soc. (2020) 17:1085–93. doi: 10.1513/AnnalsATS.201905-375OC
21. Rosenfeld M, Allen J, Arets BH, Aurora P, Beydon N, Calogero C, et al. An official American thoracic society workshop report: optimal lung function tests for monitoring cystic fibrosis, bronchopulmonary dysplasia, and recurrent wheezing in children less than 6 years of age. Ann Am Thorac Soc. (2013) 10:S1–S11. doi: 10.1513/AnnalsATS.201301-017ST
22. Li Y, Wu R, Tian Y, Bao T, Tian Z. Fraction of exhaled nitric oxide and soluble receptors for advanced glycation end products are negatively correlated in children with recurrent wheezing. Asian Pac J Allergy Immunol. (2017) 35:33–7. doi: 10.12932/AP0746
23. Horváth I, Barnes PJ, Loukides S, Sterk PJ, Högman M, Olin A-C, et al. A European Respiratory Society technical standard: exhaled biomarkers in lung disease. Eur Respir J. (2017) 49:4. doi: 10.1183/13993003.00965-2016
24. Dweik RA, Boggs PB, Erzurum SC, Irvin CG, Leigh MW, Lundberg JO, et al. An official ATS clinical practice guideline: interpretation of exhaled nitric oxide levels (FENO) for clinical applications. Am J Respir Crit Care Med. (2011) 184:602–15. doi: 10.1164/rccm.9120-11ST
25. Ferrante G, Malizia V, Antona R, Corsello G, La Grutta S. The value of FeNO measurement in childhood asthma: uncertainties and perspectives. Multidiscip Respir Med. (2013) 8:1–8. doi: 10.1186/2049-6958-8-50
26. Exhaled N. ATS/ERS recommendations for standardized procedures for the online and offline measurement of exhaled lower respiratory nitric oxide and nasal nitric oxide, 2005. Am J Respir Crit Care Med. (2005) 171:912–30. doi: 10.1164/rccm.200406-710ST
27. Zeng X, Xu X, Boezen HM, Vonk JM, Wu W, Huo X. Decreased lung function with mediation of blood parameters linked to e-waste lead and cadmium exposure in preschool children. Environ Pollut. (2017) 230:838–48. doi: 10.1016/j.envpol.2017.07.014
28. Zeng X, Xu X, Zhang Y, Li W, Huo X. Chest circumference and birth weight are good predictors of lung function in preschool children from an e-waste recycling area. Environ sci Pollut Res. (2017) 24:22613–21. doi: 10.1007/s11356-017-9885-5
29. Al-Qerem WA, Jarab AS. Applicability of GLI 2012 spirometry equation among preschool aged Jordanian. Respir Med. (2021) 182:106397. doi: 10.1016/j.rmed.2021.106397
30. França DC, Camargos PA, Martins JA, Abreu MC. Avelar e Araújo GH, Parreira VF. Feasibility and reproducibility of spirometry and inductance plethysmography in healthy Brazilian preschoolers. Pediatr Pulmonol. (2013) 48:716–24. doi: 10.1002/ppul.22743
31. Veras TN, Pinto LA. Feasibility of spirometry in preschool children. J Bras Pneumol. (2011) 37:69–74. doi: 10.1590/S1806-37132011000100011
32. Franca DC, Moreira Camargos PA, Jones MH, Martins JA., Pinto Pinheiro Vieira BdS, Colosimo EA, et al. Prediction equations for spirometry in four- to six-year-old children. Jornal De Pediatria. (2016) 92:400–8. doi: 10.1016/j.jped.2015.10.005
33. Burity EF, Pereira CAC, Rizzo JA, Brito MCA, Sarinho ESC. Reference values for spirometry in preschool children. J Pediatr. (2013) 89:374–80. doi: 10.1016/j.jped.2013.01.002
34. Jones MH, Vidal PCV, Lanza FC, Silva D, Pitrez PM, Olmedo A, et al. Reference values for spirometry in Brazilian children. J Bras Pneumol. (2020) 46:e20190138. doi: 10.36416/1806-3756/e20190138
35. Jian W, Gao Y, Hao C, Wang N, Ai T, Liu C, et al. Reference values for spirometry in Chinese aged 4–80 years. J Thorac Dis. (2017) 9:4538. doi: 10.21037/jtd.2017.10.110
36. Tabatabaie SS, Boskabady MH, Mohammadi SS, Mohammadi O, Saremi P, Amery S, et al. Prediction equations for pulmonary function values in healthy children in Mashhad city, North East Iran. J Res Med Sci. (2014) 19:128–33. doi: 10.1111/j.1440-1843.2004.00623.x
37. Bolla KC, Shanmuganathan A, Narasimhan M, Ganga N, Fathima Zehra Razvin H. Prediction equations for spirometry parameters of healthy school-going children in South India. Ann Trop Med Public Health. (2020) 23:15. doi: 10.36295/ASRO.2020.231514
38. Bougrida M, Bourahli MK, Aissaoui A, Rouatbi S, Mehdioui H, Saad HB. Spirometric reference values for children living in Constantine (Eastern region of Algeria). Tunisie Medicale. (2012) 90:51–61.
39. Asif M, Mustafa G, Aslam M, Altaf S. Predictors of pulmonary function test values for Pakistani children, aged 5-14 years. J Pak Med Assoc. (2017) 67:1323–6.
40. Akodui OS, Faleti AO, Adekanmbi FA, Ogunlesi TA. Predicted reference equations for spirometry indices as a proxy from anthropometry measurements of Nigerian children with sickle cell Anaemia Aged 5-12 Years. J Trop Pediatr. (2021) 67:1. doi: 10.1093/tropej/fmaa095
41. Quanjer PH, Capderou A, Mazicioglu MM, Aggarwal AN, Banik SD, Popovic S, et al. All-age relationship between arm span and height in different ethnic groups. Eur Respir J. (2014) 44:905–12. doi: 10.1183/09031936.00054014
42. Lum S, Bountziouka V, Quanjer P, Sonnappa S, Wade A, Beardsmore C, et al. Challenges in collating spirometry reference data for South-Asian children: an observational study. PLoS ONE. (2016) 11:e0154336. doi: 10.1371/journal.pone.0154336
43. Smith S-J, Gray DM, MacGinty RP, Hall GL, Stanojevic S, Mphahlele R, et al. Choosing the better global lung initiative 2012 equation in south african population groups. Am J Respir Crit Care Med. (2020) 202:1724–7. doi: 10.1164/rccm.202005-2085LE
44. Sonnappa S, Lum S, Kirkby J, Bonner R, Wade A, Subramanya V, et al. Disparities in pulmonary function in healthy children across the Indian urban-rural continuum. Am J Respir Crit Care Med. (2015) 191:79–86. doi: 10.1164/rccm.201406-1049OC
45. Asrul S, Juliana J. Indoor air quality and its association with respiratory health among preschool children in urban and suburban area. Malays J Public Health Med. (2017) 2017:78– 88.
46. Choo CP, Jalaludin J, Hamedon TR, Adam NM. Indoor air quality assessment and lung functions among children in preschool at Selangor, Malaysia. Adv Environ Biol. (2015) 9:1–9.
47. Rawi NAMN, Jalaludin J, Chua PC. Indoor air quality and respiratory health among malay preschool children in Selangor. Biomed Res Int. (2015) 2015: 248178. doi: 10.1155/2015/248178
48. Heinzerling AP, Guarnieri MJ, Mann JK, Diaz JV, Thompson LM, Diaz A, et al. Lung function in woodsmoke-exposed Guatemalan children following a chimney stove intervention. Thorax. (2016) 71:421–8. doi: 10.1136/thoraxjnl-2015-207783
49. He L, Norris C, Cui X, Li Z, Barkjohn KK, Brehmer C., et al. Personal Exposure to PM(25) Oxidative Potential in Association with Pulmonary Pathophysiologic Outcomes in Children with Asthma. Environ Sci Technol. (2021) 55:3101–11. doi: 10.1021/acs.est.0c06114
50. Kumar SPS, Jain S. Effect of Obesity on Asthma Severity in Urban School Children of Kanpur, India: An Analytical Cross-Sectional Study. J Community Health. (2020) 45:342–7. doi: 10.1007/s10900-019-00749-z
51. Ghasempour M, Bilan N, Vafaei I. The Role of Spirometry in the diagnosis of cough variant asthma in children aged 5 to 15 years. Int J Pediatr. (2017) 5:6329–36. doi: 10.22038/ijp.2017.26518.2276
52. Sun W, Liu HY. Montelukast and budesonide for childhood cough variant asthma. J Coll Physicians Surg Pak. (2019) 29:345–8. doi: 10.29271/jcpsp.2019.04.345
53. Matos SMA, Jesus SR, Saldiva SRDM, Prado MS, D'Innocenzo S, Assis AMO, et al. Overweight, asthma symptoms, atopy and pulmonary function in children of 4-12 years of age: Findings from the SCAALA cohort in Salvador, Bahia, Brazil. Public Health Nutr. (2011) 14:1270–8. doi: 10.1017/S1368980011000462
54. Jiffri EH, Elhawary NA. The impact of common tumor necrosis factor haplotypes on the development of asthma in children: an egyptian model. Genet Test Mol Biomarkers. (2011) 15:293–9. doi: 10.1089/gtmb.2010.0157
55. Leung TF, Tang MF, Leung ASY, Kong APS, Liu TC, Chan RWY, et al. Cadherin-related family member 3 gene impacts childhood asthma in Chinese children. Pediatr Allergy Immunol. (2020) 31:133–42. doi: 10.1111/pai.13138
56. Kang XH, Wang W, Cao L. A clinical study to determine the threshold of bronchodilator response for diagnosing asthma in Chinese children. World J Clin Pediatr. (2019) 15:559–64. doi: 10.1007/s12519-019-00293-9
57. Kumari J, Jat KR, Lodha R, Jana M, Xess I, Kabra SK. Prevalence and Risk Factors of Allergic Bronchopulmonary Aspergillosis and Aspergillus Sensitization in Children with Poorly Controlled Asthma. J Trop Pediatr. (2020) 66:275–83. doi: 10.1093/tropej/fmz066
58. Wang X, Zhou J, Zhao X, Yi X. Montelukast Treatment of Acute Asthma Exacerbations in Children Aged 2 to 5 Years: A Randomized, Double-Blind, Placebo-Controlled Trial. Pediatr Emerg Care. (2018) 34:160–4. doi: 10.1097/PEC.0000000000001184
59. Ventura JC, Hauschild DB, Moreira EAM, Pereira LCR, Rosa AF, Barbosa E, et al. C-reactive protein/albumin ratio is associated with lung function among children/adolescents with cystic fibrosis: a three-year longitudinal study. São Paulo Med J. (2018) 136:29–36. doi: 10.1590/1516-3180.2017.0109100917
60. Sasihuseyinoglu AS, Altintaş DU, Soyupak S, Dogruel D, Yilmaz M, Serbes M, et al. Evaluation of high resolution computed tomography findings of cystic fibrosis. Korean J Intern Med. (2019) 34:335–43. doi: 10.3904/kjim.2017.287
61. Siwarom S, Puranitee P, Plitponkarnpim A, Manuyakorn W, Sinitkul R, Vallipakorn SAO. Association of indoor air quality and preschool children's respiratory symptoms. Asian Pac J Allergy Immunol. (2017) 35:119–26.
62. Zhang X, Fan Q, Bai X, Li T, Zhao Z, Fan X, et al. Levels of fractional exhaled nitric oxide in children in relation to air pollution in Chinese day care centres. Int J Tuberc Lung Dis. (2018) 22:813–9. doi: 10.5588/ijtld.17.0601
63. Zhu Z, Xia S, Chen X, Guan WJ, Guo ZJ, Sun BQ. Factors associated with exhaled nitric oxide in children with asthma and allergic rhinitis. Clin Respir J. (2020) 14:9–15. doi: 10.1111/crj.13093
64. Ardura-Garcia C, Arias E, Hurtado P, Bonnett LJ, Sandoval C, Maldonado A, et al. Predictors of severe asthma attack re-attendance in Ecuadorian children: A cohort study. Eur Respir J. (2019) 54:1–56. doi: 10.1183/13993003.02419-2018
65. Han L, Shangguan J, Yu G, Li T, Wu Y, Zhou Y, et al. Association between family management and asthma control in children with asthma. J Spec Pediatr Nurs. (2020) 25:2. doi: 10.1111/jspn.12285
66. Dutta A, Alaka M, Ibigbami T, Adepoju D, Adekunle S, Olamijulo J, et al. Impact of prenatal and postnatal household air pollution exposure on lung function of 2-year old Nigerian children by oscillometry. Sci Total Environ. (2021) 755:143419. doi: 10.1016/j.scitotenv.2020.143419
67. Dubowski K, Kaali S, Jack D, Prah RKD, Clemente JC, Tawiah T, et al. Infant Nasopharyngeal Microbiota Subphenotypes and Early Childhood Lung Function: Evidence from a Rural Ghanaian Pregnancy Cohort. Int J Environ Res Public Health. (2021) 18:14. doi: 10.3390/ijerph18147276
68. Gutierrez-Delgado RI, Barraza-Villarreal A, Escamilla-Nunez C, Hernandez-Cadena L, Garcia-Feregrino R, Shackleton C, et al. Effect of omega-3 fatty acids supplementation during pregnancy on lung function in preschoolers: a clinical trial. J Asthma. (2019) 56:296–302. doi: 10.1080/02770903.2018.1452934
69. Gupta N, Sachdev A, Gupta D, Gupta S. Assessment of airway reversibility in asthmatic children using forced oscillation technique-A single-center experience from North India. Lung India. (2021) 38:229–35. doi: 10.4103/lungindia.lungindia_354_20
70. Udomittipong K, Srisukhon W, Nimmannit A, Komoltri C. Respiratory impedance reference values for forced oscillation technique predicted by arm span and height in Thai preschool children. Pediatr Allergy Immunol Pulmonol. (2017) 30:97–102. doi: 10.1089/ped.2017.0747
71. Gochicoa-Rangel L, Torre-Bouscoulet L, Martínez-Briseño D, Rodríguez-Moreno L, Cantú-González G, Vargas MH. Values of impulse oscillometry in healthy Mexican children and adolescents. Respir Care. (2015) 60:119–27. doi: 10.4187/respcare.03374
72. Shackleton C, Barraza-Villarreal A, Chen L, Gangell CL, Romieu I, Sly PD. Reference ranges for Mexican preschool-aged children using the forced oscillation technique. Archivos de Bronconeumología (English Edition). (2013) 49:326–9. doi: 10.1016/j.arbr.2013.06.002
73. Udomittipong K, Triwatanawong J, Nimmannit A, Komoltri C. Cut-off values for positive bronchodilator response in healthy thai preschool children using forced oscillation technique. Asian Pac J Allergy Immunol. (2020) 38:258–63. doi: 10.12932/AP-280718-0381
74. Medeiros D, Castro P, Dela Bianca AC, Sarinho E, Araujo JF, Correia M. Jr., et al. Impulse oscillometry: pulmonary function assessment in preschool children. Expert Rev Respir Med. (2020) 14:1261–6. doi: 10.1080/17476348.2020.1813573
75. Gochicoa-Rangel L., del Río-Hidalgo R, Hernández-Ruiz J, Rodríguez-Moreno L, Martínez-Briseño D, Mora-Romero U, et al. Validating reference equations for impulse oscillometry in healthy. Mexican Child Res Care. (2017) 62:1156–65. doi: 10.4187/respcare.05247
76. Er I, Günlemez A, Baydemir C, Kiliçbay F, Ersu R, Uyan ZS. Impulse oscillometry reference values and correlation with predictors in Turkish preschool children. Turkish J Pediatr. (2019) 61:560–7. doi: 10.24953/turkjped.2019.04.013
77. Duenas-Meza E, Correa E, López E, Morales JC, Aguirre-Franco CE, Morantes-Ariza CF, et al. Impulse oscillometry reference values and bronchodilator response in three- to five-year old children living at high altitude. J Asthma Allergy. (2019) 12:263–71. doi: 10.2147/JAA.S214297
78. Zhang J, Zhao J, Chen M, Liu S, Zhang X, Zhang F, et al. Airway resistance and allergic sensitization in children with obstructive sleep apnea hypopnea syndrome. Pediatr Pulmonol. (2016) 51:426–30. doi: 10.1002/ppul.23264
79. Rocha A, Donadio MVF, de Ávila DV, Hommerding PX, Marostica PJC. Using the interrupter technique to evaluate airway resistance in cystic fibrosis patients. J Bras Pneumol. (2012) 38:188–93. doi: 10.1590/s1806-37132012000200007
80. Gochicoa LG, Thomé-Ortiz LP, Furuya MEY, Canto R, Ruiz-García ME, Zúñiga-Vázquez G, et al. Reference values for airway resistance in newborns, infants and preschoolers from a Latin American population. Respirology. (2012) 17:667–73. doi: 10.1111/j.1440-1843.2012.02156.x
81. Kumar P, Mukherjee A, Randev S, Medigeshi GR, Jat KR, Kapil A, et al. Effect of acute respiratory infections in infancy on pulmonary function test at 3 years of age: A prospective birth cohort study. BMJ Open Res Res. (2020) 7:10. doi: 10.1136/bmjresp-2019-000436
82. Li YN, Liu L, Qiao HM, Cheng H, Cheng HJ. Post-infectious bronchiolitis obliterans in children: A review of 42 cases. BMC Pediatr. (2014) 14:19. doi: 10.1186/1471-2431-14-238
83. Vanker A, Barnett W, Workman L, Nduru PM, Sly PD, Gie RP, et al. Early-life exposure to indoor air pollution or tobacco smoke and lower respiratory tract illness and wheezing in African infants: a longitudinal birth cohort study. Lancet Planetary Heal. (2017) 1:e328–e36. doi: 10.1016/S2542-5196(17)30134-1
84. Masekela R, Vanker A. Lung Health in Children in Sub-Saharan Africa: Addressing the Need for Cleaner Air. Int J Environ Res Public Health. (2020) 17:6178. doi: 10.3390/ijerph17176178
85. Marangu D, Zar HJ. Childhood pneumonia in low-and-middle-income countries: An update. Paediatr Respir Rev. (2019) 32:3–9. doi: 10.1016/j.prrv.2019.06.001
86. Pusdekar YV, Patel AB, Kurhe KG, Bhargav SR, Thorsten V, Garces A, et al. Rates and risk factors for preterm birth and low birthweight in the global network sites in six low-and low middle-income countries. Reprod Health. (2020) 17:1–16. doi: 10.1186/s12978-020-01029-z
87. Lai CK, Beasley R, Crane J, Foliaki S, Shah J, Weiland S, et al. Global variation in the prevalence and severity of asthma symptoms: phase three of the International Study of Asthma and Allergies in Childhood (ISAAC). Thorax. (2009) 64:476–83. doi: 10.1136/thx.2008.106609
88. Baard CB, Franckling-Smith Z, Munro J, Workman L, Zar HJ. Asthma in South African adolescents: a time trend and risk factor analysis over two decades. ERJ Open Res. (2021) 7:1–92. doi: 10.1183/23120541.00576-2020
89. Gaillard EA, Kuehni CE, Turner S, Goutaki M, Holden KA, de Jong CCM, et al. European Respiratory Society clinical practice guidelines for the diagnosis of asthma in children aged 5-16 years. Eur Respir J. (2021) 58:5. doi: 10.1183/13993003.04173-2020
90. Schluger NW. The Vanishing Rationale for the Race Adjustment in Pulmonary Function Test Interpretation. Am J Respir Crit Care Med. (2022) 10:900. doi: 10.1164/rccm.202112-2772ED
91. Gray D, Willemse L, Visagie A, Czovek D, Nduru P, Vanker A, et al. Determinants of early-life lung function in African infants. Thorax. (2017) 72:445–50. doi: 10.1136/thoraxjnl-2015-207401
92. Gulla KM, Balaji A, Mukherjee A, Jat KR, Sankar J, Lodha R, et al. Course of illness after viral infection in Indian children with cystic fibrosis. J Trop Pediatr. (2019) 65:176–82. doi: 10.1093/tropej/fmy033
93. Kamaruddin AS, Jalaludin J, Choo CP. Indoor air quality and its association with respiratory health among malay preschool children in Shah Alam and Hulu Langat, Selangor. Adv Environ Biol. (2015) 9:17–26.
94. Thacher JD, Emmelin A, Madaki AJ, Thacher TD. Biomass fuel use and the risk of asthma in Nigerian children. Respir Med. (2013) 107:1845–51. doi: 10.1016/j.rmed.2013.09.009
95. Corten L, Morrow BM. Motor Performance in South African children with cystic fibrosis. Phys Occup Ther Pediatr. (2020) 40:192–200. doi: 10.1080/01942638.2019.1647329
Keywords: spirometry, fractional exhaled nitric oxide, oscillometry, interrupter technique, tidal breathing, multiple breath washout
Citation: Chaya S, Zar HJ and Gray DM (2022) Lung Function in Preschool Children in Low and Middle Income Countries: An Under-Represented Potential Tool to Strengthen Child Health. Front. Pediatr. 10:908607. doi: 10.3389/fped.2022.908607
Received: 30 March 2022; Accepted: 10 May 2022;
Published: 06 June 2022.
Edited by:
Renato Cutrera, Bambino Gesù Children's Hospital (IRCCS), ItalyReviewed by:
Mario Barreto, Sapienza University of Rome, ItalyCopyright © 2022 Chaya, Zar and Gray. This is an open-access article distributed under the terms of the Creative Commons Attribution License (CC BY). The use, distribution or reproduction in other forums is permitted, provided the original author(s) and the copyright owner(s) are credited and that the original publication in this journal is cited, in accordance with accepted academic practice. No use, distribution or reproduction is permitted which does not comply with these terms.
*Correspondence: Shaakira Chaya, c2hhYWtpcmEuY2hheWFAZ21haWwuY29t
Disclaimer: All claims expressed in this article are solely those of the authors and do not necessarily represent those of their affiliated organizations, or those of the publisher, the editors and the reviewers. Any product that may be evaluated in this article or claim that may be made by its manufacturer is not guaranteed or endorsed by the publisher.
Research integrity at Frontiers
Learn more about the work of our research integrity team to safeguard the quality of each article we publish.