- 1Department of Pediatric Cardiology, Hospital HM Nens, HM Hospitales, Barcelona, Spain
- 2Autonomous University of Barcelona, Barcelona, Spain
- 3Department of Pediatric Cardiology, Vall d’Hebron University Hospital, Barcelona, Spain
- 4Biosfer Teslab, Reus, Spain
- 5Department of Basic Medical Sciences, Universitat Rovira I Virgili, Institut D'Investigació Sanitària Pere Virgili (IISPV), Tarragona, Spain
- 6Centro de Investigación Biomédica en Red de Diabetes y Enfermedades Metabólicas Asociadas (CIBERDEM), Instituto de Salud Carlos III (ISCIII), Madrid, Spain
- 7Department of Pediatric Endocrinology, Vall d'Hebron University Hospital, Barcelona, Spain
- 8Department of Pediatric Radiology, Vall d'Hebron University Hospital, Barcelona, Spain
- 9Department of Pediatric Endocrinology, Hospital HM Nens, HM Hospitales, Barcelona, Spain
- 10Fundació Institut d’Investigació en Ciències de la Salut Germans Trias i Pujol (IGTP), Badalona, Spain
- 11Centro de Investigación Biomédica en Red de Enfermedades Raras (CIBERER), Instituto de Salud Carlos III (ISCIII), Madrid, Spain
Introduction: Dyslipidemia secondary to obesity is a risk factor related to cardiovascular disease events, however a pathological conventional lipid profile (CLP) is infrequently found in obese children. The objective is to evaluate the advanced lipoprotein testing (ALT) and its relationship with cardiac changes, metabolic syndrome (MS) and inflammatory markers in a population of morbidly obese adolescents with normal CLP and without type 2 diabetes mellitus, the most common scenario in obese adolescents.
Methods: Prospective case-control research of 42 morbidly obese adolescents and 25 normal-weight adolescents, whose left ventricle (LV) morphology and function had been assessed. The ALT was obtained by proton nuclear magnetic resonance spectroscopy, and the results were compared according to the degree of cardiac involvement – normal heart, mild LV changes, and severe LV changes (specifically LV remodeling and systolic dysfunction) – and related to inflammation markers [highly-sensitive C-reactive protein and glycoprotein A (GlycA)] and insulin-resistance [homeostatic model assessment for insulin-resistance (HOMA-IR)]. A second analysis was performed to compare our results with the predominant ALT when only body mass index and metabolic syndrome criteria were considered.
Results: The three cardiac involvement groups showed significant increases in HOMA-IR, inflammatory markers and ALT ratio LDL-P/HDL-P (40.0 vs. 43.9 vs. 47.1, p 0.012). When only cardiac change groups were considered, differences in small LDL-P (565.0 vs. 625.1 nmol/L, p 0.070), VLDL size and GlycA demonstrated better utility than just traditional risk factors to predict which subjects could present severe LV changes [AUC: 0.79 (95% CI: 0.54–1)]. In the second analysis, an atherosclerotic ALT was detected in morbidly obese subjects, characterized by a significant increase in large VLDL-P, small LDL-P, ratio LDL-P/HDL-P and ratio HDL-TG/HDL-C. Subjects with criteria for MS presented overall worse ALT (specially in triglyceride-enriched particles) and remnant cholesterol values.
Conclusions: ALT parameters and GlycA appear to be more reliable indicators of cardiac change severity than traditional CV risk factors. Particularly, the overage of LDL-P compared to HDL-P and the increase in small LDL-P with cholesterol-depleted LDL particles appear to be the key ALT's parameters involved in LV changes. Morbidly obese adolescents show an atherosclerotic ALT and those with MS present worse ALT values.
Introduction
Cardiovascular diseases (CVD) are the main cause of death in the world (1) and, if the trend initiated in 2,000 continues, CVD will also become the main cause of death among contemporary children. Obesity is a multifactorial disease that includes several preceding disorders of CVD, such as high blood pressure (BP), insulin resistance, diabetes and dyslipidemia. However, the majority of obese children present a pre-pathological condition, therefore identifying which of them are at increased risk of developing CVD is a challenge for pediatricians.
In the conventional lipid profile (CLP), the increase in cholesterol in low-density lipoproteins (LDL-C) has been demonstrated as one of the most important factors associated with CVD risk (2), but the role of high-density lipoprotein cholesterol (HDL-C) or triglycerides (TG) remains unclear (3). Additionally, the remnant cholesterol – a derivative from the CLP that accounts for the cholesterol enclosed in the very low-density lipoproteins (VLDL), intermediate-density lipoproteins (IDL) and chylomicrons – has been also associated with increased prevalence of ischemic heart disease (4) and myocardial infarction (5). On the other hand, the advanced lipoprotein testing (ALT), assessed by proton nuclear magnetic resonance spectroscopy (1H-NMR) of plasma, provides much information about lipoprotein characteristics as it quantifies the concentration, particle size and composition (cholesterol or TG) of each lipoprotein subclass. Previous studies have shown how in situations of apparent normality, specific lipoprotein alterations are highly associated with future CVD events (6–9). Thus, the concept of atherogenic dyslipidemia – applied to subjects with hypo HDL-C, hypertriglyceridemia and normal LDL-C, but increases in some ALT components – has been associated with an elevated risk of CVD events (10–12).
Various studies have evaluated the ALT in obese adults and adolescents and established its utility in predicting arterial wall changes, coronary heart disease or CVD events (13–15), however to our knowledge, none have analyzed which ALT components are closely associated with cardiac changes in obese adolescents. Our group showed in a previous publication that morbidly obese adolescents presented left ventricular remodeling and systo-diastolic dysfunctions, closely related to body mass index (BMI) (16). The objective of the current study is to evaluate the ALT and its relationship with cardiac changes, metabolic syndrome (MS) and inflammatory markers in the same cohort of morbidly obese adolescents with normal CLP and without type 2 diabetes mellitus (T2-DM), the most common scenario in obese adolescents.
Methods
Study population
Prospective observational case-control research of 67 adolescents of both genders between 10 and 17 years old. Subjects with morbid obesity were recruited from endocrinology clinics and control participants were recruited from healthy volunteers in the cardiology and sports medicine clinics.
Subjects with current infectious or recent acute inflammatory processes, history of prematurity or birth weight <2,000 g, and smokers or those whose pathologies could affect the cardiovascular system – such as congenital heart disease, chronic kidney disease, transplant, rheumatic diseases, and HIV infection – were excluded. Similarly, obese subjects with T2-DM and control subjects who practiced >7 weekly sport hours, a threshold explained in our previous study (16), were also excluded in this analysis.
Subjects were classified depending on the degree of cardiac involvement and three groups were established: no cardiac changes (n 25), formed by control and obese adolescents without LV changes; mild cardiac changes (n 17), consisting of 15 obese adolescents with LV remodeling and 2 control adolescents with border systolic dysfunction; and severe cardiac changes (n 25), constituted by morbidly obese adolescents with LV remodeling and systolic dysfunction, based on the threshold below defined. For the second analysis, subjects were reclassified according to their BMI, calculated from tables of the Barcelona Longitudinal Growth Study 1995–2017 (17), in two groups: subjects with morbid obesity (SDS-BMI ≥4; n 42), and with normal weight (BMI 5th–85th percentile, n 25). Finally, the last classification of subjects was performed on the base of MS criteria from Cook et al. (18), identifying two groups: subjects without MS (n 53), and with MS (n 14).
Written informed consent for participation was obtained and the Institutional Review Board at Vall d'Hebron Hospital approved the protocol (PR-AMI-273/2018). All subjects provided assent and an informed consent was signed by their parents/legal guardians.
Clinical and laboratory assessment
Demographic data of age, gender and clinical status were obtained from patient anamnesis. Blood pressure was obtained using a Welch Allyn Spot Vital Signs Monitor (4200B, Hillrom, Batesville, Indiana) after subjects had rested for 5 min, in a supine position and with an appropriately sized cuff giving measured mid-arm circumference, according to the criteria of the European Society of Hypertension (19), which also defines the high BP values by age and gender.
EDTA blood samples were obtained at the time of enrollment at the participating hospitals in the morning after at least 8 h of fasting. Samples for lipoprotein particle analysis were aliquoted, stored in liquid nitrogen (−80°C) and shipped on dry ice to Biosfer Teslab, where the ALT was measured by 1H-NMR spectroscopy, based on the LipoScale test® (20). For each main lipoprotein class (VLDL, LDL and HDL) we obtained large, medium and small subclass particle concentrations (VLDL-P, LDL-P, HDL-P), mean particle size and composition (ratio VLDL-TG/VLDL-C, ratio LDL-TG/LDL-C, ratio HDL-TG/HDL-C). Additionally, tests performed on blood samples from all subjects were: fasting glycemia and insulin, glycated hemoglobin and the CLP (total cholesterol, HDL-C, LDL-C and TG). To evaluate insulin resistance, the homeostasis model assessment of insulin resistance (HOMA-IR) was calculated using the equation: fasting insulin (µU/ml) × fasting glucose (mmol/L)/22.5. Inflammation markers evaluated were highly-sensitive C-reactive protein (hs-CPR) and an NMR derived glycoprotein biomarker, termed glycoprotein A (GlycA), arising from the concentration of the acetyl groups of N-acetylglucosamine and N-acetylgalactosamine bond to plasmatic proteins. Glyc A is able to detect low-grade chronic inflammation in obesity and insulin resistance's disorders (21–23), and in atherosclerosis progression (24).
Echocardiographic image acquisition and analysis
Patients were examined using a Vivid S60 commercial ultrasound scanner (GE Vingmed Ultrasound AS, Horten, Norway) with a phased-array transducer (GE 3-MHz; GE Vingmed Ultrasound AS). Images were obtained at rest in the supine or left lateral decubitus position in the standard tomographic views of the LV (parasternal long and short axis and apical 4-chamber, 2-chamber, and long-axis views). All echocardiographic images were obtained prospectively by an experienced pediatric cardiologist, according to the criteria of the American Society of Echocardiography (ASE) (25).
To evaluate the LV geometry, relative wall thickness (RWT) and LV mass were calculated using LV linear dimensions and following the recommendation of ASE (25). The LV mass was determined by the adjusted Devereux's equation and the resultant value was indexed to height to the power of 2.7 (LVMI, g/m2.7). LV geometry was categorized as normal or pathological (concentric remodeling, eccentric hypertrophy and concentric hypertrophy) considering the following cutoff values (95th percentile in the pediatric population): LVMI >45 g/m2.7 in males and >40 g/m2.7 in females (26), and RWT >0.41 (27).
The LV function was determined by two-dimensional speckle tracking echocardiography, a well-validated and precise method to quantify ventricular function with lower variability than LV ejection fraction in pediatric patients (28). Strain and strain rate (SR) were calculated according to the criteria of the ASE (25) and the European Association of Cardiovascular Imaging (28). Two-dimensional video loops were obtained for each patient in apical four, three, and two-chamber views, acquiring images at a frame rate of >65 frames/s. Offline image processing was performed using EchoPAC (version 11.2, GE Vingmed Ultrasound, Horten, Norway). After manually tracing along the endomyocardial border, the software automatically generated the epicardial border and the six segments, which were accepted after a visual inspection. To determine SR and midline strain, at least 17 out of 18 segments had to be included. Measured parameters were LV end-systolic global longitudinal strain (GLS, %) and LV early diastolic global longitudinal SR (early GLSR, 1/sec). Resultant values were calculated by adding the strain of all accepted segments and dividing the value by the total number of segments. The GLS cutoff value which defined systolic dysfunction in this study was −16.7%, which corresponds to the lowest GLS value reported in a meta-analysis of LV strain measures by echocardiography in children (29).
Statistical analysis
Data from the study were analyzed using descriptive statistics. Quantitative results were expressed as median and 25–75 interquartile range, while qualitative or dichotomous variables were expressed as percentages. Chi-square test (χ2) and Fisher's exact test were used, according to the size and characteristic of qualitative variables, to compare proportions and to study relationships between them. The comparison between quantitative variables was made by nonparametric Mann–Whitney U test or nonparametric Kruskal–Wallis test followed by Dunn's post hoc test for multiple comparisons. To estimate correlations between parameters, the Pearson and Spearman's Rho correlation coefficients were calculated as appropriate for the type of the data. Data was analyzed by using IBM SPSS Statistics for Windows, version 23.0 (IBM Corp., Armonk, N.Y.) and values of p < 0.05 were considered significant.
A three-step multivariate analysis was applied to identify important variables and patterns that allowed distinguishing between the three cardiac involvement groups. In the first step, we applied three statistical approaches to identify the variables that make the largest contributions to the discrimination between groups. These approaches include the Wilcoxon rank-sum test, the Random Forest, and the Partial Least Squares discriminant analysis (PLS-DA). Those variables that resulted significant with a p-value <0.05 were selected as the candidate for the Wilcoxon rank-sum test, and the 5–10 most important variables were determined by the variable importance score or the variable importance in projection (VIP) score using the Random Forest or the PLSA-DA, respectively. To avoid overfitting, 10-fold cross-validation was performed. In the second step, by using a Venn diagram we selected the most prominent variables and those that will be included in the model by determining those that overlap by the statistical approaches. In the third step, we used the Principal Component Analysis (PCA) as an unsupervised method to visualize the capacity of the selected variables to drive group separation. Ellipses in PCA represent 90% confidence intervals around the centroid of each data cluster. Finally, we built a linear fitting model, and by computing the area under the curve (AUC) and the 95% confidence interval of a receiver operating characteristics (ROC) curve we evaluated and quantified how accurately the selected variables were able to discriminate between groups. Patients were randomly assigned to training (60%) and test (40%) sets. We performed 10-fold cross-validation with 100 replicates on the training data during the model construction process and tested the model on the hold-out data. Analysis was performed using the R statistical software version 4.1.1 (Chapman & Hall/CRC Computational Biology Series). Additionally, a logistic regression was performed to model the probability of dichotomous events (mild or severe cardiac changes, and no changes or severe cardiac changes) with the selected variables in the previous statistical analysis.
Results
A total of 42 adolescents with obesity and 25 adolescents without obesity, age-matched, were included in our study. No subject was excluded, although HbA1c was not registered in ten subjects of the obese group and hs-CPR values were missed in one control and three obese subjects. Main clinical, laboratory and echocardiographic data from the three cardiac groups are summarized in Table 1. No significant differences were noted in age, but most obese subjects were classified in the mild or severe cardiac change groups. However, the significant increase observed in BP, HOMA-IR and inflammation markers among the three groups suggests a relationship between the severity of cardiac changes and the worsening in CV risk factors, except in the conventional lipid profile, where no differences were found among both groups with cardiac changes. In contrast, significant differences were detected in the ALT (Table 1), and a pathological phenotype related to cardiac changes was defined (Figure 1): increases in concentrations of total VLDL-P, large VLDL-P, total LDL-P, small LDL-P, ratio LDL-P/HDL-P, and ratio HDL-TG/HDL-C, and decreases in concentrations of total HDL-P and medium HDL-P, and in the LDL size.
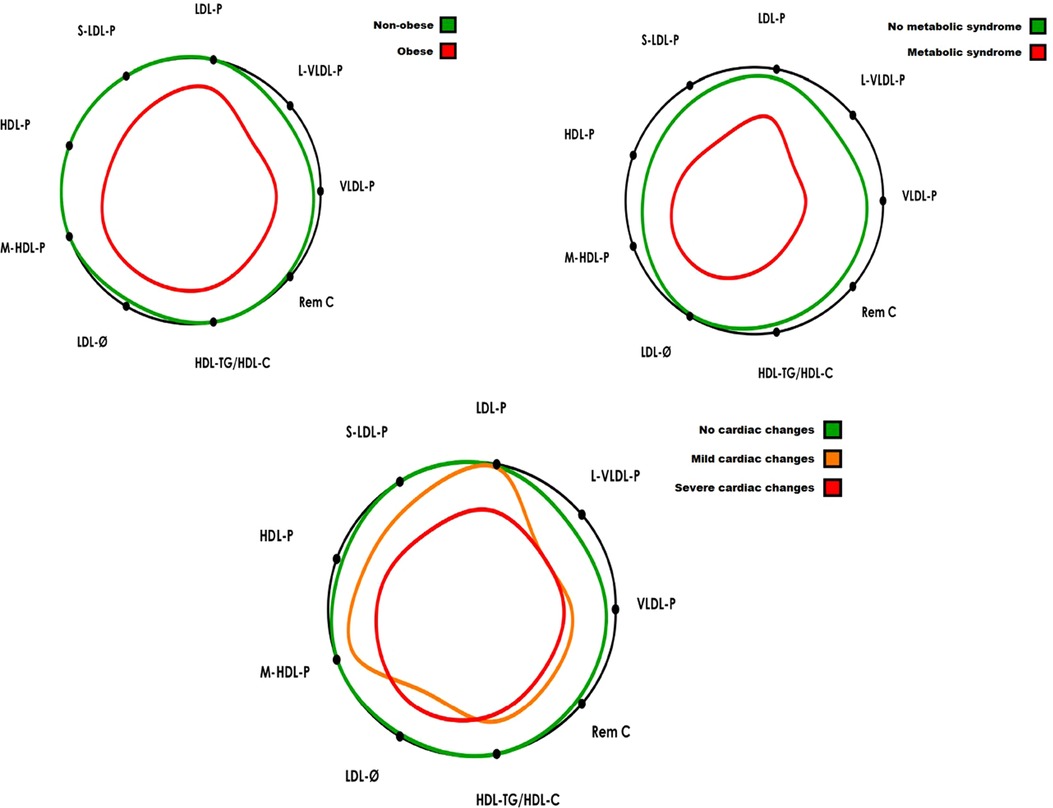
Figure 1. The lipid contour is a graphical model to facilitate the lipoprotein profile interpretation and its association with cardiovascular risk. The colored silhouettes represent the patient groups’ values with respect to the values of an apparently healthy population (black circle) (30). The study group's contour delimits a smaller central area when the variables have values associated with an increased risk of developing CVD (i.e., values higher than the reference population’s mean for L-VLDL-P, VLDL-P, Rem-C, S-LDL-P, LDL-P, HDL-TG/HDL-C variables; or lower than reference population’s mean for LDL-Ø, HDL-P and M-HDL-P variables). Colored silhouettes are represented in percentage of increase or reduction regarding data in Tables 1–3. Bottom of the figure: VLDL-P, total VLDL particles; L-VLDL-P, large VLDL particles; LDL-P, total LDL particles; S-LDL-P, small LDL particles; HDL-P, total HDL particles; M-HDL-P, medium HDL particles; LDL-Ø, LDL particles diameter; HDL-TG/HDL-C, triglyceride enriched HDL; Rem C, remnant cholesterol.
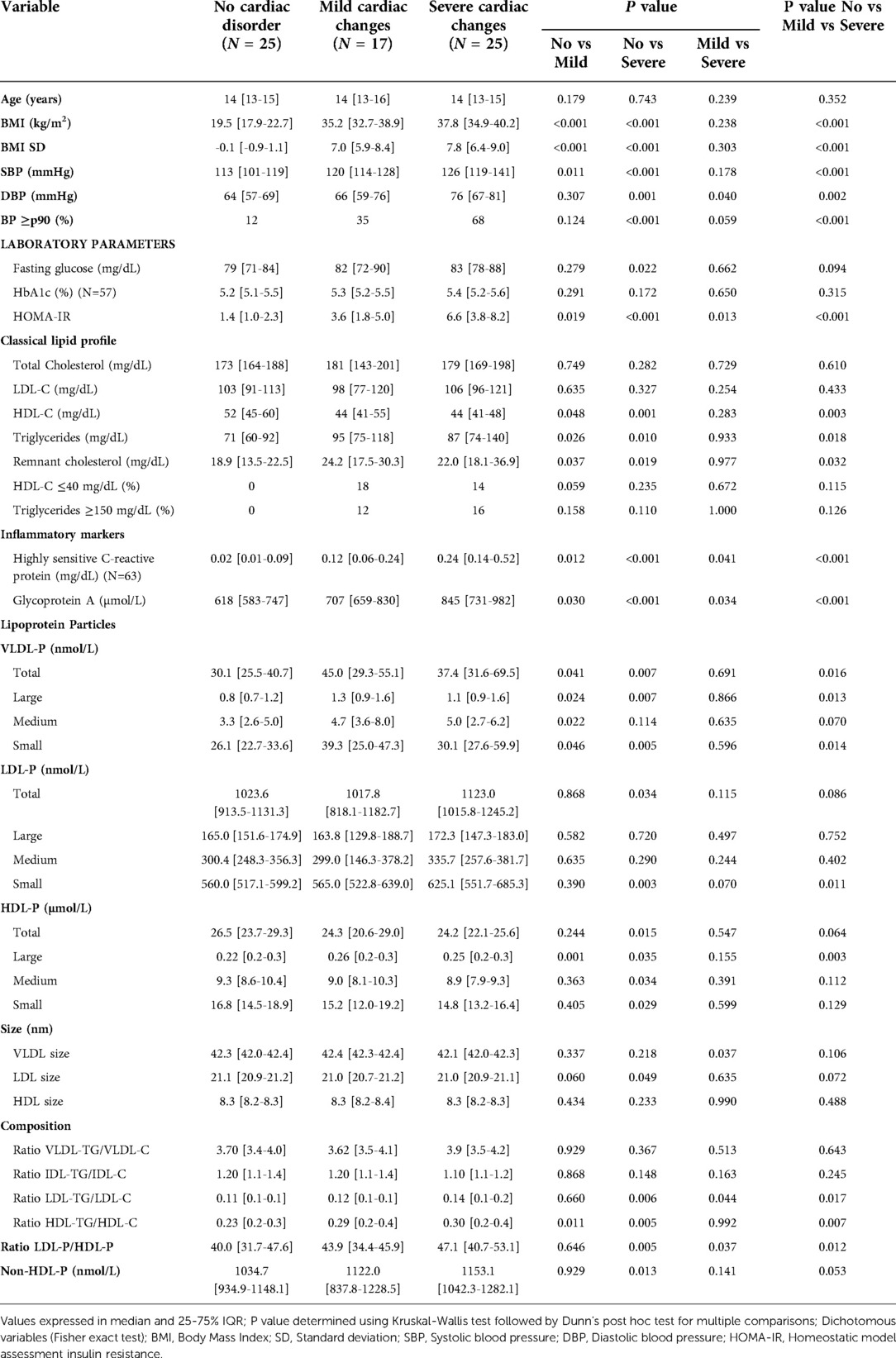
Table 1. Clinical and laboratory characteristics of subjects depending on cardiac involvement degree groups.
Data of LV geometry and speckle tracking determinations from the echocardiographic evaluation are summarized in Supplementary Table S1.
Table 2 shows the clinical characteristics, laboratory parameters and ALT of subjects classified by obesity index. The obese subjects exhibited, in addition to the pathological ALT phenotype observed in subjects with cardiac changes, a mild, though significant, difference in the concentration of HDL-C (52 vs. 44 mg/dl, p < 0.001), triglycerides (77 vs. 91 mg/dl, p < 0.001) and remnant cholesterol (19.1 vs. 23.9 mg/dl, p 0.010) (Figure 1). Finally, data of subjects categorized by MS criteria are summarized in Table 3. Obese adolescents who accomplished MS criteria showed overall worse BP, insulin resistance and hs-CRP values than any of the previous groups. Their CLP was characterized by marked differences in triglycerides (78 vs 124 mg/dL, p < 0.001) and remnant cholesterol (19.6 vs 30.3 mg/dL, p < 0.001), while the ALT was characterized by significant increases in: total VLDL-P, large VLDL-P, ratio LDL-P/HDL-P and ratio HDL-TG/HDL-C (Figure 1). The resultant ALT did not differ when only obese subjects were categorized by MS criteria, remarking the central role of hypertriglyceridemia in subjects with MS (Supplemental Table S2).
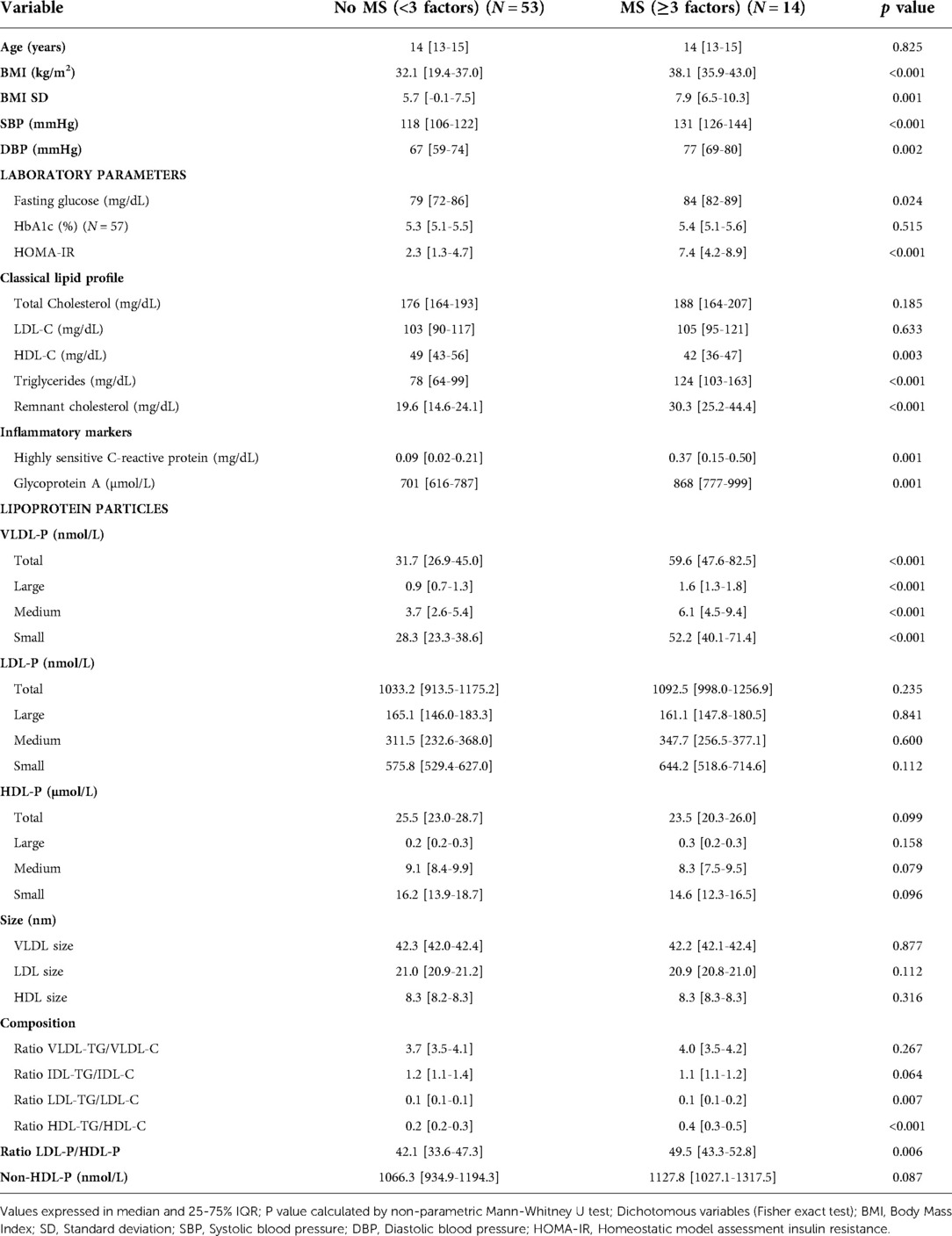
Table 3. Clinical and laboratory characteristics of subjects depending on metabolic syndrome (MS) diagnosis.
Correlation analysis between ALT components and variables of LV geometry and function, HOMA-IR and inflammatory markers are summarized in Table 4. The ratios LDL-P/HDL-P and HDL-TG/HDL-C were the variables better correlated to cardiac changes as well as to insulin resistance and inflammatory parameters. Moreover, considering the specific subclasses of lipoprotein particles, the results indicated that large VLDL-P, small LDL-P and total HDL-P (inversely) subclasses have the best correlation to LV remodeling and systo-diastolic dysfunction, while lipoprotein particles rich in triglycerides (VLDL-P, TG-enriched HDL and cholesterol-depleted LDL) had the highest association with HOMA-IR. Lastly, total HDL-P and small LDL-P were particles related with hs-CRP values, whereas GlycA proved to be better correlated with every component of the ALT, so this inflammatory marker was chosen for the multivariable analysis. Correlation analysis between ALT and CLP components are summarized in Supplementary Table S3. Triglycerides appeared particularly associated with VLDL-P and large HDL-P.
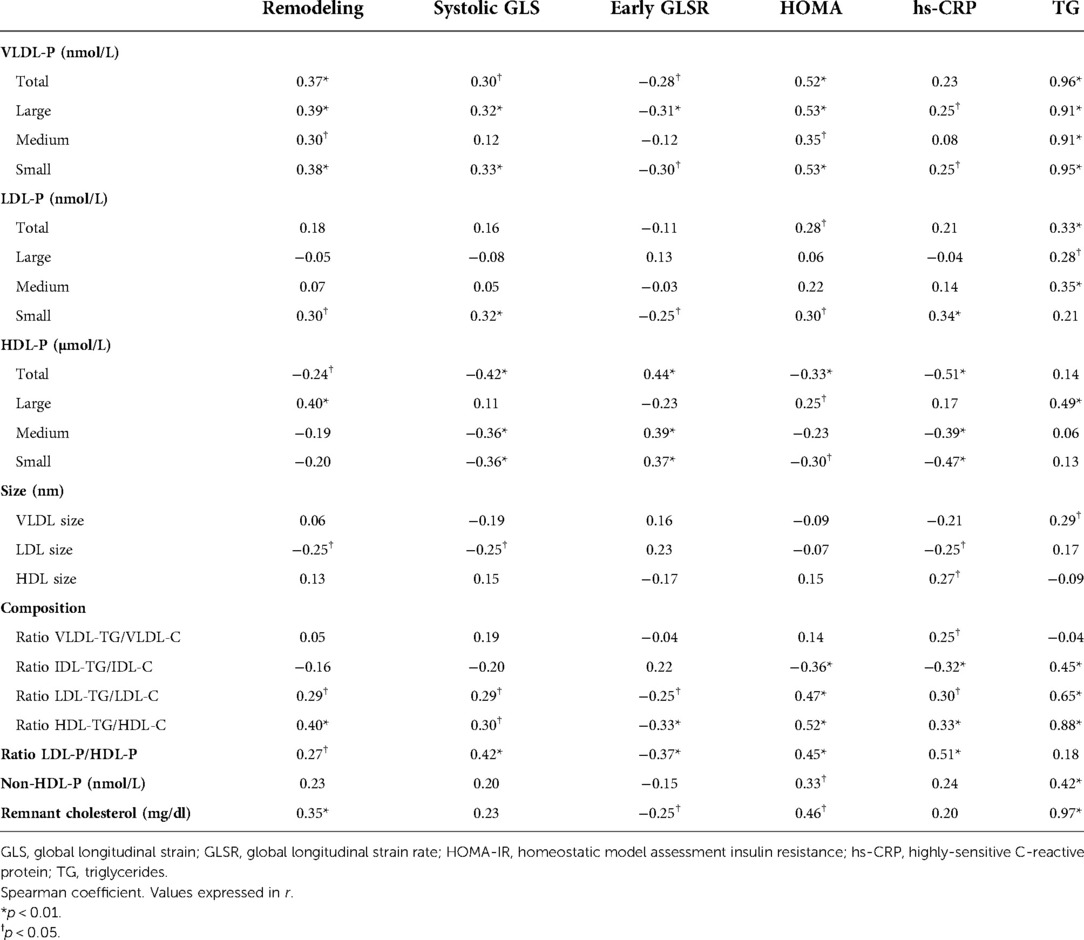
Table 4. Bivariate correlations between lipoprotein particles and left ventricle structural and functional parameters, insulin resistance index, inflammatory marker and triglycerides.
The final multivariable model with the largest AUC [0.79 (95% CI: 0.54–1)] for distinguishing mild cardiac change subjects from those with severe cardiac changes identified the following pattern: HOMA-IR, GlycA, VLDL-diameter and large HDL-P (Figure 2). In contrast, for differentiating normal heart subjects from those with severe cardiac changes the largest AUC [0.91 (95% CI: 0.74–1)] resulted from the following variables: BMI standard deviation, HOMA-IR, systolic BP, diastolic BP, GlycA, small VLDL-P, small LDL-P and ratio HDL-TG/HDL-C (Figure 3). To assess the usefulness of lipoprotein subclasses and glycoprotein A in the multivariable diagnostic model, ROC curves with only traditional CV risk factors (BMI standard deviation, HOMA-IR, systolic and diastolic BP) were developed and compared with the previous analysis. Figure 4 shows the comparative ROC curve analysis. A binomial logistic regression was performed on mild vs. severe cardiac changes groups, where remaining independent parameters of the final model (adjusted R2 = 0.53, p < 0.0001) were HOMA-IR (OR 1.4; 95% CI: 1.0–1.9; p < 0.050), VLDL size (OR 0.0; 95% CI: 0.0–0.9; p < 0.050) and large HDL-P (OR 0.0; 95% CI: 0.0–0.3; p < 0.050); and on normal vs. severe cardiac changes groups, where the remaining independent parameter of the final model (adjusted R2 = 0.84, p < 0.0001) was the BMI standard deviation (OR 2.8; 95% CI: 1.2–6.6; p 0.010).
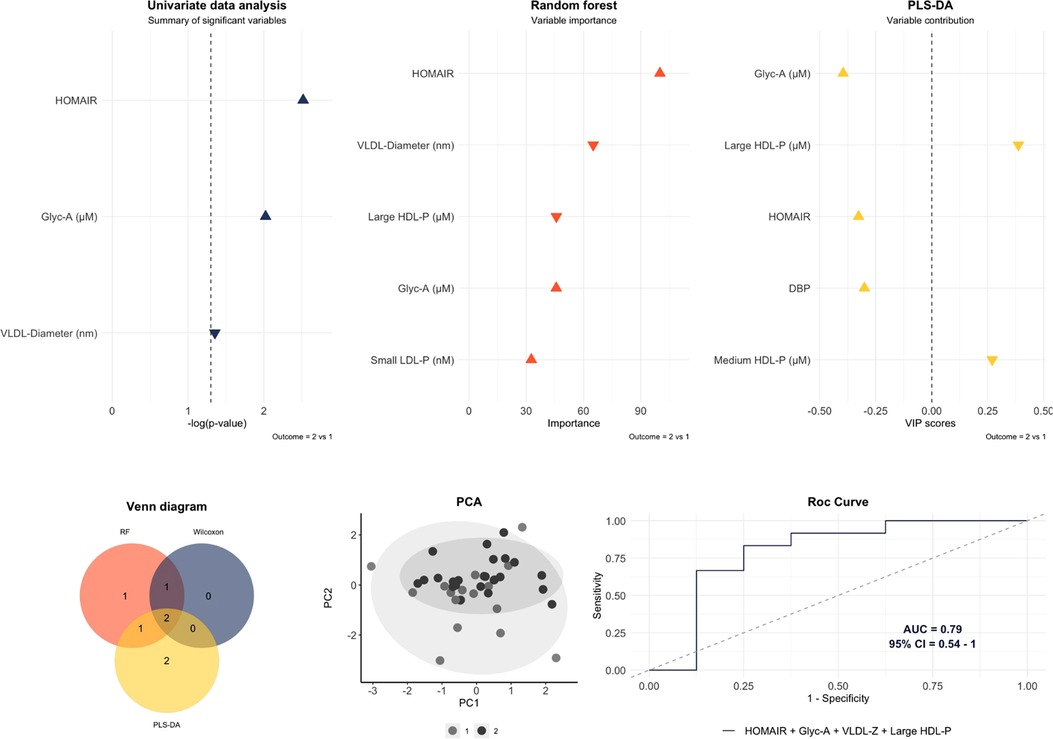
Figure 2. Multivariable model to differentiate mild cardiac change subjects (1) than those with severe cardiac changes (2). The variables included in the model were those that overlap by at least two of the three statistical approaches. An area under the curve (AUC) of 0.79 (95% CI: 0.54–1) was obtained with the next pattern: HOMA-IR, glycoprotein A (Glyc-A), VLDL diameter and large HDL-P. Predictive accuracy = 0.8; p-value = 0.051; out-of-bag error = 0.27.
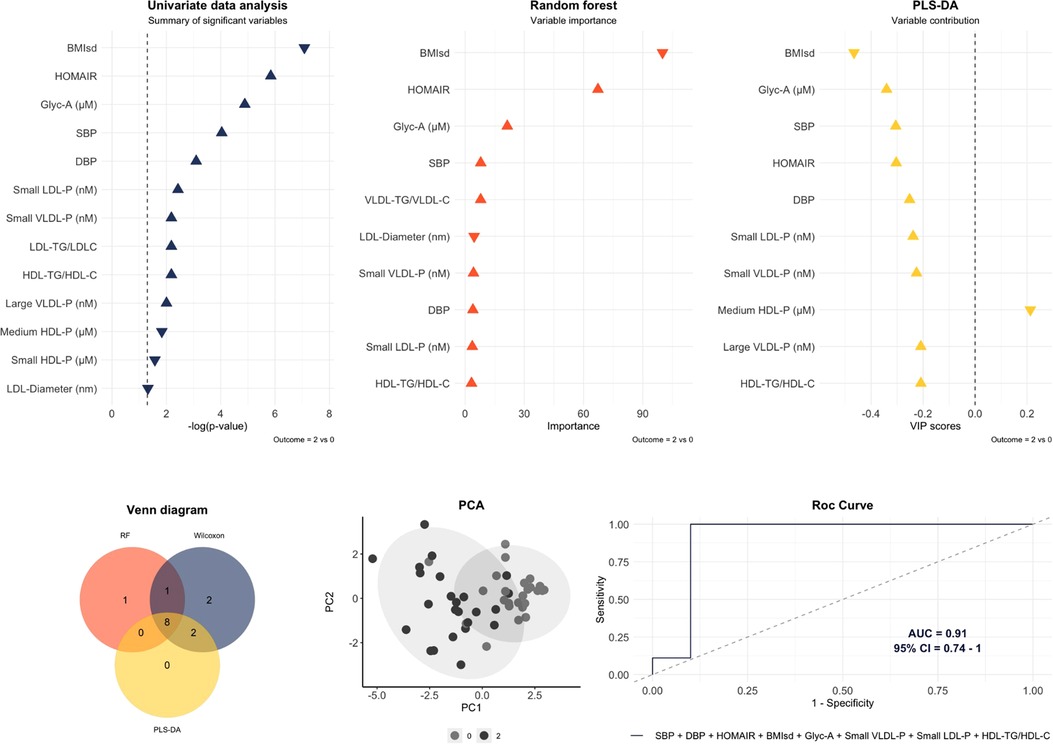
Figure 3. Multivariable model to differentiate normal heart subjects (0) from those with severe cardiac changes (2). The variables included in the model were those that overlap by the three statistical approaches. An area under the curve (AUC) of 0.91 (95% CI: 0.74–1) was obtained with the next pattern: body mass index standard deviation (BMIsd), HOMA-IR, systolic blood pressure (SBP), diastolic blood pressure (DBP), glycoprotein A (Glyc-A), small VLDL-P, small LDL-P and ratio HDL-TG/HDL-C. Predictive accuracy = 0.84; p-value = 0.004; out-of-bag error = 0.1.
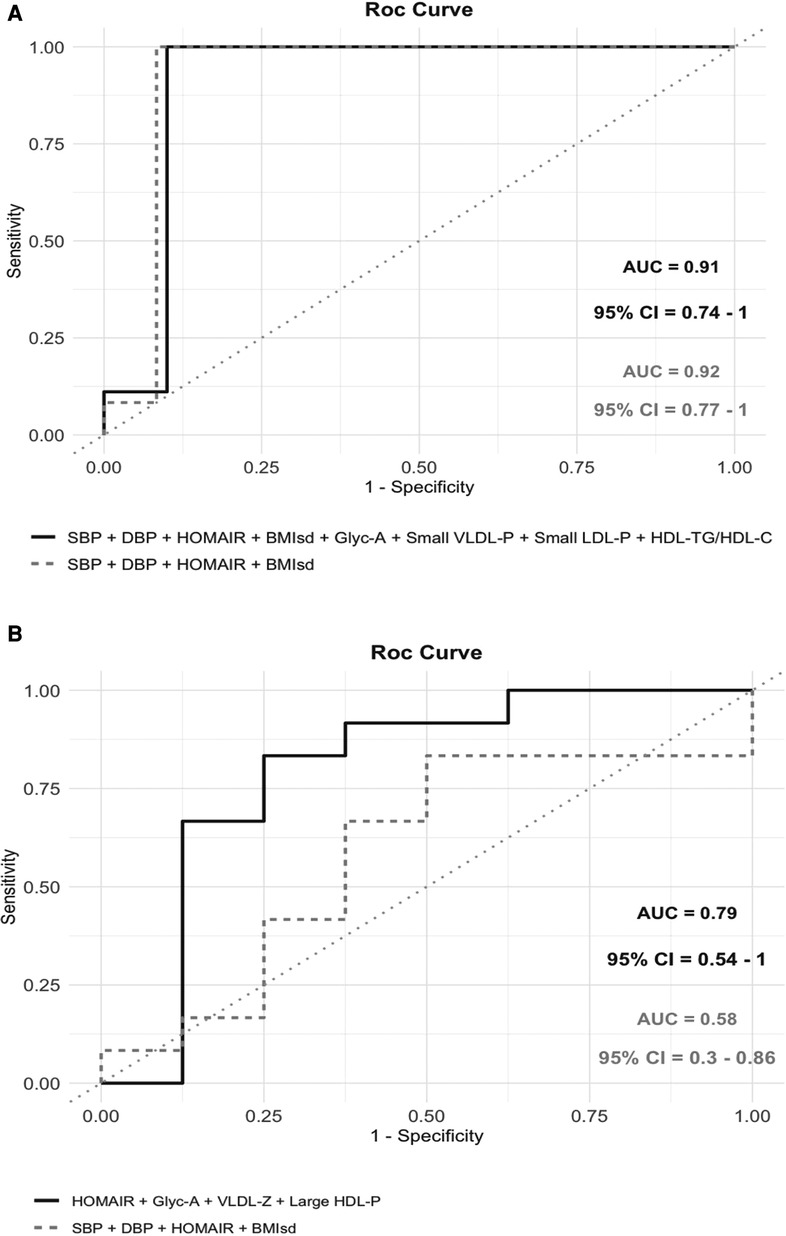
Figure 4. Comparative analysis between ROC curves considering only traditional cardiovascular risk factors (body mass index standard deviation, blood pressure and HOMA-IR) and ROC curves shown in Figures 2, 3. (A) When Normal heart subjects were compared to those with severe cardiac changes, the addition of lipoprotein subclasses and glycoprotein A to the model with traditional risk factors did not change the area under the curve (AUC) (0.92 vs. 0.91) [Only traditional risk factors: AUC 0.92 (95% CI: 0.77–1); predictive accuracy = 0.88; p-value = 0.0001; out-of-bag error = 0.1]. (B) When mild cardiac change subjects were compared to those with severe cardiac changes, the addition of lipoprotein subclasses and glycoprotein A caused an increase in the AUC (0.58 vs. 0.79) [only traditional risk factors: AUC 0.58 (95% CI: 0.3–0.86); predictive accuracy = 0.6; p-value = 0.596; out-of-bag error = 0.32].
Reproducibility of echocardiographic parameters has been demonstrated previously (16), showing good intraclass correlation coefficients.
Discussion
This study showed that morbidly obese adolescents with LV changes presented a pathological phenotype in the ALT despite exhibiting normal values in the CLP (Figure 1). Large VLDL-P, small LDL-P and total HDL-P were the subclasses more closely related to cardiac changes, while the ratio which highlighted the excess of LDL-P in relation to HDL-P appeared to be the variable more closely associated with severity in the LV changes. Furthermore, when obese adolescents were classified by MS criteria or obesity index, the resulting ALT was determined by the high triglyceridemia of these subjects, and hence, predominated TG-enriched lipoproteins, like VLDL and TG-enriched HDL (increase in the ratio HDL-TG/HDL-C) (Figure 1). Additionally, the inflammatory markers exhibited a correlation with the aforementioned pathological ALT and especially with lower levels of total HDL-P and an altered LDL subclass distribution, moved to the smallest LDL-P. Finally, multivariable models showed the relevance of VLDL size and large HDL-P—as well as BP, insulin resistance and inflammation—in the changes observed in obese adolescent's hearts. Our results have highlighted the importance of ALT and GlycA as differentiating indicators of cardiac severity in adolescents with a similar degree of obesity, where minimum differences can be found in BMI, BP or insulin resistance levels.
Previous studies performed in obese or diabetic children have shown similar results. Thus, the most frequently reported ALT was defined by increases of small LDL-P and VLDL-P and decreases of LDL size and large-medium HDL-P (13, 31–33).
VLDL are the main carriers of plasmatic triglycerides, and hence appear particularly increased in subjects with hypertriglyceridemia. In contrast, LDL are cholesterol-enriched lipoproteins and their concentration is not so triglyceride-dependent, however in hypertriglyceridemia conditions the LDL particles have been found to be smaller and compositionally cholesterol-depleted (34). The small LDL-P possesses an increased atherogenicity – on account of mechanisms like endothelial barrier crossing facility or oxidation susceptibility (34) – and it has been postulated as a predictor risk factor for coronary heart disease (35). Similarly, the relevance of LDL-P concentration to predict future CVD events rather than LDL-C has been also documented in the Framingham Offspring Study (6), where the highest risk was attributed to subjects with high LDL-P and low LDL-C. Our study, performed in a population with low LDL-C, has shown a significant increase in the small LDL-P subclass in the obese group. Additionally, in the descriptive statistics between subjects with severe cardiac changes and those with mild cardiac changes, the small LDL-P and cholesterol-depleted LDL (increase in the ratio LDL-TG/LDL-C) seemed to be the best differentiating ALT parameters, having also been found correlated with LV remodeling, systo-diastolic dysfunction, insulin resistance and inflammation. Concerning triglyceride levels, a strong positive correlation was found with all subclasses of VLDL-P and large HDL-P, but apparently, their levels were independent of the small LDL-P, as reported in the Framingham Offspring Study. Regarding VLDL-P, greater significant differences were noted in adolescents with obesity and metabolic syndrome, especially in large and small subclasses, and VLDL size seemed to be useful to differentiate levels of cardiac involvement. The hypersecretion of large VLDL-P, as a result of overnutrition and insulin resistance, has been previously proposed as a key pathological mechanism in atherogenic dyslipidemia (36, 37).
Cardioprotective functions of HDL include, in addition to the reverse cholesterol transport to the liver, the inhibition of LDL oxidation and anti-inflammatory actions in the endothelium (38, 39). However, in conditions of hypertriglyceridemia and insulin resistance, the overstimulated cholesteryl-ester-transfer protein enriches HDL composition in TG by means of exchanging TG by cholesteryl esters with other lipoprotein subclasses (40–42). Consequently, TG-enriched HDL have their beneficial features reduced – in T2-DM patients by up to 52% of the HDL antioxidative capacity (43) – and have been associated with atheroma plaque formation (44). Our results have highlighted the prevalence of TG-enriched HDL among participants with cardiac changes and especially in those with MS, as well as a relationship between TG-enriched HDL and LDL and the insulin resistance. Hence, the HDL's cardioprotective function might be decreased in these subjects.
Furthermore, a reduced number of total and medium HDL-P concentration and size has been associated with higher CVD risk in adults (45, 46), and previous studies conducted in obese adolescents with insulin resistance or T2-DM noted a significant decrease in large HDL-P (47). Nevertheless, these findings were not reproduced in the descriptive analysis of our cohort, where the significant reduction was shown in the total HDL-P concentration, without significant distinctions between the subclasses, however the large HDL-P reduction was identified as a differentiating factor between mild and severe cardiac changes in the multivariable analysis, which gives prominence to the widely recognized role of smaller HDL size in CVD (48).
Remnant cholesterol marks the overall load of TG-enriched lipoproteins (VLDL and IDL), which in situations of hypertriglyceridemia can carry as much or more cholesterol than LDL (49). How it interacts in the atherosclerosis physiopathology is still unclear but its association with CVD, as a factor related to coronary artery disease, has been established and an increased risk – up to 2.7 times in concentrations ≥39 mg/dl – has been reported as independent of obesity (5). The highest remnant cholesterol values in the present study were near to this hypothetical threshold and belonged to obese adolescents with metabolic syndrome, who also were the subjects with the highest triglyceride levels. However, a close relationship with cardiac changes was not demonstrated.
This study has some limitations. The cross-sectional design and small sample size limit the extraction of causal conclusions. The criteria for the degrees of cardiac involvement have been defined by the authors and have not been previously tested in other publications. They are based on the concept that severity increases with the addition of cardiac changes, from only LV remodeling to systolic dysfunction, defined by GLS, a widely studied parameter linked to mortality (50). The diastolic function was not included in the group definition criteria because of the lack of a single parameter for its identification, but the early GLSR was incorporated to the correlation analysis instead.
In conclusion, our results have shown that morbidly obese adolescents present an atherosclerotic ALT despite showing no pathological concentrations in the conventional lipid profile. Subjects that meet the criteria for metabolic syndrome present overall worse ALT and remnant cholesterol values because of the highest triglyceride levels.
Furthermore, when the obese adolescents are classified by the degree of cardiac change, ALT and GlycA appear to be more reliable indicators of severity than traditional CV risk factors as BMI, BP or insulin resistance. Particularly, the overage of LDL-P compared to HDL-P and the increase in small LDL-P with cholesterol-depleted LDL particles appear to be the key ALT's parameters involved in LV changes.
Data availability statement
The raw data supporting the conclusions of this article will be made available by the authors, without undue reservation.
Ethics statement
The studies involving human participants were reviewed and approved by Institutional Review Board at Vall d'Hebron Hospital approved the protocol (PR-AMI-273/2018). Written informed consent to participate in this study was provided by the participants’ legal guardian/next of kin.
Author contributions
JMS, AS, DY, PSV and NA designed the study, conducted the bibliographic search and wrote different parts of the manuscript. LA and EM contributed to gathering patient data and to the discussion of the study results. LR, NM and FR participated in the results evaluation, analysis and discussion. JMS and DY coordinated all authors’ participation. All authors contributed to the article and approved the submitted version.
Funding
J.M.S. received grant funding for this project from the Spanish Paediatric Cardiology and Congenital Cardiopathies Society (J.V. Comas 2019). D.Y. used funds to finance the analysis of blood samples from “The Growth and Development group” of the Vall d'Hebron Research Institute, partially financed by Novo Nordisk Pharma S.A.
Acknowledgments
The authors wish to acknowledge the work of cardiologists and endocrinologist Dr. Ariadna Campos and Dr. Maria Clemente from Vall d'Hebron Hospital, and Dr. Marisa Torres, Dr. Georges Akel and Dr. Zelmira Bosch from HM Nens Hospital for their contributions to patient recruitment and image analysis related to this study. We also wish to thank Dr. Roser Ferrer from the biochemistry department of Vall d'Hebron Hospital. The project was funded by the Spanish Paediatric Cardiology and Congenital Cardiopathies Society and by “The Growth and Development group” (Vall d'Hebron Research Institute). Statistical analysis has been carried out in the Statistics and Bioinformatics Unit (UEB) Vall d'Hebron Hospital Research Institute (VHIR) and in the Department of Basic Medical Sciences (Universitat Rovira i Virgili, Institut d'Investigació Sanitària Pere Virgili) by Dr. Martínez-Micaelo.
Conflict of interest
NA has a patent and is stock owner of Biosfer Teslab, the company that commercializes the lipoprotein profiling described in the present manuscript. This study received funding from Novo Nordisk Pharma S.A. through “The Growth and Development Group” of the Vall d’Hebron Research Institute. The funder was not involved in the study design, collection, analysis, interpretation of data, the writing of this article or the decision to submit it for publication. All authors declare no other competing interests.
Publisher's note
All claims expressed in this article are solely those of the authors and do not necessarily represent those of their affiliated organizations, or those of the publisher, the editors and the reviewers. Any product that may be evaluated in this article, or claim that may be made by its manufacturer, is not guaranteed or endorsed by the publisher.
Supplementary material
The Supplementary Material for this article can be found online at: https://www.frontiersin.org/articles/10.3389/fped.2022.887771/full#supplementary-material.
References
1. World Health Organization. Global health estimates. (2020). Available at: http://www.who.int/healthinfo/global_health_estimates/en/ (Accessed December 15, 2021).
2. Borén J, Chapman MJ, Krauss RM, Packard CJ, Bentzon JF, Binder CJ, et al. Low-density lipoproteins cause atherosclerotic cardiovascular disease: pathophysiological, genetic, and therapeutic insights: a consensus statement from the European atherosclerosis society consensus panel. Eur Heart J. (2020) 41(24):2313–30. doi: 10.1093/eurheartj/ehz962
3. Emerging Risk Factors Collaboration, Di Angelantonio E, Sarwar N, Perry P, Kaptoge S, Ray KK, et al. Major lipids, apolipoproteins, and risk of vascular disease. JAMA. (2009) 302:1993–2000. doi: 10.1001/jama.2009.1619
4. Varbo A, Benn M, Smith GD, Timpson NJ, Tybjaerg-Hansen A, Nordestgaard BG. Remnant cholesterol, low-density lipoprotein cholesterol, and blood pressure as mediators from obesity to ischemic heart disease. Circ Res. (2015) 116:665–73. doi: 10.1161/CIRCRESAHA.116.304846
5. Varbo A, Freiberg JJ, Nordestgaard BG. Remnant cholesterol and myocardial infarction in Normal weight, overweight, and obese individuals from the Copenhagen general population study. Clin Chem. (2018) 64(1):219–30. doi: 10.1373/clinchem.2017.279463
6. Cromwell WC, Otvos JD, Keyes MJ, Pencina MJ, Sullivan L, Vasan RS, et al. LDL Particle number and risk of future cardiovascular disease in the framingham offspring study - implications for LDL management. J Clin Lipidol. (2007) 1:583–92. doi: 10.1016/j.jacl.2007.10.001
7. Davidson MH, Ballantyne CM, Jacobson TA, Bittner VA, Braun LT, Brown AS, et al. Clinical utility of inflammatory markers and advanced lipoprotein testing: advice from an expert panel of lipid specialists. J Clin Lipidol. (2011) 5(5):338–67. doi: 10.1016/j.jacl.2011.07.005
8. Girona J, Amigó N, Ibarretxe D, Plana N, Rodríguez-Borjabad C, Heras M, et al. HDL Triglycerides: a new marker of metabolic and cardiovascular risk. Int J Mol Sci. (2019) 20(13):3151. doi: 10.3390/ijms20133151
9. Fernández-Cidón B, Candás-Estébanez B, Gil-Serret M, Amigó N, Corbella E, Rodríguez-Sánchez MÁ, et al. Physicochemical properties of lipoproteins assessed by nuclear magnetic resonance as a predictor of premature cardiovascular disease. PRESARV-SEA study. J Clin Med. (2021) 10(7):1379. doi: 10.3390/jcm10071379
10. Pichler G, Amigo N, Tellez-Plaza M, Pardo-Cea MA, Dominguez-Lucas A, Marrachelli VG, et al. LDL Particle size and composition and incident cardiovascular disease in a south-European population: the hortega-liposcale follow-up study. Int J Cardiol. (2018) 264:172–8. doi: 10.1016/j.ijcard.2018.03.128
11. Mora S, Buring JE, Ridker PM. Discordance of low-density lipoprotein (LDL) cholesterol with alternative LDL-related measures and future coronary events. Circulation. (2014) 129(5):553–61. doi: 10.1161/CIRCULATIONAHA.113.005873
12. Tehrani DM, Zhao Y, Blaha MJ, Mora S, Mackey RH, Michos ED, et al. Discordance of low-density lipoprotein and high-density lipoprotein cholesterol particle versus cholesterol concentration for the prediction of cardiovascular disease in patients with metabolic syndrome and diabetes Mellitus (from the multi-ethnic study of atherosclerosis [MESA]). Am J Cardiol. (2016) 117(12):1921–7. doi: 10.1016/j.amjcard.2016.03.040
13. Urbina EM, McCoy CE, Gao Z, Khoury PR, Shah AS, Dolan LM, et al. Lipoprotein particle number and size predict vascular structure and function better than traditional lipids in adolescents and young adults. J Clin Lipidol. (2017) 11(4):1023–31. doi: 10.1016/j.jacl.2017.05.011
14. Mackey RH, McTigue KM, Chang YF, Barinas-Mitchell E, Evans RW, Tinker LF, et al. Lipoprotein particles and size, total and high molecular weight adiponectin, and leptin in relation to incident coronary heart disease among severely obese postmenopausal women: the women's health initiative observational study. BBA Clin. (2015) 3:243–50. doi: 10.1016/j.bbacli.2015.03.005
15. Mora S, Caulfield MP, Wohlgemuth J, Chen Z, Superko HR, Rowland CM, et al. Atherogenic lipoprotein subfractions determined by Ion mobility and first cardiovascular events after random allocation to high-intensity statin or placebo: the justification for the use of statins in prevention: an intervention trial evaluating rosuvastatin (JUPITER) trial. Circulation. (2015) 132(23):2220–9. doi: 10.1161/CIRCULATIONAHA.115.016857
16. Siurana JM, Ventura PS, Yeste D, Riaza-Martin L, Arciniegas L, Clemente M, et al. Myocardial geometry and dysfunction in morbidly obese adolescents (BMI 35–40 kg/m2). Am J Cardiol. (2021) 157:128–34. doi: 10.1016/j.amjcard.2021.07.026
17. Carrascosa A, Yeste D, Moreno-Galdó A, Gussinyé M, Ferrández Á, Clemente M, et al. Body mass index and tri-ponderal mass index of 1,453 healthy non-obese, non-undernourished millennial children. The Barcelona longitudinal growth study. An Pediatr (Engl Ed). (2018) 89(3):137–43. doi: 10.1016/j.anpedi.2017.12.016
18. Cook S, Weitzman M, Auinger P, Nguyen M, Dietz WH. Prevalence of a metabolic syndrome phenotype in adolescents: findings from the third national health and nutrition examination survey, 1988–1994. Arch Pediatr Adolesc Med. (2003) 157(8):821–7. doi: 10.1001/archpedi.157.8.821
19. Lurbe E, Agabiti-Rosei E, Cruickshank JK, Dominiczak A, Erdine S, Hirth A, et al. 2016 European society of hypertension guidelines for the management of high blood pressure in children and adolescents. J Hypertens. (2016) 34(10):1887–920. doi: 10.1097/HJH.0000000000001039
20. Mallol R, Amigó N, Rodríguez MA, Heras M, Vinaixa M, Plana N, et al. Liposcale: a novel advanced lipoprotein test based on 2D diffusion-ordered 1H NMR spectroscopy. J Lipid Res. (2015) 56(3):737–46. doi: 10.1194/jlr.D050120
21. Lorenzo C, Festa A, Hanley AJ, Rewers MJ, Escalante A, Haffner SM. Novel protein glycan-derived markers of systemic inflammation and C-reactive protein in relation to glycemia, insulin resistance, and insulin secretion. Diabetes Care. (2017) 40(3):375–82. doi: 10.2337/dc16-1569
22. Fuertes-Martín R, Moncayo S, Insenser M, Martínez-García MÁ, Luque-Ramírez M, Grau NA, et al. Glycoprotein A and B height-to-width ratios as obesity-independent novel biomarkers of low-grade chronic inflammation in women with polycystic ovary syndrome (PCOS). J Proteome Res. (2019) 18(11):4038–45. doi: 10.1021/acs.jproteome.9b00528
23. Amigó N, Fuertes-Martín R, Malo AI, Plana N, Ibarretxe D, Girona J, et al. Glycoprotein profile measured by a 1H-nuclear magnetic resonance based on approach in patients with diabetes: a new robust method to assess inflammation. Life (Basel). (2021) 11(12):1407. doi: 10.3390/life11121407
24. Malo AI, Girona J, Ibarretxe D, Rodríguez-Borjabad C, Amigó N, Plana N, et al. Serum glycoproteins A and B assessed by 1H-NMR in familial hypercholesterolemia. Atherosclerosis. (2021) 330:1–7. doi: 10.1016/j.atherosclerosis.2021.06.905
25. Chamber Quantification Writing Group; American Society of Echocardiography’s Guidelines and Standards Committee; European Association of Echocardiography, Lang RM, Bierig M, Devereux RB, Flachskampf FA, Foster E, Pellikka PA, et al. Recommendations for chamber quantification: a report from the American society of echocardiography’s guidelines and standards committee and the chamber quantification writing group, developed in conjunction with the European association of echocardiography, a branch of the European society of cardiology. J Am Soc Echocardiogr. (2005) 18:1440–63. doi: 10.1016/j.echo.2005.10.005
26. Khoury PR, Mitsnefes M, Daniels SR, Kimball TR. Age-specific reference intervals for indexed left ventricular mass in children. J Am Soc Echocardiogr. (2009) 22:709–14. doi: 10.1016/j.echo.2009.03.003
27. Daniels SR, Loggie JM, Khoury P, Kimball TR. Left ventricular geometry and severe left ventricular hypertrophy in children and adolescents with essential hypertension. Circulation. (1998) 97:1907–11. doi: 10.1161/01.CIR.97.19.1907
28. Voigt JU, Pedrizzetti G, Lysyansky P, Marwick TH, Houle H, Baumann R, et al. Definitions for a common standard for 2D speckle tracking echocardiography: consensus document of the EACVI/ASE/industry task force to standardize deformation imaging. J Am Soc Echocardiogr. (2015) 28:183–93. doi: 10.1016/j.echo.2014.11.003
29. Levy PT, Machefsky A, Sanchez AA, Patel MD, Rogal S, Fowler S, et al. Reference ranges of left ventricular strain measures by two-dimensional speckle-tracking echocardiography in children: a systematic review and meta-analysis. J Am Soc Echocardiogr. (2016) 29(3):209–25. doi: 10.1016/j.echo.2015.11.016
30. Puig-Jové C, Castelblanco E, Falguera M, Hernández M, Soldevila B, Julián MT, et al. Advanced lipoprotein profile in individuals with normal and impaired glucose metabolism. Rev Esp Cardiol (Engl Ed). (2022) 75(1):22–30. doi: 10.1016/j.recesp.2021.02.013
31. Shah AS, Davidson WS, Gao Z, Dolan LM, Kimball TR, Urbina EM. Superiority of lipoprotein particle number to detect associations with arterial thickness and stiffness in obese youth with and without prediabetes. J Clin Lipidol. (2016) 10(3):610–8. doi: 10.1016/j.jacl.2016.01.007
32. Higgins V, Asgari S, Hamilton JK, Wolska A, Remaley AT, Hartmann B, et al. Postprandial dyslipidemia, hyperinsulinemia, and impaired gut peptides/bile acids in adolescents with obesity. J Clin Endocrinol Metab. (2020) 105(4):1228–41. doi: 10.1210/clinem/dgz261
33. Mietus-Snyder M, Drews KL, Otvos JD, Willi SM, Foster GD, Jago R, et al. Low-density lipoprotein cholesterol versus particle number in middle school children. J Pediatr. (2013) 163(2):355–62. doi: 10.1016/j.jpeds.2013.01.012
34. Sattar N, Petrie JR, Jaap AJ. The atherogenic lipoprotein phenotype and vascular endothelial dysfunction. Atherosclerosis. (1998) 138(2):229–35. doi: 10.1016/S0021-9150(98)00037-9
35. Hoogeveen RC, Gaubatz JW, Sun W, Dodge RC, Crosby JR, Jiang J, et al. Small dense low-density lipoprotein-cholesterol concentrations predict risk for coronary heart disease: the atherosclerosis risk in communities (ARIC) study. Arterioscler Thromb Vasc Biol. (2014) 34(5):1069–77. doi: 10.1161/ATVBAHA.114.303284
36. Mackey RH, Mora S, Bertoni AG, Wassel CL, Carnethon MR, Sibley CT, et al. Lipoprotein particles and incident type 2 diabetes in the multi-ethnic study of atherosclerosis. Diabetes Care. (2015) 38(4):628–36. doi: 10.2337/dc14-0645
37. Sparks JD, Sparks CE, Adeli K. Selective hepatic insulin resistance, VLDL overproduction, and hypertriglyceridemia. Arterioscler Thromb Vasc Biol. (2012) 32(9):2104–12. doi: 10.1161/ATVBAHA.111.241463
38. Rye KA, Barter PJ. Cardioprotective functions of HDLs. J Lipid Res. (2014) 55(2):168–79. doi: 10.1194/jlr.R039297
39. Rosenson RS, Brewer HB Jr, Ansell BJ, Barter P, Chapman MJ, Heinecke JW, et al. Dysfunctional HDL and atherosclerotic cardiovascular disease. Nat Rev Cardiol. (2016) 13(1):48–60. doi: 10.1038/nrcardio.2015.124
40. Brown RJ, Rader DJ. When HDL gets fat. Circ Res. (2008) 103(2):131–2. doi: 10.1161/CIRCRESAHA.108.180638
41. Pintó X, Masana L, Civeira F, Ibarretxe D, Candas B, Puzo J, et al. Consensus document of an expert group from the spanish society of arteriosclerosis (SEA) on the clinical use of nuclear magnetic resonance to assess lipoprotein metabolism (liposcale®). Clin Investig Arterioscler. (2020) 32(5):219–29. doi: 10.1016/j.arteri.2020.04.004
42. Amigó N, Mallol R, Heras M, Martínez-Hervás S, Blanco Vaca F, Escolà-Gil JC, et al. Lipoprotein hydrophobic core lipids are partially extruded to surface in smaller HDL: “herniated” HDL, a common feature in diabetes. Sci Rep. (2016) 6:19249. doi: 10.1038/srep19249
43. Nobécourt E, Jacqueminet S, Hansel B, Chantepie S, Grimaldi A, Chapman MJ, et al. Defective antioxidative activity of small dense HDL3 particles in type 2 diabetes: relationship to elevated oxidative stress and hyperglycaemia. Diabetologia. (2005) 48(3):529–38. doi: 10.1007/s00125-004-1655-5
44. Amor AJ, Catalan M, Pérez A, Herreras Z, Pinyol M, Sala-Vila A, et al. Nuclear magnetic resonance lipoprotein abnormalities in newly-diagnosed type 2 diabetes and their association with preclinical carotid atherosclerosis. Atherosclerosis. (2016) 247:161–9. doi: 10.1016/j.atherosclerosis.2016.02.014
45. Mora S, Glynn RJ, Ridker PM. High-density lipoprotein cholesterol, size, particle number, and residual vascular risk after potent statin therapy. Circulation. (2013) 128(11):1189–97. doi: 10.1161/CIRCULATIONAHA.113.002671
46. Holmes MV, Millwood IY, Kartsonaki C, Hill MR, Bennett DA, Boxall R, et al. Lipids, lipoproteins, and metabolites and risk of myocardial infarction and stroke. J Am Coll Cardiol. (2018) 71(6):620–32. doi: 10.1016/j.jacc.2017.12.006
47. Davidson WS, Heink A, Sexmith H, Dolan LM, Gordon SM, Otvos JD, et al. Obesity is associated with an altered HDL subspecies profile among adolescents with metabolic disease. J Lipid Res. (2017) 58(9):1916–23. doi: 10.1194/jlr.M078667
48. Aday AW, Lawler PR, Cook NR, Ridker PM, Mora S, Pradhan AD. Lipoprotein particle profiles, standard lipids, and peripheral artery disease incidence. Circulation. (2018) 138(21):2330–41. doi: 10.1161/CIRCULATIONAHA.118.035432
49. Varbo A, Benn M, Tybjærg-Hansen A, Jørgensen AB, Frikke-Schmidt R, Nordestgaard BG. Remnant cholesterol as a causal risk factor for ischemic heart disease. J Am Coll Cardiol. (2013) 61(4):427–36. doi: 10.1016/j.jacc.2012.08.1026
50. Singh GK, Cupps B, Pasque M, Woodard PK, Holland MR, Ludomirsky A. Accuracy and reproducibility of strain by speckle tracking in pediatric subjects with normal heart and single ventricular physiology: a two-dimensional speckle-tracking echocardiography and magnetic resonance imaging correlative study. J Am Soc Echocardiogr. (2010) 23(11):1143–52. doi: 10.1016/j.echo.2010.08.010
Keywords: morbid obesity, adolescents, lipoprotein subclasses, atherosclerotic phenotype, metabolic syndrome, cardiac changes, systolic dysfunction, small LDL particles
Citation: Siurana JM, Sabaté-Rotés A, Amigó N, Martínez-Micaelo N, Arciniegas L, Riaza L, Mogas E, Rosés-Noguer F, Ventura PS and Yeste D (2022) Different profiles of lipoprotein particles associate various degrees of cardiac involvement in adolescents with morbid obesity. Front. Pediatr. 10:887771. doi: 10.3389/fped.2022.887771
Received: 2 March 2022; Accepted: 2 November 2022;
Published: 22 November 2022.
Edited by:
Dimpna Calila Albert-Brotons, King Faisal Specialist Hospital & Research Centre, Saudi ArabiaReviewed by:
Hayato Tada, Kanazawa University, JapanStefano Cacciatore, International Centre for Genetic Engineering and Biotechnology (ICGEB), South Africa
© 2022 Siurana, Sabaté-Rotés, Amigó, Martínez-Micaelo, Arciniegas, Riaza, Mogas, Rosés-Noguer, Ventura and Yeste. This is an open-access article distributed under the terms of the Creative Commons Attribution License (CC BY). The use, distribution or reproduction in other forums is permitted, provided the original author(s) and the copyright owner(s) are credited and that the original publication in this journal is cited, in accordance with accepted academic practice. No use, distribution or reproduction is permitted which does not comply with these terms.
*Correspondence: José M. Siurana am1zaXVyYW5hQGhvdG1haWwuY29t
†These authors have contributed equally to this work and share first authorship
‡These authors have contributed equally to this work and share senior authorship
Specialty Section: This article was submitted to Pediatric Cardiology, a section of the journal Frontiers in Pediatrics