- 1Department of Cardiovascular Medicine, National Clinical Research Center for Child Health and Disorders, Ministry of Education Key Laboratory of Child Development and Disorders, China International Science and Technology Cooperation Base of Child Development and Critical Disorders, Children's Hospital of Chongqing Medical University, Chongqing, China
- 2Chongqing Key Laboratory of Pediatrics, Chongqing, China
Background: Coronary artery lesions including aneurysm, as the most severe complications of Kawasaki disease (KD), remain of great concern. Lipoprotein-associated phospholipase A2 (Lp-PLA2) is implicated in the regulation of inflammatory response and lipid metabolism. Since excessive inflammatory response and aberrant lipid metabolism have involved in the development of KD, we in this study sought to investigate the relationship between coronary artery aneurysm (CAA) and Lp-PLA2 and other blood parameters in children with KD.
Methods: The participants included 71 KD patients, 63 healthy controls (HCs) and 51 febrile controls (FCs). KD patients were divided into KD-CAA (KD with CAA) group and KD-NCAA (KD without CAA) group. Serum Lp-PLA2 levels were measured using enzyme-linked immunosorbent assays. Other routine clinical parameters were also detected.
Results: Serum Lp-PLA2 levels in KD group [4.83 μg/mL (3.95–6.77)] were significantly higher than those in HC [1.29 μg/mL (0.95–2.05)] and FC [1.74 μg/mL (1.18–2.74)] groups. KD-CAA group [5.56 μg/mL (4.55–22.01)] presented substantially higher serum Lp-PLA2 levels as compared with KD-NCAA group [4.64 μg/mL (2.60–5.55)]. In KD group, serum Lp-PLA2 level was positively related with erythrocyte sedimentation rate, the levels of leukocytes, platelets, albumin, creatine kinase-MB, and D-dimer, and the Z-scores of left main CA, right CA, left anterior descending CA, and left circumflex CA; and negatively related with mean corpuscular hemoglobin concentration and mean platelet volume. Moreover, receiver operating characteristic curves showed that Lp-PLA2 exhibited superior and moderate diagnostic performance for distinguishing KD patients from HC and FC ones, respectively, and possessed the potential ability to predict the occurrence of CAAs in KD.
Conclusion: Lp-PLA2 may be related to KD and the formation of CAAs, and thus may serve as a potential diagnostic biomarker for KD.
Background
Kawasaki disease (KD), also known as mucocutaneous lymph node syndrome, is an acute febrile pediatric disorder characterized by systemic and self-limited vasculitis in small-sized and especially medium-sized arteries (1). KD predominantly afflicts infants and children under age of 5 years. Its pathogenesis has been universally attributed to inflammatory cascade and aberrant immunoreaction. Coronary artery aneurysm (CAA) is recognized as the most important complication of KD and is a high-risk factor for myocardial ischemia, infarction, and even coronary artery rupture (2, 3). Although KD was initially reported by Dr. Tomisaku Kawasaki in 1967 (4), its specific pathogenesis remains obscure. Intravenous immunoglobulin (IVIG) still persists as the mainstay of KD treatment. However, KD in ~10–20% of its patients fail to response to IVIG treatment, and it is more likely to bring about CAAs (2, 5–8). KD has become the leading cause of pediatric acquired heart disease in the developed countries (2). It is urgent, therefore, to further uncover the potential pathogenesis of the development of KD and CAAs, and find more effective treatment strategies.
Lipoprotein-associated phospholipase A2 (Lp-PLA2) is a 45kDa calcium-independent hydrophobic protein that belongs to the phospholipase A2 superfamily (9). Lp-PLA2 is predominantly secreted by monocytes and macrophages, and mainly exists in combination with low-density and high-density lipoprotein particles in blood circulation (10, 11). It was initially identified as an enzyme capable of hydrolyzing platelet-activating factor and truncate phospholipids in the settings of inflammation and oxidant stress (12, 13), and it was deemed as a mediator of inflammation and lipid metabolism (14). Mounting studies have demonstrated recently that Lp-PLA2 is associated with coronary heart disease (15–17). At present, Lp-PLA2 has been applied as a potential biomarker for predicting the risk of coronary heart disease and ischemic stroke associated with atherosclerosis (15, 18). Certain researches have proved that KD is a kind of systematic vasculitis caused by excessive inflammatory response (2). Taken together, it seems that the dysregulation of lipid metabolism may be one of the potential pathogenic mechanisms of KD (19, 20). We therefore speculated that Lp-PLA2 might be related to KD and its complications. Thus, we in this study aimed to investigate the relationships between Lp-PLA2 and KD, and Lp-PLA2 and CAA.
Methods
Participants and Sample Collection
In this retrospective study, all the participants were enrolled from Children's Hospital of Chongqing Medical University (Chongqing, China). The diagnosis criteria of KD were rigorously based on the guidelines proposed by Japanese Circulation Society Joint Working Group (21). KD patients with inflammatory diseases, immune diseases, metabolic diseases, hematological diseases or other heart diseases were excluded. A total of 71 children with KD were recruited (male: 46, female: 25, age: 29.44 ± 22.52 months) in KD group. We also recruited 63 healthy children (male: 41, female: 22, age: 30.33 ± 11.59 months) from health checkup for HC (healthy control) group, and 51 children (male: 29, female: 22, age: 28.05 ± 14.68 months) with acute febrile disease for FC (febrile control) group (Supplementary Table S1). FCs included patients with bronchopneumonia, infectious diarrhea, urinary tract infection, sepsis, hand-foot-mouth disease, or infectious mononucleosis.
Echocardiography was performed 1 day before treatment with intravenous immunoglobulin (IVIG) and anticoagulants in KD children. All the cardiac ultrasound examinations were performed by the expert physicians who were unblinded to the clinical information. Patients with Z-score > 2.5 were allocated into KD-CAA (KD patients with CAAs) group (n = 33), and those with Z-score <2.5 were into KD-NCAA (KD patients without CAAs) group (n = 38) (22).
All venous blood samples were collected before treatment with IVIG and anticoagulants. The samples were centrifuged at 3,000 rpm for 10 min to separate serum and red blood cells, and then stored at −80°C. Same sampling procedure was performed in healthy controls and febrile controls.
This study was approved by the Ethics Committee of Children's Hospital, Chongqing Medical University (Chongqing, China), and informed consents were obtained from the parents or guardians. All methods were carried out in accordance with relevant guidelines and regulations addressed in the Declaration of Helsinki.
Measurement of Serum Levels of Lp-PLA2 and Routine Blood Parameters
Serum levels of Lp-PLA2 were determined by quantitative enzyme-linked immunosorbent assay as per the manufacturer's instructions (Cloud-Clone Corp USCN Life Science, Wuhan, China). Routine blood parameters were also measured in the blood samples, including white blood cell (WBC) count, percentage of neutrophils, percentage of leukomonocytes, red blood cell count, hemoglobin, mean corpuscular volume, mean corpuscular hemoglobin concentration (MCHC), erythrocyte sedimentation rate (ESR), procalcitonin, aspartate aminotransferase, alanine aminotransferase, albumin (ALB), creatine kinase-MB (CK-MB), platelet (PLT) count, C-reactive protein, Z-score of left main coronary artery (LMCA), Z-score of right coronary artery (RCA), Z-score of left anterior descending coronary artery (LAD), Z-score of left circumflex coronary artery (LCX), as well as blood coagulation parameters such as prothrombin time, activated partial thromboplastin time, fibrinogen, thrombin time and D-dimer (DD).
Statistical Analysis
All statistical analyses were performed using the SPSS software for Windows (Version 25.0, SPSS, Chicago, IL, USA). Student's t-test was used for the data simulated from a normal distribution, and the nonparametric Mann-Whitney U test was applied to analyze the data that failed the normality assumption. Chi-square test was used for comparisons of frequencies between groups. Spearman's rank correlation was used to measure the correlation between serum Lp-PLA2 level and other clinical parameters. Receiver operating characteristic (ROC) curves were plotted and areas under the curves (AUC) were calculated to evaluate the diagnostic performance of Lp-PLA2 for KD. All values were shown as mean ± standard deviation, median (P25-P75) or number (percentage). Two-sided p-values < 0.05 were considered statistically significant.
Results
Characterization of Serum Lp-PLA2 and Routine Clinical Parameters in All Groups
As presented in Figure 1, serum Lp-PLA2 levels in KD group [4.83 μg/mL (3.95–6.77)] were significantly higher than those in HC [1.29 μg/mL (2.05)] and FC groups [1.74 μg/mL (1.18–2.74)]. KD-CAA group [5.56 μg/mL (4.55–22.01)] showed substantially higher Lp-PLA2 level in contrast to KD-NCAA group [4.64 μg/mL (2.60–5.55)].
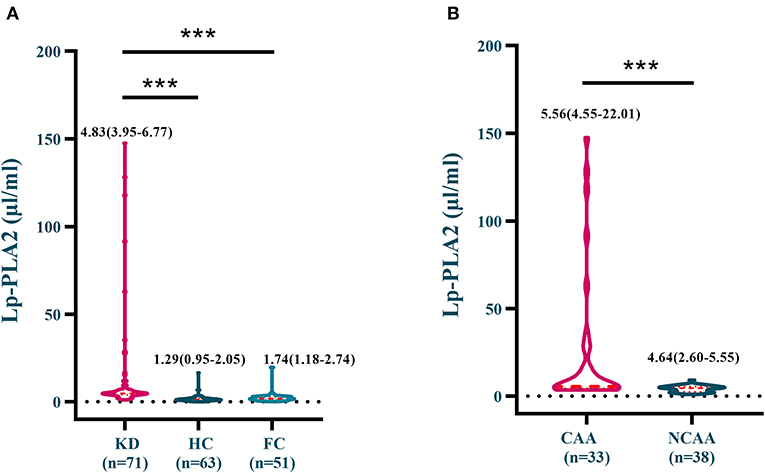
Figure 1. (A) Serum Lp-PLA2 levels in the KD, the HC and the FC groups; (B) Serum Lp-PLA2 levels in the KD-CAA and the KD-NCAA groups. ***p < 0.0001.
There was no significant difference in percentage of neutrophils, percentage of lymphocytes, red blood cell count, mean corpuscular volume, ESR, and the levels of hemoglobin, procalcitonin, aspartate aminotransferase, alanine aminotransferase, ALB, CK-MB, and C-reactive protein between KD-CAA and KD-NCAA groups. However, KD-CAA group presented significantly higher WBC and PLT levels and lower MCHC by contrast with KD-NCAA group (Table 1).
As shown in Tables 2–4, serum Lp-PLA2 levels, in KD group, were positively related with ESR, the levels of WBC, ALB, CK-MB, and the Z-scores of LMCA, RCA, LAD and LCX, and negatively related with MCHC. Likewise, KD-CAA group saw a similar trend in terms of those parameters, and in addition, its serum Lp-PLA2 levels were strongly related to WBC level and the Z-scores of aforementioned branches of coronary arteries.
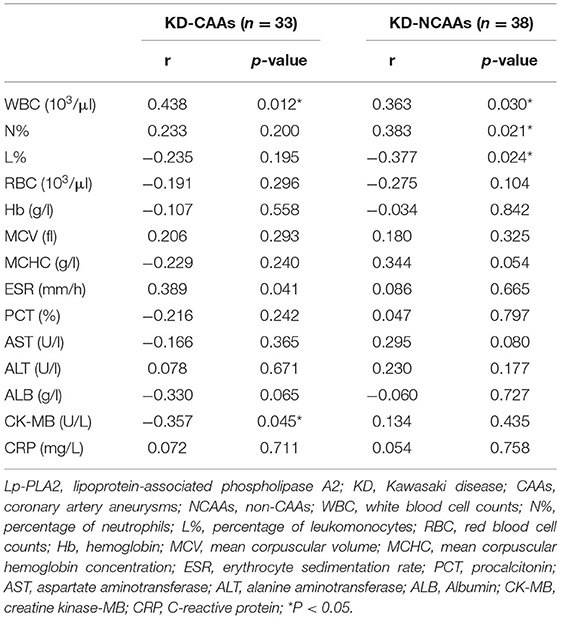
Table 3. Correlations between serum Lp-PLA2 levels and general laboratory variables in KD-CAAs and KD-NCAAs groups.
With regards to blood coagulation, serum Lp-PLA2 levels were positively related with PLT and DD levels in KD and KD-CAA groups. Moreover, in KD group, serum Lp-PLA2 levels were negatively related with MPV (Table 5).
Diagnostic Performance of Lp-PLA2 for KD
The diagnostic performance of Lp-PLA2 between KD patients and HCs, and KD patients & FCs were verified using receiver operating characteristic (ROC) analysis. As shown in Figures 2A,B, Lp-PLA2 exhibited superior diagnostic performance in distinguishing the KD patients from HCs. The AUC value was 0.939 (95% confidence interval 0.895–0.983, P < 0.0001), and the cut-off value was 1.743 μg/mL with sensitivity and specificity ratios of 69.84% and 98.59%, respectively. In addition, Lp-PLA2 possessed capacity to distinguish the KD patients from FCs. The ROC analysis showed that the AUC value was 0.910 (95% confidence interval 0.856–0.964, P < 0.0001), and the cut-off value was 1.756 μg/mL with sensitivity and specificity ratios of 50.98 and 98.59%, respectively. As presented in Figure 2C, Lp-PLA2 also had moderate diagnostic performance for predicting CAAs in the KD patients. The AUC value was 0.725 (95% confidence interval 0.606–0.844, P = 0.001), and the cut-off value was 3.700 μg/mL with sensitivity and specificity ratios of 38.64 and 96.97%, respectively.
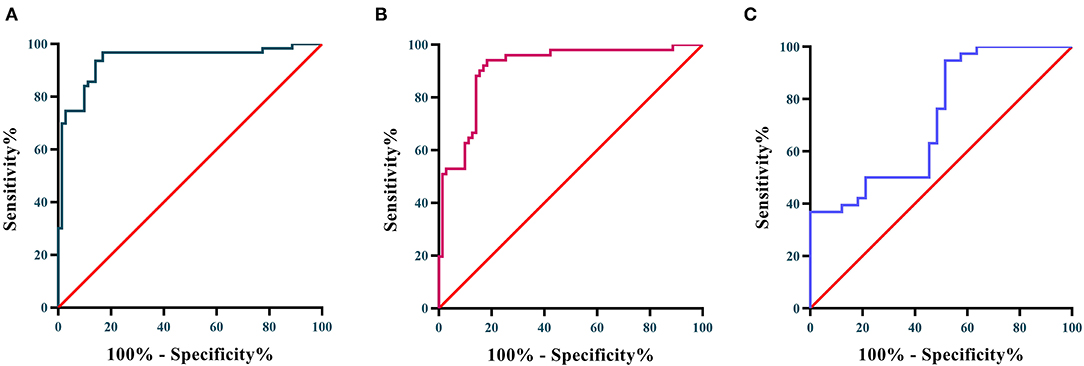
Figure 2. (A) ROC curves of Lp-PLA2 for distinguishing KD patients from HCs; (B) ROC curve of Lp-PLA2 for distinguishing KD from FCs; (C) ROC curve of Lp-PLA2 for predicting the occurrence of CAA.
Discussion
Lp-PLA2, conventionally known as platelet-activating factor acetyl hydrolase, is a unique member of the A2 phospholipase superfamily (23). It is synthesized mainly by macrophages and monocytes and binds to LDL and HDL particles in blood circulation (10). Previous studies have demonstrated the strong association of Lp-PLA2 with some inflammation-related cardiovascular diseases (14, 18, 24), and its involvement in the regulation of inflammation and lipid metabolism (25). KD is recognized as a severe inflammatory disorder associated with systemic vasculitis of unclear etiology. Excessive inflammation due to the presence of certain inflammatory mediators has been universally perceived as the contributor to KD development (2). Moreover, recent studies have found that adipocytokines like resistin and adiponectin were aberrantly expressed in the acute phase of KD (19, 26). This phenomenon indicates that abnormal lipid metabolism may also contribute to the formation of KD.
In the current study, we found that serum Lp-PLA2 levels were significantly increased in the KD patients. In particular, KD patients complicated with CAAs had substantial higher serum Lp-PLA2 levels. Moreover, we performed correlation analyses and found serum Lp-PLA2 levels were positively related to ESR, the levels of WBC, ALB, CK-MB, PLT, and DD, and the Z-scores of LMCA, RCA, LAD, and LCX, but negatively associated with MCHC and MPV. Furthermore, we found Lp-PLA2 exhibited superior diagnostic performance for distinguishing KD patients from healthy and febrile children, and also possessed the capacity to predict the occurrence of CAAs.
Lp-PLA2 is initially identified as an enzyme that contributes to degradation and inactivation of platelet-activating factor. It is also responsible for catalyzing hydrolysis of phospholipids within oxidized LDL molecules at the sn-2 position, which could contribute to the generation of pro-atherogenic and pro-inflammatory mediators, lysophosphatidylcholine and oxidized non-esterified fatty acids, and thus lead to the dysfunction of endothelial cells and smooth muscle cells (9, 12). Researches have shown that the damage to vascular endothelial cells and smooth muscle cells caused by excessive inflammatory response plays a critical role in the development of KD (1). This study demonstrated that serum Lp-PLA2 levels were significantly elevated in the KD patients, and were positively related to inflammation-related clinical parameters such as WBC and ESR. It indicated that Lp-PLA2 was more likely to be a pathogenic factor in KD. Besides, Lp-PLA2 also has an anti-inflammatory and anti-atherogenic role. Introduction of Lp-PLA2 gene into apoE-deficient mice would lead to a reduction in the mean arterial wall thickness and less spontaneous atherosclerosis (11). This phenomenon is due to the failure of mouse LDL to bind to Lp-PLA2 and thus the elevation of serum HDL-associated Lp-PLA2. Lp-PLA2 exerts its anti- or pro-inflammatory, and anti- or pro-atherogenic effects depending on its binding to high-density or low-density lipoproteins. Therefore, further studies are required to demonstrate whether Lp-PLA2 is involved in KD as a pathogenic or protective factor. Moreover, previous studies found that adipocytokines was significantly up-regulated in the KD patients (19, 26), and resistin could aggravate coronary artery lesions via nuclear factor-kB signaling pathway in KD (20). Lp-PLA2 and resistin, mainly expressed by macrophages, are both coincidentally involved in some immune-related or lipid-related diseases (27), such as rheumatoid arthritis (27, 28), atherosclerosis (23, 29), obesity, and diabetes (30). There might be an association between Lp-PLA2 and resistin. Based on the results and these evidence, we could speculate that Lp-PLA2 might be related to KD, However, further studies are required to verify the findings.
CAA is the most important complication of KD, and ~20% KD patients would develop CAAs even after standard treatment (infusion of IVIG) (2, 3). Therefore, novel therapy targets are critically required for improving the prognosis of KD patients. In this study, we found that serum Lp-PLA2 levels in KD-CAA group were significantly higher than those in KD-NCAA group. Correlation analysis showed that serum Lp-PLA2 levels were positively related with Z-scores of LMCA, RCA, LAD, and LCX. These results implied that Lp-PLA2 might strongly related to the formation of CAAs. Numerous studies have demonstrated that Lp-PLA2 is related to coronary heart disease. In atherosclerosis, Lp-PLA2 and its hydrolyzed products, viz, lysophosphatidylcholine, are abundant in the necrotic cores and thin fibrous caps of the lesions (10). Lp-PLA2 would be released throughout the body from macrophages and monocytes, possibly under the stimulation of certain proinflammatory cytokines, and is mainly carried by LDL in blood circulation (11). The LDL and Lp-PLA2 complexes penetrate the artery wall and locate in the subendothelial space. Additionally, in a deleterious feed-forward manner, Lp-PLA2 in the pathological areas could recruit macrophages, T-cells and mast cells, which might cause further release of Lp-PLA2 (10, 23). Certain CD163-positive macrophages were observed in the lesions of vessels in the acute phase of KD (31). It is relatively straightforward to envisage a scenario that Lp-PLA2 was released by macrophages under inflammation and accumulated in the artery wall; subsequently, macrophages would be recruited by Lp-PLA2 in the artery wall and might further release Lp-PLA2 and other inflammatory cytokines, and eventually lead to the damage to and dysfunction of endothelial cells and smooth muscle cells that contribute to CAAs.
Clinical researches have shown that high serum Lp-PLA2 levels were more likely to have a coronary event in atherosclerosis, and Lp-PLA2 was used as a biomarker for predicting a patient's risk for coronary heart disease and ischemic stroke associated with atherosclerosis (24). In this study, we analyzed the diagnostic performance of Lp-PLA2 using ROC analysis, along with the calculation of AUC values. We found Lp-PLA2 had superior diagnostic performance for distinguishing the KD patients from healthy children, with an AUC value of 0.921; and the cut-off value was 1.688 μg/mL with sensitivity and specificity ratios of 61.90 and 98.59%, respectively. In addition, Lp-PLA2 had moderate diagnostic performance for differentiating the KD patients from febrile children. Reliable predictive biomarkers for CAAs are still scarce (32) although there are various biomarkers for the diagnosis of KD and the prediction of IVIG-resistance (33, 34). In this study, we found that serum Lp-PLA2 had modest diagnostic potential for predicting the occurrence of CAAs, and the AUC value was 0.725 (95% confidence interval 0.606–0.844, P = 0.001), with sensitivity and specificity ratios of 38.64% and 96.97% respectively under the most appropriated cut-off value of 3.700 μg/mL. These findings might facilitate the early diagnosis and management of CAAs in KD patients.
Conclusions
Taken together, serum Lp-PLA2 levels in KD patients were significantly higher than those in healthy and febrile controls. In addition, KD patients with CAAs had substantially higher serum Lp-PLA2 levels in contrast to the ones without CAAs. Moreover, Lp-PLA2 was positively related to ESR, the levels of WBC, ALB, CK-MB, PLT, and DD, and the Z-scores of LMCA, RCA, LAD, and LCX, but negatively related with MCHC and MPV in KD group. When Lp-PLA2 is at the crossroads of lipid metabolism and the inflammatory response, it presents pro-inflammation and proatherogenic effects in some inflammation-related cardiovascular diseases. Base on above findings and evidence, we might conclude that Lp-PLA2 is related to KD and the formation of CAAs. Furthermore, Lp-PLA2 showed superior diagnostic performance for distinguishing KD patients from healthy or febrile controls, and might serve as a potential biomarker for predicting the occurrence of CAAs in KD. These findings might facilitate the early diagnosis and management of KD.
Study Limitations
There are some limitations in our study. Firstly, the sample size was small, and increasing our sample size could help our results become more convincing. Secondly, whether high levels of serum Lp-PLA2 were the results or the causes of KD was not clear. Finally, the potential mechanisms that Lp-PLA2 may involve in KD and CAA were required to explore. Taken together, future studies are required to further confirm and validate our findings.
Data Availability Statement
The raw data supporting the conclusions of this article will be made available by the authors, without undue reservation.
Ethics Statement
The studies involving human participants were reviewed and approved by Ethics Committee of Children's Hospital, Chongqing Medical University. Written informed consent to participate in this study was provided by the participants' legal guardian/next of kin.
Author Contributions
ZC conceived and designed the study, acquired and analyzed the data, and drafted the paper. HW designed the study, interpreted the data, and revised the manuscript. JZ collected the blood samples. QY critically reviewed and revised the manuscript for important intellectual content and approved its final version. All authors approved the submission of the manuscript in its current form.
Funding
This work is funded by National Natural Science Foundation of China (No. 81270412).
Conflict of Interest
The authors declare that the research was conducted in the absence of any commercial or financial relationships that could be construed as a potential conflict of interest.
Publisher's Note
All claims expressed in this article are solely those of the authors and do not necessarily represent those of their affiliated organizations, or those of the publisher, the editors and the reviewers. Any product that may be evaluated in this article, or claim that may be made by its manufacturer, is not guaranteed or endorsed by the publisher.
Acknowledgments
All parents/guardians that agreed for their children/wards to participate in this study are truly acknowledged.
Supplementary Material
The Supplementary Material for this article can be found online at: https://www.frontiersin.org/articles/10.3389/fped.2022.854079/full#supplementary-material
Abbreviations
ALB, albumin; AUC, area under the curve; CAA, coronary artery aneurysm; CK-MB, creatine kinase-MB; DD, D-dimer; ESR, erythrocyte sedimentation rate; FC, febrile control; HC, healthy control; HDL, high-density lipoprotein; IVIG, Intravenous immunoglobulin; KD, Kawasaki disease; LAD, left anterior descending coronary artery; LCX, left circumflex coronary artery; LDL, low-density lipoprotein; LMCA, left main coronary artery; Lp-PLA2, lipoprotein-associated phospholipase A2; MCHC, mean corpuscular hemoglobin concentration; NCAA, non-CAA; PLT, platelet; RCA, right coronary artery; ROC, receiver operating characteristic; WBC, white blood cell.
References
1. Shulman ST, Rowley AH. Kawasaki disease: insights into pathogenesis and approaches to treatment. Nat Rev Rheumatol. (2015) 11:475–82. doi: 10.1038/nrrheum.2015.54
2. Newburger JW, Takahashi M, Burns JC. Kawasaki Disease. J Am Coll Cardiol. (2016) 67:1738–49. doi: 10.1016/j.jacc.2015.12.073
3. Rife E, Gedalia A. Kawasaki disease: an update. Curr Rheumatol Rep. (2020) 22:75. doi: 10.1007/s11926-020-00941-4
4. Kawasaki T. Acute febrile mucocutaneous syndrome with lymphoid involvement with specific desquamation of the fingers and toes in children. Arerugi. (1967) 16:178–222.
5. Eleftheriou D, Levin M, Shingadia D, Tulloh R, Klein NJ, Brogan PA. Management of Kawasaki disease. Arch Dis Child. (2014) 99:74–83. doi: 10.1136/archdischild-2012-302841
6. Kobayashi T, Inoue Y, Takeuchi K, Okada Y, Tamura K, Tomomasa T, et al. Prediction of intravenous immunoglobulin unresponsiveness in patients with Kawasaki disease. Circulation. (2006) 113:2606–12. doi: 10.1161/CIRCULATIONAHA.105.592865
7. McCrindle BW, Rowley AH, Newburger JW, Burns JC, Bolger AF, Gewitz M, et al. Diagnosis, treatment, and long-term management of kawasaki disease: a scientific statement for health professionals From the American Heart Association. Circulation. (2017) 135:e927–99. doi: 10.1161/CIR.0000000000000484
8. Noval Rivas M, Arditi M. Kawasaki disease: pathophysiology and insights from mouse models. Nat Rev Rheumatol. (2020) 16:391–405. doi: 10.1038/s41584-020-0426-0
9. Dennis EA, Cao J, Hsu YH, Magrioti V, Kokotos G. Phospholipase A2 enzymes: physical structure, biological function, disease implication, chemical inhibition, and therapeutic intervention. Chem Rev. (2011) 111:6130–85. doi: 10.1021/cr200085w
10. Huang F, Wang K, Shen J. Lipoprotein-associated phospholipase A2: the story continues. Med Res Rev. (2020) 40:79–134. doi: 10.1002/med.21597
11. Packard CJ. Role of lipoprotein-associated phospholipase A2 in vascular disease. In: Ballantyne CM, editor. Clinical Lipidology. Philadelphia: W.B. Saunders (2009). p. 167–77. doi: 10.1016/B978-141605469-6.50018-4
12. Jialal I, Devaraj S. Modulation of biomarkers of inflammation. In: Ballantyne CM, editor. Clinical lipidology. Philadelphia: W.B. Saunders (2009). p. 396–409. doi: 10.1016/B978-141605469-6.50037-8
13. Stafforini DM. Chapter six—plasma PAF-AH (PLA2G7): biochemical properties, association with LDLs and HDLs, and regulation of expression. In: Inoue K, Stafforini DM, Tamanoi F, editors. The Enzymes. Academic Press (2015). p. 71–93. doi: 10.1016/bs.enz.2015.09.004
14. Epps KC, Wilensky RL. Lp-PLA(2)- a novel risk factor for high-risk coronary and carotid artery disease. J Intern Med. (2011) 269:94–106. doi: 10.1111/j.1365-2796.2010.02297.x
15. Younus A, Humayun C, Ahmad R, Ogunmoroti O, Kandimalla Y, Aziz M, et al. Lipoprotein-associated phospholipase A2 and its relationship with markers of subclinical cardiovascular disease: a systematic review. J Clin Lipidol. (2017) 11:328–37. doi: 10.1016/j.jacl.2017.02.005
16. Yin YJ, Chen YC, Xu L, Zhao XH, Song Y. Relationship of lipoprotein-associated phospholipase A2(Lp-PLA2) and periprocedural myocardial injury in patients undergoing elective percutaneous coronary intervention. Int J Cardiol Heart Vasc. (2020) 28:100541. doi: 10.1016/j.ijcha.2020.100541
17. Bonnefont-Rousselot D. Lp-PLA2, a biomarker of vascular inflammation and vulnerability of atherosclerosis plaques. Ann Pharm Fr. (2016) 74:190–7. doi: 10.1016/j.pharma.2015.09.002
18. Maiolino G, Bisogni V, Rossitto G, Rossi GP. Lipoprotein-associated phospholipase A2 prognostic role in atherosclerotic complications. World J Cardiol. (2015) 7:609–20. doi: 10.4330/wjc.v7.i10.609
19. Liu R, He B, Gao F, Liu Q, Yi Q. Relationship between adipokines and coronary artery aneurysm in children with Kawasaki disease. Transl Res. (2012) 160:131–6. doi: 10.1016/j.trsl.2012.01.013
20. Gao F, Si F, Feng S, Yi Q, Liu R. Resistin enhances inflammatory cytokine production in coronary artery tissues by activating the NF-kappaB signaling. Biomed Res Int. (2016) 2016:3296437. doi: 10.1155/2016/3296437
21. Group JCSJW. Guidelines for diagnosis and management of cardiovascular sequelae in Kawasaki disease (JCS 2013). Digest version Circ J. (2014) 78:2521–62. doi: 10.1253/circj.CJ-66-0096
22. Manlhiot C, Millar K, Golding F, McCrindle BW. Improved classification of coronary artery abnormalities based only on coronary artery z-scores after Kawasaki disease. Pediatr Cardiol. (2010) 31:242–9. doi: 10.1007/s00246-009-9599-7
23. Zalewski A, Macphee C. Role of lipoprotein-associated phospholipase A2 in atherosclerosis: biology, epidemiology, and possible therapeutic target. Arterioscler Thromb Vasc Biol. (2005) 25:923–31. doi: 10.1161/01.ATV.0000160551.21962.a7
24. French D, Wu AHB. Cardiac Markers. In: Wild D, editor. The Immunoassay Handbook. Oxford: Elsevier (2013). p. 817–31. doi: 10.1016/B978-0-08-097037-0.00066-X
25. Tellis CC, Tselepis AD. Pathophysiological role and clinical significance of lipoprotein-associated phospholipase A(2) (Lp-PLA(2)) bound to LDL and HDL. Curr Pharm Des. (2014) 20:6256–69. doi: 10.2174/1381612820666140622200916
26. Kim HJ, Choi EH, Kil HR. Association between adipokines and coronary artery lesions in children with Kawasaki Disease. J Korean Med Sci. (2014) 29:1385–90. doi: 10.3346/jkms.2014.29.10.1385
27. Vachatova S, Andrys C, Krejsek J, Salavec M, Ettler K, Rehacek V, et al. Metabolic Syndrome and selective inflammatory markers in psoriatic patients. J Immunol Res. (2016) 2016:5380792. doi: 10.1155/2016/5380792
28. Lourida ES, Georgiadis AN, Papavasiliou EC, Papathanasiou AI, Drosos AA, Tselepis AD. Patients with early rheumatoid arthritis exhibit elevated autoantibody titers against mildly oxidized low-density lipoprotein and exhibit decreased activity of the lipoprotein-associated phospholipase A2. Arthritis Res Ther. (2007) 9:R19. doi: 10.1186/ar2129
29. Jung HS, Park KH, Cho YM, Chung SS, Cho HJ, Cho SY, et al. Resistin is secreted from macrophages in atheromas and promotes atherosclerosis. Cardiovasc Res. (2006) 69:76–85. doi: 10.1016/j.cardiores.2005.09.015
30. Jackisch L, Kumsaiyai W, Moore JD, Al-Daghri N, Kyrou I, Barber TM, et al. Differential expression of Lp-PLA2 in obesity and type 2 diabetes and the influence of lipids. Diabetologia. (2018) 61:1155–66. doi: 10.1007/s00125-018-4558-6
31. Sato W, Yokouchi Y, Oharaseki T, Asakawa N, Takahashi K. The pathology of Kawasaki disease aortitis: a study of 37 cases. Cardiovasc Pathol. (2021) 51:107303. doi: 10.1016/j.carpath.2020.107303
32. Weng H, Pei Q, Yang M, Zhang J, Cheng Z, Yi Q. Hypomethylation of C1q/tumor necrosis factor-related protein-1 promoter region in whole blood and risks for coronary artery aneurysms in Kawasaki disease. Int J Cardiol. (2020) 307:159–63. doi: 10.1016/j.ijcard.2020.02.002
33. Kentsis A, Shulman A, Ahmed S, Brennan E, Monuteaux MC, Lee YH, et al. Urine proteomics for discovery of improved diagnostic markers of Kawasaki disease. EMBO Mol Med. (2013) 5:210–20. doi: 10.1002/emmm.201201494
34. Kuo HC, Chang JC, Kuo HC Yu HR, Wang CL, Lee CP, et al. Identification of an association between genomic hypomethylation of FCGR2A and susceptibility to Kawasaki disease and intravenous immunoglobulin resistance by DNA methylation array. Arthritis Rheumatol. (2015) 67:828–36. doi: 10.1002/art.38976
Keywords: Kawasaki disease, lipoprotein-associated phospholipase A2, coronary artery aneurysm, children, pediatric cardiology
Citation: Cheng Z, Weng H, Zhang J and Yi Q (2022) The Relationship Between Lipoprotein-Associated Phospholipase-A2 and Coronary Artery Aneurysm in Children With Kawasaki Disease. Front. Pediatr. 10:854079. doi: 10.3389/fped.2022.854079
Received: 13 January 2022; Accepted: 07 March 2022;
Published: 31 March 2022.
Edited by:
Emanuele Monda, University of Campania Luigi Vanvitelli, ItalyCopyright © 2022 Cheng, Weng, Zhang and Yi. This is an open-access article distributed under the terms of the Creative Commons Attribution License (CC BY). The use, distribution or reproduction in other forums is permitted, provided the original author(s) and the copyright owner(s) are credited and that the original publication in this journal is cited, in accordance with accepted academic practice. No use, distribution or reproduction is permitted which does not comply with these terms.
*Correspondence: Qijian Yi, qjyi2003@hotmail.com