- 1Department of Neonatology, Royal Hospital for Children, Glasgow, United Kingdom
- 2Ospedale San Filippo Neri, Rome, Italy
- 3Intensive Care Unit, Department of Pediatric Surgery, Erasmus MC-Sophia Children’s Hospital, Rotterdam, Netherlands
- 4Department of Neonatology and Pediatric Intensive Care, Children’s Hospital, University of Bonn, Bonn, Germany
There is growing recognition that the heart is a key contributor to the pathophysiology of congenital diaphragmatic hernia (CDH), in conjunction with developmental abnormalities of the lung and pulmonary vasculature. Investigations to date have demonstrated altered fetal cardiac morphology, notably relative hypoplasia of the fetal left heart, as well as early postnatal right and left ventricular dysfunction which appears to be independently associated with adverse outcomes. However, many more unknowns remain, not least an understanding of the genetic and cellular basis for cardiac dysplasia and dysfunction in CDH, the relationship between fetal, postnatal and long-term cardiac function, and the impact on other parts of the body especially the developing brain. Consensus on how to measure and classify cardiac function and pulmonary hypertension in CDH is also required, potentially using both non-invasive imaging and biomarkers. This may allow routine assessment of the relative contribution of cardiac dysfunction to individual patient pathophysiological phenotype and enable better, individualized therapeutic strategies incorporating targeted use of fetal therapies, cardiac pharmacotherapies, and extra-corporeal membrane oxygenation (ECMO). Collaborative, multi-model approaches are now required to explore these unknowns and fully appreciate the role of the heart in CDH.
Introduction
The past decade has seen growing appreciation of the heart as a key component of disease pathophysiology in congenital diaphragmatic hernia (CDH) (1). The application of advanced imaging modalities combined with multi-center registry analysis has shone new light on fetal cardiac development and the role of postnatal cardiac function, alongside pulmonary hypertension and pulmonary hypoplasia, in determining clinical phenotypes and outcomes in CDH. However, there is much more to understand in relation to cardiac development, mechanisms of dysfunction, and their clinical significance throughout life. Addressing these uncertainties may lead to new therapeutic strategies and improved outcomes in CDH.
This review aims to provide a current “state of the art,” comprehensively reviewing what is known of the heart from fetal life to adulthood in CDH, highlighting the major areas of ongoing uncertainty, and identifying key priorities for further investigation.
The fetal heart in congenital diaphragmatic hernia
Cardiac abnormalities in CDH, as with those in the lungs and pulmonary circulation, undoubtably have their origins in the fetus.
Congenital heart disease in fetal congenital diaphragmatic hernia
Congenital diaphragmatic hernia and congenital heart disease (CHD) are frequently associated. In recent systematic review up to 15% of live born CDH patients also have CHD, though rates may be as high as 28% when stillborn and terminated CDH cases are included (2). Conversely, 0.3% of infants requiring surgery for CHD have associated CDH (3).
Forty-two percent of infants with CDH and CHD are considered to have critical lesions, rather than simple shunts (ventricular and atrial septal defects, patent ductus arteriosus). The commonest associated cardiac lesions are, in order of frequency, ventricular and atrial septal defects, hypoplastic left heart syndrome (HLHS), coarctation of the aorta or aortic hypoplasia, tetralogy of Fallot and double outlet right ventricle (2, 4).
The relationship between measures of CDH severity (e.g., defect size, abdominal organ position, fetal lung size) and CHD incidence is as not yet understood. Similarly, the potential shared mechanisms, including genetic and environmental factors, contributing to CDH and associated CHD remain unclear, but may involve disruption of common pathways in cardiac and diaphragmatic development (5, 6). An increasing number of genetic mutations have been identified in CDH, however, the frequency of these is not affected by the presence or absence of associated anomalies including CHD (7, 8). Of note, HLHS and aortic anomalies may represent one end of a spectrum of left heart hypoplasia in CDH that is distinct from other mechanisms of CHD, as discussed in more detail below.
The presence of any CHD significantly affects surgical management of the diaphragmatic hernia. Systematic review indicates that CDH repair rates are lower (72% vs. 85%), patch repair more frequent (45% vs. 30%), and minimally invasive approaches are employed less often (5% vs. 17%). CDH repair typically precedes any cardiac intervention, and notably only 10% of affected cases received a cardiac intervention during the neonatal period (2). ECMO use is similar between CHD and non-CHD groups (9). The commonest cardiac surgeries performed in CDH cases are hybrid procedures, coarctation and aortic arch repair, VSD repair and pulmonary artery banding (3).
Importantly, any CHD confers lower survival rates in CDH, which approach 50% overall and as low as 30% for infants with critical cardiac lesions, and 1–5% for infants with CDH and HLHS (2, 3, 9, 10). Conversely, the presence of CDH also confers higher overall and peri-operative mortality when compared to all CHD, as well as increased rates of post-op complications and longer length of stay after cardiac surgery in both high and low risk cardiac lesions (3, 4).
Though no formal guidelines exist for management of CHD in CDH, an algorithmic, team approach has been advocated to assist in complex decision-making for critical lesions (11).
The remainder of this review will now focus on abnormalities of cardiac structure and function distinct from classical CHD, and which appear to be specific to CDH pathophysiology.
Cardiac hypoplasia in congenital diaphragmatic hernia
Hypoplasia of the developing heart in CDH is an established finding, observed first in post-mortem studies and confirmed by echocardiography analyses, Table 1 (12–14).
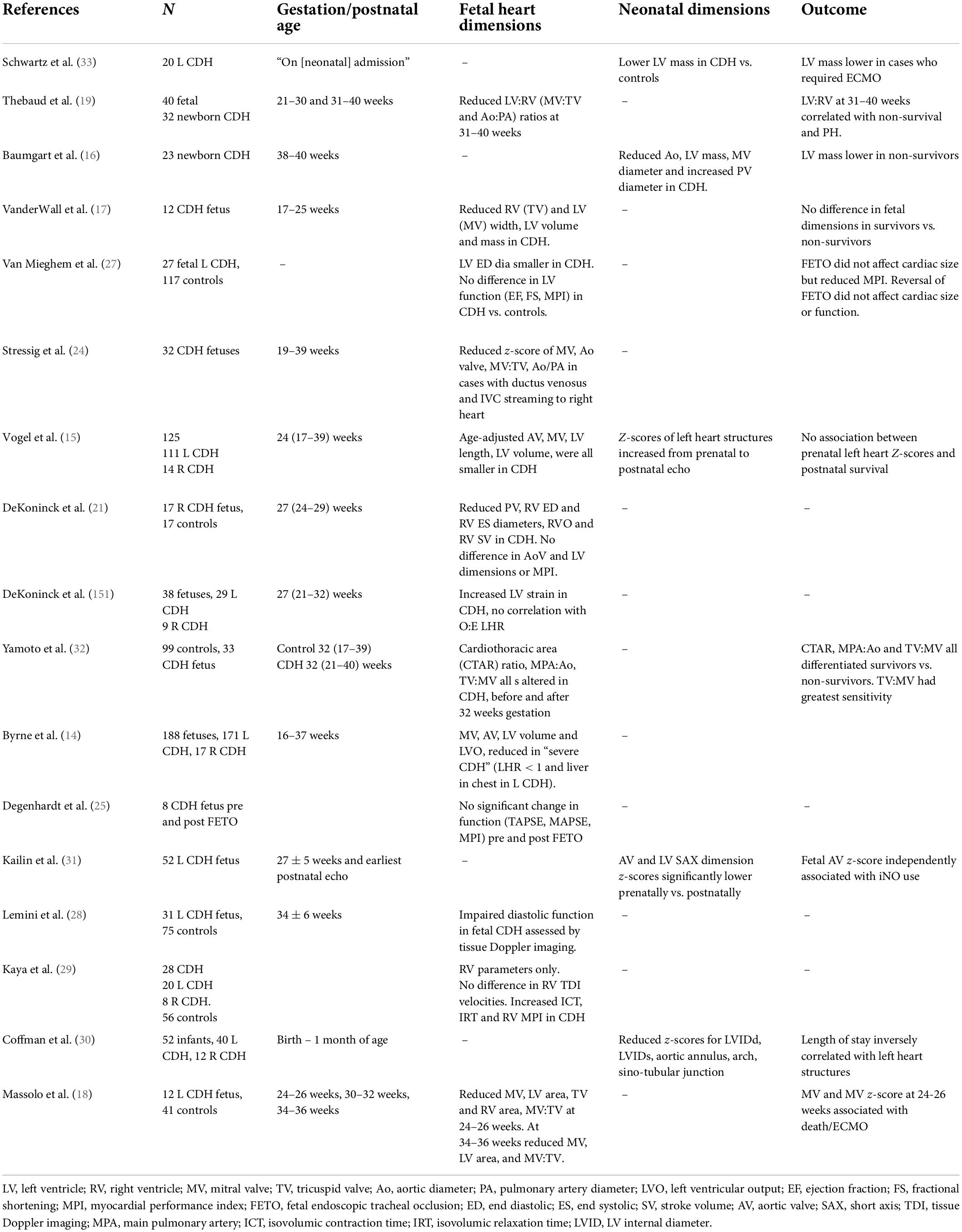
Table 1. Echocardiographic studies of fetal and early postnatal cardiac dimensions and fetal cardiac function in CDH.
In fetuses with left-sided CDH ventricular hypoplasia appears to predominantly affect the left ventricle (LV), characterized by reduced ventricular width and associated reductions in LV area and mass, together with reduced aortic valve diameter (14, 15). Right ventricular (RV) dimensions may also be reduced at earlier gestation but appear to increase, along with pulmonary artery diameter, at later gestation (16, 17). Accordingly, ratios of left to right cardiac dimensions in left-sided CDH are lowest at later gestations (18–20). Conversely, in right-sided CDH the limited available data indicate reduced fetal right ventricular and pulmonary arterial dimensions combined and less severe LV hypoplasia than in left-sided CDH (14, 21).
Multiple mechanisms of fetal cardiac hypoplasia have been proposed, though the relative contribution and timing of these remains uncertain (Figure 1):
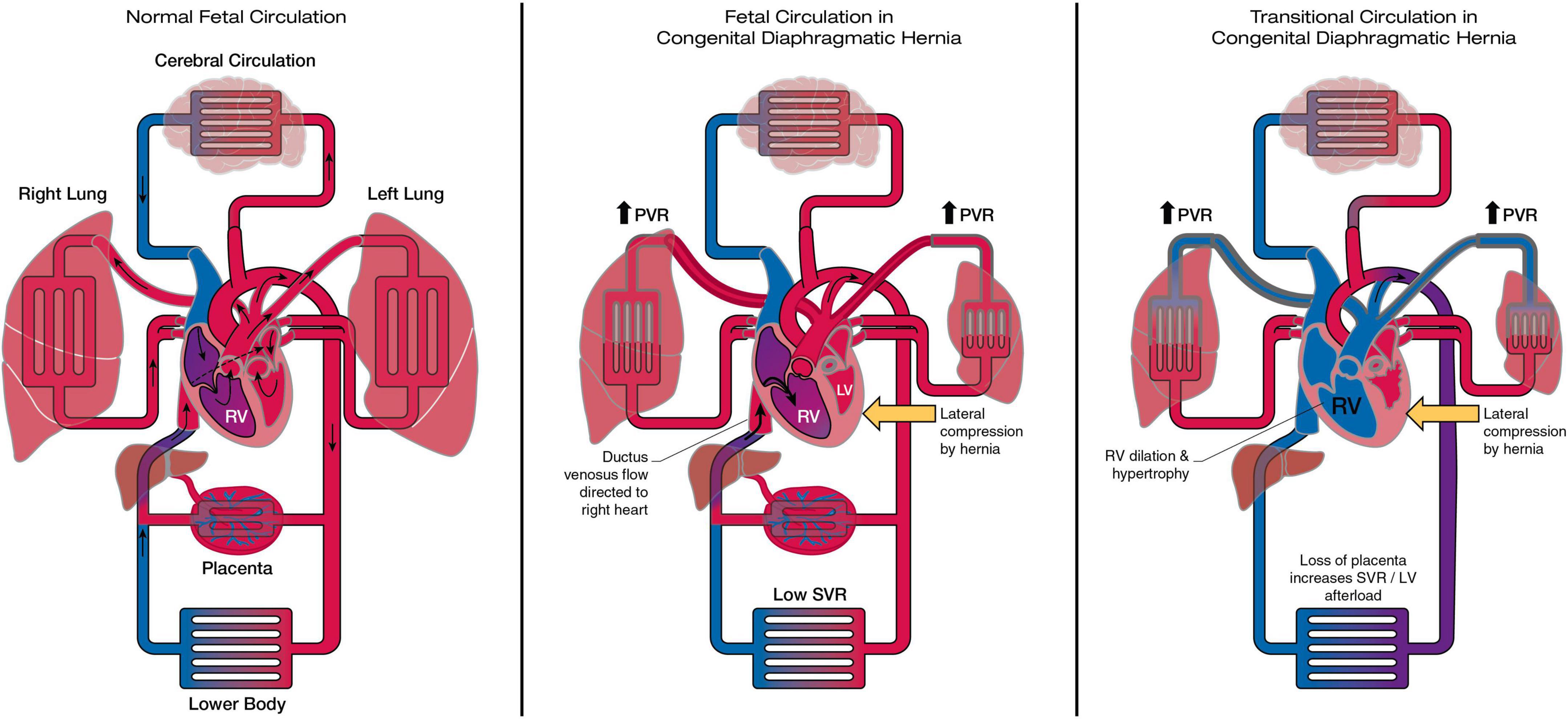
Figure 1. The heart and circulation in CDH during the fetal and transitional period. In the fetal CDH circulation LV hypoplasia may be related to redirection of ductus venosus flow to the right heart and reduced pulmonary blood flow, together with lateral compression by the herniating abdominal contents. In the transitional period removal of the placenta increases afterload on the ventricles. The right ventricle dilates and becomes dysfunctional in the face of sustained postnatal increase in PVR. LV function is at risk due to pre-existent hypoplasia, septal displacement and the acute increase in afterload. RV, right ventricle; LV, left ventricle; PVR, pulmonary vascular resistance; SVR, systemic vascular resistance.
1. Mechanical compression of the developing heart by herniating abdominal contents. Consistent with this hypothesis ventricular hypoplasia is greatest on the ipsilateral side and appears to disproportionately affects ventricular width rather than length (14, 18). Analogous reductions in fetal mitral valve and AV diameter are observed in fetuses with large, compressive left-sided congenital lung masses (22).
2. Reduced pulmonary blood flow in CDH compared to normal fetuses, due to the primary structural changes in the pulmonary vasculature characteristic of CDH. This in turn leads to reduced pulmonary venous return, LV filling and growth. In support of this flow-based mechanism reduced LV dimensions are similarly observed in fetuses with anomalous pulmonary venous drainage (23). This mechanism would in theory have greatest impact later in gestation when, in the normal fetus, increases in fetal pulmonary blood flow and LV size are usually observed.
3. An additional flow-based mechanism of LV hypoplasia in CDH may be due to mediastinal shift and liver herniation resulting in re-direction of ductus venosus (DV) and inferior vena cava (IVC) streaming away from the foramen ovale and resulting in reduced LV filling and growth (24). Oxygenated venous return is instead re-directed to the right heart and thereafter the majority will pass across the patent ductus and a minority to the pulmonary arteries. Increased oxygenation of the fetal pulmonary blood flow, as a secondary consequence of this mechanism, has also been hypothesized as a possible cause of the excessive muscularization of the pulmonary arterioles in CDH.
Fetal cardiac function in congenital diaphragmatic hernia
Prenatal cohort studies of myocardial function have not to date demonstrated significant fetal cardiac dysfunction (25–29). Theoretically, the fetal circulation may mitigate against cardiac dysfunction in utero; the presence of a patent ductus and low resistance placenta protecting the RV from excessive afterload (despite increased resistance in the pulmonary vasculature) and ensure that the non-dominant, hypoplastic LV remains untaxed by excessive preload or afterload, Figure 1. Prospective studies throughout gestation are needed to understand the natural history and clinical significance of fetal cardiac function.
Clinical significance of fetal cardiac hypoplasia in congenital diaphragmatic hernia
The relationship between fetal cardiac hypoplasia and clinical outcome remains unresolved. In single center cohorts of left-sided CDH left heart dimensions, notably LV width, mitral and aortic valve diameters, and ratios of left to right-sided dimensions have been associated with adverse outcome including higher neonatal mortality, higher ECMO use, increased inhaled nitric oxide use, and prolonged length of stay (18, 19, 30–33).
However, these findings have not been replicated in other series. VanderWall did not observe any association between fetal ventricular dimensions and outcome, though small cohort size may have been a factor (17). Vogel et al. observed mild to moderate fetal LV hypoplasia in a cohort of 125 CDH cases. LV fetal dimensions, expressed as z-scores, were not associated with postnatal survival when analyzed as continuous data, but were on categorical analysis (15). In the same cohort, there was a trend toward normalization of LV dimensions on paired postnatal, post-CDH repair, echocardiograms. This may suggest that reduced LV volumes are recruitable in response to postnatal hemodynamics.
Further investigation is a priority to determine which, if any, fetal cardiac dimensions have the greatest prognostic utility, when and how these should be measured, and the mechanisms by which these might directly influence postnatal LV dimensions, function, and outcome.
Cellular and metabolic function in the fetal heart in congenital diaphragmatic hernia
Whether structural changes in the fetal heart in CDH are associated with primary or secondary abnormalities at a genetic, epigenetic, cellular or metabolic level remains largely unknown. Cardiac hypoplasia in nitrofen rat models of CDH is associated with reduced expression of insulin-like growth factor-1, epidermal growth factor, basic fibroblast growth factor and platelet derived growth factor, Table 2 (34, 35). Zhaourigetu et al. also recently observed abnormal cardiomyocyte structure associated with reduced expression of mitochondrial and fatty acid biogenesis genes in this CDH model (36).
A single post-mortem study of hearts from human CDH fetuses also demonstrated dis-homogenous growth factor expression (37). In the same study fetal CDH hearts had abnormal thickening and proliferation of intra-myocardial vessels, particularly in the inter-ventricular septum. This raises the hypothesis that intra-cardiac vasculature in CDH might demonstrate developmental abnormalities analogous to those in the pulmonary vasculature (18).
In fetal rabbit models of CDH, though not lamb models, cardiac hypoplasia is associated with decreased ventricular wall thickness and increased septal thickness (38, 39). Ventricular wall thickening, due to cardiomyocyte hyperplasia, is also observed in hypoplastic left heart syndrome (HLHS), potentially pointing to common mechanisms of cardiac dysgenesis in these two conditions (40).
Fetal therapies and the developing heart in congenital diaphragmatic hernia
In limited cohort studies fetal tracheal occlusion (FETO), the principal prenatal therapy attempted for CDH, does not appear to significantly affect fetal cardiac dimensions or function in CDH (16, 18). Of note, the recent larger multicenter TOTAL trials of FETO did not include assessment of cardiac dimensions or function FETO (41).
A variety of pre-natal pharmacotherapies aimed at modulating pulmonary vascular development have been investigated in pre-clinical CDH models (42). Sildenafil, a phosphodiesterase 5 inhibitor partially reverses pulmonary vascular abnormalities and lowering pulmonary vascular resistance and reducing RV hypertrophy in animal models (43–45). However, adverse events associated with of prenatal administration in non-CDH patients have prevented clinical trials in diaphragmatic hernia (46). Maternal hyperoxygenation may be an alternative therapeutic approach which in congenital heart disease has been observed to increase pulmonary blood flow, and could potentially increase left ventricular flow and size in turn (47, 48). Further investigation is required of the impact of prenatal therapies on cardiac development and postnatal function in CDH.
The heart in early postnatal life in congenital diaphragmatic hernia
Changes in the fetal heart in CDH may be important precedents of postnatal cardiac function. Recent investigation using functional echocardiography and multi-center registry analysis have led to new, but still incomplete, understanding of the heart’s unsteady transition from pre-natal to post-natal environment in CDH, Table 3. Figure 1 provides a visual overview of these changes in loading conditions, cardiac morphology and function from fetal to postnatal life.
Right ventricle dysfunction in congenital diaphragmatic hernia
Dysfunction in the RV, in conjunction with ventricular dilatation and hypertrophy, has been demonstrated from the first days of life in CDH (49). These are considered to result from pathological increases in RV afterload as a result of the structural and functional pulmonary vascular abnormalities characteristic of CDH (50). Increased RV pressure, and a concomitant reduced systemic pressure, may also reduce coronary artery flow gradient, leading to RV ischemia and dysfunction. Whilst recognized in other pulmonary hypertensive disease this mechanism has not been studied directly in CDH (51).
Early RV dysfunction in CDH is characterized by both impaired systolic function and, notably early diastolic dysfunction, demonstrated by reduced diastolic myocardial velocities and shortened diastolic duration in the RV (52–55).
Right ventricle dysfunction, when present, likely contributes to early clinical instability via a number of mechanisms. First, the failing RV may become “uncoupled” from the pulmonary circulation, unable to maintain adequate pulmonary blood flow, contributing to impaired oxygenation, and reduced LV filling and output (56). Second, RV dysfunction negatively impacts LV performance via mechanisms of ventricular interdependence including shared myocardial fibers, disruption of normal systolic and diastolic time intervals, and septal displacement reducing LV volume (40). Through these mechanisms RV dysfunction may be a key mediator of adverse effects of pulmonary hypertension in CDH (57, 58).
In cohort studies early RV dysfunction is associated with prolonged duration of respiratory support, increased mortality and ECMO use (49, 59–61). In a recent large multi-center registry analysis of cardiac function in the first 48 h of life RV dysfunction was present in 34% of CDH cases either in isolation in combination with LV dysfunction, and was associated with increased mortality and ECMO use (62).
Left ventricular dysfunction in congenital diaphragmatic hernia
The potential for postnatal left ventricular dysfunction in newborns with CDH has been long-recognized by experienced clinicians, and more recently quantified using functional echocardiographic techniques (52, 55, 63). Its importance as a component of CDH pathophysiology was highlighted by recent multi-center analysis demonstrating that LV dysfunction, in isolation or combined with RV dysfunction, independently predicted death and ECMO use (62).
Early postnatal LV dysfunction is frequent. In registry analysis of over 1100 CDH cases from 59 centers LV dysfunction was reported in 24% of CDH cases (62). However, this may be an underestimate; In smaller cohort studies utilizing sensitive, quantitative strain analysis of myocardial function LV dysfunction was observed in 56% of cases (63).
Postnatal LV dysfunction is characterized by both global systolic and diastolic dysfunction affecting longitudinal, circumferential and radial function, and dyssynchrony of myocardial segments (60, 63, 64). Postnatal LV volumes may also be reduced possibly due to compressive actions of a dilated RV and the herniated organs, combined with the legacy of fetal LV hypoplasia.
Left ventricle dysfunction is frequently observed in combination with RV dysfunction and may therefore be a secondary consequence via mechanisms of ventricular interdependence, as discussed above (64). However primary LV dysfunction may occur in the absence of, or disproportionate to, RV dysfunction (62). Multiple factors are hypothesized to contribute to primary postnatal LV dysfunction in the transitional period (1, 65):
I. Fetal LV dysfunction
II. Fetal LV hypoplasia
III. Changes in LV loading conditions: reduced preload, due to failure of normal increases in pulmonary blood flow at birth, and increased afterload due to removal of the low-resistance placenta from the systemic circulation
IV. Hypoxia and acidosis contributing to worsening ventricular function
Left ventricle dysfunction likely contributes to adverse clinical outcome via reduced LV output and systemic blood flow, resulting in impaired tissue oxygenation and a viscous cycle of worsening hypoxia and acidosis (66). Accordingly, the systemic hypotension frequently observed in early CDH is likely to be a consequence of impaired cardiac function and cardiac output, rather than hypovolemia or low systemic vascular resistance.
Early LV dysfunction is associated with other markers of CDH severity, including smaller fetal lung volumes, larger diaphragmatic defect size, and liver herniation (62, 63). However, Dao et al. have observed that LV dysfunction may also occur in “lower risk” CDH cases with smaller defects (67).
Of note, LV dysfunction appears to be a transitional phenomenon, apparently present from soon after birth but with the potential to improve rapidly over the first week of life (68, 69). This may have important consequences for individualized management strategies, including ECMO, as discussed below.
Left ventricle dysfunction as a mechanism of pulmonary hypertension
Left ventricle dysfunction leading to increased end diastolic, left atrial and pulmonary venous pressures is well-recognized as a mechanism of increased pulmonary vascular resistance in adult heart disease (70). There is increasing recognition that similar mechanisms may occur in CDH in the setting of early, transition LV dysfunction, contributing to a post-capillary increase in pulmonary venous resistance, distinct from pre-capillary changes in pulmonary arterial resistance (1). This may have important implications for targeted management strategies in CDH, including the suitability of pulmonary vasodilators, as discussed below.
Hemodynamic phenotypes in congenital diaphragmatic hernia
The complex interplay of ventriculo-arterial, inter-ventricular, and cardio-respiratory interactions in CDH results in dynamic hemodynamic phenotypes with variable RV and LV function and dysfunction. As discussed later, this concept may be important in targeted, individualized therapeutic strategies.
Atrial shunting patterns may help define these phenotypes in the clinical setting. As recently highlighted by Wehrmann et al., the frequent presence of left-to-right atrial shunting in CDH, despite elevated pulmonary artery pressures, should prompt a closer examination of the left ventricular size and function (71).
Variability in cardiac dysfunction and clinical phenotypes
Current models of CDH are based on three key inter-related pathophysiologies; pulmonary hypertension, pulmonary hypoplasia, and cardiac dysfunction (65). However, the severity of each of these may be variable and disproportionate. Although severe cardiac dysfunction is associated with larger diaphragmatic defects, smaller lung volumes, and more severe pulmonary hypertension, it may also be observed in patients with smaller diaphragmatic defects and milder respiratory compromise (62, 63, 67). Improved recognition and characterization of individual clinical phenotypes may be an important concept in CDH, and potentially inform more effective, targeted therapies.
Echocardiographic assessment of cardiac function and hemodynamics in congenital diaphragmatic hernia
The absence of standardized definitions and measurement tools are a major ongoing barrier to hemodynamic research and clinical management in CDH.
A variety of functional echocardiographic parameters have been used to assess cardiac function and pulmonary hypertension in CDH, as listed in Tables 1, 4. However, each has practical and technical limitations and there is no single “gold standard” measure (72). Though useful definitions of cardiac dysfunction and pulmonary hypertension have been employed in individual studies, there is no established consensus agreement on these (58, 73).
To enable consistent multi-center hemodynamic data collection four actions are required:
1. Recommendations for routine use of functional echocardiography in acute CDH care
2. International consensus on measurement parameters, definitions and classification of cardiac function and pulmonary hypertension based on existing international guidance and adapted specifically for CDH (74–78).
3. Exploration of multi-modal measures of hemodynamic performance in CDH, including systemic oxygen delivery, cardiac output and microcirculatory function.
Bedside assessment of hemodynamic function in congenital diaphragmatic hernia
Echocardiographic assessment should be accompanied by wider multi-modal physiological assessment, as in any infant with hemodynamic instability. Where possible, near infrared spectroscopy enables monitoring of systemic oxygen delivery and may precede (79) changes in plasma lactate or end organ injury (80, 81). Exploratory studies are combining cerebral NIRS with EEG to investigate neuro-cardiovascular coupling (82).
Invasive arterial monitoring is a requisite during hemodynamic instability for monitoring of blood pressure and arterial gas sampling. However, line position and the impact of intra and extracardiac shunts must be considered. Right to left ductal shunting will decrease pH, PaO2 and increase PaCO2 of post-ductal measurements, whereas a right-to-left atrial shunt, whilst uncommon in CDH, will also reduce pre-ductal oxygenation (71, 83).
Biomarkers of cardiac function in congenital diaphragmatic hernia
Plasma biomarkers may be useful in CDH to assess cardiac performance and pulmonary hypertension. These may be produced in response to hemodynamic compromise, or contribute to the primary pathways mediating CDH pathophysiology.
Natriuretic peptides brain natriuretic peptide (BNP) and its precursor N-terminal (NT) proBNP are established biomarkers in other pulmonary hypertensive diseases. BNP has a short half-life complicating measurement, but in CDH has been shown to be associated with pulmonary hypertension, need for ECMO, predictive of adverse outcome at 1 month of age, Table 4 (84, 85). Recently Avitabile et al. also demonstrated that BNP was associated with impaired RV strain after CDH repair, though not in the pre-operative period (61).
NT-proBNP has a longer half-life making clinical measurement more reliable, and has been observed to correlate with cardiac dysfunction, pulmonary hypertension, and ECMO use in case series and large registry analysis (86–88). However, neither NT-proBNP, nor high sensitivity troponin (hsTnT) correlated with outcomes in a recent RCT of ventilation modalities in CDH (89).
Other potential biomarkers include the vascular endothelial growth factor-A (VEGFA) and placental growth factor (PLGF), the ratio of which correlated with RV diastolic dysfunction in a pilot investigation (90). Also, the soluble receptor of advanced glycation end products (sRAGE) is a new potential mediator of endothelial dysfunction in CDH, associated with mortality, severity of PH, and adverse outcome in CDH (91). MicroRNA’s, a group of small non-coding RNA, are involved in the development and function of the lungs and the pulmonary vasculature in CDH, though to date their relationship to cardiac dysfunction in CDH remains unstudied (92–94).
The heart and the brain in congenital diaphragmatic hernia
Congenital diaphragmatic hernia is associated with neurodevelopmental impairment in a significant minority of affected people (95). Altered fetal brain development and postnatal brain injury may be contributing factors, and neuro-cardiovascular interactions may be central to these (82, 96).
Abnormalities in ventricular size and function may plausibly lead to alterations in cerebral blood flow, as is also suspected in infants with hypoplastic left heart syndrome (97).
This is itself a major topic for further discussion and investigation in CDH, beyond the scope of this review.
Management of cardiac dysfunction in congenital diaphragmatic hernia
Physiological approaches to managing the transition at birth
Recent animal and human feasibility studies have explored the use of physiologically based strategies for managing the transition at birth, combining lung recruitment with delayed cord clamping. These have demonstrated short-term improvements in pulmonary blood flow, pulmonary artery pressure and systemic blood pressure, though the impact on cardiac function per se has not been directly investigated (98–100). Randomized trials are in progress, but do not include cardiac function as a key outcome measure (101, 102).
Pharmacological therapies
Hemodynamic pharmacotherapy in CDH remains a challenging and unresolved issue. Historical approaches focused on maintaining systemic blood pressure and promoting pulmonary arterial vasodilation. The list of possible pharmacological therapies is ever-increasing, but with limited and often contradictory evidence leading to clinical confusion and risk of inappropriate use (103).
The current use of pulmonary vasodilators exemplifies this. Inhaled nitric oxide and sildenafil (in oral and intravenous formulations) are widely used in CDH patients with the intention of reducing pulmonary vascular resistance via endogenous nitric oxide pathways (104). However, only a minority of recipients demonstrate improved oxygenation, historic RCTs did not demonstrate improved outcomes, and recent registry analysis has suggested that iNO may be associated with increased mortality (105, 106).
Improved understanding of cardiac dysfunction may help address the uncertainty and anxiety around the use of these agents. The presence of LV dysfunction appears to be associated with non-response to iNO and sildenafil (107, 108). Possible mechanisms may be post-capillary hypertension secondary to LV dysfunction unresponsive to pre-capillary vasodilatation, or increased pulmonary blood flow exacerbating LV dysfunction (109).
The actions of other cardiovascular therapies which have been directly investigated in CDH are summarized in Table 5. Milrinone, a phospho-diesterase 3 inhibitor acting on the endogenous prostacyclin pathway appears well suited to treat both RV and LV dysfunction in CDH, for its inotropic, lusitropic and pulmonary vasodilating effects (110). In a case series of infants with pre-existent RV dysfunction milrinone use was associated with improved oxygenation and RV diastolic function (111). However, a recent retrospective analysis in mild to moderate CDH observed no effect on oxygenation or LV dimensions (112). An RCT of early milrinone use in CDH is ongoing but does not include cardiac dysfunction as an enrollment parameter (113).
Levosimendan a calcium-sensitizing drug is commonly used in infants with congenital heart defects in the setting of low cardiac output syndrome (114). There is preliminary evidence that levosimendan is also associated with improvement of right and left ventricular dysfunction and a decrease in the Vasopressor-Inotropic Score in CDH (115).
Vasopressin use in CDH has also been associated with improved blood pressure, and reduced pulmonary:systemic blood pressure ratio, though the specific impact on LV function remains unstudied (116). The utility of systemic vasoconstrictors in CDH is unclear, and may depend on individual patient pathophysiology. In the setting of severe LV dysfunction increasing afterload may exacerbate ventricular failure (117). However, if LV function is preserved and RV function impaired there may be theoretical benefits to increasing systemic vascular resistance; to improve RV coronary blood flow, augment LV function via the eponymous Anrep effect, and restore septal positioning (51, 118, 119). Further investigation is required to understand which patient phenotypes are likely to benefit, and which of these potential mechanisms are beneficial in the clinical setting.
Prostaglandin E1 (PGE1) use been proposed in early cardiovascular management in CDH to maintain ductal patency, as well as for its pulmonary vasodilating properties. In the setting of supra-systemic pulmonary hypertension a patent ductus permits right-to-left shunting, reducing the effective afterload on the RV, and supporting systemic blood flow, with theoretical benefits in the setting of both RV and/or LV dysfunction. Case series have demonstrated improvements in pulmonary artery pressure, oxygenation and LV function with PGE1 use, however the optimal timing, dosing and duration remains undefined (120–122).
From the investigations described here it has become clear that there is no universally effective single agent for treating cardiac function and pulmonary hypertension in CDH. However, that does not necessarily mean that current therapies are ineffective. Instead new therapeutic strategies may be required, based on characterization of individual patient phenotype, including the relative contributions of RV and LV dysfunction, pre and postcapillary PVR, and ductal patency. This will allow investigation of the efficacy of targeted, pathophysiology-based therapeutic approaches, rather than indiscriminate use of single agents.
Hydrocortisone is also frequently used in the management of infants with CDH as an adjunct treatment for hypotensive cardiovascular compromise, and appears to elicit useful increases in both systemic vascular resistance and cardiac output (123, 124). Up to two thirds of CDH cases may have biochemical evidence of adrenal insufficiency in the immediate pre and post-operative periods, as observed by Kamath et al. (125). Low cortisol levels were associated with need for higher levels of cardio-respiratory support including ECMO, but not survival.
Extra-corporeal membrane oxygenation and cardiac function in congenital diaphragmatic hernia
Early ventricular dysfunction is predictive of extra-corporeal membrane oxygenation (ECMO) use in CDH, and ECMO may be an important means of mechanically supporting the failing heart (62). However, there is minimal published data on longitudinal changes of ventricular function during the ECMO therapy. Additionally, decision-making regarding the relative benefits of venoarterial or venovenous ECMO based on the severity of concomitant ventricular dysfunction is at present supported only by clinician experience and opinion and not by published data (126). Bo et al. recently observed that ventricular function improves rapidly over the first days of life on ECMO support and may be monitored using biomarkers including NT-pro BNP (69).
Although cardiac dysfunction is commonly observed before and immediately after commencement of ECMO, it is rarely the cause of failure to wean support. As discussed above, severe LV dysfunction is a transient phenomenon and typically resolves in a matter of days. Nevertheless, ongoing right ventricular dysfunction after ECMO has been described, associated with persistent elevation of pulmonary artery pressure (61).
The two principal phenotypes responsible for ECMO weaning failure are first severe ventilation failure secondary to pulmonary hypoplasia, and second severe ongoing pulmonary hypertension. The latter may be due to irreversible developmental anomalies of pulmonary vasculature structure and function, decruitment of lung, or other factors such as ongoing infection.
Improved understanding of the relative contribution of cardiac dysfunction to individual pathophysiology before and during ECMO may be important for improved ECMO management strategies and help resolve ongoing uncertainties and controversies (127, 128).
Future priorities in congenital diaphragmatic hernia cardiac function therapy
To achieve effective use of these and other cardiovascular therapies in CDH future priorities should include:
• Standardized hemodynamic assessment parameters in CDH, incorporating routine functional echocardiography. Consensus definitions of pulmonary hypertension and cardiac function and dysfunction based on standardized measures.
• Pathophysiology-based treatment strategies rather than “one-size fits all” approach to use of single agents (1).
• Routine assessment of cardiac function in studies of hemodynamic therapies, as in the recent CoDiNOS trial of IV sildenafil (73).
• Investigation of the relationship between LV performance and response to pulmonary vasodilator therapies.
• Improved understanding of cardiac function to guide individualized ECMO strategies including improved patient selection and timing of repair.
• Investigation of whether improvement in cardiac function translates to improved short and long-term clinical outcomes.
Surgical repair and cardiac function in congenital diaphragmatic hernia
Current international guidelines recommend delaying CDH repair until physiological stability including normalized blood pressure and lactate have been achieved, but do not specifically reference cardiac function (129). RV function may deteriorate within 72 h of surgical repair (49). In a recent cohort Avitabile et al. observed that RV strain improved in the recovery phase after surgery, though over 50% of cases had ongoing reduction in RV strain (61). Chronic elevation of PVR, exacerbated by surgery, may contribute to this ongoing RV dysfunction. Conversely in the LV, Tanaka et al. have observed improved LV diastolic wall strain following early CDH repair, potentially as a result of removing the compressive action of the hernia (130). Further investigation is required to fully understand the impacts of the herniating abdominal contents, anesthesia and the surgical techniques on cardiac function and hemodynamics in CDH.
Cardiac loading conditions may also be altered in the post-operative period. Chylothorax occurs in 5% of CDH cases after repair or ECMO cannulation, possibly resulting from superior vena cava obstruction (131, 132). The associated reduction in preload may in turn affect ventricular function and cardiac output.
Long-term cardiac function in congenital diaphragmatic hernia
The natural history of long-term cardiac function in CDH is not well understood.
Kraemer et al. observed that pulmonary hypertension largely resolves in childhood in CDH survivors (133). Similarly, a recent literature review identified highly variable rates of pulmonary hypertension (4.5–38%) in CDH survivors over 2 years old, and diminishing rates by 5 years of age (134). However, the limitations of echocardiographic techniques make accurate non-invasive assessment of pulmonary artery pressure challenging. Ventricular function may be a more sensitive measure of ongoing changes in RV loading conditions and the systemic circulation, as demonstrated in non-CDH pulmonary hypertensive diseases (135–137).
Analysis in our own center demonstrated abnormal RV and LV strain in 70% and 44% of CDH patients respectively at the time of neonatal discharge (Massolo et al., unpublished data). Furthermore, Egan et al. observed abnormal RV strain in CDH survivors at a median of age of 6 years (138). Altered cardiac function, in conjunction with ongoing respiratory compromise, may conceivably contribute to functional exercise restriction in a minority of CDH patients (139).
In survivors of preterm birth there is increasing evidence that altered ventriculo-arterial interactions may contribute to adult cardiovascular dysfunction, raising potential concerns that similar pathologies might occur in CDH (140–142). Prospective, longitudinal studies are now required to understand lifelong patterns and functional significance of cardiac function in CDH.
Cardiac catheterization may be an important adjunct for longitudinal hemodynamic assessment in CDH. Limited case series have demonstrated the ability to directly assess pulmonary artery and intra-cardiac pressures, including in the left heart, ventricular outputs, occult shunts, additional congenital lesions, and responsiveness to therapies including pulmonary vasodilators in CDH (143, 144). Earlier, standardized use of cardiac catheterization in cases of CDH with ongoing hemodynamic compromise, is an important future consideration.
In young adults who have been born prematurely and have low adaptive capacity during exercise cardiac catheterization has revealed evidence of pulmonary hypertension (145). This raises the question of whether catheterization may also be indicated in CDH survivors who have ongoing evidence of cardio-respiratory exercise intolerance (146, 147).
Discussion
There is increasing evidence and recognition of the heart’s contribution to disease pathophysiology in CDH (68). However, the “knowns” are outweighed by ongoing uncertainties or “unknowns,” as summarized in Table 6. Addressing these may be critical to developing new therapeutic approaches and improved outcomes.
Beginning with the fetus, further investigation is required to investigate the nature and mechanisms of fetal LV hypoplasia at a cellular, metabolic, genetic and morphometric level, as well as the functional significance for fetal hemodynamics including cerebral perfusion. The relationship between fetal cardiac dimensions and function, early postnatal function and outcomes is also a priority that may identify improved prenatal predictors and postnatal therapeutic strategies. Assessment of any fetal therapies should include the impact on the heart, as well as the lungs and pulmonary vasculature.
Though RV and LV function have been characterized in early postnatal life, a deeper physiological understanding of underlying ventricular interdependence, ventriculo-arterial and cardio-respiratory interactions at this time will enable more informed, physiology-based therapeutic approaches.
Use of cardiovascular pharmacotherapies in CDH remains a challenging area. Ongoing trials of milrinone and pulmonary vasodilators may shed some light (73, 113). Hemodynamic assessment should be incorporated in all future interventional studies in CDH. To do so requires urgent consensus on what to measure, how, and when, as well as standardized definitions of cardiac function and pulmonary hypertension. This will enable the robust, multi-center data collection and analysis required in this rare disease.
Investigating new therapeutic paradigms may also be important. Rather than a “one size fits all” approach, improved assessment of individual patient pathophysiological phenotype and the relative contributions of cardiac function, alongside pulmonary hypertension and ventilatory function, may lead to improved, targeted use of therapies including pulmonary vasodilators, cardiotropes, and vasopressors. This approach may also improve ECMO strategies addressing thorny uncertainties around patient selection and timing of repair both on and off ECMO.
The relationship between the heart and the brain in CDH is also a critical area for research, to understand the neuro-cardiovascular coupling mechanisms contributing to fetal brain development and postnatal injury in CDH, and their relationship to long-term neurodevelopment.
Finally, the long-term nature of cardiovascular function in CDH survivors is another priority, to determine whether longer-term changes in cardiac morphology and function, or abnormalities of the systemic or pulmonary circulation, impact functional status later in life.
Addressing each of these uncertainties will require innovative approaches. First, in terms of methodology combining human clinical investigation, animal studies, and novel cellular and organoid models, as well as new imaging modalities including advanced functional echocardiography and MR (148–150). Second, by applying learning from other related conditions, including congenital heart disease and non-CDH pulmonary hypertensive disease. Third, and above all, collaboration is required to share knowledge, consolidate expertise and capabilities between researchers, clinicians and people with CDH themselves.
We hope that this review can act as a call to action, highlighting the importance of the heart and the next steps to progress our understanding, develop new therapies, and improve outcomes in CDH.
Author contributions
NP, AM, UK, and FK contributed to the concept, outline of the manuscript, reviewed drafts, and approved the final manuscript. NP provided co-ordination of each authors’ contributions. All authors contributed to the article and approved the submitted version.
Acknowledgments
We are grateful to Michael Cooper for his assistance with the figure.
Conflict of interest
The authors declare that the research was conducted in the absence of any commercial or financial relationships that could be construed as a potential conflict of interest.
Publisher’s note
All claims expressed in this article are solely those of the authors and do not necessarily represent those of their affiliated organizations, or those of the publisher, the editors and the reviewers. Any product that may be evaluated in this article, or claim that may be made by its manufacturer, is not guaranteed or endorsed by the publisher.
References
1. Patel N, Massolo AC, Kipfmueller F. Congenital diaphragmatic hernia-associated cardiac dysfunction. Semin Perinatol. (2020) 44:151168. doi: 10.1053/j.semperi.2019.07.007
2. Montalva L, Lauriti G, Zani A. Congenital heart disease associated with congenital diaphragmatic hernia: a systematic review on incidence, prenatal diagnosis, management, and outcome. J Pediatr Surg. (2019) 54:909–19. doi: 10.1016/j.jpedsurg.2019.01.018
3. Fraser CD, Hill KD, Wallace A, Chiswell K, Zhou X, Jelin EB, et al. The prevalence and impact of congenital diaphragmatic hernia among patients undergoing surgery for congenital heart disease. Semin Thorac Cardiovasc Surg. (2019) 31:69–77. doi: 10.1053/j.semtcvs.2018.09.014
4. Graziano JN. Cardiac anomalies in patients with congenital diaphragmatic hernia and their prognosis: a report from the congenital diaphragmatic hernia study group. J Pediatr Surg. (2005) 40:1045–9. doi: 10.1016/j.jpedsurg.2005.03.025
5. Kardon G, Ackerman KG, McCulley DJ, Shen Y, Wynn J, Shang L, et al. Congenital diaphragmatic hernias: from genes to mechanisms to therapies. Dis Model Mech. (2017) 10:955–70. doi: 10.1242/dmm.028365
6. Losty PD, Connell MG, Freese R, Laval S, Okoye BO, Smith A, et al. Cardiovascular malformations in experimental congenital diaphragmatic hernia. J Pediatr Surg. (1999) 34:1352–8. doi: 10.1016/S0022-3468(99)90152-5
7. Yu L, Hernan RR, Wynn J, Chung WK. The influence of genetics in congenital diaphragmatic hernia. Semin Perinatol. (2020) 44:151169. doi: 10.1053/j.semperi.2019.07.008
8. Brosens E, Peters NCJ, van Weelden KS, Bendixen C, Brouwer RWW, Sleutels F, et al. Unraveling the genetics of congenital diaphragmatic hernia: an ongoing challenge. Front Pediatr. (2022) 9:800915. doi: 10.3389/fped.2021.800915
9. Gray BW, Fifer CG, Hirsch JC, Tochman SW, Drongowski RA, Mychaliska GB, et al. Contemporary outcomes in infants with congenital heart disease and bochdalek diaphragmatic hernia. Ann Thorac Surg. (2013) 95:929–34. doi: 10.1016/j.athoracsur.2012.07.010
10. Balks J, Mueller A, Herberg U, Geipel A, Gembruch U, Schroeder L, et al. Therapy and outcome of neonates with congenital diaphragmatic hernia and congenital heart defects. Z Geburtshilfe Neonatol. (2021) 225:432–40. doi: 10.1055/a-1392-1460
11. Balduf K, Kumar TKS, Boston U, Sathanandam S, Lee MV, Jancelewicz T, et al. Improved outcomes in management of hypoplastic left heart syndrome associated with congenital diaphragmatic hernia: an algorithmic approach. Semin Thorac Cardiovasc Surg. (2018) 30:191–6. doi: 10.1053/j.semtcvs.2018.02.010
12. Losty PD, Vanamo K, Rintala RJ, Donahoe PK, Schnitzer JJ, Lloyd DA. Congenital diaphragmatic hernia – Does the side of the defect influence the incidence of associated malformations? J Pediatr Surg. (1998) 33:507–10. doi: 10.1016/S0022-3468(98)90099-9
13. Siebert JR, Haas JE, Beckwith JB. Left ventricular hypoplasia in congenital diaphragmatic hernia. J Pediatr Surg. (1984) 19:567–71. doi: 10.1016/S0022-3468(84)80105-0
14. Byrne FA, Keller RL, Meadows J, Miniati D, Brook MM, Silverman NH, et al. Severe left diaphragmatic hernia limits size of fetal left heart more than does right diaphragmatic hernia. Ultrasound Obstet Gynecol. (2015) 46:688–94. doi: 10.1002/uog.14790
15. Vogel M, McElhinney DB, Marcus E, Morash D, Jennings RW, Tworetzky W. Significance and outcome of left heart hypoplasia in fetal congenital diaphragmatic hernia. Ultrasound Obstet Gynecol. (2010) 35:310–7. doi: 10.1002/uog.7497
16. Baumgart S, Paul JJ, Huhta JC, Katz AL, Paul KE, Spettell C, et al. Cardiac malposition, redistribution of fetal cardiac output, and left heart hypoplasia reduce survival in neonates with congenital diaphragmatic hernia requiring extracorporeal membrane oxygenation. J Pediatr. (1998) 133:57–62. doi: 10.1016/s0022-3476(98)70178-7
17. VanderWall KJ, Kohl T, Adzick NS, Silverman NH, Hoffman JI, Harrison MR. Fetal diaphragmatic hernia: echocardiography and clinical outcome. J Pediatr Surg. (1997) 32:223–5. doi: 10.1016/S0022-3468(97)90183-4
18. Massolo AC, Romiti A, Viggiano M, Vassallo C, Ledingham MA, Lanzone A, et al. Fetal cardiac dimensions in congenital diaphragmatic hernia: relationship with gestational age and postnatal outcomes. J Perinatol. (2021) 41:1651–9. doi: 10.1038/s41372-021-00986-y
19. Thébaud B, Azancot A, de Lagausie P, Vuillard E, Ferkadji L, Benali K, et al. Congenital diaphragmatic hernia: antenatal prognostic factors. Does cardiac ventricular disproportion in utero predict outcome and pulmonary hypoplasia? Intensive Care Med. (1997) 23:1062–9. doi: 10.1007/s001340050457
20. Yamoto M, Inamura N, Terui K, Nagata K, Kanamori Y, Hayakawa M, et al. Echocardiographic predictors of poor prognosis in congenital diaphragmatic hernia. J Pediatr Surg. (2016) 51:1926–30. doi: 10.1016/j.jpedsurg.2016.09.014
21. Dekoninck P, Richter J, van Mieghem T, van Schoubroeck D, Allegaert K, de Catte L, et al. Cardiac assessment in fetuses with right-sided congenital diaphragmatic hernia: case-control study. Ultrasound Obstet Gynecol. (2014) 43:432–6. doi: 10.1002/uog.12561
22. Mardy C, Blumenfeld YJ, Arunamata AA, Girsen AI, Sylvester KG, Halabi S, et al. In fetuses with congenital lung masses, decreased ventricular and atrioventricular valve dimensions are associated with lesion size and clinical outcome. Prenat Diagn. (2020) 40:206–15. doi: 10.1002/pd.5612
23. Bravo-Valenzuela NJM, Peixoto AB, Araujo Júnior E. Prenatal diagnosis of total anomalous pulmonary venous connection: 2D and 3D echocardiographic findings. J Clin Ultrasound. (2021) 49:240–7. doi: 10.1002/jcu.22973
24. Stressig R, Fimmers R, Eising K, Gembruch U, Kohl T. Preferential streaming of the ductus venosus and inferior caval vein towards the right heart is associated with left heart underdevelopment in human fetuses with left-sided diaphragmatic hernia. Heart. (2010) 96:1564–8. doi: 10.1136/hrt.2010.196550
25. Degenhardt J, Enzensberger C, Tenzer A, Kaweckir A, Kohl T, Widriani E, et al. Myocardial function pre- and post-fetal endoscopic tracheal occlusion (FETO) in fetuses with left-sided moderate to severe congenital diaphragmatic hernia. Ultraschall Med. (2017) 38:65–70. doi: 10.1055/s-0041-108501
26. Dekoninck P, Jan D, Mieghem T, Richter J, Deprest J. Speckle tracking echocardiography in fetuses diagnosed with congenital diaphragmatic hernia. Prenat Diagn. (2014) 34:1262–7.
27. van Mieghem T, Gucciardo L, Doné E, van Schoubroeck D, Graatsma EM, Visser GHA, et al. Left ventricular cardiac function in fetuses with congenital diaphragmatic hernia and the effect of fetal endoscopic tracheal occlusion. Ultrasound Obstet Gynecol. (2009) 34:424–9. doi: 10.1002/uog.7340
28. Lemini MC, Josep M, Montiel JG. Characterizing cardiac dysfunction in fetuses with left congenital diaphragmatic hernia. Prenat Diagn. (2018) 38:422–7. doi: 10.1002/pd.5253
29. Kaya B, Tayyar A, Sezer S, Kaya S. The assessment of cardiac function with tissue Doppler imaging in fetuses with congenital diaphragmatic hernia. J Mater Fetal Neonatal Med. (2020) 33:1233–8. doi: 10.1080/14767058.2019.1674806
30. Coffman ZJ, McGahren ED, Vergales BD, Saunders CH, Vergales JE. The effect of congenital diaphragmatic hernia on the development of left-sided heart structures. Cardiol Young. (2019) 29:813–8. doi: 10.1017/S1047951119000891
31. Kailin JA, Dhillon GS, Maskatia SA, Cass DL, Shamshirsaz AA, Mehollin-ray AR, et al. Fetal left-sided cardiac structural dimensions in left-sided congenital diaphragmatic hernia – association with severity and impact on postnatal outcomes. Prenat Diagn. (2017) 37:502–9. doi: 10.1002/pd.5045
32. Yamoto M, Tanaka Y, Fukumoto K, Miyake H, Nakajima H, Koyama M, et al. Cardiac fetal ultrasonographic parameters for predicting outcomes of isolated left-sided congenital diaphragmatic hernia. J Pediatr Surg. (2015) 50:2019–24. doi: 10.1016/j.jpedsurg.2015.08.016
33. Schwartz SM, Vermilion RP, Hirschl RB. Evaluation of left ventricular mass in children with left-sided congenital diaphragmatic hernia. J Pediatr. (1994) 125:447–51. doi: 10.1016/s0022-3476(05)83293-7
34. Teramoto H, Puri P. Gene expression of insulin-like growth factor-1 and epidermal growth factor is downregulated in the heart of rats with nitrofen-induced diaphragmatic hernia. Pediatr Surg Int. (2001) 17:284–7. doi: 10.1007/s003830100595
35. Guarino N, Shima H, Puri P. The hypoplastic heart in congenital diaphragmatic hernia: reduced expression of basic fibroblast growth factor and platelet-derived growth factor. Pediatr Surg Int. (2000) 16:243–6. doi: 10.1007/s003830050737
36. Zhaorigetu S, Gupta VS, Jin D, Harting MT. Cardiac energy metabolism may play a fundamental role in congenital diaphragmatic hernia-associated ventricular dysfunction. J Mol Cell Cardiol. (2021) 157:14–6. doi: 10.1016/j.yjmcc.2021.04.001
37. Pelizzo G, Bussani R, Zandonà L, Custrin A, Bellieni CV, de Silvestri A, et al. Cardiac adaptation to severe congenital diaphragmatic hernia. Fetal Pediatr Pathol. (2016) 35:10–20. doi: 10.3109/15513815.2015.1122125
38. Karamanoukian HL, Glick PL, Wilcox DT, O’Toole SJ, Rossman JE, Azizkhan RG. Pathophysiology of congenital diaphragmatic hernia XI: anatomic and biochemical characterization of the heart in the fetal lamb CDH model. J Pediatr Surg. (1995) 30:925–8. doi: 10.1016/0022-3468(95)90314-3
39. Tannuri U. Heart hypoplasia in an animal model of congenital diaphragmatic hernia. Rev Hosp Clín. (2001) 56:173–8. doi: 10.1590/s0041-87812001000600003
40. Grossfeld P, Nie S, Lin L, Wang L, Anderson RH. Hypoplastic left heart syndrome: a new paradigm for an old disease? J Cardiovasc Dev Dis. (2019) 6:10. doi: 10.3390/jcdd6010010
41. Deprest JA, Nicolaides KH, Benachi A, Gratacos E, Ryan G, Persico N, et al. Randomized trial of fetal surgery for severe left diaphragmatic hernia. N Engl J Med. (2021) 385:107–18. doi: 10.1056/NEJMoa2027030
42. Marulanda K, Tsihlis ND, McLean SE, Kibbe MR. Emerging antenatal therapies for congenital diaphragmatic hernia-induced pulmonary hypertension in preclinical models. Pediatr Res. (2021) 89:1641–9. doi: 10.1038/s41390-020-01191-x
43. Luong C, Rey-Perra J, Vadivel A, Gilmour G, Sauve Y, Koonen D, et al. Antenatal sildenafil treatment attenuates pulmonary hypertension in experimental congenital diaphragmatic hernia. Circulation. (2011) 123:2120–31. doi: 10.1161/CIRCULATIONAHA.108.845909
44. Russo FM, Toelen J, Eastwood MP, Jimenez J, Miyague AH, Velde G. Transplacental sildenafil rescues lung abnormalities in the rabbit model of diaphragmatic hernia. Thorax. (2016) 71:517–25. doi: 10.1136/thoraxjnl-2015-207949
45. Kashyap AJ, Dekoninck PLJ, Rodgers KA, Thio M, Mcgillick EV, Amberg BJ, et al. Antenatal sildenafil treatment improves neonatal pulmonary hemodynamics and gas exchange in lambs with diaphragmatic hernia. Ultrasound Obstet Gynecol. (2019) 54:506–16. doi: 10.1002/uog.20415
46. Smith GCS. The STRIDER trial: one step forward, one step back. Lancet Child Adolesc Health. (2018) 2:80–1. doi: 10.1016/S2352-4642(17)30176-1
47. Lee FT, Marini D, Seed M, Sun L. Maternal hyperoxygenation in congenital heart disease. Transl Pediatr. (2021) 10:2197–209
48. Enzensberger C, Axt-Fliedner R, Degenhardt J, Kawecki A, Tenzer A, Kohl T, et al. Pulmonary vasoreactivity to materno-fetal hyperoxygenation testing in fetuses with hypoplastic left heart. Ultraschall Med. (2016) 37:195–200. doi: 10.1055/s-0034-1385668
49. Moenkemeyer F, Patel N. Right ventricular diastolic function measured by tissue doppler imaging predicts early outcome in congenital diaphragmatic hernia. Pediatr Critic Care Med. (2014) 15:49–55. doi: 10.1097/PCC.0b013e31829b1e7a
50. Harting MT. Congenital diaphragmatic hernia-associated pulmonary hypertension. Semin Pediatr Surg. (2017) 26:147–53. doi: 10.1053/j.sempedsurg.2017.04.008
51. Brooks H, Kirk ES, Vokonas PS, Urschel CW, Sonnenblick EH. Performance of the right ventricle under stress: relation to right coronary flow. J Clin Invest. (1971) 50:2176–83. doi: 10.1172/JCI106712
52. Aggarwal S, Stockmann P, Klein MD, Natarajan G. Echocardiographic measures of ventricular function and pulmonary artery size: prognostic markers of congenital diaphragmatic hernia? J Perinatol. (2011) 31:561–6. doi: 10.1038/jp.2011.3
53. Patel N, Mills JF, Cheung MMH. Assessment of right ventricular function using tissue doppler imaging in infants with pulmonary hypertension. Neonatology. (2009) 96:193–9. doi: 10.1159/000215585
54. Patel N, Mills JF, Cheung MMH. Use of the myocardial performance index to assess right ventricular function in infants with pulmonary hypertension. Pediatr Cardiol. (2009) 30:133–7. doi: 10.1007/s00246-008-9285-1
55. Altit G, Bhombal S, van Meurs K, Tacy TA. Diminished cardiac performance and left ventricular dimensions in neonates with congenital diaphragmatic hernia. Pediatr Cardiol. (2018) 39:993–1000. doi: 10.1007/s00246-018-1850-7
56. Nagiub M, Klein J, Gullquist S. Echocardiography derived pulmonary artery capacitance and right ventricular outflow velocity time integral on first day of life can predict survival in congenital diaphragmatic hernia. Prog Pediatr Cardiol. (2018) 48:107–10. doi: 10.1016/j.ppedcard.2018.01.001
57. Ferguson DM, Gupta VS, Lally PA, Luco M, Tsao K, Lally KP, et al. Early, postnatal pulmonary hypertension severity predicts inpatient outcomes in congenital diaphragmatic hernia. Neonatology. (2021) 118:147–54. doi: 10.1159/000512966
58. Lusk LA, Wai KC, Moon-Grady AJ, Steurer MA, Keller RL. Persistence of pulmonary hypertension by echocardiography predicts short-term outcomes in congenital diaphragmatic hernia. J Pediatr. (2015) 166:251–6. doi: 10.1016/j.jpeds.2014.10.024
59. Aggarwal S, Stockman PT, Klein MD, Natarajan G. The right ventricular systolic to diastolic duration ratio: a simple prognostic marker in congenital diaphragmatic hernia? Acta Paediatr. (2011) 100:1315–8. doi: 10.1111/j.1651-2227.2011.02302.x
60. Altit G, Bhombal S, van Meurs K, Tacy TA. Ventricular performance is associated with need for extracorporeal membrane oxygenation in newborns with congenital diaphragmatic hernia. J Pediatr. (2017) 191:28–34.e1. doi: 10.1016/j.jpeds.2017.08.060
61. Avitabile CM, Wang Y, Zhang X, Griffis H, Saavedra S, Adams S, et al. Right ventricular strain, brain natriuretic peptide, and mortality in congenital diaphragmatic hernia. Ann Am Thorac Soc. (2020) 17:1431–9. doi: 10.1513/AnnalsATS.201910-767OC
62. Patel N, Lally PA, Kipfmueller F, Massolo AC, Luco M, van Meurs KP, et al. Ventricular dysfunction is a critical determinant of mortality in congenital diaphragmatic hernia. Am J Respir Crit Care Med. (2019) 200:1522–30. doi: 10.1164/rccm.201904-0731OC
63. Patel N, Massolo AC, Paria A, Stenhouse EJ, Hunter L, Finlay E, et al. Early postnatal ventricular dysfunction is associated with disease severity in patients with congenital diaphragmatic hernia. J Pediatr. (2018) 203:400–7. doi: 10.1016/j.jpeds.2018.07.062
64. Massolo AC, Paria A, Hunter L, Finlay E, Davis CF, Patel N. Ventricular dysfunction, interdependence, and mechanical dispersion in newborn infants with congenital diaphragmatic hernia. Neonatology. (2019) 116:68–75. doi: 10.1159/000499347
65. Kinsella J, Steinhorn R, Mullen M, Hopper R, Keller R, Ivy D, et al. The left ventricle in congenital diaphragmatic hernia: implications for the management of pulmonary hypertension. J Pediatr. (2018) 197:17–22. doi: 10.1016/j.jpeds.2018.02.040
66. Gaffar S, Ellini AR, Ahmad I, Chen Y, Ashrafi AH. Left ventricular cardiac output is a reliable predictor of extracorporeal life support in neonates with congenital diaphragmatic hernia. J Perinatol. (2019) 39:648–53. doi: 10.1038/s41372-019-0348-3
67. Duy D, Patel N, Harting MT, Lally KP, Lally PA, Buchmiller TL. Early left ventricular dysfunction and severe pulmonary hypertension predict adverse outcomes in “low-risk” congenital diaphragmatic hernia. Pediatr Crit Care Med. (2020) 21:637–46. doi: 10.1097/PCC.0000000000002318
68. Patel N, Kipfmueller F. Cardiac dysfunction in congenital diaphragmatic hernia: pathophysiology, clinical assessment, and management. Semin Pediatr Surg. (2017) 26:154–8. doi: 10.1053/j.sempedsurg.2017.04.001
69. Bo B, Balks J, Gries K, Holdenrieder S, Mueller A, Kipfmueller F. Increased N-terminal Pro-B-Type natriuretic peptide during extracorporeal life support is associated with poor outcome in neonates with congenital diaphragmatic hernia. J Pediatr. (2021) 241:83–9. doi: 10.1016/j.jpeds.2021.09.034
70. Rosenkranz S, Gibbs JSR, Wachter R, de Marco T, Vonk-Noordegraaf A, Vachiéry JL. Left ventricular heart failure and pulmonary hypertension. Eur Heart J. (2016) 37:942–54. doi: 10.1093/eurheartj/ehv512
71. Wehrmann M, Patel SS, Haxel C, Cassidy C, Howley L, Cuneo B, et al. Implications of atrial-level shunting by echocardiography in newborns with congenital diaphragmatic hernia. J Pediatr. (2020) 219:43–7. doi: 10.1016/j.jpeds.2019.12.037
72. Rudski LG, Afilalo J. The blind men of indostan and the elephant in the echo lab. J Am Soc Echocardiogr. (2012) 25:714–7. doi: 10.1016/j.echo.2012.05.022
73. Cochius-Den Otter S, Schaible T, Greenough A, van Heijst A, Patel N, Allegaert K, et al. The CoDiNOS trial protocol: an international randomised controlled trial of intravenous sildenafil versus inhaled nitric oxide for the treatment of pulmonary hypertension in neonates with congenital diaphragmatic hernia. BMJ Open. (2019) 9:e032122. doi: 10.1136/bmjopen-2019-032122
74. Rudski LG, Lai WW, Afilalo J, Hua L, Handschumacher MD, Chandrasekaran K, et al. Guidelines for the echocardiographic assessment of the right heart in adults: a report from the american society of echocardiography. J Am Soc Echocardiogr. (2010) 23:685–713. doi: 10.1016/j.echo.2010.05.010
75. Tissot C, Singh Y, Sekarski N. Echocardiographic evaluation of ventricular function-for the neonatologist and pediatric intensivist. Front Pediatr. (2018) 6:79. doi: 10.3389/fped.2018.00079
76. de Boode WP, Singh Y, Molnar Z, Schubert U, Savoia M, Sehgal A, et al. Application of neonatologist performed echocardiography in the assessment and management of persistent pulmonary hypertension of the newborn. Pediatr Res. (2018) 84:68–77. doi: 10.1038/s41390-018-0082-0
77. Hansmann G, Koestenberger M, Alastalo TP, Apitz C, Austin ED, Bonnet D, et al. 2019 updated consensus statement on the diagnosis and treatment of pediatric pulmonary hypertension: the european pediatric pulmonary vascular disease network (EPPVDN), endorsed by AEPC, ESPR and ISHLT. J Heart Lung Transplant. (2019) 38:879–901. doi: 10.1016/j.healun.2019.06.022
78. Abman SH, Hansmann G, Archer SL, Ivy DD, Adatia I, Chung WK, et al. Pediatric pulmonary hypertension. Circulation. (2015) 132:2037–99. doi: 10.1161/CIR.0000000000000329
79. Dempsey EM, El-Khuffash AF. Objective cardiovascular assessment in the neonatal intensive care unit. Arch Dis Child Fetal Neonatal Ed. (2018) 103:F72–7. doi: 10.1136/archdischild-2017-313837
80. Lau PE, Cruz S, Garcia-Prats J, Cuevas M, Rhee C, Cass DL, et al. Use of renal near-infrared spectroscopy measurements in congenital diaphragmatic hernia patients on ECMO. J Pediatr Surg. (2017) 52:689–92. doi: 10.1016/j.jpedsurg.2017.01.015
81. Ryan A, Gilhooley M, Patel N, Reynolds BC. Prevalence of acute kidney injury in neonates with congenital diaphragmatic hernia. Neonatology. (2020) 117:88–94. doi: 10.1159/000503293
82. Hendrikx D, Costerus SA, Katrin Z, Perez-Ortiz A, Caicedo Dorado A, van Huffel S, et al. Neurocardiovascular coupling in congenital diaphragmatic hernia patients undergoing different types of surgical treatment. Eur J Anaesthesiol. (2021) 39:662–72. doi: 10.1097/EJA.0000000000001642
83. Gien J, Kinsella JP. Differences in preductal and postductal arterial blood gas measurements in infants with severe congenital diaphragmatic hernia. Arch Dis Child Fetal Neonatal Ed. (2016) 101:F314–8. doi: 10.1136/archdischild-2014-307714
84. Partridge EA, Hanna BD, Rintoul NE, Herkert L, Flake AW, Adzick NS, et al. Brain-type natriuretic peptide levels correlate with pulmonary hypertension and requirement for extracorporeal membrane oxygenation in congenital diaphragmatic hernia. J Pediatr Surg. (2015) 50:263–6. doi: 10.1016/j.jpedsurg.2014.11.009
85. Guslits E, Steurer MA, Nawaytou H, Keller RL. Longitudinal B-Type natriuretic peptide levels predict outcome in infants with congenital diaphragmatic hernia. J Pediatr. (2021) 229:191–8. doi: 10.1016/j.jpeds.2020.09.064
86. Baptista MJ, Rocha G, Clemente F, Azevedo LF, Tibboel D, Leite-Moreira AF, et al. N-terminal-pro-B type natriuretic peptide as a useful tool to evaluate pulmonary hypertension and cardiac function in CDH infants. Neonatology. (2008) 94:22–30. doi: 10.1159/000112641
87. Heindel K, Holdenrieder S, Patel N, Bartmann P, Schroeder L, Berg C, et al. Early postnatal changes of circulating N-terminal-pro-B-type natriuretic peptide in neonates with congenital diaphragmatic hernia. Early Hum Dev. (2020) 146:105049. doi: 10.1016/j.earlhumdev.2020.105049
88. Gupta VS, Patel N, Kipfmueller F, Lally PA, Lally KP, Harting MT. Elevated proBNP levels are associated with disease severity, cardiac dysfunction, and mortality in congenital diaphragmatic hernia. J Pediatr Surg. (2021) 56:1214–9. doi: 10.1016/j.jpedsurg.2021.02.042
89. Snoek KG, Kraemer US, Ten Kate CA, Greenough A, van Heijst A, Capolupo I, et al. High-Sensitivity troponin T and N-terminal pro-brain natriuretic peptide in prediction of outcome in congenital diaphragmatic hernia: results from a multicenter, randomized controlled trial. J Pediatr. (2016) 173:245–9. doi: 10.1016/j.jpeds.2016.03.026
90. Patel N, Moenkemeyer F, Germano S, Cheung MMH. Plasma vascular endothelial growth factor A and placental growth factor: novel biomarkers of pulmonary hypertension in congenital diaphragmatic hernia. Am J Physiol Lung Cell Mol Physiol. (2015) 308:L378–83. doi: 10.1152/ajplung.00261.2014
91. Kipfmueller F, Heindel K, Geipel A, Berg C, Bartmann P, Reutter H, et al. Expression of soluble receptor for advanced glycation end products is associated with disease severity in congenital diaphragmatic hernia. Am J Physiol Lung Cell Mol Physiol. (2019) 316:L1061–9. doi: 10.1152/ajplung.00359.2018
92. Herrera-Rivero M, Zhang R, Heilmann-Heimbach S, Mueller A, Bagci S, Dresbach T, et al. Circulating microRNAs are associated with pulmonary hypertension and development of chronic lung disease in congenital diaphragmatic hernia. Sci Rep. (2018) 8:10735. doi: 10.1038/s41598-018-29153-8
93. Eastwood MP, Deprest J, Russo FM, Wang H, Mulhall D, Iwasiow B, et al. MicroRNA 200b is upregulated in the lungs of fetal rabbits with surgically induced diaphragmatic hernia. Prenat Diagn. (2018) 38:645–53. doi: 10.1002/pd.5318
94. Khoshgoo N, Kholdebarin R, Pereira-Terra P, Mahood TH, Falk L, Day CA, et al. Prenatal microRNA miR-200b therapy improves nitrofen-induced pulmonary hypoplasia associated with congenital diaphragmatic hernia. Ann Surg. (2019) 269:979–87. doi: 10.1097/SLA.0000000000002595
95. van der Veeken L, Vergote S, Kunpalin Y, Kristensen K, Deprest J, Bruschettini M. Neurodevelopmental outcomes in children with isolated congenital diaphragmatic hernia: a systematic review and meta-analysis. Prenat Diagn. (2021) 42:318–29. doi: 10.1002/pd.5916
96. Hassan M, Patel D, LaRusso K, Koclas L, Smith-Morin OTM, Shapiro AJ, et al. Risk stratification helps identify congenital diaphragmatic hernia (CDH) infants in need of formal neurodevelopmental assessment: observations from a structured, interdisciplinary long-term follow-up clinic. J Pediatr Surg. (2022) 57:846–50. doi: 10.1016/j.jpedsurg.2021.12.048
97. Lloyd DFA, Rutherford MA, Simpson JM, Razavi R. The neurodevelopmental implications of hypoplastic left heart syndrome in the fetus. Cardiol Young. (2017) 27:217–23. doi: 10.1017/S1047951116001645
98. Foglia EE, Ades A, Hedrick HL, Rintoul N, Munson DA, Moldenhauer J, et al. Initiating resuscitation before umbilical cord clamping in infants with congenital diaphragmatic hernia: a pilot feasibility trial. Arch Dis Child Fetal Neonatal Ed. (2020) 105:322–6. doi: 10.1136/archdischild-2019-317477
99. Lefebvre C, Rakza T, Weslinck N, Vaast P, Houfflin-debarge V, Mur S, et al. Feasibility and safety of intact cord resuscitation in newborn infants with congenital diaphragmatic hernia (CDH). Resuscitation. (2017) 120:20–5. doi: 10.1016/j.resuscitation.2017.08.233
100. Kashyap AJ, Hodges RJ, Thio M, Rodgers KA, Amberg BJ, McGillick EV, et al. Physiologically based cord clamping improves cardiopulmonary haemodynamics in lambs with a diaphragmatic hernia. Arch Dis Child Fetal Neonatal Ed. (2020) 105:18–25. doi: 10.1136/archdischild-2019-316906
101. Le Duc K, Mur S, Rakza T, Boukhris MR, Rousset C, Vaast P, et al. Efficacy of intact cord resuscitation compared to immediate cord clamping on cardiorespiratory adaptation at birth in infants with isolated congenital diaphragmatic hernia (Chic). Children. (2021) 8:339. doi: 10.3390/children8050339
102. Dekoninck PLJ. Physiological-based cord clamping versus immediate cord clamping for infants born with congenital diaphragmatic hernia: a multicentre, randomised controlled trial. BMJ Open. (2022) 12:e054808. doi: 10.1136/bmjopen-2021-054808
103. de Bie FR, Avitabile CM, Joyeux L, Hedrick HL, Russo FM, Basurto D, et al. Neonatal and fetal therapy of congenital diaphragmatic hernia-related pulmonary hypertension. Arch Dis Child Fetal Neonatal Ed. (2021) fetalneonatal-2021-322617. doi: 10.1136/archdischild-2021-322617 [Epub ahead of print].
104. Campbell BT, Herbst KW, Briden KE, Neff S, Ruscher KA, Hagadorn JI. Inhaled nitric oxide use in neonates with congenital diaphragmatic hernia. Pediatrics. (2014) 134:e420–6.
105. Barrington KJ, Finer N, Pennaforte T, Altit G. Nitric oxide for respiratory failure in infants born at or near term. Cochrane Database Syst Rev. (2017) 1:CD000399.
106. Putnam LR, Tsao K, Morini F, Lally PA, Miller CC, Lally KP, et al. Evaluation of variability in inhaled nitric oxide use and pulmonary hypertension in patients with congenital diaphragmatic hernia. JAMA Pediatr. (2016) 170:1188–94.
107. Lawrence KM, Monos S, Adams S, Herkert L, Peranteau WH, Munson DA, et al. Inhaled nitric oxide is associated with improved oxygenation in a subpopulation of infants with congenital diaphragmatic hernia and pulmonary hypertension. J Pediatr. (2020) 219:167–72. doi: 10.1016/j.jpeds.2019.09.052
108. Kipfmueller F, Schroeder L, Berg C, Heindel K, Bartmann P, Mueller A. Continuous intravenous sildenafil as an early treatment in neonates with congenital diaphragmatic hernia. Pediatr Pulmonol. (2018) 53:452–60. doi: 10.1002/ppul.23935
109. Loh E, Stamler JS, Hare JM, Loscalzo J, Colucci WS. Cardiovascular effects of inhaled nitric oxide in patients with left ventricular dysfunction. Circulation. (1994) 90:2780–5.
110. Stocker CF, Shekerdemian LS, Nørgaard MA, Brizard CP, Mynard JP, Horton SB, et al. Mechanisms of a reduced cardiac output and the effects of milrinone and levosimendan in a model of infant cardiopulmonary bypass. Crit Care Med. (2007) 35:252–9. doi: 10.1097/01.CCM.0000251123.70632.4E
111. Patel N. Use of milrinone to treat cardiac dysfunction in infants with pulmonary hypertension secondary to congenital diaphragmatic hernia: a review of six patients. Neonatology. (2012) 102:130–6.
112. Mears M, Yang M, Yoder BA. Is milrinone effective for infants with mild-to-moderate congenital diaphragmatic hernia? Am J Perinatol. (2020) 37:258–63. doi: 10.1055/s-0039-1678558
113. Lakshminrusimha S, Keszler M, Kirpalani H, van Meurs K, Chess P, Ambalavanan N, et al. Milrinone in congenital diaphragmatic hernia – a randomized pilot trial: study protocol, review of literature and survey of current practices. Matern Health Neonatol Perinatol. (2017) 3:27. doi: 10.1186/s40748-017-0066-9
114. Angadi U, Westrope C, Chowdhry MF. Is levosimendan effective in paediatric heart failure and post-cardiac surgeries? Interact Cardiovasc Thorac Surg. (2013) 17:710–4.
115. Schroeder L, Gries K, Ebach F, Mueller A, Kipfmueller F. Exploratory assessment of levosimendan in infants with congenital diaphragmatic hernia. Pediatr Crit Care Med. (2021) 22:e382–90. doi: 10.1097/PCC.0000000000002665
116. Acker SN, Kinsella JP, Abman SH, Gien J. Vasopressin improves hemodynamic status in infants with congenital diaphragmatic hernia. J Pediatr. (2014) 165:53–8. doi: 10.1016/j.jpeds.2014.03.059
117. Cheung MMH, Smallhorn JF, Redington AN, Vogel M. The effects of changes in loading conditions and modulation of inotropic state on the myocardial performance index: comparison with conductance catheter measurements. Eur Heart J. (2004) 25:2238–42. doi: 10.1016/j.ehj.2004.07.034
118. Apitz C, Honjo O, Friedberg MK, Assad RS, van Arsdell G, Humpl T, et al. Beneficial effects of vasopressors on right ventricular function in experimental acute right ventricular failure in a rabbit model. Thorac Cardiovasc Surg. (2012) 60:17–23. doi: 10.1055/s-0031-1298058
119. Apitz C, Honjo O, Humpl T, Li J, Assad RS, Cho MY, et al. Biventricular structural and functional responses to aortic constriction in a rabbit model of chronic right ventricular pressure overload. J Thorac Cardiovasc Surg. (2012) 144:1494–501. doi: 10.1016/j.jtcvs.2012.06.027
120. Inamura N, Kubota A, Ishii R, Ishii Y, Kawazu Y, Hamamichi Y, et al. Efficacy of the circulatory management of an antenatally diagnosed congenital diaphragmatic hernia: outcomes of the proposed strategy. Pediatr Surg Int. (2014) 30:889–94. doi: 10.1007/s00383-014-3574-y
121. Le Duc K, Mur S, Sharma D, Aubry E, Recher M, Rakza T, et al. Prostaglandin E1 in infants with congenital diaphragmatic hernia (CDH) and life-threatening pulmonary hypertension. J Pediatr Surg. (2020) 55:1872–8. doi: 10.1016/j.jpedsurg.2020.01.008
122. Lawrence K, Berger K, Herker L, Franciscovich C, O’Dea C, Waqar L, et al. Use of prostaglandin E1 to treat pulmonary hypertension in congenital diaphragmatic hernia. J Pediatr Surg. (2019) 54:55–9.
123. Noori S, Friedlich P, Wong P, Ebrahimi M, Siassi B, Seri I. Hemodynamic changes after low-dosage hydrocortisone administration in vasopressor-treated preterm and term neonates. Pediatrics. (2006) 118:1456–66. doi: 10.1542/peds.2006-0661
124. Seabrook RB, Grover TR, Rintoul N, Weems M, Keene S, Brozanski B, et al. Treatment of pulmonary hypertension during initial hospitalization in a multicenter cohort of infants with congenital diaphragmatic hernia (CDH). J Perinatol. (2021) 41:803–13. doi: 10.1038/s41372-021-00923-z
125. Kamath BD, Fashaw L, Kinsella JP. Adrenal insufficiency in newborns with congenital diaphragmatic hernia. J Pediatr. (2010) 156:495–7.e1.
126. Rafat N, Schaible T. Extracorporeal membrane oxygenation in congenital diaphragmatic hernia. Front Pediatr. (2019) 7:336. doi: 10.3389/fped.2019.00336
127. Kays DW. ECMO in CDH: is there a role? Semin Pediatr Surg. (2017) 26:166–70. doi: 10.1053/j.sempedsurg.2017.04.006
128. Jancelewicz T, Brindle ME, Harting MT, Tolley EA, Langham MR, Lally PA, et al. Extracorporeal membrane oxygenation (ECMO) risk strati fi cation in newborns with congenital diaphragmatic hernia (CDH). J Pediatr Surg. (2018) 53:1890–5. doi: 10.1016/j.jpedsurg.2018.04.014
129. Canadian T, Diaphragmatic C, Collaborative H. Diagnosis and management of congenital diaphragmatic hernia: a clinical practice guideline. Can Med Assoc J. (2018) 190:E103–12.
130. Tanaka T, Inamura N, Ishii R, Kayatani F, Yoneda A, Tazuke Y, et al. The evaluation of diastolic function using the diastolic wall strain (DWS) before and after radical surgery for congenital diaphragmatic hernia. Pediatr Surg Int. (2015) 31:905–10. doi: 10.1007/s00383-015-3766-0
131. Mills J, Safavi A, Skarsgard ED. Chylothorax after congenital diaphragmatic hernia repair: a population-based study. J Pediatr Surg. (2012) 47:842–6. doi: 10.1016/j.jpedsurg.2012.01.031
132. Levy SM, Lally PA, Lally KP, Tsao K. The impact of chylothorax on neonates with repaired congenital diaphragmatic hernia. J Pediatr Surg. (2013) 48:724–9. doi: 10.1016/j.jpedsurg.2012.11.035
133. Kraemer US, Leeuwen L, Krasemann TB, Wijnen RMH, Tibboel D, IJsselstijn H. Characteristics of infants with congenital diaphragmatic hernia who need follow-up of pulmonary hypertension. Pediatr Crit Care Med. (2018) 19:e219–26.
134. Lewis L, Sinha I, Kang SL, Lim J, Losty PD. Long term outcomes in CDH: cardiopulmonary outcomes and health related quality of life. J Pediatr Surg. (2022) S0022-3468(22)00251-2. doi: 10.1016/j.jpedsurg.2022.03.020 [Epub ahead of print].
135. Tonelli AR, Plana JC, Heresi GA, Dweik RA. Prevalence and prognostic value of left ventricular diastolic dysfunction in idiopathic and heritable pulmonary arterial hypertension. Chest. (2012) 141:1457–65.
136. van Wolferen SA, Marcus JT, Boonstra A, Marques KMJ, Bronzwaer JGF, Spreeuwenberg MD, et al. Prognostic value of right ventricular mass, volume, and function in idiopathic pulmonary arterial hypertension. Eur Heart J. (2007) 28:1250–7.
137. Badagliacca R, Poscia R, Pezzuto B, Papa S, Gambardella C, Francone M, et al. Right ventricular dyssynchrony in idiopathic pulmonary arterial hypertension: determinants and impact on pump function. J Heart Lung Transplant. (2015) 34:381–9. doi: 10.1016/j.healun.2014.06.010
138. Egan MJ, Husain N, Stines JR, Moiduddin N, Stein MA, Nelin LD, et al. Mid-term differences in right ventricular function in patients with congenital diaphragmatic hernia compared with controls. World J Pediatr. (2012) 8:350–4. doi: 10.1007/s12519-012-0380-2
139. Toussaint-Duyster LCC, van der Cammen-van Zijp MHM, de Jongste JC, Tibboel D, Wijnen RMH, Gischler SJ, et al. Congenital diaphragmatic hernia and exercise capacity, a longitudinal evaluation. Pediatr Pulmonol. (2019) 54:628–36.
140. Goss KN, Haraldsdottir K, Beshish AG, Barton GP, Watson AM, Palta M, et al. Association between preterm birth and arrested cardiac growth in adolescents and young adults. JAMA Cardiol. (2020) 5:910–8. doi: 10.1001/jamacardio.2020.1511
141. Lewandowski AJ, Raman B, Bertagnolli M, Mohamed A, Williamson W, Pelado JL, et al. Association of preterm birth with myocardial fibrosis and diastolic dysfunction in young adulthood. J Am Coll Cardiol. (2021) 78:683–92. doi: 10.1016/j.jacc.2021.05.053
142. Lewandowski AJ, Augustine D, Lamata P, Davis EF, Lazdam M, Francis J, et al. Preterm heart in adult life: cardiovascular magnetic resonance reveals distinct differences in left ventricular mass, geometry, and function. Circulation. (2013) 127:197–206. doi: 10.1161/CIRCULATIONAHA.112.126920
143. Kinsella JP, Ivy DD, Abman SH. Pulmonary vasodilator therapy in congenital diaphragmatic hernia: acute, late, and chronic pulmonary hypertension. Semin Perinatol. (2005) 29:123–8. doi: 10.1053/j.semperi.2005.04.008
144. Zussman ME, Bagby M, Benson DW, Gupta R, Hirsch R. Pulmonary vascular resistance in repaired congenital diaphragmatic hernia vs. age-matched controls. Pediatr. Res. (2012) 71:697–700. doi: 10.1038/pr.2012.16
145. Goss KN, Beshish AG, Barton GP, Haraldsdottir K, Levin TS, Tetri LH, et al. Early pulmonary vascular disease in young adults born preterm. Am J Respir Crit Care Med. (2018) 198:1549–58.
146. Bojanić K, Grizelj R, Dilber D, Šarić D, Vuković J, Pianosi PT, et al. Cardiopulmonary exercise performance is reduced in congenital diaphragmatic hernia survivors. Pediatr Pulmonol. (2016) 51:1320–9.
147. Peetsold MG, Heij HA, Nagelkerke AF, Ijsselstijn H, Tibboel D, Quanjer PH, et al. Pulmonary function and exercise capacity in survivors of congenital diaphragmatic hernia. Eur Respir J. (2009) 34:1140–7.
148. Aryal SR, Sharifov OF, Lloyd SG. Emerging role of cardiovascular magnetic resonance imaging in the management of pulmonary hypertension. Eur Respir Rev. (2020) 29:190138.
149. Roy CW, van Amerom JFP, Marini D, Seed M, Macgowan CK. Fetal cardiac MRI: a review of technical advancements. Top Magn Reson Imaging. (2019) 28:235–44.
150. Zhao D, Lei W, Hu S. Cardiac organoid-a promising perspective of preclinical model. Stem Cell Res Ther. (2021) 12:272.
151. DeKoninck P, D’hooge J, van Mieghem T, Richter J, Deprest J. Speckle tracking echocardiography in fetuses diagnosed with congenital diaphragmatic hernia. Prenat Diagn. (2014) 34:1262–7. doi: 10.1002/pd.4461
152. Baptista MJ, Nogueira-Silva C, Areias JC, Correia-Pinto J. Perinatal profile of ventricular overload markers in congenital diaphragmatic hernia. J Pediatr Surg. (2008) 43:627–33. doi: 10.1016/j.jpedsurg.2007.08.044
153. Zambaiti E, Bussani R, Calcaterra V, Zandonà L, Silvestri F, Peiró JL, et al. Myocardial effects of fetal endoscopic tracheal occlusion in lambs with CDH. Prenat Diagn. (2016) 36:362–7. doi: 10.1002/pd.4789
154. Aggarwal S, Shanti C, Lelli J, Natarajan G. Prognostic utility of noninvasive estimates of pulmonary vascular compliance in neonates with congenital diaphragmatic hernia. J Pediatr Surg. (2019) 54:439–44. doi: 10.1016/j.jpedsurg.2018.08.057
Keywords: congenital diaphragmatic hernia, ventricular function, ventricular hypoplasia, pulmonary hypertension, echocardiography, biomarkers, cardiac
Citation: Patel N, Massolo AC, Kraemer US and Kipfmueller F (2022) The heart in congenital diaphragmatic hernia: Knowns, unknowns, and future priorities. Front. Pediatr. 10:890422. doi: 10.3389/fped.2022.890422
Received: 05 March 2022; Accepted: 25 July 2022;
Published: 16 August 2022.
Edited by:
Hannes Sallmon, Deutsches Herzzentrum Berlin, GermanyReviewed by:
Jason Gien, University of Colorado Anschutz Medical Campus, United StatesNeysan Rafat, University of Heidelberg, Germany
Paul D. Losty, University of Liverpool, United Kingdom
Christe Weiss, University of Heidelberg, Germany
Copyright © 2022 Patel, Massolo, Kraemer and Kipfmueller. This is an open-access article distributed under the terms of the Creative Commons Attribution License (CC BY). The use, distribution or reproduction in other forums is permitted, provided the original author(s) and the copyright owner(s) are credited and that the original publication in this journal is cited, in accordance with accepted academic practice. No use, distribution or reproduction is permitted which does not comply with these terms.
*Correspondence: Neil Patel, neil.patel@ggc.scot.nhs.uk