- 1Department of Pediatrics, University of Alberta, Edmonton, AB, Canada
- 2Department of Pediatrics, University of British Columbia, Vancouver, BC, Canada
- 3Division of Infectious Diseases, Department of Pediatrics, Université de Montreal, Montreal, QC, Canada
- 4Department of Pharmacology and Physiology, Université de Montréal, Montreal, QC, Canada
- 5Division of Neonatology, Department of Pediatrics, University of Toronto, Toronto, ON, Canada
- 6Department of Pharmacy, Sunnybrook Health Sciences Centre, Toronto, ON, Canada
- 7Department of Microbiology, Infectious Diseases and Immunology, Université de Montreal, Montreal, QC, Canada
- 8Division of Neonatology, Department of Pediatrics, McMaster University, Hamilton, ON, Canada
- 9Division of Neonatology, Department of Pediatrics, University of Manitoba, Winnipeg, MB, Canada
- 10Department of Microbiology, McMaster University, Hamilton, ON, Canada
- 11Department of Pediatrics, University of Massachusetts, Worcester, MA, United States
- 12School of Medicine, Queen's University, Kingston, ON, Canada
- 13Northern Ontario School of Medicine, Thunder Bay, ON, Canada
- 14Division of Infectious Diseases, Department of Pediatrics, CHU de Québec-Université Laval, Québec, QC, Canada
- 15Division of Pediatric Surgery, Department of Surgery, University of British Columbia, Vancouver, BC, Canada
- 16Division of Infectious Diseases, Department of Pediatrics, University of Alberta, Edmonton, AB, Canada
Neonates are highly susceptible to infections owing to their immature cellular and humoral immune functions, as well the need for invasive devices. There is a wide practice variation in the choice and duration of antimicrobial treatment, even for relatively common conditions in the NICU, attributed to the lack of evidence-based guidelines. Early decisive treatment with broad-spectrum antimicrobials is the preferred clinical choice for treating sick infants with possible bacterial infection. Prolonged antimicrobial exposure among infants without clear indications has been associated with adverse neonatal outcomes and increased drug resistance. Herein, we review and summarize the best practices from the existing literature regarding antimicrobial use in commonly encountered conditions in neonates.
Introduction
Neonates admitted to the neonatal intensive care unit (NICU) are highly susceptible to overwhelming infections, which can progress rapidly with potentially disastrous consequences. Neonates, especially those born preterm, are highly susceptible to over-whelming generalized infections because of their immature immune system, and the need for invasive devices such as central catheters.
Antimicrobials are commonly prescribed in the NICU (1, 2). However, there is a wide practice variation in the choice and duration of antimicrobial treatment, even for relatively common conditions, due to a lack of evidence-based guidelines (3–8). Here we conducted a narrative review of the best practices regarding antimicrobial use from the existing literature in six commonly encountered conditions in neonatology, namely early-onset sepsis (EOS), late-onset sepsis (LOS), ventilator-associated pneumonia (VAP), NEC, urinary tract infection (UTI), and surgical site infection (SSI) (Table 1).
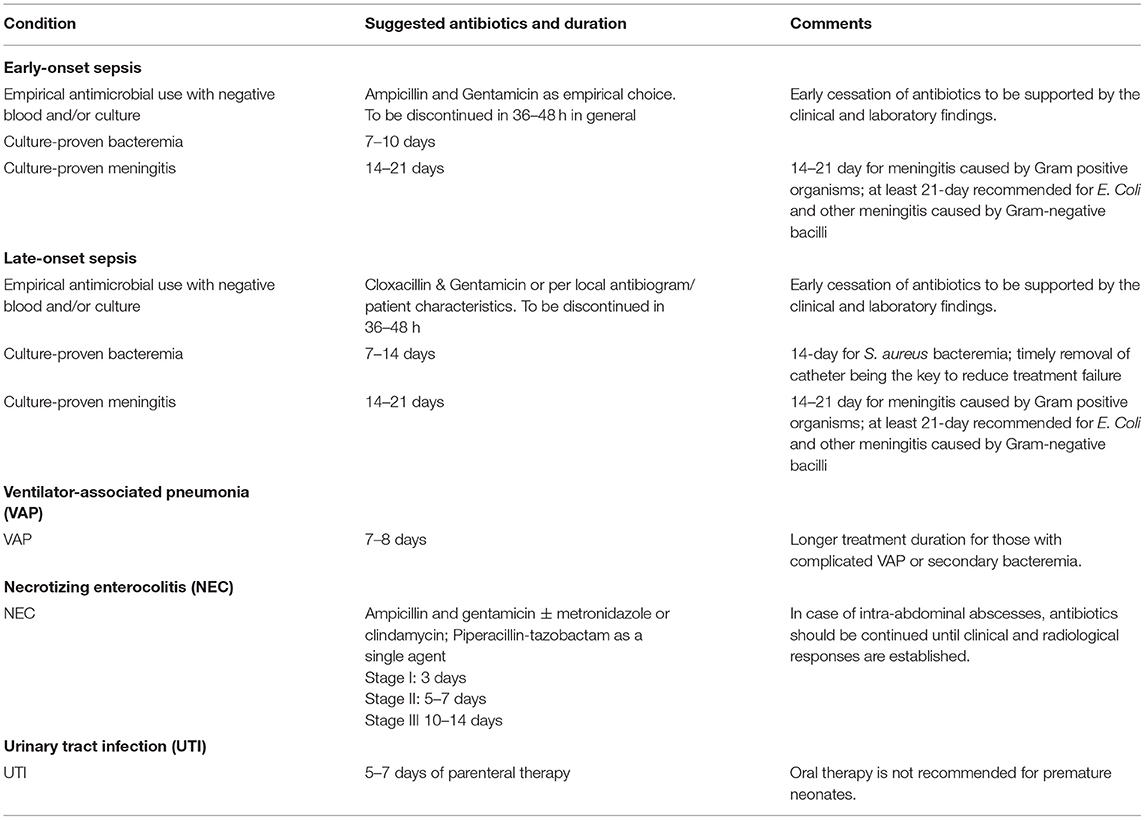
Table 1. Summary of recommendations for agent and duration of antimicrobial therapy for commonly encountered conditions in the NICU.
Importance of Antimicrobial Stewardship in the Neonatal Populations
Antimicrobials are commonly prescribed for treatment or prophylaxis purposes in the NICU, since the clinical signs and symptoms of infection are often non-specific and difficult to differentiate from common non-infectious pathologic processes (1). Furthermore, early decisive treatment with broad-spectrum antimicrobials is the preferred clinical choice for treating sick infants with possible bacterial infection. Since it is often impossible or even ethical to conduct blinded randomized controlled trials on the choice or duration of antibiotics for critically ill infants, recommendations are often derived from combining center-specific data on the most common infecting organisms to make the most rationale choices for empiric therapy. Therapy duration has been shown to not correlate with clinical findings or risk index (9–12). Variability in antimicrobial prescribing across sites has been reported even after adjustment for patient characteristics correlated with illness severity (13).
Exposure to broad-spectrum antibiotics can lead to gut dysbiosis, adverse neonatal outcomes, (14–19), and emergence of multi-drug resistant organisms (MDRO) such as bacteria producing extended-spectrum β-lactamases (ESBL) (20, 21), vanco-mycin–resistant Enterococcus, (22) carbapenem-resistant Enterobacteriaceae, (23, 24) as well as invasive Candidal infection (17, 25). Prolonged antimicrobial exposure without culture-proven sepsis or necrotizing enterocolitis (NEC) has been associated with increased mortality, morbidities, and possibly worse early neurodevelopmental outcomes in preterm infants (18, 26–28).
Antimicrobial stewardship programs (ASP) optimize clinical outcomes while mitigating unintended consequences of antimicrobial misuse for control of emergence of MDRO (29). ASP includes active monitoring for antimicrobial resistance, fostering of appropriate antimicrobial use, and collaboration with an effective infection control program and pharmacy department to minimize secondary spread of resistance (30). There is currently lack of NICU-specific antimicrobial stewardship best practices and strategies that address the unique challenges faced in the management of NICU patients (31, 32). Moreover, there are no established sets of evidence-based NICU-specific metrics to measure the success or failure of ASP initiatives (31).
Review of the current best evidences in commonly encountered neonatal conditions sets the stage for the establishment of a proper NICU-specific ASP to promote the judicious use of antimicrobials.
Early Onset Sepsis
EOS is a significant contributor to neonatal mortality and other adverse outcomes (33). Antibiotics are administered to approximately 2–15% of term newborns and 75–95% of preterm newborns owing to the risk of EOS (34). In the Canadian Neonatal Network, EOS refers to culture-proven sepsis or meningitis within the first two calendar days of life (35). The Center for Disease Control and Prevention (CDC) defines EOS as blood and/or cerebrospinal fluid (CSF) culture-proven infection in newborns occurring at less than 7 days of life (36). Preterm EOS is defined as the isolation of a pathogenic bacterial species from a blood or cerebrospinal fluid culture obtained within 72 h after birth (37, 38).
Epidemiology and Microbiology
EOS mainly develops from the transmission of bacteria that colonize the maternal genito-urinary and gastrointestinal system during the intrapartum period (39). Group B streptococcus (GBS) and Escherichia coli account for 70–80% of blood and cerebrospinal fluid cultures (40–42). Intra-partum antimicrobial prophylaxis (IAP) became the standard of clinical care to prevent GBS transmission in the 1990s (43). With its wide implementation, the incidence of EOS due to GBS in the United States has decreased from 1.5–1.7 cases per 1000 live births to 0.23 per 1,000 live births (44). Recent population surveillance studies in North America revealed that E. coli disease primarily occurs among preterm infants; GBS disease primarily occurs among term infants, with almost half occurring in infants born to mothers with negative GBS screening test results (45).
Predictors of Development of EOS
Gestational age (GA) is the strongest predictor of EOS (39). The incidence of EOS is inversely associated with GA, with 0.5 cases per 1000 neonates born at ≥34 weeks' gestation and up to 32 cases per 1,000 in those born at 22–24 weeks of gestation (39, 46). The EOS rate was reported to be 4.3–5.2% among infants born at a GA of <25 weeks in Canadian cohorts (47, 48). The risk factors associated with EOS in term infants include intrapartum GBS colonization or GBS bacteriuria during the current pregnancy, previous infant with invasive GBS disease, prolonged rupture of membranes ≥18 h, and maternal temperature ≥38°C (49, 50). In preterm infants (≤35 weeks' gestation), unexplained spontaneous preterm labor, premature rupture of membranes (PROM), acute onset of atypical fetal heart rate, or concern for intra-uterine infection (or chorioamnionitis) are risk factors for EOS (39).
Diagnostic Tools
Infants often have nonspecific clinical manifestations that can be indistinguishable from normal newborn transitions or prematurity conditions (38). Positive blood or cerebrospinal fluid culture is the hallmark of EOS. In general, blood cultures growing clinically significant pathogens are positive within 24–36 h of incubation (51, 52). Molecular assays like real-time PCR have been evaluated for the rapid detection of pathogens in neonatal EOS. They provide care providers with the critical information of organism identification and antimicrobial susceptibility from cultures flagged as positive, faster than with traditional microbiology methods, which may allow the clinicians to narrow empiric antibiotic choices more efficiently. They are also being studied as 'add-on' tests to identify pathogens that are not detected by conventional blood or CSF culture (53, 54).
Low white blood cell counts, low absolute neutrophil counts, and high immature-to-total neutrophil ratios are associated with increased odds of infection, but the sensitivities of complete blood count (CBC) indices were low (0.3–54.5%) to rule out EOS (55, 56). There is concern regarding falsely normal white blood cell screening tests among infants with fulminant sepsis, particularly when the CBC screening is performed between 1 and 7 h of life; consequently, repeated CBC screenings between 12 and 24 h of age are reasonable (57, 58).
C-reactive protein (CRP) is a non-specific inflammatory marker that typically begins to increase 6–8 h after infection onset. Levels are only elevated in 35–55% of neonates at the onset of illness, and for the first few hours after birth (59, 60). It is sensitive but not specific, so if it remains normal at 24–36 hours and the infant appears well, antibiotics can be safely discontinued (61, 62).
In the Neonatal Procalcitonin Intervention Study (NeoPInS), Procalcitonin-guided decision making was found to be superior to standard care in reducing antibiotic therapy in neonates with suspected EOS (intention to treat: 55.1 vs. 65.0 h, p < 0.0001) (63). Dongen et al. reported that umbilical cord blood procalcitonin (PCT) levels are increased in newborns ≥32 weeks with proven or probable EOS, but PCT might not be a reliable marker after maternal antibiotic treatment (64).
Cytokines, including interleukin 6 (IL-6), interleukin 8 (IL-8), gamma interferon (IFN-γ), and tumor necrosis factor alpha (TNF-α), and cell surface antigens such as soluble intercellular adhesion molecule (sICAM) and CD64, have been studied as measures for neonatal sepsis; however, the heterogeneity of available evidence prevents conclusions regarding their routine use as definitive diagnostic tools (38, 65, 66).
There is no additional benefit to include urine microscopy or culture as part of the investigations for early-onset neonatal infection in the first 24 h of life among both preterm and term infants because most infections of the urinary tract in this population are secondary to hematogenous seeding of the kidney by bacteremia (67, 68).
While lumbar puncture (LP) is an important means of obtaining CSF to rule out the presence of meningitis in infants, its routine use in neonates for EOS evaluation remains controversial (38). In any infants, the timely collection of CSF samples should balance the cardiorespiratory stability of the infant, the risk of EOS, and the potential harm associated with delayed antibiotic therapy. While antibiotics can render the CSF culture negative and no single CSF parameter can reliably exclude the presence of meningitis, antibiotic initiation should not be delayed by the LP procedure itself in critically ill infants (69).
Risk Assessment for EOS in Infants Born at ≥35 Weeks' Gestation
The primary focus is to identify infants at high risk for infection to warrant empiric antibiotic therapy. Three approaches currently exist in the literature for the use of risk factors to identify infants who are at increased risk of EOS (50).
In the first approach, the following threshold values for intrapartum risk factors are utilized: (i) any newborn infant who is ill appearing; (ii) a mother with a clinical diagnosis of chorioamnionitis; (iii) a mother with GBS colonization and who received inadequate IAP, with a duration of ROM of >18 hours or birth before 37 weeks of gestation; or (iv) a mother with GBS colonization and who received inadequate IAP but with no additional risk factors. Recommendations include observation in the hospital, laboratory testing, empirical antibiotic therapy for infants, or a combination of these (50, 70).
In the second approach of the multivariate risk prediction model, an individualized risk of EOS based on several risk factors is generated, and then that risk is modified depending on the infant's clinical condition specified by explicit clinical characteristics in the first 12 h after birth (34). The Kaiser Permanente Research group developed an online sepsis risk calculator (SRC) to evaluate the risk of neonatal EOS, which has been validated as a safe way to reduce the proportion of infants receiving empiric antibiotics without apparent adverse effects in infants born after ≥35 weeks' gestation (71).
The final approach consists of serial evaluations within the first 48 hours of age for clinical signs of illness among infants with risk factors to identify infants with EOS, which have been shown to significantly decrease with the use of laboratory tests, blood cultures, and empirical antibiotic agents (50, 72).
Canadian Pediatric Society has also developed an algorithm to provide updated recommendations for the care of term (≥37 weeks' gestation) newborns with risk factors for EOS during the first 24 h of life (73).
The above mentioned approaches and algorithms must be used in conjunction with meticulous history-taking, careful clinical examination, and serial assessment of the at-risk infant. Used in this way, clinicians can identify those infants who are at high risk of developing EOS and prevent unnecessary antibiotic exposure in a substantially larger number of infants who are uninfected.
Blood culture is the gold standard for diagnosis of EOS, but its sensitivity can be affected due to small volume of blood sample and antibiotics given to newborn before sampling. In the recent years, umbilical cord blood culture for the diagnosis of EOS in term infants has been advocated as a large volume can be readily obtained and shown to have >80% sensitivity and specificity compared to venous blood samples (74), though strict and proper sterile techniques are needed to prevent contamination (75).
Risk Assessment for EOS in Infants Born at ≤34 Weeks' Gestation
GA is the strongest predictor of EOS, and two-thirds of preterm births are associated with preterm labor, PROM, or clinical concern for intrauterine infection (49). Clinical features and common laboratory tests, such as CBC and CRP, are impacted by maternal conditions and cannot be reliably used to predict EOS among preterm infants. The objective of EOS risk assessment among preterm infants is to determine which infants are at the lowest risk for infection such that they are eligible to be spared from the administration of empirical antibiotics, or at the very least to be administered a shorter duration of empirical antimicrobial coverage (39).
Preterm infants who are considered at a lower risk for EOS have the following characteristics at the time of delivery: (i) obstetric indications for preterm birth (e.g., maternal preeclampsia or other noninfectious medical illness or placental insufficiency); (ii) cesarean birth; and (iii) absence of labor, attempts to induce labor, or any ROM before delivery (76, 77). Infants fulfilling these criteria can be considered at the lowest risk of EOS and may be managed without empiric antibiotics at birth (39). In a single-center study evaluating the impact of such a risk-factor approach in EOS evaluation among preterm infants, the guideline implementation was associated with decreased antibiotic initiation among low-risk, extremely low birth weight (ELBW) infants, without any change in incidence of confirmed infection or death (78). A randomized controlled trial is currently being conducted to test if the incidence of adverse outcomes is higher in babies receiving empiric antibiotics in the first week of life compared to babies receiving placebo (79). This study targets a population of ELBW infants that are clinically stable that did not have a known exposure to intraamniotic infection and were not born preterm for maternal indications.
In contrast, infants born preterm because of maternal cervical incompetence, preterm labor, PROM, clinical concern for intra-amniotic infection, or acute onset of unexplained non-reassuring fetal status are at the highest risk for EOS. All should undergo EOS evaluation with blood culture and empirical antibiotic treatment (39). Clinicians often express concerns about poor sensitivity of blood cultures in neonates resulting from inadequate inoculant volumes, resulting in frequent diagnosis of “culture-negative sepsis”, and prolonged use of empiric antibiotics in neonatal units (80). To help avoid a “culture-negative sepsis” diagnosis and prolonged use of unnecessary empiric antibiotics in neonates, a minimum of 1 ml of blood culture volume has been recommended, based on the bench study and expert recommendations (80–83). Real-time PCR has the advantage of detecting organisms present in low concentrations using small volumes of blood and providing rapid results. However, the sensitivity and specificity for diagnosis of EOS and detection of antibiotic resistance remains under investigation (53, 84).
Empirical Antimicrobial Therapy of EOS
The combination of ampicillin and gentamicin is most commonly administered to neonates at risk for EOS. This combination targets the most common pathogens pending culture results (39, 50). Aminoglycoside-based regimens should generally be used instead of cefotaxime-based treatments because of lower levels of susceptibility to cefotaxime and to potentially prevent the development of antibiotic resistance (85).
The empirical administration of more broad-spectrum agents may be indicated in infants who are critically ill with additional risk factors, particularly after prolonged antepartum maternal antibiotic treatment, or if the mother is known to be colonized with an antimicrobial resistant organism, until appropriate culture results are known (39, 50). In some places, benzylpenicillin is being used instead of ampicillin, which is a narrower-spectrum alternative. Decisions regarding the optimal empirical coverage should be guided by the local epidemiology and antibiogram.
Importance of Judicious Antimicrobial Use in Early Life
The use of the narrowest-spectrum effective antimicrobials allows for the targeted killing of the infecting pathogen while minimizing undesirable impacts on the colonizing microbial flora of the infants. At the individual level, antibiotic use either perinatally or postnatally has been linked to disruptions in the microbiome (86, 87). Alteration in intestinal microbiota has been postulated to play a critical role in the development of significant neonatal morbidities, through the regulation of systemic inflammation (19). Antibiotic treatment in the first week after birth may also increase the subsequent risk of wheezing, infantile colic, and childhood obesity (88, 89). A study from the Canadian Neonatal Network demonstrated that a prolonged initial antibiotic exposure of >3 days within the first week after birth was associated with increased odds of mortality or significant morbidities, including chronic lung disease, patent ductus arteriosus, retinopathy of prematurity, and severe neurologic injury, even after adjustment for baseline differences in the characteristics of very-low-birth-weight (VLBW) infants (26).
Empirical cefotaxime use without justification should be avoided, as its overuse may increase the prevalence of MDRO, candidiasis, and possibly neonatal death (20, 21, 90, 91).
Re-evaluation for the Continuation of Antibiotic Treatment 36 h After Initiation
In the absence of positive blood culture or clear evidence of site-specific infection, infants that were administered empirical antibiotics because of risk factors for EOS or clinical indicators of possible infection who remain well should have their antibiotics discontinued after 36 h, particularly when the trends of CRP concentration are reassuring (39, 50, 65, 92). If antibiotics are continued for longer than 36 h despite negative blood cultures, the infant should be reexamined at least once every 24 h to determine whether it is appropriate to stop the antibiotic treatment (92).
Persistent cardiorespiratory instability is common among preterm infants with VLBW and this symptom is not by itself an indication for prolonged empirical antibiotic administration (39). Continuing empirical antibiotic administration in response to laboratory test abnormalities is rarely justified, particularly among preterm infants at a lower risk for EOS and those born with maternal obstetric conditions known to affect fetal hematopoiesis (39).
There have been concerns regarding the incomplete detection of low-level bacteremia and the effects of intrapartum antibiotic administration; modern blood culture systems can reliably detect low levels of bacteremia provided that 1 mL of blood is inoculated, and studies have shown no impact of IAP on time to detection of EOS (51, 93, 94).
Duration of Therapy for Positive Blood Culture
When EOS is confirmed by blood culture, a lumbar puncture should be performed if not done previously (39). Antibiotic choice should be pathogen-specific with a narrow effective spectrum of activity and should be guided by sensitivity results. There are a few studies that have guided the duration of therapy. The duration of antimicrobial therapy for uncomplicated bacteremia without a focus is usually 7–10 days with a pathogen-specific antibiotic (95). Seven days may be considered for some low-risk cases of neonates over 32 weeks of gestation and weighing over 1500 g (96–98). When uncomplicated meningitis attributable to GBS is diagnosed, therapy is extended to 14 days (95). Extended courses of treatment are indicated for complications, including cerebritis and osteomyelitis. Antimicrobial therapy is usually recommended to be administered for a minimum of 3 weeks for gram-negative meningitis, provided sterility of the CSF is ensured 24-48 hours after the appropriate treatment commences (99–101).
Prevention
IAP for at-risk women has become the standard of care in many countries over the past two decades and is an effective way to prevent EOS (102–104). A meta-analysis revealed that compared with a risk-based strategy, screening-based prophylaxis was associated with a reduced risk of GBS-EOS (105). The Society of Obstetricians and Gynecologists of Canada (SOGC), in 2018, reaffirmed the recommendation of offering all women screening for colonization with GBS at 35–37 weeks of gestation, by performing culture tests with one swab from the vagina and one from the rectum (106).
Practice Summary
• GBS and E. coli are the most common organisms responsible for EOS in term and preterm infants, respectively.
• CBC-derived index or CRP cannot reliably rule out EOS.
• Ampicillin and gentamicin in combination have good efficacy for most causative organisms in EOS, although the choice of therapy should be guided by local antibiotic susceptibility data.
• Unless there is clear evidence of sepsis or site-specific infection, empirical antibiotic therapy should be discontinued after 36 h if the blood culture is negative, particularly when there are no other markers of infection (e.g. C-reactive protein).
• Preterm infants at a lower risk for EOS may be managed without empiric antibiotics at birth.
• The judicious use of antibiotics should be promoted to decrease the risks associated with prolonged antibiotic administration in the face of sterile cultures and with the use of unnecessary broad-spectrum therapy.
Late Onset Sepsis
In general, LOS refers to an invasive infection occurring in neonates older than 3 days of life, indicating postnatal (hospital or community) acquisition (107–109). It can lead to life-threatening organ dysfunction caused by a dysregulated host response to infection (110, 111). LOS accounts for a significant proportion of morbidity and mortality in neonates and may adversely affect brain development and lead to neurodevelopmental impairment among survivors (33, 112).
Epidemiology, Microbiology, and Risk Factors
The two major risk factors that predispose neonates in the NICU to sepsis are preterm birth and low birth weight (111). In the Canadian Neonatal Network, a 5% incidence of LOS has been reported among neonates with a BW of 1000–1499 g, who survived for more than two calendar days, but the incidence increased to 30–36% in those with a BW of 750–999 g (47, 48). Other known risk factors for LOS include prolonged mechanical ventilation, use of intravascular devices, failure of early breast milk feeding, and prolonged hospitalization (111, 113, 114). Central line–associated bloodstream infections (CLABSIs) are the most common type of LOS among infants in the NICU, resulting from extraluminal or intraluminal catheter contamination after colonization of the neonatal skin, oropharynx, intestinal tract, and/or the catheter surface (115).
LOS can result from viral, bacterial, or fungal microorganisms (111). In infants admitted to NICUs, coagulase-negative Staphylococcus (CoNS) is the most common pathogen in LOS (116). Other common bacteria include Staphylococcus aureus, E. coli, Klebsiella spp, Enterococcus spp, and GBS (116). Neonates are also susceptible to invasive infections from organisms found in the hospital environment, such as Enterobacter spp, Pseudomonas spp, and Serratia spp. Fungi, specifically yeasts as Candida spp., can also cause systemic infections; however, its incidences varied across different NICUs (117).
Diagnostics
The gold standard for the diagnosis of LOS is a positive blood culture. However, limitations to positive blood culture include the delay between collection and positivity, false positive results from contamination, and false negatives resulting from antibiotic therapy prior to collection or submission of inadequate blood volume (116). A minimum of 1 mL of blood should preferably be obtained from two different venipuncture sites (83, 118). This minimum volume is recommended due to the potential for paucibacillary infection (1–10 colony-forming units (CFU) per milliliter), with a reported sensitivity of 98% for volumes >1 mL (119). In adults, if an intravascular catheter is in place, peripheral and central blood cultures can improve the diagnosis of central line-associated bloodstream infection based on differences in time to positivity of culture from the two sites of >2 h and may also aid in the identification of sample contamination (120). This concept has not been studied in the neonatal population, likely because of the relatively small-sized lumen of their central venous catheters.
Neonatal meningitis frequently occurs in the absence of bacteremia in patients with LOS (69). Conducting LP is important in the diagnosis of meningitis, although its interpretation can be affected by the high frequency of traumatic LPs and the variability of cytologic and biochemical indices in the neonatal population (121). Repeated CSF fluid parameters may provide additional insights into the treatment response and adverse effects from the outcomes of bacterial meningitis (122). Bacterial nucleic acid detection in CSF (such as 16s ribosomal RNA) can be useful if CSF culture results are negative because of preceding antibiotic therapy. Urine examination is also an important component of LOS evaluation, as UTI is not uncommon, particularly among preterm infants [Please refer to Section 6 on “urinary tract infection” for diagnostics, interpretation, and management].
Due to non-specific symptomatology, biomarkers are used in addition to microbiologic cultures to guide the initiation and duration of antibiotic therapy (123, 124). There are numerous sepsis biomarkers like chemokines, cytokines, leucocyte cell surface antigens, and acute phase proteins described, however, none of these are considered standard of care at the bedside (125). Procalcitonin and CRP are the most extensively studied biomarkers and appear to have the greatest diagnostic utility at 12–24 h (mid-phase) and >24 h (late phase) from initial clinical suspicion of LOS (123, 124). Single values of CRP or procalcitonin are neither sensitive nor specific to guide care decisions in the diagnosis of sepsis. Two CRP levels (<10 mg/L obtained at 24 hours apart and at 8–48 h after onset of possible LOS) indicated that bacterial infection is unlikely (60).
Empirical Antimicrobial Therapy of LOS
Empiric antimicrobial therapy should be guided by local epidemiology (e.g., methicillin-resistant Staphylococcus aureus (MRSA) incidence) and antibiogram data, including coverage for common gram-negative and gram-positive pathogens (111). One must also consider the pharmacokinetic and pharmacodynamic properties of antibiotics in neonates (126). Cloxacillin, a narrow-spectrum antibiotic, is recommended to treat gram-positive LOS, especially if S. aureus infection is suspected. Vancomycin—a broad-spectrum antibiotic—is now discouraged as empiric LOS therapy, if the prevalence of MRSA in the community remains low, as it then becomes difficult to determine whether blood cultures positive for CoNS are contaminated (127). No survival benefit with empirical vancomycin therapy has been demonstrated for CoNS bloodstream infections in infants from a retrospective review including 4,364 infants from 348 NICUs, even though the median duration of bacteremia was 1 d longer in infants who received delayed vancomycin therapy (128).
Unless meningitis is suspected, gram-negative coverage with an aminoglycoside is preferred over third-generation cephalosporins because of the potential for the latter to induce antimicrobial resistance. In most jurisdictions, aminoglycosides are more likely to cover nosocomial gram-negative bacteremia (e.g., Enterobacter spp, Serratia spp, Pseudomonas spp) (129).
Duration of Antimicrobials for Established Bacteremia and Bacterial Meningitis
Currently, there are no evidence-based guidelines on the duration of antimicrobial treatment for neonatal bacteremia and meningitis. The duration is determined by each patient's risk factors, clinical evaluation, laboratory tests, specific pathogens that are identified, and their susceptibility to antimicrobials (111, 130).
For bacteremia, in general, the recommended duration of antibiotics ranges from 7 to 14 days, depending on the pathogen isolated. Using CRP and blood cultures as a measure, it was found that a 10-day antibiotic duration was as effective as 14 days in neonatal bacteremia (97). In another study, 69 neonates with septicemia were randomized to receive a 7- or 14-day course of antibiotics (98). Although the 7-day antibiotic treatment appeared to be sufficient for other pathogens, neonates with S. aureus bacteremia experienced more treatment failure with 7 days of treatment compared to that with 14 days of treatment, which thus remains the recommended duration (98). Of note, retention of the central venous catheter in neonates with bloodstream infection is associated with delayed resolution of bacteremia and a higher incidence of recurrence (131).
The treatment duration for meningitis has traditionally ranged from 14 to 21 days, depending on the pathogen isolated. For example, 14 days of treatment is recommended for uncomplicated GBS meningitis, whereas a minimum of 21 days is recommended for uncomplicated meningitis caused by gram-negative bacteria (132, 133). However, it is important to note that the treatment duration should ultimately be individualized to the pathogen and the patient's clinical response.
Management of Culture-Negative Sepsis
Culture-negative sepsis implies that a neonate is clinically suspected to have sepsis, often due to nonspecific symptoms; therefore, a complete course of antibiotics is prescribed despite sterile cultures. As aforementioned, sterile cultures may be falsely negative owing to low-level bacteremia, antibiotic pre-treatment, or bacterial infection at a site that is not readily cultured (such as the lung) (134). As per recent reports, up to ten-fold higher antibiotic utilization for culture-negative sepsis is observed than that for culture-proven sepsis, (127, 135) however, this varies widely between institutions. Empirical therapy should be limited to 24–36 h if the infant shows no subsequent clinical or laboratory evidence of infection and cultures are negative (126).
Prolonged antibiotic courses (>5 days) in culture-negative settings have been associated with increased odds of necrotizing enterocolitis (NEC) and death (4% increased risk with each additional day) (136). Unnecessary antimicrobial use is increasingly recognized to pose significant harm to NICU patients, including increased risk of invasive candidiasis, prolonged hospitalization, bacterial resistance, (137) and predisposition to inflammatory dysregulation with long-term adverse health outcomes (138).
Practice Summary
• Blood cultures are the gold standard for diagnosis of LOS. Every effort should be taken to optimize the sensitivity and specificity of blood culture (1 mL is considered the minimal volume).
• Normal values of serial CRP obtained at 24 h apart and at 8–48 h after the onset of possible LOS indicated that bacterial infection is unlikely.
• For LOS without concerns of meningitis, cloxacillin, and gentamicin are the recommended antibiotics to provide empirical coverage for S. aureus and most gram-negative bacteria, considering the local epidemiology and antimicrobial resistance risk factors.
• For patients with clusters of gram-positive cocci or CoNS as the preliminary finding in blood cultures, a second culture should be obtained prior to initiating vancomycin to reduce the overtreatment of potential CoNS-contaminated cultures.
• The duration of therapy is dependent on the type of pathogens and infectious complications, but generally 7 to 14 days are adequate for neonatal bacteremia.
• Retention of the central venous catheter in neonates with bloodstream infection can be associated with delayed resolution of bacteremia and a higher incidence of recurrence.
• Prolonged antibiotic courses (especially >5 days) in culture-negative settings have been associated with adverse neonatal outcomes and are thus discouraged.
Ventilator-Associated Pneumonia
Neonatal ventilator-associated pneumonia (VAP) is an important healthcare-associated infection (HAI) with significant morbidity, ranging from 1.1 to 10.9 episodes per 1,000 ventilator-days, and remains a leading cause of antibiotic use in the NICU (139–142). Quality improvement efforts are challenging from many perspectives, including surveillance, diagnostic accuracy, and optimal therapy. Barriers to improvement in neonatal VAP practices include the lack of standardization or guidelines for definition and surveillance, over-reliance on microbiological results, uncertainty regarding the choice of empiric antibiotics, and duration of antimicrobial treatment (143–147). These barriers result in major practice variations and antimicrobial overuse in this era of heightened antimicrobial stewardship efforts.
Definition and Surveillance
Historically, the CDC definition of VAP for infants up to one year of age was the most widely used definition for neonates, but this definition was criticized for its diagnostic inconsistency, especially for preterm neonates (148). Initiatives to refine the CDC definition of VAP have led to the introduction of the term pediatric ventilator-associated condition (VAC) by the National Health and Safety Network (NHSN). Pediatric VAC has an objective definition for respiratory deterioration defined as increase in mean airway pressure ≥4 mmHg and/or FiO2 ≥0.25 for a specified duration of ≥2 calendar days (149). As pediatric VAC is not specific for infectious ventilator events, additional clinical and management criteria are required to fulfill the conditions for infection-related ventilator-associated complication (IVAC), and additional microbiological criteria are required to fulfill the criteria for possible VAP (150). Chest radiograph findings have been removed from the NHSN criteria for VAC, IVAC, and possible VAP for all populations due to concerns regarding reliability, which is particularly relevant in that the interpretation of radiological changes is especially challenging in neonates with pre-existing lung pathologies (148, 151). Quality improvement initiatives that target VAP, using internally consistent definitions, have been successful in improving practices (148–157) and are, therefore, encouraged (148).
Microbiology
Purulence defined as ≥25 polymorphonuclear leukocytes per low power field on tracheal aspirates (TA) and bronchioalveolar lavage (BAL) microscopy has been correlated with the duration of mechanical ventilation and the incidence of bronchopulmonary dysplasia, but not with clinical symptoms (146, 147, 158–161). Similarly, time since intubation is the strongest predictor of positive TA quantitative cultures, with approximately half of the bacterial growth occurring after 4–10 days of mechanical ventilation and almost 100% after 10 days (148, 161, 162). Most positive TA cultures represent endotracheal tube colonization and oropharyngeal contamination rather than infection (147, 148, 160) as supported by the fact that up to 58% of TA cultures are polymicrobial (139). Thus, a positive microbiological result is only one of several criteria for VAP. Certain groups have reported that less invasive BAL (“mini-BAL”) is safe in preterm infants and may decrease the false positive rates in VAP diagnosis (140, 158, 160).
Antimicrobial Treatment – Choice and Duration
The choice of empirical therapy should be made after considering the severity of illness, prior colonization, local antibiogram data, and risk factors for multidrug-resistant (MDR) pathogens (163). The most common pathogens for VAP are S. aureus and Enterobacteriaceae, and these organisms should be the target of empirical antimicrobials, whereas Pseudomonas aeruginosa should be targeted only in NICUs with a high prevalence of this pathogen. Narrow-spectrum therapy (e.g., cefazolin or a combination of cloxacillin plus either an aminoglycoside or cefotaxime) should be favored in non-critically ill neonates with no prior history of colonization with resistant bacteria, and in NICUs with low rates (<10%) of multidrug-resistant pathogens (164, 165).
After initiation of empirical therapy for possible VAP, antimicrobial agents should be reassessed 24–36 h after their initiation (148, 166). If an alternative diagnosis is established that explains the patient's clinical or radiological deterioration, or if TA cultures are negative, antimicrobial therapy for VAP should be discontinued. In neonates with probable VAP, the antimicrobial spectrum should be narrowed based on the culture results (164, 167). Combinations of antibiotics are not recommended as this does not hasten clinical recovery.
The optimal treatment duration for VAP in neonates is unknown (168). However, some key principles, such as time to clinical improvement, microbial burden/source control, and host immune status should be considered (169). A meta-analysis of four randomized controlled trials in immunocompetent adults suggests that a 7-to 8-day antibiotic treatment, irrespective of the causative pathogen, is as effective as a 15-day course in terms of clinical cure, mortality, and relapse risks, and is associated with a lower risk of subsequent MDR infections (164, 170). Treatment duration should be longer for those with complicated VAP (e.g., pneumatocele, lung abscess, or empyema), VAP with secondary bacteremia, and for those with underlying comorbidities impacting immunological response.
Practice Summary
• Definitions for neonatal VAP should be internally consistent and align with external standards to allow for future benchmarking. A dedicated multidisciplinary team that includes neonatology and infectious disease specialists with VAP expertise should review all possible VAP cases. Due to high rates of colonization/contamination, positive microbiologic results should not be the major consideration for defining VAP. An objective review of all clinical criteria is required to determine the pathogenicity of bacteria grown from tracheal secretions.
• Narrow-spectrum therapy should be favored in non-critically ill neonates with no prior history of colonization with resistant bacteria.
• After initiation of empirical therapy for possible VAP, antimicrobial agents should be reassessed 24–36 h after their initiation. If an alternative diagnosis is identified to explain the patient's clinical or radiological deterioration, or if tracheal aspirate cultures are negative, antimicrobial therapy that is specifically targeting VAP should be discontinued.
• The optimal treatment duration for VAP in neonates is unknown, although a 7- to 8-day antibiotic treatment may be reasonable based on the limited existing literature.
Necrotizing Enterocolitis
Necrotizing enterocolitis (NEC) is a leading cause of neonatal morbidity, affecting approximately 1 in 1000 live births (171). Premature infants are especially at risk, with an incidence of up to 2.5 and 4.5% in low birth weight (BW) and VLBW infants, respectively (172). Classical management plans include bowel rest, supportive care, antibiotics, and surgery, when necessary. Despite optimal care, outcomes can be poor with high mortality rates and serious complications such as intestinal strictures, short bowel syndrome, or neurodevelopmental impairment (172–179).
Definition
Multiple risk factors are involved in the pathogenesis of NEC, including intestinal inflammation, ischemia, and bacterial overgrowth in a highly vulnerable gastrointestinal tract (180). Bacterial overgrowth may progress to bacterial translocation across the intestinal wall and intra-abdominal infection with localized or diffused peritonitis, which can lead to bowel perforation (181, 182). Spontaneous intestinal perforation (SIP) is different from NEC. SIP generally occurs in the first week of age in very preterm infants and is not discussed in this narrative review (183).
Diagnosis and staging rely on a combination of clinical, laboratory, and radiologic findings, typically using the modified Bell's staging criteria (184). Bell stage I or suspected NEC presentation is non-specific, and symptoms include feeding intolerance, abdominal distension, and occult blood in the stool. Stage II NEC presents with ileus, dilated bowel loops, grossly bloody stools, and pneumatosis. Severe NEC or stage III is characterized by respiratory and metabolic acidosis, a fixed bowel loop, and may progress to intestinal perforation (184, 185).
Microbiology
Numerous pathogens are associated with NEC. For most infants, blood and peritoneal fluid cultures remain negative, but intra-abdominal polymicrobial infections are assumed to be present (186–189). Since NEC is associated with local bacterial overgrowth of endogenous intestinal flora, antibiotic empirical therapy should target enteric gram-negative aerobic and facultative anaerobic bacilli such as Enterobacteriaceae (e.g., E. coli and Klebsiella species), enteric gram-positive streptococci (e.g., Streptococcus anginosus), and in some situations, obligate anaerobic bacilli (e.g., Clostridium perfringens and Bacteroides fragilis) (190–192).
Antimicrobial Treatment – Choice and Duration
There is a wide variability in empiric antibiotic regimens used in clinical practice to treat NEC, reflecting the lack of clear guidelines on the type and duration of antibiotic treatment (175, 189, 193). The Surgical Infection Society and the Infectious Diseases Society of America (IDSA) published the only guideline addressing this issue in 2010 (190); it mainly discusses the treatment of complicated intra-abdominal infections (cIAI) in adults and older children. For NEC specifically, IDSA recommendations are based on experts' opinions and include the following combinations of broad-spectrum antibiotics: (i) ampicillin, gentamicin, and metronidazole, (ii) ampicillin, cefotaxime, and metronidazole, or (iii) meropenem (190).
To date, published studies have failed to demonstrate the optimal antimicrobial regimen for NEC in terms of efficacy and safety (190, 194). A Cochrane systematic review included two randomized controlled trials (RCT) conducted in the 1980s (194). The first RCT compared ampicillin-gentamicin to ampicillin-gentamicin-clindamycin in 42 premature infants with NEC (195); it showed no beneficial effect on mortality or intestinal perforation with the addition of clindamycin, but found a significantly longer time to successful reinstitution of enteral feeding and a higher incidence of stricture formation in the clindamycin group (195). The other RCT included 20 infants and showed no difference in outcomes with enteral vs. parenteral gentamicin when administered in combination with parenteral ampicillin and gentamicin (196).
More recently, a large multicenter, partly randomized controlled trial was completed with 128 infants selected randomly, and an additional 52 infants were enrolled on a non-randomized basis (197). All infants <121 days of age (≤33 weeks GA) had a cIAI and received one of the following three antibiotic combinations: ampicillin/gentamicin/metronidazole; ampicillin/ gentamicin/ clindamycin; or piperacillin-tazobactam/ gentamicin within 48 h of diagnosis. Mortality assessed at 30 days was similar in all three arms at 8–9%. Adverse events up to 90 days follow-up, events of special interest (including gastrointestinal surgeries, progression to a higher stage of NEC, intestinal stricture or perforation, feeding intolerance), and therapeutic success on day 30 were also similar among the three groups. This large RCT does not support the choice of one regimen over another for the treatment of infants with cIAI.
Finally, several observational retrospective studies have compared antibiotic regimens for NEC management. Most were single-center studies with a limited sample size (189, 193, 198, 199). The largest of all observational retrospective studies involved 2,780 infants with <1500 g BW and diagnosed with NEC from 348 centers (175). After propensity score matching, the results suggest that for infants with NEC that were managed without surgery (stage II), anaerobic coverage was not associated with lower mortality, but resulted in an increased risk of intestinal strictures. However, in infants with NEC that was managed surgically (stage III), anaerobic antimicrobial therapy was associated with lower mortality. These results suggest that anaerobic coverage is beneficial in cases of severe and perforated NEC (stage III).
In summary, there is insufficient evidence to support one antibiotic regimen over another for the treatment of NEC. Empirical therapy should be effective against most pathogenic bacteria usually present in the intestinal flora (e.g., Enterobacteriaceae and gram-positive cocci). Clinicians should also consider local resistance rates, individual patients' microbiologic results, and clinical evolution to guide their antibiotic choice. Given that broad-spectrum antibiotics (including 3rd generation cephalosporins and carbapenems) increase the risk of adverse outcomes (IC, increased risk of colonization with antimicrobial-resistant organisms, and microbiota alterations), narrow-spectrum regimens and short therapy durations are encouraged.
There is no evidence supporting the optimal duration of antibiotic therapy for NEC (189). In stage I and stage IIA NEC (modified Bell's stage) (184), experts are generally in favor of discontinuing antibiotics after 3 days and after 5–7 days, respectively, if symptoms are resolved. In higher-stage NEC (IIB and III) or when the infant presents with bacteremia or sepsis, the duration of the antibiotics is generally extended to 10–14 days. If an intra-abdominal abscess has been identified, antibiotics should be continued until clinical and radiological responses are established. The recurrence rate of NEC after the first course of antimicrobial or surgical treatment does not appear to be reduced by a longer duration of treatment (200).
Finally, NEC is a risk factor for invasive candidiasis (IC), and fluconazole prophylaxis may be beneficial for high-risk infants. A study in very low BW infants, <6 weeks postnatal age, receiving broad-spectrum antibiotics for >2 days with 1 additional risk factor including NEC, showed that fluconazole prophylaxis reduced the incidence of IC, but failed to demonstrate a survival benefit (201).
Practice Summary
• In infants with Bell stage I or suspected NEC, consider parenteral antibiotics such as ampicillin and gentamicin for 48 to 72 h pending cultures and evolution.
• In infants with Bell stage II or III NEC, therapy may include the following parenteral antibiotic regimens:
- Ampicillin and gentamicin +/- metronidazole or clindamycin;
- Piperacillin-tazobactam as a single agent, especially for more severe presentation.
- Anaerobic coverage may be added for more severe presentation (stage IIB and III NEC)
• In infants with Bell stage II NEC, antibiotics may be discontinued after 5–7 days if symptoms are resolved.
• In infants with Bell stage III NEC or when the infant presents with bacteremia or sepsis, the duration of the antibiotics should be 10–14 days.
Urinary Tract Infection
Urinary tract infections (UTIs) are significant bacterial infections in neonates. The incidence and prevalence of neonatal UTIs range from 3–25% in preterm and 0.1–20% in term infants (202–207). Clinical presentation of neonatal UTIs is non-specific and may include the following: poor feeding, failure to thrive, vomiting, diarrhea, prolonged jaundice, lethargy, irritability, hypothermia, hypoglycemia, abdominal distention, and bradycardic events (204–206). Over 50% of premature infants present with respiratory symptoms including apnea, hypoxia, or tachypnea (206). Risk factors include male sex, being uncircumcised, prematurity, VLBW (<1,500 g), renal/urinary tract malformations, and prolonged hospitalization (202–209). Urine collection should be part of LOS evaluations.
Microbiology
The most common pathogen found in neonatal UTIs is E. coli, followed by Klebsiella pneumoniae and Enterobacter spp (204–206, 210, 211). Less common pathogens include: Pseudomonas aeruginosa, Enterococcus spp, Group B Streptococcus, S. aureus, viridans group Streptococci, Citrobacter freundii, Serratia marcescens, Klebsiella oxytoca, and Proteus vulgaris (205, 206, 210). Neonatal UTIs caused by fungal pathogens, specifically Candida species, could be associated with higher mortality (204, 208, 212, 213). Patients with vesicoureteral reflux may present with less common pathogens and are predisposed to pyelonephritis (204, 205, 211).
Sample Collection
Suprapubic aspiration is the recommended method, but it is an invasive procedure that requires operators with skills in point-of-care ultrasound (POCUS) (206, 214, 215). Urinary catheterization has also been shown to be feasible in preterm infants, even with 24 weeks gestation, although it can also be technically difficult (214). Proper disinfection of the penis or perineum is essential to minimize the possibility of sample contamination. Sterile bag collection is not recommended because of its high contamination rate (204–206).
Diagnostic Definitions
The thresholds for laboratory values diagnostic for UTIs vary across literature. The current gold standard for UTI diagnosis is a urine sample obtained by suprapubic aspiration or urinary catheterization that is positive for a single pathogenic organism (204–206). The most accepted threshold of pathogen growth is a concentration of ≥5x107CFU/L (211). However, some studies have included a threshold of ≥107CFU/L, especially in the presence of pyuria, to define a positive culture (204–206).
Elevated CRP and erythrocyte sedimentation rates were reported to have low sensitivity and specificity among neonates with UTI (204–206).
Antimicrobial Treatment—Choice and Duration
The initial treatment for neonatal UTI involves empirical parenteral broad-spectrum antibiotics (204–206). Most commonly, a combination of ampicillin and gentamicin or ampicillin and cefotaxime is administered to cover Enterococcus spp and gram-negative bacilli (204–206). Once a pathogenic organism is identified, treatment is tailored based on antimicrobial sensitivities (204–206). There is currently a lack of evidence on the optimal treatment duration. Traditionally, neonates were treated intravenously for 5–7 days (204–206). However, there is increasing evidence that term infants can complete therapy with oral antibiotics (216). The use of antibiotic prophylaxis for neonates with recurrent UTIs is not supported by evidence (205, 206).
Sequelae and Associations
The risk of detecting bacteremia in an infant with a UTI is moderate, ranging from 3–17%, and is inversely related to age (204, 205, 211, 217). Renal ultrasonography is recommended for all neonates after the first episode of an UTI because of the high incidence of associated congenital anomalies in this population (204–206, 211, 218, 219). Early diagnosis of urological abnormalities is critical for managing recurrent infections and preventing renal scarring (205, 212).
Practice Summary
• The clinical presentation of neonatal UTIs is often non-specific.
• Obtaining a urine sample for culture should be considered in LOS evaluations for neonates. Suprapubic tap should be attempted if expertise exists and especially if POCUS is available. Meticulous site disinfection is essential when obtaining a catheter sample to minimize contamination.
• A combination of ampicillin and gentamicin or ampicillin and cefotaxime can be administered to provide empirical coverage for UTI, although local resistance patterns should be considered.
• There is currently a lack of evidence regarding the optimal treatment duration for neonatal UTI. Most studies recommend a treatment duration of 5–7 days.
Surgical Prophylaxis
Surgical site infections (SSIs) involve the skin, subcutaneous, or deep tissue of an area of surgical intervention within 30 days of surgery (220). These are one of the most common healthcare-associated infections (221) and are an important cause of morbidity in the NICU (222). The reported incidence of SSI in infants in the NICU ranges from 4.7 (222) to 11.7% (223). Prophylactic antibiotics (given within 60 minutes of skin incision to mitigate the risk of SSI) may be unnecessary, overly broad spectrum, or given inappropriately for prolonged periods (224). Inappropriate antibiotic use drives antimicrobial resistance. In the NICU, it has been associated with altered intestinal microbiota, increased risk of IC, and LOS, and in VLBW infants, NEC and death (16–18, 225, 226). Although guidelines exist to aid clinicians with the timing, class, and duration of antimicrobial prophylaxis, there is a paucity of evidence for the NICU (227, 228). Institutional guidelines for SSI prophylaxis can help reduce the rates of infection while limiting adverse effects from inappropriate antimicrobials administration (224) (Table 2).
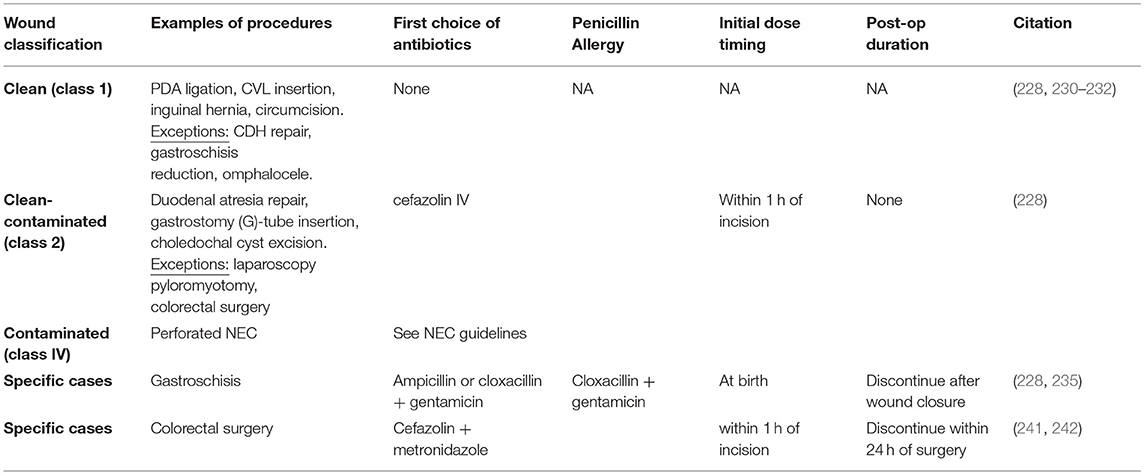
Table 2. Prophylaxis recommendations for surgical site infections in neonates [adapted from Laituri et al. (228)].
Clean (Class 1)
Clean procedures—an incision in which no inflammation is encountered in a surgical procedure, without a break in sterile technique, and during which the respiratory, alimentary, and genitourinary tracts are not entered—include the insertion of a central venous line (CVL), patent ductus arteriosus (PDA) ligation, immediate repair of omphalocele, gastroschisis reduction, repair of congenital diaphragmatic hernia (CDH), inguinal hernia repair, and circumcision (228, 229). Data on the need for prophylactic antibiotics for peripherally-inserted central catheter placement is mixed (230–232). While some RCTs demonstrated reduced catheter-related bloodstream infection (230) and CoNS catheter-related sepsis, (231) others failed to show benefit (232). The rates of SSI with CVL can be as low as 0 per 100 procedures; therefore, the risks of antimicrobial exposure are unlikely to outweigh the benefits (222, 230).
Although omphalocele is considered a clean procedure, short-term preoperative antibiotics are recommended for primary closure of omphalocele, or antibiotics are continued until closure in staged repairs (233, 234).
Despite its classification as a clean procedure, wound infections in gastroschisis repair are common, leading to the argument that this procedure should be re-classified based on a higher level of contamination (222). Wound infections were more common in neonates undergoing delayed closure (21%) than in those undergoing immediate closure (8.2%) (235). Empiric coverage with a beta-lactamase-resistant penicillin derivative (i.e., cloxacillin) combined with an aminoglycoside initiated at birth and discontinued after wound closure is suggested (235).
Retrospective data demonstrated that SSI is an uncommon source of infection in children with congenital diaphragmatic hernia (CDH), occurring in less than 1% of 1,085 neonates, but prophylactic antibiotics are typically prescribed (236, 237).
There are no specific neonatal guidelines on SSI prophylaxis for PDA ligation, inguinal hernia, or circumcision, but apart from the above exceptions, prophylactic antibiotics are not recommended for clean procedures based on the expert opinion (228).
Clean-Contaminated (Class 2)
This class describes an operative wound in which the respiratory, alimentary, genital, or urinary tract is entered under controlled conditions and without unusual contamination. Clean-contaminated surgical procedures include esophageal atresia (EA), tracheoesophageal fistula (TEF) repair, duodenal atresia repair, gastrostomy G-tube insertion, choledochal cyst excision, and colorectal surgery.
A small retrospective review of 732 neonatal surgeries found no statistical difference in SSI among infants receiving greater than 24 h of postoperative antibiotics and those receiving less than 24 h for clean contaminated or contaminated gastrointestinal operations. Guidelines recommend a single dose of preoperative intravenous (IV) cefazolin prior to most clean-contaminated procedures (228).
A consensus on the role of antimicrobial prophylaxis for EA and TEF repair is lacking (228). A study on 48 patients with EA, isolated either normal oropharyngeal organisms or no organisms from the upper esophageal pouch of infants who underwent repair within the first 24 h despite half of them being on prophylactic antibiotics (238). All infants who underwent delayed closure demonstrated growth of micro-organisms, irrespective of whether they were on antibiotics, and only those on prophylactic antibiotics demonstrated growth of Pseudomonas and Serratia (238). Antibiotic prophylaxis for EA/TEF repair is often recommended with amoxicillin-clavulanate, or 24–48 h of cefazolin and metronidazole, piperacillin/tazobactam, or cefoxitin (228) in the absence of evidence of aspiration (238–240).
Despite a lack of pediatric data, the Surgical Care Improvement Project (SCIP) recommends SSI prophylaxis in children undergoing colorectal surgery (241, 242). This recommendation is based on a Cochrane review and meta-analysis of adult studies demonstrating significantly improved rates of SSI when prophylactic antibiotics were used compared to when no treatment was provided [risk ratio (RR): 0.34] (241, 242). There was a further 50% reduction in SSI when anaerobic coverage was added to agents targeting aerobic bacteria (RR: 0.55), and a 60% reduction when aerobic coverage was added to agents targeting anaerobes (RR: 0.41) (241, 242). SCIP guidelines recommend parenteral cefazolin + metronidazole within 1 h of incision and their discontinuation within 24 h of surgery completion (241).
Contaminated (Class 3)
There are no common class 3 procedures in the NICU population.
Contaminated Perforated Necrotizing Enterocolitis (Class 4)
Antimicrobial recommendations for NEC are covered in Section 5 of this narrative review.
Practice Summary
• Prophylactic antibiotics have not been shown to reduce SSI in neonates undergoing clean procedures (class I).
• Prior to most clean-contaminated procedures (class II), a single dose of preoperative cefazolin IV is suggested.
• For colorectal surgeries, 24 h of antimicrobial coverage with cefazolin and metronidazole is recommended as SSI prophylaxis.
• For the management of gastroschisis, empiric coverage with a beta-lactamase-resistant penicillin derivative combined with an aminoglycoside is recommended for SSI prophylaxis.
• More high-quality evidence is needed to guide the use of antimicrobials for SSIs in the NICU.
Conclusion
Antimicrobials are the most frequently used medications for neonates, yet the untoward side effects of unnecessary antimicrobial exposure need to be acknowledged. The NICU houses immunocompromised newborns who are highly susceptible to overwhelming infections, but at the same time, there is a lack of high-quality evidence on the definition and optimal antimicrobial management of infection-related conditions in these vulnerable populations. This narrative review summarizes the best practice points based on the existing yet limited literature.
Further research is required to promote the judicious use of antibiotics based on the best practices and to elucidate the most impactful stewardship interventions for very preterm infants. This can only be achieved by national collaborations to develop consensus around definition, management approaches, and quality improvement efforts to enhance the health outcomes of neonates in both the short- and long-term.
Future Direction of Research
Neonatal infection can result in significant short- and long-term adverse outcomes. Current practices are often based on expert consensus, because of lack of well-powered trials and the high risk of systematic errors. The definitions of some conditions and treatment recommendations are extrapolated from the pediatric or even adult studies, without proper validation in the neonatal populations.
Large randomized controlled trials assessing different antibiotic regimens in early- and late-onset neonatal sepsis with low risk of bias are warranted. Further exploration on the use of sepsis risk calculator in the initiation and cessation of antibiotic use, particularly among those born at <35 weeks GA is needed. Development of specific standardized diagnostic criteria for neonatal UTI and VAP is urgently needed, before one can evaluate their treatment outcomes properly. Study on the correlation of antibiotic treatment strategies with bowel recovery and other clinically relevant outcomes are lacking. The optimal management of “mild” NEC cases picked up by ultrasound but not the traditional radiographic and clinical criteria needs further studies. Further evaluation of the best surgical prophylaxis for infants undergoing surgeries with relatively new techniques like flap closure for gastroschisis and bronchoscopic repair of thoracic conditions are necessary.
Author Contributions
JT, JA, MD, AR, K-sL, JR, and PS contributed to conception of the review. JT, JA, SG-B, KA, SK, JL, GL, FM, and IV-T wrote the first draft of the manuscript. All authors contributed to manuscript revision, read, and approved the final version.
Funding
This work was supported by the Canadian Institutes of Health Research (201903PJT-420294-CA2-CAAA-245530), which has no role in the development of this review and the writing of manuscript.
Conflict of Interest
The authors declare that the research was conducted in the absence of any commercial or financial relationships that could be construed as a potential conflict of interest.
Publisher's Note
All claims expressed in this article are solely those of the authors and do not necessarily represent those of their affiliated organizations, or those of the publisher, the editors and the reviewers. Any product that may be evaluated in this article, or claim that may be made by its manufacturer, is not guaranteed or endorsed by the publisher.
Supplementary Material
The Supplementary Material for this article can be found online at: https://www.frontiersin.org/articles/10.3389/fped.2022.894005/full#supplementary-material
References
1. Osowicki J, Gwee A, Noronha J, Britton PN, Isaacs D, Lai TB, et al. Australia-wide point prevalence survey of antimicrobial prescribing in neonatal units: how much and how good? Pediatr Infect Dis J. (2015) 34:e185–90. doi: 10.1097/INF.0000000000000719
2. Clark RH, Bloom BT, Spitzer AR, Gerstmann DR. Reported medication use in the neonatal intensive care unit: data from a large national data set. Pediatrics. (2006) 117:1979–87. doi: 10.1542/peds.2005-1707
3. Engle WA, Kominiarek MA. Late preterm infants, early term infants, and timing of elective deliveries. Clin Perinatol. (2008) 35:325–41, vi. doi: 10.1016/j.clp.2008.03.003
4. Engle WD, Jackson GL, Sendelbach D, Ford D, Olesen B, Burton KM, et al. Neonatal pneumonia: comparison of 4 vs 7 days of antibiotic therapy in term and near-term infants. J Perinatol. (2000) 20:421–6. doi: 10.1038/sj.jp.7200416
5. Wojkowska-Mach J, Rozanska A, Borszewska-Kornacka M, Domanska J, Gadzinowski J, Gulczynska E, et al. Necrotising enterocolitis in preterm infants: epidemiology and antibiotic consumption in the Polish neonatology network neonatal intensive care units in 2009. PLoS ONE. (2014) 9:e92865. doi: 10.1371/journal.pone.0092865
6. Liem TB, Krediet TG, Fleer A, Egberts TC, Rademaker CM. Variation in antibiotic use in neonatal intensive care units in the Netherlands. J Antimicrob Chemother. (2010) 65:1270–5. doi: 10.1093/jac/dkq107
7. Korang SK, Safi S, Nava C, Greisen G, Gupta M, Lausten-Thomsen U, et al. Antibiotic regimens for late-onset neonatal sepsis. Cochrane Database Syst Rev. (2021) 5:CD013836. doi: 10.1002/14651858.CD013836.pub2
8. Korang SK, Safi S, Nava C, Gordon A, Gupta M, Greisen G, et al. Antibiotic regimens for early-onset neonatal sepsis. Cochrane Database Syst Rev. (2021) 5:CD013837. doi: 10.1002/14651858.CD013837.pub2
9. Cantey JB, Patel SJ. Antimicrobial stewardship in the NICU. Infect Dis Clin North Am. (2014) 28:247–61. doi: 10.1016/j.idc.2014.01.005
10. Cantey JB, Sanchez PJ. Prolonged antibiotic therapy for “culture-negative” sepsis in preterm infants: it's time to stop! J Pediatr. (2011) 159:707–8. doi: 10.1016/j.jpeds.2011.07.032
11. Cordero L, Ayers LW. Duration of empiric antibiotics for suspected early-onset sepsis in extremely low birth weight infants. Infect Control Hosp Epidemiol. (2003) 24:662–6. doi: 10.1086/502270
12. Spitzer AR, Kirkby S, Kornhauser M. Practice variation in suspected neonatal sepsis: a costly problem in neonatal intensive care. J Perinatol. (2005) 25:265–9. doi: 10.1038/sj.jp.7211252
13. Ting JY, Roberts A, Abou Mehrem A, Khurshid F, Drolet C, Monterrosa L, et al. Canadian Neonatal Network Investigators, Variability in antimicrobial use among infants born at <33 weeks gestational age. Infect Control Hosp Epidemiol. (2021) 1–5. doi: 10.1017/ice.2021.380
14. Saiman L. Risk factors for hospital-acquired infections in the neonatal intensive care unit. Semin Perinatol. (2002) 26:315–21. doi: 10.1053/sper.2002.36264
15. Saiman L, Ludington E, Dawson JD, Patterson JE, Rangel-Frausto S, Wiblin RT, et al. National epidemiology of mycoses study, risk factors for Candida species colonization of neonatal intensive care unit patients. Pediatr Infect Dis J. (2001) 20:1119–24. doi: 10.1097/00006454-200112000-00005
16. Kuppala VS, Meinzen-Derr J, Morrow AL, Schibler KR. Prolonged initial empirical antibiotic treatment is associated with adverse outcomes in premature infants. J Pediatr. (2011) 159:720–5. doi: 10.1016/j.jpeds.2011.05.033
17. Cotten CM, McDonald S, Stoll B, Goldberg RN, Poole K, Benjamin DK, et al. National institute for child, and N human development neonatal research, the association of third-generation cephalosporin use and invasive candidiasis in extremely low birth-weight infants. Pediatrics. (2006) 118:717–22. doi: 10.1542/peds.2005-2677
18. Cotten CM, Taylor S, Stoll B, Goldberg RN, Hansen NI, Sanchez PJ, et al. Prolonged duration of initial empirical antibiotic treatment is associated with increased rates of necrotizing enterocolitis and death for extremely low birth weight infants. Pediatrics. (2009) 123:58–66. doi: 10.1542/peds.2007-3423
19. Neu J, Douglas-Escobar M, Lopez M. Microbes and the developing gastrointestinal tract. Nutr Clin Pract. (2007) 22:174–82. doi: 10.1177/0115426507022002174
20. Bryan CS, John JF Jr., Pai MS, Austin TL. Gentamicin vs cefotaxime for therapy of neonatal sepsis Relationship to drug resistance. Am J Dis Child. (1985) 139:1086–9. doi: 10.1001/archpedi.1985.02140130024022
21. de Man P, Verhoeven BA, Verbrugh HA, Vos MC, van den Anker JN. An antibiotic policy to prevent emergence of resistant bacilli. Lancet. (2000) 355:973–8. doi: 10.1016/S0140-6736(00)90015-1
22. Rubinstein E, Keynan Y. Vancomycin-resistant enterococci. Crit Care Clin. (2013) 29:841–52. doi: 10.1016/j.ccc.2013.06.006
23. Patel G, Huprikar S, Factor SH, Jenkins SG, Calfee DP. Outcomes of carbapenem-resistant Klebsiella pneumoniae infection and the impact of antimicrobial and adjunctive therapies. Infect Control Hosp Epidemiol. (2008) 29:1099–106. doi: 10.1086/592412
24. Hussein K, Sprecher H, Mashiach T, Oren I, Kassis I, Finkelstein R. Carbapenem resistance among Klebsiella pneumoniae isolates: risk factors, molecular characteristics, susceptibility patterns. Infect Control Hosp Epidemiol. (2009) 30:666–71. doi: 10.1086/598244
25. Benjamin DK. Jr., Stoll BJ, Gantz MG, Walsh MC, Sanchez PJ, et al. Eunice Kennedy Shriver National Institute of Child, and N Human Development Neonatal Research, Neonatal candidiasis: epidemiology, risk factors, clinical judgment. Pediatrics. (2010) 126:e865–73. doi: 10.1542/peds.2009-3412
26. Ting JY, Roberts A, Sherlock R, Ojah C, Cieslak Z, Dunn M, et al. Canadian neonatal network, duration of initial empirical antibiotic therapy and outcomes in very low birth weight infants. Pediatrics. (2019) 143:e20182286. doi: 10.1542/peds.2018-2286
27. Ting JY, Synnes A, Roberts A, Deshpandey A, Dow K, Yoon EW, et al. for the Canadian neonatal network, association between antibiotic use and neonatal mortality and morbidities in very low-birth-weight infants without culture-proven sepsis or necrotizing enterocolitis. JAMA Pediatr. (2016) 170:1181–7. doi: 10.1001/jamapediatrics.2016.2132
28. Ting JY, Synnes A, Roberts A, Deshpandey AC, Dow K, Yang J, et al. Canadian neonatal follow-up, association of antibiotic utilization and neurodevelopmental outcomes among extremely low gestational age neonates without proven sepsis or necrotizing enterocolitis. Am J Perinatol. (2018) 35:972–8. doi: 10.1055/s-0038-1632390
29. Dellit TH, Owens RC, McGowan JE. Jr., Gerding DN, Weinstein RA, et al. Infectious Diseases Society of, and A Society for Healthcare Epidemiology of, Infectious Diseases Society of America and the Society for Healthcare Epidemiology of America guidelines for developing an institutional program to enhance antimicrobial stewardship. Clin Infect Dis. (2007) 44:159–77. doi: 10.1086/510393
30. Patel SJ, Saiman L. Antibiotic resistance in neonatal intensive care unit pathogens: mechanisms, clinical impact, and prevention including antibiotic stewardship. Clin Perinatol. (2010) 37:547–63. doi: 10.1016/j.clp.2010.06.004
31. Johnson CL, Saiman L. A blueprint for targeted antimicrobial stewardship in neonatal intensive care units. Infect Control Hosp Epidemiol. (2017) 38:1144–6. doi: 10.1017/ice.2017.183
32. Ting JY, Shah PS. Antibiotic stewardship in neonates: challenges and opportunities. Transl Pediatr. (2020) 9:198–201. doi: 10.21037/tp-20-134
33. Stoll BJ, Hansen NI, Adams-Chapman I, Fanaroff AA, Hintz SR, Vohr B, et al. Neurodevelopmental and growth impairment among extremely low-birth-weight infants with neonatal infection. Jama. (2004) 292:2357–65. doi: 10.1001/jama.292.19.2357
34. Kuzniewicz MW, Puopolo KM. Antibiotic stewardship for early-onset sepsis. Semin Perinatol. (2020) 44:151325. doi: 10.1016/j.semperi.2020.151325
35. Canadian Neonatal Network Abstracors' Manual. Available online at: http://www.canadianneonatalnetwork.org/Portal/LinkClick.aspx?fileticket=krvGeUTtLck%3d&tabid=69 2015 (accessed March 10, 2022).
36. Phares CR, Lynfield R, Farley MM, Mohle-Boetani J, Harrison LH, Petit S, et al. Active bacterial core surveillance/emerging infections program, epidemiology of invasive group B streptococcal disease in the United States, 1999-2005. JAMA. (2008) 299:2056–65. doi: 10.1001/jama.299.17.2056
37. Flannery DD, Ross RK, Mukhopadhyay S, Tribble AC, Puopolo KM, Gerber JS. Temporal trends and center variation in early antibiotic use among premature infants. JAMA Netw Open. (2018) 1:e180164. doi: 10.1001/jamanetworkopen.2018.0164
38. Simonsen KA, Anderson-Berry AL, Delair SF, Davies HD. Early-onset neonatal sepsis. Clin Microbiol Rev. (2014) 27:21–47. doi: 10.1128/CMR.00031-13
39. Puopolo KM, Benitz WE, Zaoutis TE, Committee Committee On F, Newborn D. Committee on infectious, management of neonates born at < /=34 6/7 weeks' gestation with suspected or proven early-onset bacterial sepsis. Pediatrics. (2018) 142:e20182896. doi: 10.1542/peds.2018-2896
40. Baltimore RS, Huie SM, Meek JI, Schuchat A, O'Brien KL. Early-onset neonatal sepsis in the era of group B streptococcal prevention. Pediatrics. (2001) 108:1094–8. doi: 10.1542/peds.108.5.1094
41. Singh T, Barnes EH, Isaacs D, Australian Study Group for Neonatal. Early-onset neonatal infections in Australia and New Zealand, 2002-2012. Arch Dis Child Fetal Neonatal Ed. (2019) 104:F248–52. doi: 10.1136/archdischild-2017-314671
42. Braye K, Foureur M, de Waal K, Jones M, Putt E, Ferguson J. Epidemiology of neonatal early-onset sepsis in a geographically diverse Australian health district 2006-2016. PloS one. (2019) 14:e0214298. doi: 10.1371/journal.pone.0214298
43. Money D, Allen VM, Infectious Diseases Committee. The prevention of early-onset neonatal group B streptococcal disease. J Obstet Gynaecol Can. (2013) 35:939–48. doi: 10.1016/S1701-2163(15)30818-5
44. Nanduri SA, Petit S, Smelser C, Apostol M, Alden NB, Harrison LH, et al. Epidemiology of invasive early-onset and late-onset Group B streptococcal disease in the United States, 2006 to 2015: multistate laboratory and population-based surveillance. JAMA Pediatr. (2019) 173:224–33. doi: 10.1001/jamapediatrics.2018.4826
45. Stoll BJ, Puopolo KM, Hansen NI, Sanchez PJ, Bell EF, Carlo WA, et al. Early-onset neonatal sepsis 2015 to 2017, the rise of escherichia coli, and the need for novel prevention strategies. JAMA Pediatr. (2020) 174:e200593. doi: 10.1001/jamapediatrics.2020.0593
46. Stoll BJ, Hansen NI, Bell EF, Walsh MC, Carlo WA, Shankaran S, et al. Trends in care practices, morbidity, and mortality of extremely preterm neonates, 1993-2012. Jama. (2015) 314:1039–51. doi: 10.1001/jama.2015.10244
47. Canadian Neonatal Network Annual Report Review Committee 2018 Annual Report. Available online at: http://www.canadianneonatalnetwork.org/Portal/LinkClick.aspx?fileticket=PvniYH94zm0%3d&tabid=39 (accessed March 10, 2022).
48. Canadian Neonatal Network Annual Report Review, Committee, 2017 Annual, Report,. Available online at: http://www.canadianneonatalnetwork.org/Portal/LinkClick.aspx?fileticket=XhPMIxFgc2M%3d&tabid=39 (accessed March 10, 2022).
49. Goldenberg RL, Culhane JF, Iams JD, Romero R. Epidemiology and causes of preterm birth. Lancet. (2008) 371:75–84. doi: 10.1016/S0140-6736(08)60074-4
50. Puopolo KM, Benitz WE, Zaoutis TE, Committee Committee On F, Newborn D. Committee on infectious, management of neonates born at >/=35 0/7 weeks' gestation with suspected or proven early-onset bacterial sepsis. Pediatrics. (2018) 142:20182894. doi: 10.1542/peds.2018-2894
51. Garcia-Prats JA, Cooper TR, Schneider VF, Stager CE, Hansen TN. Rapid detection of microorganisms in blood cultures of newborn infants utilizing an automated blood culture system. Pediatrics. (2000) 105:523–7. doi: 10.1542/peds.105.3.523
52. Jardine L, Davies MW, Faoagali J. Incubation time required for neonatal blood cultures to become positive. J Paediatr Child Health. (2006) 42:797–802. doi: 10.1111/j.1440-1754.2006.00980.x
53. Oeser C, Pond M, Butcher P, Bedford Russell A, Henneke P, Laing K, et al. PCR for the detection of pathogens in neonatal early onset sepsis. PLoS ONE. (2020) 15:e0226817. doi: 10.1371/journal.pone.0226817
54. Pammi M, Flores A, Versalovic J, Leeflang MM. Molecular assays for the diagnosis of sepsis in neonates. Cochrane Database Syst Rev. (2017) 2:CD011926. doi: 10.1002/14651858.CD011926.pub2
55. Hornik CP, Benjamin DK, Becker KC, Benjamin DK Jr., Li J, et al. Use of the complete blood cell count in early-onset neonatal sepsis. Pediatr Infect Dis J. (2012) 31:799–802. doi: 10.1097/INF.0b013e318256905c
56. Newman TB, Puopolo KM Wi S, Draper D, Escobar GJ. Interpreting complete blood counts soon after birth in newborns at risk for sepsis. Pediatrics. (2010) 126:903–9. doi: 10.1542/peds.2010-0935
57. Greenberg DN, Yoder BA. Changes in the differential white blood cell count in screening for group B streptococcal sepsis. Pediatr Infect Dis J. (1990) 9:886–9. doi: 10.1097/00006454-199012000-00006
58. Rozycki HJ, Stahl GE, Baumgart S. Impaired sensitivity of a single early leukocyte count in screening for neonatal sepsis. Pediatr Infect Dis J. (1987) 6:440–2. doi: 10.1097/00006454-198705000-00004
59. Polin RA, Committee on Fetus and Newborn. Management of neonates with suspected or proven early-onset bacterial sepsis. Pediatrics. (2012) 129:1006–15. doi: 10.1542/peds.2012-0541
60. Benitz WE, Han MY, Madan A, Ramachandra P. Serial serum C-reactive protein levels in the diagnosis of neonatal infection. Pediatrics. (1998) 102:E41. doi: 10.1542/peds.102.4.e41
61. Benitz WE. Adjunct laboratory tests in the diagnosis of early-onset neonatal sepsis. Clin Perinatol. (2010) 37:421–38. doi: 10.1016/j.clp.2009.12.001
62. Stocker M, van Herk W, El Helou S, Dutta S, Schuerman F, van den Tooren-de Groot RK, et al. C-reactive protein, procalcitonin, and white blood count to rule out neonatal early-onset sepsis within 36 hours: a secondary analysis of the neonatal procalcitonin intervention study. Clin Infect Dis. (2021) 73:e383–90. doi: 10.1093/cid/ciaa876
63. Stocker M, van Herk W, El Helou S, Dutta S, Fontana MS, Schuerman F, et al. Procalcitonin-guided decision making for duration of antibiotic therapy in neonates with suspected early-onset sepsis: a multicentre, randomised controlled trial (NeoPIns). Lancet. (2017) 390:871–881. doi: 10.1016/S0140-6736(17)31444-7
64. Dongen ORE, van Leeuwen LM, de Groot PK, Vollebregt K, Schiering I, Wevers BA, et al. Umbilical cord procalcitonin to detect early-onset sepsis in newborns: a promising biomarker. Front Pediatr. (2021) 9:779663. doi: 10.3389/fped.2021.779663
65. A.R. Bedford Russell, Kumar R. Early onset neonatal sepsis: diagnostic dilemmas and practical management. Arch Dis Child Fetal Neonatal Ed. (2015) 100:F350–4. doi: 10.1136/archdischild-2014-306193
66. Koizumi Y, Sakanashi D, Ohno T, Nakamura A, Yamada A, Shibata Y, et al. Plasma procalcitonin levels remain low at the onset of gram-positive bacteremia regardless of severity or the presence of shock: a retrospective analysis of patients with detailed clinical characteristics. J Microbiol Immunol Infect. (2021) 54:1028–37. doi: 10.1016/j.jmii.2020.08.015
67. Riskin A, Toropine A, Bader D, Hemo M, Srugo I, Kugelman A. Is it justified to include urine cultures in early (<72 hours) neonatal sepsis evaluations of term and late preterm infants? Am J Perinatol. (2013) 30:499–504. doi: 10.1055/s-0032-1329180
68. Tamim MM, Alesseh H, Aziz H. Analysis of the efficacy of urine culture as part of sepsis evaluation in the premature infant. Pediatr Infect Dis J. (2003) 22:805–8. doi: 10.1097/01.inf.0000083822.31857.43
69. Garges HP, Moody MA, Cotten CM, Smith PB, Tiffany KF, Lenfestey R, et al. Neonatal meningitis: what is the correlation among cerebrospinal fluid cultures, blood cultures, and cerebrospinal fluid parameters? Pediatrics. (2006) 117:1094–100. doi: 10.1542/peds.2005-1132
70. Verani JR, McGee L, Schrag SJ. Prevention of perinatal group B streptococcal disease–revised guidelines from CDC, 2010. MMWR Recomm Rep. (2010) 59:1–36.
71. Kuzniewicz MW, Puopolo KM, Fischer A, Walsh EM Li S, Newman TB, et al. A quantitative, risk-based approach to the management of neonatal early-onset sepsis. JAMA Pediatr. (2017) 171:365–71. doi: 10.1001/jamapediatrics.2016.4678
72. Cantoni L, Ronfani L, Da Riol R, Demarini S, Perinatal Perinatal Study Group of the Region Friuli-Venezia Giulia. Physical examination instead of laboratory tests for most infants born to mothers colonized with group B Streptococcus: support for the Centers for Disease Control and Prevention's 2010 recommendations. J Pediatr. (2013) 163:568–73. doi: 10.1016/j.jpeds.2013.01.034
73. Jefferies AL. Management of term infants at increased risk for early-onset bacterial sepsis. Paediatr Child Health. (2017) 22:223–8. doi: 10.1093/pch/pxx023
74. Kalathia MB, Shingala PA, Parmar PN, Parikh YN, Kalathia IM. Study of Umbilical Cord Blood Culture in Diagnosis of Early-onset Sepsis Among Newborns with High-risk Factors. J Clin Neonatol. (2013) 2:169–72. doi: 10.4103/2249-4847.123092
75. Quinones Cardona V, Lowery V, Cooperberg D, Anday EK, Carey AJ. Eliminating contamination in umbilical cord blood culture sampling for early-onset neonatal sepsis. Front Pediatr. (2021) 9:794710. doi: 10.3389/fped.2021.794710
76. Mukhopadhyay S, Puopolo KM. Clinical and microbiologic characteristics of early-onset sepsis among very low birth weight infants: opportunities for antibiotic stewardship. Pediatr Infect Dis J. (2017) 36:477–81. doi: 10.1097/INF.0000000000001473
77. Puopolo KM, Draper D, Wi S, Newman TB, Zupancic J, Lieberman E, et al. Estimating the probability of neonatal early-onset infection on the basis of maternal risk factors. Pediatrics. (2011) 128:e1155–63. doi: 10.1542/peds.2010-3464
78. Garber SJ, Dhudasia MB, Flannery DD, Passarella MR, Puopolo KM, Mukhopadhyay S. Delivery-based criteria for empiric antibiotic administration among preterm infants. J Perinatol. (2021) 41:255–62. doi: 10.1038/s41372-020-00784-y
79. Morowitz M. NICU Antibiotics and Outcomes Trial (NANO), 2019. Available online at: https://clinicaltrials.gov/ct2/show/record/NCT03997266 (accessed March 10, 2022).
80. Woodford EC, Dhudasia MB, Puopolo KM, Skerritt LA, Bhavsar M, DeLuca J, et al. Neonatal blood culture inoculant volume: feasibility and challenges. Pediatr Res. (2021) 90:1086–92. doi: 10.1038/s41390-021-01484-9
81. Connell TG, Rele M, Cowley D, Buttery JP, Curtis N. How reliable is a negative blood culture result? Volume of blood submitted for culture in routine practice in a children's hospital. Pediatrics. (2007) 119:891–6. doi: 10.1542/peds.2006-0440
82. Lancaster DP, Friedman DF, Chiotos K, Sullivan KV. Blood volume required for detection of low levels and ultralow levels of organisms responsible for neonatal bacteremia by use of Bactec Peds Plus/F, Plus Aerobic/F Medium, and the BD Bactec FX system: an in vitro study. J Clin Microbiol. (2015) 53:3609–13. doi: 10.1128/JCM.01706-15
83. Singh MP, Balegar VK, Angiti RR. The practice of blood volume submitted for culture in a neonatal intensive care unit. Arch Dis Child Fetal Neonatal Ed. (2020) 105:600–4. doi: 10.1136/archdischild-2019-318080
84. Lam HS, Chan KY, Ip M, Leung KT, Lo NW, Wong RP, et al. Rapid identification of bacterial antibiotic resistance by qPCR in infants with gram-negative septicaemia: a proof-of-concept study. Neonatology. (2017) 111:145–52. doi: 10.1159/000449279
85. Muller-Pebody B, Johnson AP, Heath PT, Gilbert RE, Henderson KL, Sharland M. Empirical Empirical treatment of neonatal sepsis: are the current guidelines adequate? Arch Dis Child Fetal Neonatal Ed. (2011) 96:F4–8. doi: 10.1136/adc.2009.178483
86. Arboleya S, Sanchez B, Milani C, Duranti S, Solis G, Fernandez N, et al. Intestinal microbiota development in preterm neonates and effect of perinatal antibiotics. J Pediatr. (2015) 166:538–44. doi: 10.1016/j.jpeds.2014.09.041
87. Fouhy F, Guinane CM, Hussey S, Wall R, Ryan CA, Dempsey EM, et al. High-throughput sequencing reveals the incomplete, short-term recovery of infant gut microbiota following parenteral antibiotic treatment with ampicillin and gentamicin. Antimicrob Agents Chemother. (2012) 56:5811–20. doi: 10.1128/AAC.00789-12
88. Oosterloo BC, van Elburg RM, Rutten NB, Bunkers CM, Crijns CE, Meijssen CB, et al. Wheezing and infantile colic are associated with neonatal antibiotic treatment. Pediatr Allergy Immunol. (2018) 29:151–8. doi: 10.1111/pai.12857
89. Bailey LC, Forrest CB, Zhang P, Richards TM, Livshits A, DeRusso PA. Association of antibiotics in infancy with early childhood obesity. JAMA Pediatr. (2014) 168:1063–9. doi: 10.1001/jamapediatrics.2014.1539
90. Modi N, Damjanovic V, Cooke RW. Outbreak of cephalosporin resistant Enterobacter cloacae infection in a neonatal intensive care unit. Arch Dis Child. (1987) 62:148–51. doi: 10.1136/adc.62.2.148
91. Le J, Nguyen T, Okamoto M, McKamy S, Lieberman JM. Impact of empiric antibiotic use on development of infections caused by extended-spectrum β-Lactamase bacteria in a neonatal intensive care unit. Pediatr Infect Dis J. (2008) 27:314–8. doi: 10.1097/INF.0b013e3181606850
92. National Institute for Health Clinical Excellence, Antibiotics for early-onset neonatal infection. NICE Clinical Guideline 195. Available online at: https://www.nice.org.uk/guidance/ng195/chapter/Recommendations#duration-of-antibiotic-treatment-for-early-onset-neonatal-infection (accessed April 20, 2021).
93. Krisher KK, Gibb P, Corbett S, Church D. Comparison of the BacT/Alert PF pediatric FAN blood culture bottle with the standard pediatric blood culture bottle, the Pedi-BacT. J Clin Microbiol. (2001) 39:2880–3. doi: 10.1128/JCM.39.8.2880-2883.2001
94. Sarkar SS, Bhagat I, Bhatt-Mehta V, Sarkar S. Does maternal intrapartum antibiotic treatment prolong the incubation time required for blood cultures to become positive for infants with early-onset sepsis? Am J Perinatol. (2015) 32:357–62. doi: 10.1055/s-0034-1387933
95. Shane AL, Stoll BJ. Recent developments and current issues in the epidemiology, diagnosis, and management of bacterial and fungal neonatal sepsis. Am J Perinatol. (2013) 30:131–41. doi: 10.1055/s-0032-1333413
96. Sivanandan S, Soraisham AS, Swarnam K. Choice and duration of antimicrobial therapy for neonatal sepsis and meningitis. Int J Pediatr. (2011) 2011:712150. doi: 10.1155/2011/712150
97. Gathwala G, Sindwani A, Singh J, Choudhry O, Chaudhary U. Ten days vs. 14 days antibiotic therapy in culture-proven neonatal sepsis. J Trop Pediatr. (2010) 56:433–5. doi: 10.1093/tropej/fmq012
98. Chowdhary G, Dutta S, Narang A. Randomized controlled trial of 7-day vs. 14-day antibiotics for neonatal sepsis. J Trop Pediatr. (2006) 52:427–32. doi: 10.1093/tropej/fml054
99. Dear P. Neonatal infection. In: Rennie JM, editor. Roberton's Textbook of Neonatology. London: Churchill Livingstone (2005). p. 1011–92.
100. Coon ER, Srivastava R, Stoddard G, Wilkes J, Pavia AT, Shah SS. Shortened IV antibiotic course for uncomplicated, late-onset group B streptococcal bacteremia. Pediatrics. (2018) 142:e20180345. doi: 10.1542/peds.2018-0345
101. Report of the Committee on Infectious Diseases. Serious bacterial infections casused by Enterobacteriaceae. In: Byington CL, editor Redbook. Itasca, IL: American Academy of Pediatrics (2018). p. 328–31.
102. Verani JR, McGee L, Schrag Schrag SJ; Division of Bacterial Diseases National Center for Immunization and Respiratory Diseases Centers for Disease Control and Prevention (CDC). Prevention of perinatal group B streptococcal disease–revised guidelines from CDC, 2010. MMWR Recomm rep. (2010) 59:1–36.
103. Money DM, Dobson S, IDC Canadian Paediatric Society. The prevention of early-onset neonatal group B streptococcal disease. J Obstet Gynaecol Can. (2004) 26:826–40. doi: 10.1016/S1701-2163(16)30157-8
104. Trijbels-Smeulders M, de Jonge GA, Pasker-de Jong PC, Gerards LJ, Adriaanse AH, van Lingen RA, et al. Epidemiology of neonatal group B streptococcal disease in the Netherlands before and after introduction of guidelines for prevention. Arch Dis Child Fetal Neonatal Ed. (2007) 92:F271–6. doi: 10.1136/adc.2005.088799
105. Li QY, Wang DY Li HT, Liu JM. Screening-based and risk-based strategy for the prevention of early-onset group B streptococcus/non-group B streptococcus sepsis in the neonate: a systematic review and meta-analysis. Pediatr Infect Dis J. (2020) 39:740–8. doi: 10.1097/INF.0000000000002674
106. Money D, Allen VM. No. 298-the prevention of early-onset neonatal group B streptococcal disease. J Obstet Gynaecol Can. (2018) 40:e665–74. doi: 10.1016/j.jogc.2018.05.032
107. Stoll BJ, Hansen N, Fanaroff AA, Wright LL, Carlo WA, Ehrenkranz RA, et al. Changes in pathogens causing early-onset sepsis in very-low-birth-weight infants. N Engl J Med. (2002) 347:240–7. doi: 10.1056/NEJMoa012657
108. Vergnano S, Menson E, Kennea N, Embleton N, Russell AB, Watts T, et al. Neonatal infections in England: the NeonIN surveillance network. Arch Dis Child Fetal Neonatal Ed. (2011) 96:F9–F14. doi: 10.1136/adc.2009.178798
109. Bizzarro MJ, Raskind C, Baltimore RS, Gallagher PG. Seventy-five years of neonatal sepsis at Yale: 1928–2003. Pediatrics. (2005) 116:595. doi: 10.1542/peds.2005-0552
110. Benjamin DK, Miller W, Garges H, Benjamin DK, McKinney RE, Cotton M, et al. Central Catheters, and Neonates: when to pull the line. Pediatrics. (2001) 107:1272. doi: 10.1542/peds.107.6.1272
111. Shane AL, Sánchez PJ, Stoll BJ. Neonatal sepsis. Lancet. (2017) 390:1770–80. doi: 10.1016/S0140-6736(17)31002-4
112. Sewell E, Roberts J, Mukhopadhyay S. Association of infection in neonates and long-term neurodevelopmental outcome. Clin Perinatol. (2021) 48:251–61. doi: 10.1016/j.clp.2021.03.001
113. El Manouni El Hassani S, Berkhout DJC, Niemarkt HJ, Mann S, de Boode WP, Cossey V, et al. Risk factors for late-onset sepsis in preterm infants: a multicenter case-control study. Neonatology. (2019) 116:42–51. doi: 10.1159/000497781
114. Sohn AH, Garrett DO, Sinkowitz-Cochran RL, Grohskopf LA, Levine GL, Stover BH, et al. Pediatric prevention, prevalence of nosocomial infections in neonatal intensive care unit patients: Results from the first national point-prevalence survey. J Pediatr. (2001) 139:821–7. doi: 10.1067/mpd.2001.119442
115. Garber SJ, Puopolo KM. Prevention of central line–associated bloodstream infections among infants in the neonatal intensive care unit. Neoreviews. (2015) 16:e211–20. doi: 10.1542/neo.16-4-e211
116. Greenberg RG, Kandefer S, Do BT, Smith PB, Stoll BJ, Bell EF, et al. Late-onset sepsis in extremely premature infants: 2000-2011. Pediatr Infect Dis J. (2017) 36:774–9. doi: 10.1097/INF.0000000000001570
117. Ting JY, Roberts A, Synnes A, Canning R, Bodani J, Monterossa L, et al. Invasive fungal infections in Neonates in Canada: epidemiology and outcomes. Pediatr Infect Dis J. (2018) 37:1154–9. doi: 10.1097/INF.0000000000001968
118. Schelonka RL, Chai MK, Yoder BA, Hensley D, Brockett RM, Ascher DP. Volume of blood required to detect common neonatal pathogens. J Pediatr. (1996) 129:275–8. doi: 10.1016/S0022-3476(96)70254-8
119. Wagstaff JS, Durrant RJ, Newman MG, Eason R, Ward RM, Sherwin CMT, et al. Antibiotic treatment of suspected and confirmed neonatal sepsis within 28 days of birth: a retrospective analysis. Front Pharmacol. (2019) 10:1191. doi: 10.3389/fphar.2019.01191
120. Mermel LA, Allon M, Bouza E, Craven DE, Flynn P, O'Grady NP, et al. Clinical practice guidelines for the diagnosis and management of intravascular catheter-related infection: 2009 Update by the Infectious Diseases Society of America. Clin Infect Dis. (2009) 49:1–45. doi: 10.1086/599376
121. Greenberg RG, Smith PB, Cotten CM, Moody MA, Clark RH, Benjamin DK Jr. Traumatic lumbar punctures in neonates: test performance of the cerebrospinal fluid white blood cell count. Pediatr Infect Dis J. (2008) 27:1047–51. doi: 10.1097/INF.0b013e31817e519b
122. Ting JY, Roberts A, Khan S, Bitnun A, Hawkes M, Barton M, et al. Predictive value of repeated cerebrospinal fluid parameters in the outcomes of bacterial meningitis in infants <90 days of age. PLoS ONE. (2020) 15:e0238056. doi: 10.1371/journal.pone.0238056
123. P.Y. Iroh Tam, Bendel CM. Diagnostics for neonatal sepsis: current approaches and future directions. Pediatr Res. (2017) 82:574–583. doi: 10.1038/pr.2017.134
124. Bhandari V. Effective biomarkers for diagnosis of neonatal sepsis. J Pediatric Infect Dis Soc. (2014) 3:234–45. doi: 10.1093/jpids/piu063
125. Sharma D, Farahbakhsh N, Shastri S, Sharma P. Biomarkers for diagnosis of neonatal sepsis: a literature review. J Matern Fetal Neonatal Med. (2018) 31:1646–59. doi: 10.1080/14767058.2017.1322060
126. Dong Y, Speer CP. Late-onset neonatal sepsis: recent developments. Arch Dis Child Fetal Neonatal Ed. (2015) 100:F257–63. doi: 10.1136/archdischild-2014-306213
127. Cantey JB, Wozniak PS, Pruszynski JE, Sanchez PJ. Reducing unnecessary antibiotic use in the neonatal intensive care unit (SCOUT): a prospective interrupted time-series study. Lancet Infect Dis. (2016) 16:1178–84. doi: 10.1016/S1473-3099(16)30205-5
128. Ericson JE, Thaden J, Cross HR, Clark RH, Fowler VG. Jr., Cohen-Wolkowiez M, et al. Antibacterial resistance leadership, no survival benefit with empirical vancomycin therapy for coagulase-negative staphylococcal bloodstream infections in infants. Pediatr Infect Dis J. (2015) 34:371–5. doi: 10.1097/INF.0000000000000573
129. Tripathi N, Cotten CM, Smith PB. Antibiotic use and misuse in the neonatal intensive care unit. Clin Perinatol. (2012) 39:61–8. doi: 10.1016/j.clp.2011.12.003
130. J. van den Anker, Allegaert K. Rational use of antibiotics in neonates: still in search of tailored tools. Healthcare. (2019) 7:28. doi: 10.3390/healthcare7010028
131. Tsai MH, Hsu JF, Lien R, Huang HR, Chiang CC, Chu SM, et al. Catheter management in neonates with bloodstream infection and a percutaneously inserted central venous catheter in situ: removal or not? Am J Infect Control. (2012) 40:59–64. doi: 10.1016/j.ajic.2011.04.051
132. W.s. National Collaborating Centre for, Children's H. National Institute for Health and Clinical Excellence. Guidance, Antibiotics for Early-Onset Neonatal Infection: Antibiotics for the Prevention and Treatment of Early-Onset Neonatal Infection. London: RCOG Press Copyright © 2012, National Collaborating Centre for Women's and Children's Health (2012).
133. Tunkel AR, Hartman BJ, Kaplan SL, Kaufman BA, Roos KL, Scheld WM, et al. Practice guidelines for the management of bacterial meningitis. Clin Infect Dis. (2004) 39:1267–84. doi: 10.1086/425368
134. Cantey JB, Baird SD. Ending the culture of culture-negative sepsis in the neonatal ICU. Pediatrics. (2017) 140:e20170044. doi: 10.1542/peds.2017-0044
135. Fjalstad JW, Stensvold HJ, Bergseng H, Simonsen GS, Salvesen B, Ronnestad AE, et al. Early-onset sepsis and antibiotic exposure in term infants: a nationwide population-based study in Norway. Pediatr Infect Dis J. (2016) 35:1–6. doi: 10.1097/INF.0000000000000906
136. Cotten CM. Adverse consequences of neonatal antibiotic exposure. Curr Opin Pediatr. (2016) 28:141–9. doi: 10.1097/MOP.0000000000000338
137. Tzialla C, Borghesi A, Serra G, Stronati M, Corsello G. Antimicrobial therapy in neonatal intensive care unit. Ital J Pediatr. (2015) 41:27. doi: 10.1186/s13052-015-0117-7
138. Fjalstad JW, Esaiassen E, Juvet LK, van den Anker JN, Klingenberg C. Antibiotic therapy in neonates and impact on gut microbiota and antibiotic resistance development: a systematic review. J Antimicrob Chemother. (2018) 73:569–80. doi: 10.1093/jac/dkx426
139. Apisarnthanarak A, Holzmann-Pazgal G, Hamvas A, Olsen MA, Fraser VJ. Ventilator-associated pneumonia in extremely preterm neonates in a neonatal intensive care unit: characteristics, risk factors, and outcomes. Pediatrics. (2003) 112:1283–9. doi: 10.1542/peds.112.6.1283
140. Cernada M, Aguar M, Brugada M, Gutiérrez A, López JL, Castell M, et al. Ventilator-associated pneumonia in newborn infants diagnosed with an invasive bronchoalveolar lavage technique: a prospective observational study*. Pediatr Crit Care Med. (2013) 14:55–61. doi: 10.1097/PCC.0b013e318253ca31
141. Rosenthal VD, Bijie H, Maki DG, Mehta Y, Apisarnthanarak A, Medeiros EA, et al. International Nosocomial Infection Control Consortium (INICC) report, data summary of 36 countries, for 2004-2009. Am J Infect Control. (2012) 40:396–407. doi: 10.1016/j.ajic.2011.05.020
142. Ericson JE, McGuire J, Michaels MG, Schwarz A, Frenck R, Deville JG, et al. Hospital-acquired pneumonia and ventilator-associated pneumonia in children: a prospective natural history and case-control study. Pediatr Infect Dis J. (2020) 39:658–64. doi: 10.1097/INF.0000000000002642
143. Kenaa B, Richert ME, Claeys KC, Shipper A, Sullivan KV, Schrank GM, et al. Ventilator-associated pneumonia: diagnostic test stewardship and relevance of culturing practices. Curr Infect Dis Rep. (2019) 21:50. doi: 10.1007/s11908-019-0708-3
144. Sick-Samuels AC, Fackler JC, Berenholtz SM, Milstone AM. Understanding reasons clinicians obtained endotracheal aspirate cultures and impact on patient management to inform diagnostic stewardship initiatives. Infect Control Hosp Epidemiol. (2020) 41:240–2. doi: 10.1017/ice.2019.347
145. Willson DF, Kirby A, Kicker JS. Respiratory secretion analyses in the evaluation of ventilator-associated pneumonia: a survey of current practice in pediatric critical care. Pediatr Crit Care Med. (2014) 15:715–9. doi: 10.1097/PCC.0000000000000213
146. Willson DF, Conaway M, Kelly R, Hendley JO. The lack of specificity of tracheal aspirates in the diagnosis of pulmonary infection in intubated children. Pediatr Crit Care Med. (2014) 15:299–305. doi: 10.1097/PCC.0000000000000106
147. Ergenekon E, Çataltepe S. Ventilator-associated pneumonia in the NICU: time to boost diagnostics? Pediatr Res. (2020) 87:1143–4. doi: 10.1038/s41390-019-0672-5
148. Goerens A, Lehnick D, Büttcher M, Daetwyler K, Fontana M, Genet P, et al. Neonatal ventilator associated pneumonia: a quality improvement initiative focusing on antimicrobial stewardship. Front Pediatr. (2018) 6:262. doi: 10.3389/fped.2018.00262
149. Cocoros NM, Kleinman K, Priebe GP, Gray JE, Logan LK, Larsen G, et al. Pediatric ventilator-associated conditions study, ventilator-associated events in neonates and children–a new paradigm. Crit Care Med. (2016) 44:14–22. doi: 10.1097/CCM.0000000000001372
150. Guess R, Vaewpanich J, Coss-Bu JA, Phongjitsiri S, Kennedy C, Starke J, et al. Risk factors for ventilator-associated events in a PICU. Pediatr Crit Care Med. (2018) 19:e7–e13. doi: 10.1097/PCC.0000000000001371
151. Iosifidis E, Stabouli S, Tsolaki A, Sigounas V, Panagiotidou E-B, Sdougka M, et al. Diagnosing ventilator-associated pneumonia in pediatric intensive care. Am J Infect Control. (2015) 43:390–3. doi: 10.1016/j.ajic.2015.01.004
152. B. Jacobs Pepin, Lesslie D, Berg W, Spaulding AB, Pokora T. ZAP-VAP: A Quality improvement initiative to decrease ventilator-associated pneumonia in the neonatal intensive care unit, 2012-2016. Adv Neonatal Care. (2019) 19:253–61. doi: 10.1097/ANC.0000000000000635
153. Azab SF, Sherbiny HS, Saleh SH, Elsaeed WF, Elshafiey MM, Siam AG, et al. Reducing ventilator-associated pneumonia in neonatal intensive care unit using “VAP prevention Bundle”: a cohort study. BMC Infect Dis. (2015) 15:314. doi: 10.1186/s12879-015-1062-1
154. Gokce IK, Kutman HGK, Uras N, Canpolat FE, Dursun Y, Oguz SS. Successful implementation of a bundle strategy to prevent ventilator-associated pneumonia in a neonatal intensive care unit. J Trop Pediatr. (2018) 64:183–8. doi: 10.1093/tropej/fmx044
155. Rogers E, Alderdice F, McCall E, Jenkins J, Craig S. Reducing nosocomial infections in neonatal intensive care. J Matern Fetal Neonatal Med. (2010) 23:1039–46. doi: 10.3109/14767050903387029
156. Weber CD. Applying adult ventilator-associated pneumonia bundle evidence to the ventilated neonate. Adv Neonatal Care. (2016) 16:178–90. doi: 10.1097/ANC.0000000000000276
157. Niedzwiecka T, Patton D, Walsh S, Moore Z, O'Connor T, Nugent L. What are the effects of care bundles on the incidence of ventilator-associated pneumonia in paediatric and neonatal intensive care units? A systematic review. J Spec Pediatr Nurs. (2019) 24:e12264. doi: 10.1111/jspn.12264
158. Dell'Orto V, Bourgeois-Nicolaos N, Rouard C, Romain O, Shankar-Aguilera S, Doucet-Populaire F, et al. Cell count analysis from nonbronchoscopic bronchoalveolar lavage in preterm infants. J Pediatr. (2018) 200:30–7.e2. doi: 10.1016/j.jpeds.2018.04.074
159. Cordero L, Sananes M, Dedhiya P, Ayers LW. Purulence and gram-negative bacilli in tracheal aspirates of mechanically ventilated very low birth weight infants. J Perinatol. (2001) 21:376–81. doi: 10.1038/sj.jp.7210549
160. Claassen CC, Keenan WJ. Challenging the “Culture” of the tracheal aspirate. Neoreviews. (2019) 20:e145. doi: 10.1542/neo.20-3-e145
161. O'Horo JC, Thompson D, Safdar N. Is the gram stain useful in the microbiologic diagnosis of VAP? A meta-analysis Clin Infect Dis. (2012) 55:551–61. doi: 10.1093/cid/cis512
162. Aly H, Badawy M, El-Kholy A, Nabil R, Mohamed A. Randomized, controlled trial on tracheal colonization of ventilated infants: can gravity prevent ventilator-associated pneumonia? Pediatrics. (2008) 122:770–4. doi: 10.1542/peds.2007-1826
163. Papazian L, Klompas M, Luyt CE. Ventilator-associated pneumonia in adults: a narrative review. Intensive Care Med. (2020) 46:888–906. doi: 10.1007/s00134-020-05980-0
164. Kalil AC, Metersky ML, Klompas M, Muscedere J, Sweeney DA, Palmer LB, et al. Management of adults with hospital-acquired and ventilator-associated pneumonia: 2016 clinical practice guidelines by the infectious diseases society of america and the american thoracic society. Clin Infect Dis. (2016) 63:e61–111. doi: 10.1093/cid/ciw353
165. Torres A, Niederman MS, Chastre J, Ewig S, Fernandez-Vandellos P, Hanberger H, et al. International ERS/ESICM/ESCMID/ALAT guidelines for the management of hospital-acquired pneumonia and ventilator-associated pneumonia: Guidelines for the management of hospital-acquired pneumonia (HAP)/ventilator-associated pneumonia (VAP) of the European Respiratory Society (ERS), European Society of Intensive Care Medicine (ESICM), European Society of Clinical Microbiology and Infectious Diseases (ESCMID) and Asociación Latinoamericana del Tórax (ALAT). Eur Respir J. (2017) 50:1700582. doi: 10.1183/13993003.00582-2017
166. Shein SL, Karam O, Beardsley A, Karsies T, Prentice E, Tarquinio KM, et al. Development of an antibiotic guideline for children with suspected ventilator-associated infections. Pediatr Crit Care Med. (2019) 20:697–706. doi: 10.1097/PCC.0000000000001942
167. Barlam TF, Cosgrove SE, Abbo LM, MacDougall C, Schuetz AN, Septimus EJ, et al. Implementing an antibiotic stewardship program: guidelines by the infectious diseases society of America and the Society for Healthcare Epidemiology of America. Clin Infect Dis. (2016) 62:e51–77. doi: 10.1093/cid/ciw118
168. Cernada M, Brugada M, Golombek S, Vento M. Ventilator-associated pneumonia in neonatal patients: an update. Neonatology. (2014) 105:98–107. doi: 10.1159/000355539
169. Busch LM, Kadri SS. Antimicrobial treatment duration in sepsis and serious infections. J Infect Dis. (2020) 222:S142–55. doi: 10.1093/infdis/jiaa247
170. Dimopoulos G, Poulakou G, Pneumatikos IA, Armaganidis A, Kollef MH, Matthaiou DK. Short- vs long-duration antibiotic regimens for ventilator-associated pneumonia: a systematic review and meta-analysis. Chest. (2013) 144:1759–67. doi: 10.1378/chest.13-0076
171. Holman RC, Stoll BJ, Curns AT, Yorita KL, Steiner CA, Schonberger LB. Necrotising enterocolitis hospitalisations among neonates in the United States. Paediatr Perinat Epidemiol. (2006) 20:498–506. doi: 10.1111/j.1365-3016.2006.00756.x
172. Qian T, Zhang R, Zhu L, Shi P, Yang J, Yang CY, et al. Necrotizing enterocolitis in low birth weight infants in China: Mortality risk factors expressed by birth weight categories. Pediatr Neonatol. (2017) 58:509–15. doi: 10.1016/j.pedneo.2016.10.004
173. Blakely ML, Lally KP, McDonald S, Brown RL, Barnhart DC, Ricketts RR, et al. Postoperative outcomes of extremely low birth-weight infants with necrotizing enterocolitis or isolated intestinal perforation: a prospective cohort study by the NICHD Neonatal Research Network. Ann Surg. (2005) 241:984–9; discussion 989–94. doi: 10.1097/01.sla.0000164181.67862.7f
174. Patel RM, Kandefer S, Walsh MC, Bell EF, Carlo WA, Laptook AR, et al. Causes and timing of death in extremely premature infants from 2000 through 2011. N Engl J Med. (2015) 372:331–40. doi: 10.1056/NEJMoa1403489
175. Autmizguine J, Hornik CP, Benjamin DK. Jr., Laughon MM, Clark RH, et al. Anaerobic antimicrobial therapy after necrotizing enterocolitis in VLBW infants. Pediatrics. (2015) 135:e117–25. doi: 10.1542/peds.2014-2141
176. Fitzgibbons SC, Ching Y, Yu D, Carpenter J, Kenny M, Weldon C, et al. Mortality of necrotizing enterocolitis expressed by birth weight categories. J Pediatr Surg. (2009) 44:1072–5; discussion 1075–6. doi: 10.1016/j.jpedsurg.2009.02.013
177. Hintz SR, Kendrick DE, Stoll BJ, Vohr BR, Fanaroff AA, Donovan EF, et al. Neurodevelopmental and growth outcomes of extremely low birth weight infants after necrotizing enterocolitis. Pediatrics. (2005) 115:696–703. doi: 10.1542/peds.2004-0569
178. Rees CM, Pierro A, Eaton S. Neurodevelopmental outcomes of neonates with medically and surgically treated necrotizing enterocolitis. Arch Dis Child Fetal Neonatal Ed. (2007) 92:F193–8. doi: 10.1136/adc.2006.099929
179. Murphy R, Stewart AW, Braithwaite I, Beasley R, Hancox RJ, Mitchell EA, et al. Antibiotic treatment during infancy and increased body mass index in boys: an international cross-sectional study. Int J Obes. (2014) 38:1115–9. doi: 10.1038/ijo.2013.218
180. Lawrence G, Bates J, Gaul A. Pathogenesis of neonatal necrotising enterocolitis. Lancet. (1982) 1:137–9. doi: 10.1016/S0140-6736(82)90383-X
181. Ballance WA, Dahms BB, Shenker N, Kliegman RM. Pathology of neonatal necrotizing enterocolitis: a ten-year experience. J Pediatr. (1990) 117:S6–13. doi: 10.1016/S0022-3476(05)81124-2
182. Hsueh W, Caplan MS, Qu XW, Tan XD, De Plaen IG, Gonzalez-Crussi F. Neonatal necrotizing enterocolitis: clinical considerations and pathogenetic concepts. Pediatr Dev Pathol. (2003) 6:6–23. doi: 10.1007/s10024-002-0602-z
183. Meyer CL, Payne NR, Roback SA. Spontaneous, isolated intestinal perforations in neonates with birth weight less than 1,000 g not associated with necrotizing enterocolitis. J Pediatr Surg. (1991) 26:714–7. doi: 10.1016/0022-3468(91)90017-N
184. Bell MJ, Ternberg JL, Feigin RD, Keating JP, Marshall R, Barton L, et al. Neonatal necrotizing enterocolitis. Therapeutic decisions based upon clinical staging. Ann Surg. (1978) 187:1–7. doi: 10.1097/00000658-197801000-00001
185. Walsh MC, Kliegman RM. Necrotizing enterocolitis: treatment based on staging criteria. Pediatr Clin North Am. (1986) 33:179–201. doi: 10.1016/S0031-3955(16)34975-6
186. Uauy RD, Fanaroff AA, Korones SB, Phillips EA, Phillips JB, Wright LL. Necrotizing enterocolitis in very low birth weight infants: biodemographic and clinical correlates. National institute of child health and human development neonatal research network. J Pediatr. (1991) 119:630–8. doi: 10.1016/S0022-3476(05)82418-7
187. Kliegman RM, Walsh MC. The incidence of meningitis in neonates with necrotizing enterocolitis. Am J Perinatol. (1987) 4:245–8. doi: 10.1055/s-2007-999783
188. Chan KL, Saing H, Yung RW, Yeung YP, Tsoi NS, A. study of pre-antibiotic bacteriology in 125 patients with necrotizing enterocolitis. Acta Paediatr Suppl. (1994) 396:45–8. doi: 10.1111/j.1651-2227.1994.tb13242.x
189. Murphy C, Nair J, Wrotniak B, Polischuk E, Islam S. Antibiotic treatments and patient outcomes in necrotizing enterocolitis. Am J Perinatol. (2020) 37:1250–7. doi: 10.1055/s-0039-1693429
190. Solomkin JS, Mazuski JE, Bradley JS, Rodvold KA, Goldstein EJ, Baron EJ, et al. Diagnosis and management of complicated intra-abdominal infection in adults and children: guidelines by the Surgical Infection Society and the Infectious Diseases Society of America. Clin Infect Dis. (2010) 50:133–64. doi: 10.1086/649554
191. Elgin TG, Kern SL, McElroy SJ. Development of the neonatal intestinal microbiome and its association with necrotizing enterocolitis. Clin Ther. (2016) 38:706–15. doi: 10.1016/j.clinthera.2016.01.005
192. Brower-Sinning R, Zhong D, Good M, Firek B, Baker R, Sodhi CP, et al. Mucosa-associated bacterial diversity in necrotizing enterocolitis. PLoS ONE. (2014) 9:e105046. doi: 10.1371/journal.pone.0105046
193. Blackwood BP, Hunter CJ, Grabowski J. Variability in antibiotic regimens for surgical necrotizing enterocolitis highlights the need for new guidelines. Surg Infect (Larchmt). (2017) 18:215–20. doi: 10.1089/sur.2016.163
194. Shah D, Sinn JK. Antibiotic regimens for the empirical treatment of newborn infants with necrotising enterocolitis. Cochrane Database Syst Rev. (2012) Cd007448. doi: 10.1002/14651858.CD007448.pub2
195. Faix RG, Polley TZ, Grasela TH. A randomized, controlled trial of parenteral clindamycin in neonatal necrotizing enterocolitis. J Pediatr. (1988) 112:271–7. doi: 10.1016/S0022-3476(88)80069-6
196. Hansen TN, Ritter DA, Speer ME, Kenny JD, Rudolph AJ. A randomized, controlled study of oral gentamicin in the treatment of neonatal necrotizing enterocolitis. J Pediatr. (1980) 97:836–9. doi: 10.1016/S0022-3476(80)80283-6
197. Antibiotic Safety (SCAMP). Available online at: https://ClinicalTrials.gov/show/NCT01994993 (accessed March 10, 2022).
198. Luo LJ, Li X, Yang KD, Lu JY, Li LQ. Broad-spectrum antibiotic plus metronidazole may not prevent the deterioration of necrotizing enterocolitis from stage II to III in full-term and near-term infants: a propensity score-matched cohort study. Medicine. (2015) 94:e1862. doi: 10.1097/MD.0000000000001862
199. Scheifele DW, Ginter GL, Olsen E, Fussell S, Pendray M. Comparison of two antibiotic regimens for neonatal necrotizing enterocolitis. J Antimicrob Chemother. (1987) 20:421–9. doi: 10.1093/jac/20.3.421
200. Stringer MD, Brereton RJ, Drake DP, Kiely EM, Capps SN, Spitz L. Recurrent necrotizing enterocolitis. J Pediatr Surg. (1993) 28:979–81. doi: 10.1016/0022-3468(93)90496-8
201. Martin A, Pappas A, Lulic-Botica M, Natarajan G. Impact of 'targeted' fluconazole prophylaxis for preterm neonates: efficacy of a highly selective approach? J Perinatol. (2012) 32:21–6. doi: 10.1038/jp.2011.27
202. Levy I, Comarsca J, Davidovits M, Klinger G, Sirota L, Linder N. Urinary tract infection in preterm infants: the protective role of breastfeeding. Pediatr Nephrol. (2009) 24:527–31. doi: 10.1007/s00467-008-1007-7
203. Bauer S, Eliakim A, Pomeranz A, Regev R, Litmanovits I, Arnon S, et al. Urinary tract infection in very low birth weight preterm infants. Pediatr Infect Dis J. (2003) 22:426–30. doi: 10.1097/01.inf.0000065690.64686.c9
204. Baracco R, Mattoo TK. Diagnosis and management of urinary tract infection and vesicoureteral reflux in the neonate. Clin Perinatol. (2014) 41:633–42. doi: 10.1016/j.clp.2014.05.011
205. Lai A, Rove KO, Amin S, Vricella GJ, Coplen DE. Diagnosis and management of urinary tract infections in premature and term infants. Neoreviews. (2018) 19:e337. doi: 10.1542/neo.19-6-e337
206. Arshad M, Seed PC. Urinary tract infections in the infant. Clin Perinatol. (2015) 42:17–28, vii. doi: 10.1016/j.clp.2014.10.003
207. Ruangkit C, Satpute A, Vogt BA, Hoyen C, Viswanathan S. Incidence and risk factors of urinary tract infection in very low birth weight infants. J Neonatal Perinatal Med. (2016) 9:83–90. doi: 10.3233/NPM-16915055
208. Zorc JJ, Levine DA, Platt SL, Dayan PS, Macias CG, Krief W, et al. Clinical and demographic factors associated with urinary tract infection in young febrile infants. Pediatrics. (2005) 116:644–8. doi: 10.1542/peds.2004-1825
209. Mohseny AB, van Velze V, Steggerda SJ, Smits-Wintjens V, Bekker V, Lopriore E. Late-onset sepsis due to urinary tract infection in very preterm neonates is not uncommon. Eur J Pediatr. (2018) 177:33–8. doi: 10.1007/s00431-017-3030-9
210. Biyikli NK, Alpay H, Ozek E, Akman I, Bilgen H. Neonatal urinary tract infections: analysis of the patients and recurrences. Pediatr Int. (2004) 46:21–5. doi: 10.1111/j.1442-200X.2004.01837.x
211. Bonadio W, Maida G. Urinary tract infection in outpatient febrile infants younger than 30 days of age: a 10-year evaluation. Pediatr Infect Dis J. (2014) 33:342–4. doi: 10.1097/INF.0000000000000110
212. Nowell L, Moran C, Smith PB, Seed P, Alexander BD, Cotten CM Jr, et al. Prevalence of renal anomalies after urinary tract infections in hospitalized infants less than 2 months of age. J Perinatol. (2010) 30:281–5. doi: 10.1038/jp.2009.147
213. Robinson JL, Davies HD, Barton M, O'Brien K, Simpson K, Asztalos E, et al. Sauve, de Repentigny L, Kovacs L, Tan B, Richardson SE. Characteristics and outcome of infants with candiduria in neonatal intensive care - a Paediatric Investigators Collaborative Network on Infections in Canada (PICNIC) study. BMC Infect Dis. (2009) 9:183. doi: 10.1186/1471-2334-9-183
214. Austin BJ, Bollard C, Gunn TR. Is urethral catheterization a successful alternative to suprapubic aspiration in neonates? J Paediatr Child Health. (1999) 35:34–6. doi: 10.1046/j.1440-1754.1999.00305.x
215. Weill O, Labrosse M, Levy A, Desjardins MP, Trottier ED, Gravel J. Point-of-care ultrasound before attempting clean-catch urine collection in infants: a randomized controlled trial. CJEM. (2019) 21:646–52. doi: 10.1017/cem.2019.30
216. Desai S, Aronson PL, Shabanova V, Neuman MI, Balamuth F, Pruitt CM, et al. Febrile young infant research, parenteral antibiotic therapy duration in young infants with bacteremic urinary tract infections. Pediatrics. (2019) 144:e20183844. doi: 10.1542/peds.2018-3844
217. Averbuch D, Nir-Paz R, Tenenbaum A, Stepensky P, Brooks R, Koplewitz BZ, et al. Factors associated with bacteremia in young infants with urinary tract infection. Pediatr Infect Dis J. (2014) 33:571–5. doi: 10.1097/INF.0000000000000316
218. Sastre JB, Aparicio AR, Cotallo GD, Colomer BF, Hernández MC. Urinary tract infection in the newborn: clinical and radio imaging studies. Pediatr Nephrol. (2007) 22:1735–41. doi: 10.1007/s00467-007-0556-5
219. Vachharajani A, Vricella GJ, Najaf T, Coplen DE. Prevalence of upper urinary tract anomalies in hospitalized premature infants with urinary tract infection. J Perinatol. (2015) 35:362–6. doi: 10.1038/jp.2014.209
220. Mangram AJ, Horan TC, Pearson ML, Silver LC, Jarvis WR. Guideline for prevention of surgical site infection, 1999. Centers for Disease Control and Prevention (CDC) Hospital Infection Control Practices Advisory Committee. Am J Infect Control. (1999) 27:97–132; quiz 133–4; discussion 96. doi: 10.1016/S0196-6553(99)70088-X
221. Hranjec T, Swenson BR, Sawyer RG. Surgical site infection prevention: how we do it. Surg Infect. (2010) 11:289–94. doi: 10.1089/sur.2010.021
222. Segal I, Kang C, Albersheim SG, Skarsgard ED, Lavoie PM. Surgical site infections in infants admitted to the neonatal intensive care unit. J Pediatr Surg. (2014) 49:381–4. doi: 10.1016/j.jpedsurg.2013.08.001
223. Clements KE, Fisher M, Quaye K, O'Donnell R, Whyte C, Horgan MJ. Surgical site infections in the NICU. J Pediatr Surg. (2016) 51:1405–8. doi: 10.1016/j.jpedsurg.2016.04.002
224. Walker S, Datta A, Massoumi RL, Gross ER, Uhing M, Arca MJ. Antibiotic stewardship in the newborn surgical patient: a quality improvement project in the neonatal intensive care unit. Surgery. (2017) 162:1295–303. doi: 10.1016/j.surg.2017.07.021
225. Clark RH, Bloom BT, Spitzer AR, Gerstmann DR. Empiric use of ampicillin and cefotaxime, compared with ampicillin and gentamicin, for neonates at risk for sepsis is associated with an increased risk of neonatal death. Pediatrics. (2006) 117:67–74. doi: 10.1542/peds.2005-0179
226. Tzialla C, Borghesi A, Perotti GF, Garofoli F, Manzoni P, Stronati M. Use and misuse of antibiotics in the neonatal intensive care unit. J Matern Fetal Neonatal Med. (2012) 4:35–7. doi: 10.3109/14767058.2012.714987
227. Bratzler DW, Dellinger EP, Olsen KM, Perl TM, Auwaerter PG, Bolon MK, et al. Clinical practice guidelines for antimicrobial prophylaxis in surgery. Am J Health Syst Pharm. (2013) 70:195–283. doi: 10.2146/ajhp120568
228. Laituri C, Arnold MA. A standardized guideline for antibiotic prophylaxis in surgical neonates(). Semin Pediatr Surg. (2019) 28:53–6. doi: 10.1053/j.sempedsurg.2019.01.009
229. Vu LT, Nobuhara KK, Lee H, Farmer DL. Conflicts in wound classification of neonatal operations. J Pediatr Surg. (2009) 44:1206–11. doi: 10.1016/j.jpedsurg.2009.02.026
230. Garland JS, Alex CP, Henrickson KJ, McAuliffe TL, Maki DG, A. vancomycin-heparin lock solution for prevention of nosocomial bloodstream infection in critically ill neonates with peripherally inserted central venous catheters: a prospective, randomized trial. Pediatrics. (2005) 116:e198–205. doi: 10.1542/peds.2004-2674
231. Spafford PS, Sinkin RA, Cox C, Reubens L, Powell KR. Prevention of central venous catheter-related coagulase-negative staphylococcal sepsis in neonates. J Pediatr. (1994) 125:259–63. doi: 10.1016/S0022-3476(94)70208-X
232. Harms K, Herting E, Kron M, Schiffmann H, Schulz-Ehlbeck H. Randomized, controlled trial of amoxicillin prophylaxis for prevention of catheter-related infections in newborn infants with central venous silicone elastomer catheters. J Pediatr. (1995) 127:615–9. doi: 10.1016/S0022-3476(95)70126-5
233. Skarsgard ED. Immediate versus staged repair of omphaloceles. Semin Pediatr Surg. (2019) 28:89–94. doi: 10.1053/j.sempedsurg.2019.04.010
234. Klein MD, Chapter 75 - congenital defects of the abdominal wall. In: Coran AG, editors. Pediatric Surgery. 7th Ed. Philadelphia: Mosby (2012). p. 973–84.
235. Baird R, Puligandla P, Skarsgard E, Laberge J-M, The Canadian pediatric surgical Network. Infectious complications in the management of gastroschisis. Pediatr Surg Int. (2012) 28:399–404. doi: 10.1007/s00383-011-3038-6
236. Zani A, Eaton S, Puri P, Rintala R, Lukac M, Bagolan P, et al. International survey on the management of congenital diaphragmatic hernia. Eur J Pediatr Surg. (2016) 26:38–46. doi: 10.1055/s-0035-1564713
237. Keene S, Murthy K, Pallotto E, Brozanski B, Gien J, Zaniletti I, et al. Acquired infection and antimicrobial utilization during initial NICU hospitalization in infants with congenital diaphragmatic hernia. Pediatr Infect Dis J. (2018) 37:469–74. doi: 10.1097/INF.0000000000001835
238. Lal D, Miyano G, Juang D, Sharp NE, St Peter SD. Current patterns of practice and technique in the repair of esophageal atresia and tracheoesophageal fistua: an IPEG survey. J Laparoendosc Adv Surg Tech A. (2013) 23:635–8. doi: 10.1089/lap.2013.0210
239. Edelman B, Selvaraj BJ, Joshi M, Patil U, Yarmush J. Anesthesia practice: review of perioperative management of H-type tracheoesophageal fistula. Anesthesiol Res Pract. (2019) 2019:8621801. doi: 10.1155/2019/8621801
240. Krosnar S, Baxter A. Thoracoscopic repair of esophageal atresia with tracheoesophageal fistula: anesthetic and intensive care management of a series of eight neonates. Paediatr Anaesth. (2005) 15:541–6. doi: 10.1111/j.1460-9592.2005.01594.x
241. Rangel SJ, Islam S, St Peter SD, Goldin AB, Abdullah F, Downard CD, et al. Prevention of infectious complications after elective colorectal surgery in children: an American Pediatric Surgical Association Outcomes and Clinical Trials Committee comprehensive review. J Pediatr Surg. (2015) 50:192–200. doi: 10.1016/j.jpedsurg.2014.11.028
Keywords: neonate, sepsis, urinary tract infection, surgical prophylaxis, ventilator-associated pneumonia, necrotizing enterocolitis, antimicrobial
Citation: Ting JY, Autmizguine J, Dunn MS, Choudhury J, Blackburn J, Gupta-Bhatnagar S, Assen K, Emberley J, Khan S, Leung J, Lin GJ, Lu-Cleary D, Morin F, Richter LL, Viel-Thériault I, Roberts A, Lee K-s, Skarsgard ED, Robinson J and Shah PS (2022) Practice Summary of Antimicrobial Therapy for Commonly Encountered Conditions in the Neonatal Intensive Care Unit: A Canadian Perspective. Front. Pediatr. 10:894005. doi: 10.3389/fped.2022.894005
Received: 11 March 2022; Accepted: 13 June 2022;
Published: 08 July 2022.
Edited by:
Claus Klingenberg, UiT The Arctic University of Norway, NorwayReviewed by:
Christoph Härtel, University Hospital Würzburg, GermanyRachel Greenberg, Duke University, United States
Karen Puopolo, University of Pennsylvania, United States
Copyright © 2022 Ting, Autmizguine, Dunn, Choudhury, Blackburn, Gupta-Bhatnagar, Assen, Emberley, Khan, Leung, Lin, Lu-Cleary, Morin, Richter, Viel-Thériault, Roberts, Lee, Skarsgard, Robinson and Shah. This is an open-access article distributed under the terms of the Creative Commons Attribution License (CC BY). The use, distribution or reproduction in other forums is permitted, provided the original author(s) and the copyright owner(s) are credited and that the original publication in this journal is cited, in accordance with accepted academic practice. No use, distribution or reproduction is permitted which does not comply with these terms.
*Correspondence: Joseph Y. Ting, am9zZXBoLnRpbmdAdWFsYmVydGEuY2E=