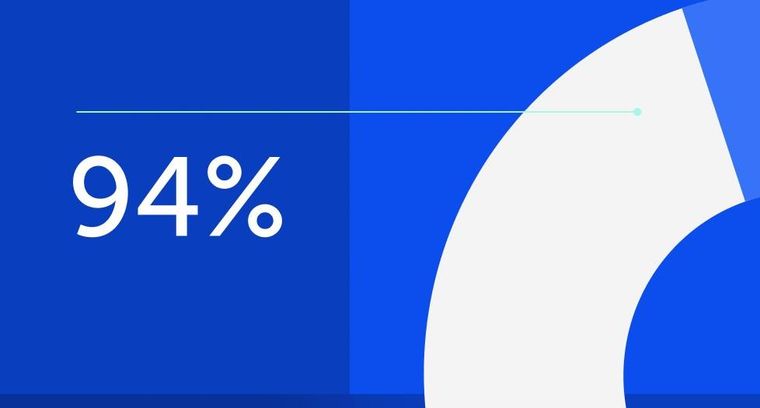
94% of researchers rate our articles as excellent or good
Learn more about the work of our research integrity team to safeguard the quality of each article we publish.
Find out more
ORIGINAL RESEARCH article
Front. Pediatr., 25 April 2022
Sec. Children and Health
Volume 10 - 2022 | https://doi.org/10.3389/fped.2022.864233
Background: To investigate the risk factors for change in refraction and refractive components in preschoolers.
Methods: Preschool children aged 3–5 years old, from the junior and the middle grades of seven randomly selected kindergartens in Jia Ding District, Shanghai, were followed for 1 year. Cycloplegic autorefraction (1% cyclopentolate) and axial length (AL) were measured at baseline and at 1-year follow-up. Questionnaires about parental myopia and environmental risk factors, such as time of outdoors and near work, were collected.
Results: A total of 603 right eyes of 603 children were included. Parental myopia was not associated with a change in refraction, but two myopic parents were associated with a longer change in AL (coefficient = 0.153, p = 0.006), after adjusted for baseline spherical refraction, age, gender, change in height, change in weight, and environment risk factors. In the multivariate analyses, boys showed a more myopic refraction shift than girls in 1 year (coefficient = −0.150, p = 0.008) and a quicker AL elongation (coefficient = 0.120, p = 0.008). Time of near work, such as watching television, using computer, reading and writing, and time of outdoor activities, was not associated with a change in refraction or AL.
Conclusions: In preschool age, environmental risk factors were not strongly associated with the change in refraction or refractive components. Parental myopia influences the refractive development of children continuously from infancy to preschool age, which might be the biological basis of school myopia.
Risk factors for myopia have been comprehensively studied, such as parental myopia, time spent in near work and outdoor activities, and intensive education (1–3). Generally, the prevalence and incidence of myopia in preschool children were quite low, despite a progressively increasing prevalence of myopia reported in high myopia prevalence regions, such as Singapore and Taiwan (4, 5). The presence of myopia at the entrance of the primary school was an important risk factor for the incidence of high myopia in the teenage and adulthood (6, 7). In addition, the refraction [spherical equivalent (SE)] at school entrance also largely determined the incidence of school myopia (8, 9). Therefore, exploring risk factors for change in refraction and refractive components in preschool children was valuable.
Studies about risk factors for refractive change or refractive errors for preschool children were limited, among which, most of the studies were cross-sectional (10–14). The reported risk factors for myopia in preschoolers included parental myopia, ethnic group, younger age (<36 months), smoking mother at pregnant or passive smoking 6 months before birth, and astigmatism (10–13, 15). However, childhood exposure to passive smoke was not associated with the mean values for SE or axial length (AL) change (15). Time spent on near work or on outdoor activities was not found to be the risk factor for myopia prevalence in 3-year-old children (10, 11). However, one study reported a close relationship between outdoor time at 3 years old and future myopia in teenage years (16). Birth season was also reported to be related to refraction in very young children, however, the relationship is controversial and might be very subtle (17, 18).
For children aged 3–6 years old, the change in SE and the prevalence of the different types of refractive errors were quite stable at this stage (19, 20). In addition, it is reported that for myopic children, refraction stability and even regression were observed in a significant proportion of preschool children (21, 22), which indicated the special characteristics of the refractive development in the preschoolers. Therefore, longitudinal studies were needed to explore the associated risk factors for the change in refraction and refractive components in the preschoolers.
Taking kindergarten as a unit, random cluster sampling was conducted on all kindergartens in Jia Ding District, Shanghai, by lottery method. Seven kindergartens were randomly selected, and all the preschool children aged 3–5 years old from the junior and the middle grades in the selected kindergartens were included in the study. Children were followed for 1 year. The first visit was carried out from November to December in 2014; the second visit was carried out from November to December in 2015. Considering the length of the schooling system of kindergartens in Shanghai, children of senior grade level were not included, because those children will enter primary schools after 1 year and will be lost to follow-up.
The research protocol was adhered to the Declaration of Helsinki for research that involves human subjects. Written informed consent was signed by either a parent or legal guardian of each subject, and oral agreement was obtained from the children themselves. Children and parents could choose whether to accept cycloplegia or not. Only those who agreed to cycloplegia received 1% cyclopentolate to dilate pupils. The study was approved by the Ethics Committee (No. 2015KY150). Children with written informed consent were included in the study. Those with severe vision-threatening diseases, such as congenital cataract or severe ocular trauma, were excluded from the study.
For cycloplegia, children were first anesthetized topically with one drop of 0.5% proparacaine hydrochloride in each eye. After about 15 s, cycloplegia was induced with two drops of 1% cyclopentolate (Cyclogyl; Alcon, Fort Worth, TX, USA) 5 min apart in each eye. Pupil size and light reflex were examined 30 min after the first drops of cyclopentolate, and if the pupil was dilated to 6 mm or larger and a light reflex was absent, cycloplegia was deemed completely. If the pupillary light reflex was still present, the third drop of cyclopentolate was given 15 s after another drop of proparacaine hydrochloride in each eye. Children, who still failed the standards for successful cycloplegia 15–20 min later, were not given more eye drops, and were recorded as unsuccessful cycloplegia.
Axial length was measured with three consecutive measurements (IOL Master, version 5.02, Carl Zeiss, Jena, Germany) and the average reading of the three measurements was calculated. If the differences in any of the two measurements were larger than 0.02 mm, a further measurement was conducted. Similarly, the average of three consecutive auto-refractor (KR-8900, Topcon, Tokyo, Japan) measurements after successful cycloplegia was used for determining the spherical diopters (DSs), cylinder diopters (DCs), and corneal curvature radius (CR) of the children. If any of the two measurements varied by more than 0.50 diopters (D), a further measurement was taken. Every day before the examination, the machines were all preheated for several minutes, and the auto-refractor and the IOL Master were calibrated using a model eye.
Risk factors were collected through questionnaires. The questionnaires were filled by parents or guardians at baseline and follow-up. Parental myopia, average eye usage time at home and school on weekdays and weekends during the most recent month were collected. Time spent on reading and writing, using tablet computer and mobile phone, playing computer, watching television, and time spent outdoors was investigated.
Since the Pearson coefficients were 0.90 and 0.94 for the baseline SE and AL between the right and the left eyes (both p < 0.001) and 0.90 and 0.96 for the follow-up SE and AL (both p < 0.001), the right eye was used for data analyses. One year change in refraction and refractive components was calculated as follows: measurement at the second visit—measurement at baseline. The SE equals to DS + 0.5 × DC. The eye usage time per day was calculated as the (time at weekdays × 5 + time at weekend × 2)/7. Eye usage time was classified into three categories according to the tertiles. Since about 63.2% of children spent ≤ 0.25 h per day on the computer, the time spent on the computer was classified into two categories as low (≤0.25 h) and high (>0.25 h). The time spent in reading, writing, and using mobile phone and tablet computer was merged together as the time spent on reading and writing, since the working distances were similar among those near work activities.
Univariate and multivariate linear regression analyses were performed to investigate the associations between risk factors and baseline SE, baseline AL, change in SE, and change in AL. The change in SE was further classified into ≥0.25 D, <0.25 and ≥0.1 D, <0.1 and ≥-0.1 D, <-0.1 and ≥-0.25 D, and <-0.25 D, and proportions of change in SE were compared between children with different number of myopic parents using a chi-square test. SAS 9.4 (SAS Institute, Cary, NC, USA) and SPSS 22.0 (IBM SPSS Inc. Chicago, IL, USA) were used for statistical analyses. A value of p < 0.05 was considered statistically significant.
At the first visit, a total of 903 children were successfully cyclopleged. Among them, a total of 857 (94.9%) children were re-examined 1 year later, at which time 613 children (67.9%) were successfully cyclopleged, and 603 children with right eye data completed. Therefore, a total of 603 right eyes of 603 children were included in the analyses. The included and excluded children did not differ significantly with respect to baseline age (p = 0.223), gender (p = 0.763) or SER (spherical equivalent refraction) (p = 0.348), and AL (p = 0.246) of their right eyes. The baseline characteristics of the study population are listed in Table 1.
At baseline, the prevalence of myopia (SE ≤ −0.5 D), astigmatism (DC ≤ −2.0 D), and hyperopia (≥+3.0 D) were 1.33% (8/603), 2.32% (14/603), and 2.82% (17/603). The 1-year incidence rates of myopia were 1.92% (3/156), 0.92% (3/327), and 0.89% (1/112) in the 3-, 4-, and 5-year olds. The 1-year incidence rates of astigmatism were 3.18% (5/157), 0.93% (3/324), and 0% (0/108) in the 3-, 4-, and 5-year olds. The 1-year incidence rates of hyperopia were 1.96% (3/153), 0.61% (2/326), and 0.93% (1/107) in the 3-, 4-, and 5-year olds.
The average changes of SE were 0.34 D [standard deviation (SD) = 0.58], −0.003 D (SD = 0.47), and −0.13 D (SD = 0.58) for those who were suffering from myopia, emmetropia (−0.5 D < SE <3 D), and hyperopia at baseline, and the median values were 0.375, 0, and −0.13 D, respectively. The average changes of AL were 0.31 mm (SD = 0.10), 0.25 mm (SD = 0.35), and 0.32 mm (SD = 0.31) for those who were suffering from myopia, emmetropia, and hyperopia at baseline, and the median values were 0.27, 0.26, and 0.23 mm, respectively.
For the change in SE, the univariate analyses showed that boys (coefficient = −0.102, p = 0.009) and a more hyperopic baseline SE (coefficient = −0.076, p < 0.001) were associated with a more myopic shift in SE; larger change in height (coefficient = 0.024, p = 0.03) was associated with a more hyperopic shift in SE (Table 2). The multivariate analyses showed that boys and a more hyperopic baseline SE were associated with a change in SE toward the myopic direction (Table 3). Parental myopia and the environmental risk factors were not significantly associated with the change in SE (Tables 2, 3; Figure 1). Although the proportion of hyperopic shift of SE ≥ 0.25 D was decreased with the increased number of myopic parents (Figure 2), the proportions of change in SE were not statistically different between children with 0, 1, or 2 myopic parents (X2 = 13.94, p = 0.08).
Figure 1. Change in SE and AL between children with different number of myopic parents. (A) The mean SE and standard deviation (SD) at the first visit and the second visit were plotted separately for children with 0, 1, and 2 myopic parents. (B) The mean AL and SD at the first visit and the second visit were plotted separately for children with 0, 1, and 2 myopic parents. The blue line represents children with no myopic parents. The orange line represents children with one myopic parent. The gray line represents children with two myopic parents. SE, spherical equivalent refraction; AL, axial length.
Figure 2. Stacked histogram of the 1-year changes in SE grouped by the amplitude of the changes between children with different numbers of myopic parents. SE, spherical equivalent refraction.
For the change in AL, the univariate analysis showed that two myopic parents (coefficient = 0.085, p = 0.02) and younger age (coefficient = −0.050, p = 0.02) were associated with more rapid AL elongation (Table 2; Figure 1). The multivariate analysis showed that more hyperopic baseline SE, two myopic parents, younger age, boys, and greater change in height were associated with more rapid elongation in AL (Table 4). The environmental risk factors were not significantly associated with the change in AL (Tables 2, 4).
In the present study, environmental risk factors, such as the time of near work and outdoor activities, were not associated with the change in SE or AL in the 1-year follow-up period. Parental myopia was not associated with the change in SE, but associated with the change in AL. The change in SE did not show the difference with the increasing age, however, the elongation in AL was decreased with age. Boys showed a quicker AL elongation than girls and were more likely to have a myopic SE change.
Animal studies suggested that the visual environment plays an important role in the ocular growth and refractive development (23). In animal models, form deprivation could cause myopia, and lens- induced myopic defocus/hyperopic defocus could lead to hyperopia or myopia (24, 25). In young humans, visual deprivation from congenital cataract, ptosis, corneal opacity, or other ocular diseases could lead to the failure of emmetropization and development of myopia (26, 27). For school-aged children, time outdoors were regarded as protective factors for incident myopia, probably because of the intensive light level in outdoor settings (28).
Studies in preschool children were limited, and most were cross-sectional and did not observe strong related factors (10–13, 15–18). In the present study, time of near work and outdoor activities were not associated with refraction or refractive change, probably because the variation of the activity time was small among the young children. Another explanation is that the refraction of children during this age period was not sensitive to the environmental risk factors and therefore, remains quite stable with very low incidence or prevalence of refractive errors.
In the present cohort, most children spent <2 h on the reading (such as using mobile phone and tablet computer) and writing per day, about 1 h on watching television, and <0.25 h on using the computer. Most children spent around 2 h per day on outdoor activities, and some even reached 3 h a day. These could be the most important reasons why the incidence of myopia was relatively low in the preschoolers. On the contrary, the school-aged children in Shanghai were reported to spend about 1 h a day on outdoor activities, and much more time in near work-related activities apart from having lessons at school (9). The change in the environment associated with much longer eye usage time and limited outdoor time could be the major reason for epidemics of myopia in school-aged children (29).
Previous studies suggested that early-onset myopia was closely related to genetics (10, 11, 30, 31). Using umbilical cord tissues, in-utero epigenetic factors were found to be related to myopia onset before 3 years old (31). Cross-sectional studies indicated that children with two myopic parents were more likely to be myopic at the age between 6 and 72 months and were more likely to have a less hyperopic SE and longer AL when compared with children without myopic parents (10, 11, 30). Children with tall height were also associated with a more myopic SE and longer AL in children younger than 6 years old (10, 11). Tideman et al. showed that weight and height during pregnancy and 2 years postnatally were closely associated with AL and corneal CR at 4.9–9 years old, which indicated the genetic basis for the development of refractive components (32).
An interesting finding was that parental myopia was not significantly associated with the 1-year changes in SE in the present children aged 3–5 years old. Further analyses showed that the changes in SE were 0.022 D (SD = 0.514), −0.014 D (0.470), and −0.030 D (0.420) for two myopic parents, one myopic parent, and none myopic parent, respectively. Although a myopic trend was observed in terms of the mean value, the difference was not statistically significant or was clinically relevant. On the contrary, the multivariate analysis showed that children with two myopic parents when compared with no myopic parents had a quicker elongation of AL by 0.15 mm, which was similar to those reported in the Population-Based Pediatric Eye Disease Study (POPEYE) (30). The difference also had clinical meaning, which was equivalent to about 0.3 D in refraction. Parental myopia was closely related to the baseline SE (not presented in the results), those with two myopic parents had a mean SE of 0.37 D [95% confidence interval (CI): 0.18–0.57, p < 0.001] more myopic than those without myopic parents, which was similar to what was reported in Singapore and the POPEYE (about 0.35–0.39 D SE difference between two myopic parents and no myopic parents) (11, 30).
The reason why the growth of the AL did not show a difference in SE could be that it was mainly compensated by changes in other refractive components, such as a decrease in crystalline lens power. But once these compensatory factors were exhausted, children with two myopic parents were more susceptible to suffer from myopia when compared with children without myopic parents. The results indicated a continuous effect of parental myopia on AL and SE, not only in the early period after birth, but also in preschool children aged 3–6 years old. This may be the biological basis of the influence of parental myopia on the incidence of myopia in school-age children.
In preschool children aged 3–6 years old, boys showed more rapid elongation of AL than girls (nearly 0.1 mm quicker than girls) and a more myopic change in SE (about 0.135 D more myopic than girls). However, at school-aged children, girls were usually at higher risk of myopia than boys. A meta-analysis revealed that after 9 years old age, gender difference begins to emerge in White and East Asians, and the difference becomes more obvious with increasing age, with girls being twice as likely as boys to be myopic at the age of 18 (33).
The phenomenon could be partially explained by the difference in the growth rate of height between the two genders. According to the growth curves of Chinese children, before 10 years old, the median height of boys was longer than that of girls, however, after 10 years old, the median height of girls exceeds that of boys. Then after 12 years old, the boys again showed longer height than girls (34).
However, even adjusted for change in height, boys still showed quicker elongation of AL than girls in the preschoolers (Table 4, Models 2 and 3), suggesting that there are other mechanisms in gender regulation of refraction and AL growth.
There were several limitations in the present study. First, the sample size is not large enough to include the adequate number of incident myopia, therefore, the risk factors for incident myopia cannot be determined in the present study. Second, the percentage of the loss of follow-up was relatively high. Although comparisons of baseline characteristics were not significantly different between those who were followed and those who were lost, cautions should be paid when interpreting the results, considering possible bias. Third, the use of cycloplegic autorefraction without retinoscopy could cause bias in the estimation of the true value of refraction for preschoolers. Studies have found that although the mean difference between cycloplegic autorefraction and retinoscopy could be <0.25 D, the variance could be as large as 3.5 D in either refractive direction (35). The use of a table-mounted auto-refractor in the present study was reported to be more accurate when compared with a hand-held auto-refractor, reducing the errors to some extent (36). Last but not the least, the environmental risk factors were collected through questionnaires, which could be imprecise due to recall bias. A recent study pointed out that using questionnaires could probably overestimate the time of near work (37). Future research can use more accurate and objective measurement methods, such as light measuring wearable devices, to determine the exposure of children to environmental risk factors, so as to obtain more accurate results.
The strength of the present study included the longitudinal design and the use of cycloplegia to obtain accurate refraction in the preschoolers. The present study revealed the change in refraction and refractive components with age and gender in the age between 3 and 5 years old. The study results also explained the reason that parental myopia could be an important risk factor for incident myopia in school-aged children. Exposure to myopia-related environmental risk factors was limited in the present population, therefore, protecting the preschoolers from being myopic.
The raw data supporting the conclusions of this article will be made available by the authors, without undue reservation.
The studies involving human participants were reviewed and approved by Ethics Committee of Shanghai General Hospital. Written informed consent to participate in this study was provided by the participants' legal guardian/next of kin.
YM and SL analyzed the data, wrote the manuscript, and participated in the design of the study. JZ and RZ collected data and helped to interpret the data. BZ, YY, and YS helped to analyze the data and interpret the data. XX, XH, and HZ designed the study and revised the manuscript. All authors contributed to the article and approved the submitted version.
Financial support for this study was provided by the Chinese National key research and development program (Project number 2021YFC2702100), The Science and Technology Commission of Shanghai Municipality (Project No. 20DZ1100200), Shanghai Municipal Commission of health (public health system three-year plan-Key Subjects) (Project No. GWV10.1-XK06), the Project of Shanghai Shen Kang Hospital Development Centre (Grant No. SHDC2020CR30538, SHDC2018110, SHDC12021613) Shanghai engineering research center of precise diagnosis and treatment of eye diseases, Shanghai, China (Project No. 19DZ2250100), Chinese Natural Science Foundation for Young Staff (No. 81800881), Shanghai Sailing Program (No. 17YF1416100), and Shanghai Municipal Health Commission (No. 20184Y0217). The funding agreement ensured the authors' independence in designing the study, interpreting the data, writing, and publishing the report.
The authors declare that the research was conducted in the absence of any commercial or financial relationships that could be construed as a potential conflict of interest.
All claims expressed in this article are solely those of the authors and do not necessarily represent those of their affiliated organizations, or those of the publisher, the editors and the reviewers. Any product that may be evaluated in this article, or claim that may be made by its manufacturer, is not guaranteed or endorsed by the publisher.
1. French AN, Morgan IG, Mitchell P, Rose KA. Risk factors for incident myopia in Australian schoolchildren: the Sydney adolescent vascular and eye study. Ophthalmology. (2013) 120:2100–8. doi: 10.1016/j.ophtha.2013.02.035
2. Mountjoy E, Davies NM, Plotnikov D, Smith GD, Rodriguez S, Williams CE, et al. Education and myopia: assessing the direction of causality by mendelian randomisation. BMJ. (2018) 361:k2022. doi: 10.1136/bmj.k2022
3. Huang PC, Hsiao YC, Tsai CY, Tsai DC, Chen CW, Hsu CC, et al. Protective behaviours of near work and time outdoors in myopia prevalence and progression in myopic children: a 2-year prospective population study. Br J Ophthalmol. (2020) 104:956–61. doi: 10.1136/bjophthalmol-2019-314101
4. Dirani M, Chan YH, Gazzard G, Hornbeak DM, Leo SW, Selvaraj P, et al. Prevalence of refractive error in Singaporean Chinese children: the strabismus, amblyopia, and refractive error in young Singaporean Children (STARS) study. Invest Ophthalmol Vis Sci. (2010) 51:1348–55. doi: 10.1167/iovs.09-3587
5. Lai YH, Hsu HT, Wang HZ, Chang SJ, Wu WC. The visual status of children ages 3 to 6 years in the vision screening program in Taiwan. J AAPOS. (2009) 13:58–62. doi: 10.1016/j.jaapos.2008.07.006
6. Chua SY, Sabanayagam C, Cheung YB, Chia A, Valenzuela RK, Tan D, et al. Age of onset of myopia predicts risk of high myopia in later childhood in myopic Singapore children. Ophthalmic Physiol Opt. (2016) 36:388–94. doi: 10.1111/opo.12305
7. Lin H, Long E, Ding X, Diao H, Chen Z, Liu R, et al. Prediction of myopia development among Chinese school-aged children using refraction data from electronic medical records: a retrospective, multicentre machine learning study. PLoS Med. (2018) 15:e1002674. doi: 10.1371/journal.pmed.1002674
8. Zadnik K, Sinnott LT, Cotter SA, Jones-Jordan LA, Kleinstein RN, Manny RE, et al. Prediction of juvenile-onset myopia. JAMA Ophthalmol. (2015) 133:683–9. doi: 10.1001/jamaophthalmol.2015.0471
9. Ma Y, Zou H, Lin S, Xu X, Zhao R, Lu L, et al. Cohort study with 4-year follow-up of myopia and refractive parameters in primary schoolchildren in Baoshan District, Shanghai. Clin Exp Ophthalmol. (2018) 46:861–72. doi: 10.1111/ceo.13195
10. Chua SY, Ikram MK, Tan CS, Lee YS, Ni Y, Shirong C, et al. Relative contribution of risk factors for early-onset myopia in young Asian children. Invest Ophthalmol Vis Sci. (2015) 56:8101–7. doi: 10.1167/iovs.15-16577
11. Low W, Dirani M, Gazzard G, Chan YH, Zhou HJ, Selvaraj P, et al. Family history, near work, outdoor activity, and myopia in Singapore Chinese preschool children. Br J Ophthalmol. (2010) 94:1012–6. doi: 10.1136/bjo.2009.173187
12. Huang J, Maguire MG, Ciner E, Kulp MT, Cyert LA, Quinn GE, et al. Risk factors for astigmatism in the Vision in Preschoolers Study. Optom Vis Sci. (2014) 91:514–21. doi: 10.1097/OPX.0000000000000242
13. Borchert MS, Varma R, Cotter SA, Tarczy-Hornoch K, McKean-Cowdin R, Lin JH, et al. Risk factors for hyperopia and myopia in preschool children the multi-ethnic pediatric eye disease and Baltimore pediatric eye disease studies. Ophthalmology. (2011) 118:1966–73. doi: 10.1016/j.ophtha.2011.06.030
14. McKean-Cowdin R, Varma R, Cotter SA, Tarczy-Hornoch K, Borchert MS, Lin JH, et al. Risk factors for astigmatism in preschool children: the multi-ethnic pediatric eye disease and Baltimore pediatric eye disease studies. Ophthalmology. (2011) 118:1974–81. doi: 10.1016/j.ophtha.2011.06.031
15. Chua SY, Ikram MK, Tan CS, Stone RA, Cai S, Gluckman PD, et al. Is there a link between passive smoke exposure and early-onset myopia in preschool Asian children? Ophthalmic Physiol Opt. (2016) 36:370–80. doi: 10.1111/opo.12285
16. Shah RL, Huang Y, Guggenheim JA, Williams C. Time outdoors at specific ages during early childhood and the risk of incident myopia. Invest Ophthalmol Vis Sci. (2017) 58:1158–66. doi: 10.1167/iovs.16-20894
17. Deng L, Gwiazda J. Birth season, photoperiod, and infancy refraction. Optom Vis Sci. (2011) 88:383–7. doi: 10.1097/OPX.0b013e31820b0517
18. Ma Q, Xu W, Zhou X, Cui C, Pan CW. The relationship of season of birth with refractive error in very young children in eastern China. PLoS ONE. (2014) 9:e100472. doi: 10.1371/journal.pone.0100472
19. Guo X, Fu M, Ding X, Morgan IG, Zeng Y, He M. Significant axial elongation with minimal change in refraction in 3- to 6-year-old Chinese preschoolers: the shenzhen kindergarten eye study. Ophthalmology. (2017) 124:1826–38. doi: 10.1016/j.ophtha.2017.05.030
20. Lan W, Zhao F, Lin L, Li Z, Zeng J, Yang Z, et al. Refractive errors in 3-6 year-old Chinese children: a very low prevalence of myopia? PLoS ONE. (2013) 8:e78003. doi: 10.1371/journal.pone.0078003
21. Hu Y, Ding X, Long W, He M, Yang X. Longitudinal changes in spherical equivalent refractive error among children with preschool myopia. Invest Ophthalmol Vis Sci. (2019) 60:154–60. doi: 10.1167/iovs.18-24862
22. Shih YF, Ho TC, Hsiao CK, Lin LL. Long-term visual prognosis of infantile-onset high myopia. Eye. (2006) 20:888–92. doi: 10.1038/sj.eye.6702035
23. Chakraborty R, Ostrin LA, Benavente-Perez A, Verkicharla PK. Optical mechanisms regulating emmetropisation and refractive errors: evidence from animal models. Clin Exp Optom. (2020) 103:55–67. doi: 10.1111/cxo.12991
24. Howlett MH, McFadden SA. Form-deprivation myopia in the guinea pig (Cavia porcellus). Vision Res. (2006) 46:267–83. doi: 10.1016/j.visres.2005.06.036
25. Smith EL, III, Hung LF. The role of optical defocus in regulating refractive development in infant monkeys. Vision Res. (1999) 39:1415–35. doi: 10.1016/S0042-6989(98)00229-6
26. Noorden GK, Lewis RA. Ocular axial length in unilateral congenital cataracts and blepharoptosis. Invest Ophthalmol Vis Sci. (1987) 28:750–2.
27. Gee SS, Tabbara KF. Increase in ocular axial length in patients with corneal opacification. Ophthalmology. (1988) 95:1276–8. doi: 10.1016/S0161-6420(88)33035-6
28. He M, Xiang F, Zeng Y, Mai J, Chen Q, Zhang J, et al. Effect of time spent outdoors at school on the development of myopia among children in China: a randomized clinical trial. JAMA. (2015) 314:1142–8. doi: 10.1001/jama.2015.10803
29. Ma Y, Qu X, Zhu X, Xu X, Zhu J, Sankaridurg P, et al. Age-specific prevalence of visual impairment and refractive error in children aged 3-10 years in Shanghai, China. Invest Ophthalmol Vis Sci. (2016) 57:6188–96. doi: 10.1167/iovs.16-20243
30. Jiang X, Tarczy-Hornoch K, Cotter SA, Matsumura S, Mitchell P, Rose KA, et al. Association of parental myopia with higher risk of myopia among multiethnic children before school age. JAMA Ophthalmol. (2020) 138:1–9. doi: 10.1001/jamaophthalmol.2020.0412
31. Seow WJ, Ngo CS, Pan H, Barathi VA, Tompson SW, Whisenhunt KN, et al. In-utero epigenetic factors are associated with early-onset myopia in young children. PLoS ONE. (2019) 14:e0214791. doi: 10.1371/journal.pone.0214791
32. Tideman JWL, Polling JR, Jaddoe VWV, Vingerling JR, Klaver CCW. Growth in foetal life, infancy, and early childhood and the association with ocular biometry Ophthalmic. Physiol Opt. (2019) 39:245–52. doi: 10.1111/opo.12630
33. Rudnicka AR, Kapetanakis VV, Wathern AK, Logan NS, Gilmartin B, Whincup PH, et al. Global variations and time trends in the prevalence of childhood myopia, a systematic review and quantitative meta-analysis: implications for aetiology and early prevention. Br J Ophthalmol. (2016) 100:882–90. doi: 10.1136/bjophthalmol-2015-307724
34. Li H, Ji C, Zong X, Zhang Y. Height and weight standardized growth charts for Chinese children and adolescents aged 0 to 18 years. Chin J Pediatr. (2009) 47:487–92. doi: 10.3760/cma.j.issn.0578-1310.2009.07.003
35. Wilson LB, Melia M, Kraker RT, VanderVeen DK, Hutchinson AK, Pineles SL, et al. Accuracy of autorefraction in children: a report by the american academy of ophthalmology. Ophthalmology. (2020) 127:1259–67. doi: 10.1016/j.ophtha.2020.03.004
36. Prabakaran S, Dirani M, Chia A, Gazzard G, Fan Q, Leo SW, et al. Cycloplegic refraction in preschool children: comparisons between the hand-held autorefractor, table-mounted autorefractor and retinoscopy. Ophthalmic Physiol Opt. (2009) 29:422–6. doi: 10.1111/j.1475-1313.2008.00616.x
Keywords: preschool children, refraction, axial length, parental myopia, outdoor activities
Citation: Ma Y, Lin S, Zhu J, Zhao R, Zhang B, Yin Y, Shao Y, He X, Xu X and Zou H (2022) Effect of Parental Myopia on Change in Refraction in Shanghai Preschoolers: A 1-Year Prospective Study. Front. Pediatr. 10:864233. doi: 10.3389/fped.2022.864233
Received: 31 January 2022; Accepted: 16 March 2022;
Published: 25 April 2022.
Edited by:
Pablo Pérez-Merino, Ghent University, BelgiumReviewed by:
Thiago Gonçalves dos Santos Martins, Federal University of São Paulo, BrazilCopyright © 2022 Ma, Lin, Zhu, Zhao, Zhang, Yin, Shao, He, Xu and Zou. This is an open-access article distributed under the terms of the Creative Commons Attribution License (CC BY). The use, distribution or reproduction in other forums is permitted, provided the original author(s) and the copyright owner(s) are credited and that the original publication in this journal is cited, in accordance with accepted academic practice. No use, distribution or reproduction is permitted which does not comply with these terms.
*Correspondence: Haidong Zou, em91aGFpZG9uZ0Bob3RtYWlsLmNvbQ==; Xun Xu, ZHJ4dXh1bkB0b20uY29t; Xiangui He, eGlhbmhlemlAMTYzLmNvbQ==
†These authors share first authorship
Disclaimer: All claims expressed in this article are solely those of the authors and do not necessarily represent those of their affiliated organizations, or those of the publisher, the editors and the reviewers. Any product that may be evaluated in this article or claim that may be made by its manufacturer is not guaranteed or endorsed by the publisher.
Research integrity at Frontiers
Learn more about the work of our research integrity team to safeguard the quality of each article we publish.