- 1Avera McKennan Hospital and University Medical Center, Avera Research Institute, Sioux Falls, SD, United States
- 2Center for Vaccines and Immunity, The Abigail Wexner Research Institute at Nationwide Children's Hospital, Columbus, OH, United States
- 3Division of Neonatology, Department of Pediatrics, Nationwide Children's Hospital, The Ohio State University College of Medicine, Columbus, OH, United States
- 4Division of Infectious Diseases, Department of Pediatrics, Nationwide Children's Hospital, The Ohio State University College of Medicine, Columbus, OH, United States
- 5Center for Perinatal Research, The Abigail Wexner Research Institute at Nationwide Children's Hospital, Columbus, OH, United States
Congenital CMV (cCMV) infection can affect infants born to mothers with preconceptional seroimmunity. To prevent cCMV due to nonprimary maternal infection, vaccines eliciting responses exceeding natural immunity may be required. Anti-gM/gN antibodies have neutralizing capacity in-vitro and in animal models, but anti-gM/gN antibodies have not been characterized among seroimmune pregnant women. Paired maternal and infant cord sera from 92 CMV seropositive mothers and their full-term or preterm infants were tested for anti-gM/gN antibody titers in comparison with anti-gB titers and neutralizing activity. Anti-gM/gN titers were significantly lower than anti-gB titers for all groups and did not correlate with serum neutralizing capacity. Further study is needed to determine if higher anti-gM/gN antibody titers might enhance serum neutralizing capacity among seropositive adults.
Introduction
Congenital cytomegalovirus (cCMV) infection is the most common congenital viral infection, affecting approximately 0.5% of newborns in the United States (1, 2). Neurodevelopmental impairments and sensorineural hearing loss can affect children with cCMV infection, with substantial personal and societal consequences (1, 3–5). Preventing cCMV infection remains a major clinical challenge. Women with preconceptional seroimmunity have lower risk of vertically transmitting CMV compared to women lacking preconceptional seroimmunity, but due to high CMV seroprevalence among women of childbearing age worldwide, a greater total number of cCMV-infected infants are born to women with nonprimary infection (4, 6–9). For seropositive women, vaccines may need to elicit serological responses exceeding that of natural immunity to prevent cCMV transmission and/or damaging sequelae for fetuses infected in utero.
Current candidate vaccines target CMV glycoprotein B (gB), the pentameric complex (gH/gL/gpUL128-131), pp65, and the IE1/2 gene products (10). To prevent primary CMV infection among seronegative pregnant women, a CMV vaccine targeting gB was tested among CMV seronegative women of childbearing age in a Phase II clinical trial and showed a vaccine efficacy of 50% (11). This vaccine elicited virus-neutralizing anti-gB antibodies at lower titers than natural infection, and its efficacy was thought to be partially due to antibody-dependent cellular phagocytosis by non-neutralizing antibodies (12, 13). Antibodies against the pentameric complex have potent neutralizing activity in-vitro and in animal models and can be elicited by vaccination (14–17). However, anti-pentamer antibody titers did not differ between women who transmitted CMV congenitally to their infants and non-transmitting mothers (18).
In addition to gB and the pentameric complex, CMV also encodes a third surface glycoprotein complex consisting of glycoproteins M and N (gM/gN). Glycoprotein M is the most abundant component of the viral proteome and is complexed to glycoprotein N, which exhibits sequence variation and glycosylation consistent with immune evasion functions (19–22). This complex elicits antibodies with in vitro neutralizing capacity that are detectable among seropositive adults and in CMV hyperimmune globulin (19, 23–27). In mice, co-immunization with DNA vaccines encoding murine CMV (MCMV) gM and gN provided mice with complete protection against lethal MCMV challenge (28). These studies support the potential utility of including gM/gN antigens in an HCMV vaccine, but it is unknown whether such a vaccine could enhance humoral responses among seropositive individuals. Anti-gM/gN antibody titers during pregnancy and their transplacental transmission to the infant in mother-infant dyads delivered at various gestational ages have not been characterized. In this study, we utilized an institutional biorepository to quantify anti-gM/gN antibody titers in mother-infant dyads in comparison with anti-gB titers and neutralizing activity.
Materials and Methods
Patient Population
For this retrospective cohort study, subjects from the Ohio Perinatal Research Network Pediatric Research Repository (OPRN PRR) who had paired maternal and infant umbilical cord sera from singleton pregnancies were identified. Maternal third trimester sera were screened by enzyme-linked immunoassay (ELISA) for CMV IgM and IgG. For subjects with IgG+/IgM- third trimester results, the serostatus was confirmed using first trimester maternal sera to exclude women with primary infection during pregnancy. Seropositive mothers with sufficient maternal and infant sera available for analysis were included in this study. Informed consent was obtained for enrollment in the PRR through an IRB-approved protocol (NCH IRB10-00035). Demographic and clinical characteristics of mothers and infants were collected through the OPRN PRR.
ELISA Assay
Expression plasmids encoding full-length HCMV ORF UL55 (gB), UL100 (gM) or UL73 (gN) (26) were transfected into 293T cells (American Type Culture Collection, Manassas VA) using Mirus LT-1 reagent (Mirus Bio LLC, Madison WI). Expression of gB or gM/gN was confirmed by immunofluorescence staining using monoclonal antibodies (mabs) for gB (IgG CH-28, Santa Cruz Biotechnology, Santa Cruz CA) or the gM/gN complex (IgM mab 14-16A, gift of W. Britt, University of Alabama at Birmingham). Transfected cell lysates were used for anti-gB or anti-gM/gN ELISAs and were validated using monoclonal antibodies and sera from known seropositive and seronegative individuals. The glycoprotein-specific antibody transplacental transmission ratio was calculated as the infant titer/maternal titer for each infant-mother dyad.
Neutralization Assay
HCMV strain AD169 with a repaired mutation in UL128-131 and expressing green fluorescent protein (GFP) (gift of T. Shenk, Princeton University, Princeton NJ) was utilized for the neutralization assays. CMV was incubated with maternal or infant sera in 2-fold serial dilution, with and without guinea pig complement (CedarLane Laboratories Limited, Ontario Canada) prior to infecting fibroblasts in duplicate as previously described (26, 29, 30). For these assays, complement was included to identify potential differences in neutralizing capacity mediated by complement-fixing antibodies in comparison with non-complement-fixing antibodies (31) Control wells were infected with CMV, with or without complement. At 72 h post-infection, green fluorescent protein (GFP)-expressing cells were quantified using an EVOS cell imaging system (ThermoFisher) and Image J software (https://imagej.github.io/ website). Percent neutralization was calculated for each serum dilution relative to the positive control wells. The IC50 (inhibitory concentration 50) titer was defined as the titer at which ≥50% neutralization was observed.
Statistical Analysis
Demographic characteristics were described as categorical or continuous variables (number, percent; median, range). The Mann-Whitney U test, Kruskal-Wallis test, or Wilcoxon signed rank test was used to compare two or more groups, accepting significance at p < 0.05. Linear regression was utilized to calculate the correlation coefficient between continuous variables. All analyses were conducted using Prism 9.0 (GraphPad, San Diego CA).
Results
Study Population Demographics
In this cohort of 92 mother-infant dyads (Table 1), 61 women (66%) delivered FT infants (range, 37–40 weeks), 24 (26%) delivered LPT infants (range, 34–36 weeks), and 7 (8%) delivered PT infants (range, 27–33 weeks). The mothers had a median age of 27 years (range, 18–38 years), 55 (60%) were African American, 6 (7%) were Hispanic, 56 (61%) were multiparous, and 71 (77%) had public insurance. There were no significant differences in maternal demographics by GA group. Among the infants, 46 (50%) were male, 67 (73%) were African American, and 6 (7%) were Hispanic.
Anti-gB Titers Are Higher Than Anti-gM/gN Titers
For all mothers and infants, anti-gB titers were significantly higher than anti-gM/gN titers (Figures 1A,B, p < 0.001) at all gestational ages (PT, LPT, FT). Anti-gM/gN titers were significantly lower among PT and LPT infants compared to their mothers, but were similar for FT infants and mothers (Figure 1C). Anti-gB titers were significantly lower among PT infants compared to their mothers, but were similar between mother-infant dyads for the LPT and FT groups (Figure 1D). Comparing anti-gM/gN titers among infant groups (Figure 1C), PT infants had significantly lower titers than LPT and FT infants, but the latter two groups were similar. Similar results were observed for anti-gB titers (Figure 1D), with those of PT infants significantly lower than LPT and FT groups. Antibody titer ranges and statistical comparisons are summarized in Table 2. To determine the efficiency of transplacental transmission by gestational age, a transmission ratio was calculated for anti-gM/gN and anti-gB antibodies (Figure 1E).
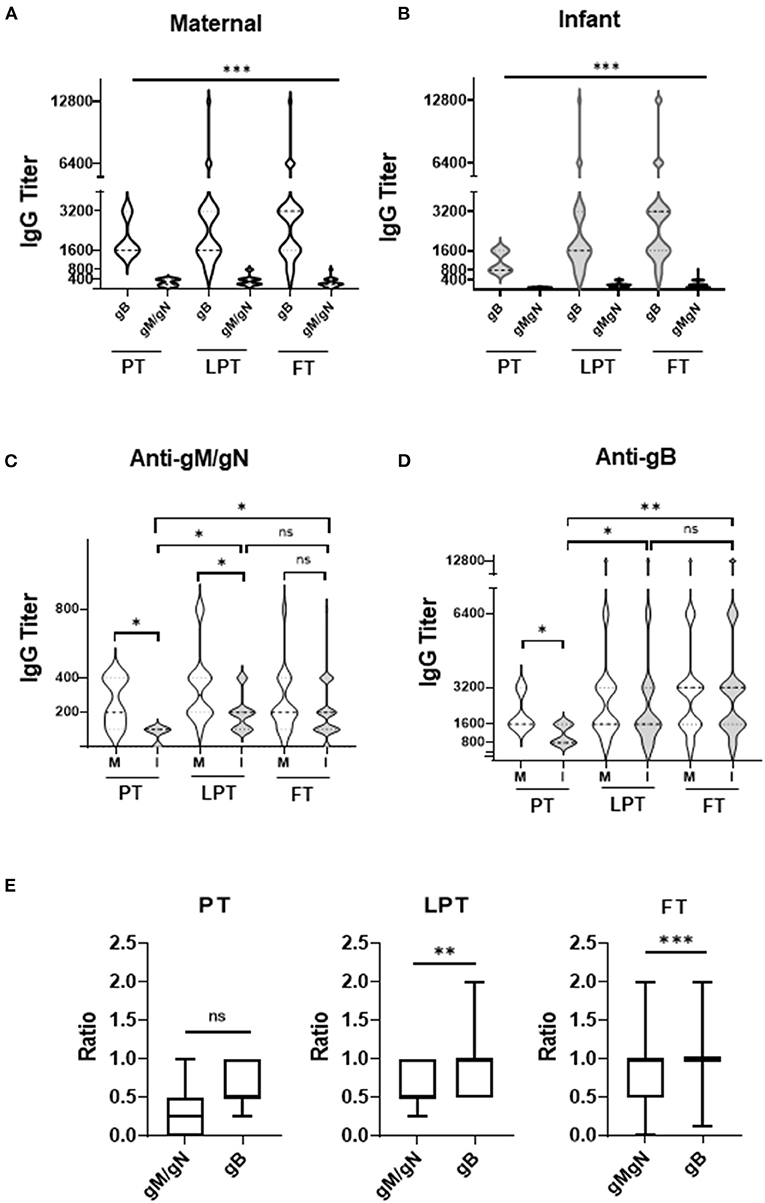
Figure 1. Anti-gM/gN and anti-gB antibody titers among mother-infant dyads. (A,B) CMV anti-gM/gN antibodies and anti-gB antibodies were quantitated by ELISA for mothers (A), and infants (B). (C,D) Antibody titers against gM/gN (C) or gB (D) were compared for preterm (PT), late-preterm (LPT), and full-term (FT) mother (M) and infant (I) groups. (E) Transplacental transmission ratio between maternal 3rd trimester and infant cord blood of anti-gB and anti-gM/gN antibody titers were calculated for all gestational ages (PT, LPT, FT). Data is shown as the median value with min-max ranges plotted. ns, not significant; *p < 0.05; **p < 0.01; ***p < 0.001.
PT infants had similar transplacental transmission ratios for anti-gM/gN and anti-gB antibodies, whereas LPT and FT infants had significantly lower ratio for anti-gM/gN antibodies compared to anti-gB antibodies. These results indicate that anti-gM/gN antibody titers are significantly lower than anti-gB titers among both mothers and infants independently of gestational age, but glycoprotein-specific antibodies are lower among PT infants than LPT and FT infants. Transplacental transmission of anti-gM/gN antibodies is lower than anti-gB antibodies among LPT and FT infants, possible related to the overall higher maternal anti-gB titers.
Neutralization Assays
The neutralizing capacity of maternal and infant sera was tested in serial dilution, with and without complement (+/- complement), and neutralizing titers compared for mother-infant dyads (Figure 2). Complement was included to identify any enhancement of neutralizing capacity conferred by complement-fixing antibodies as compared with complement non-fixing antibodies, which has been described for both anti-gB and anti-gM/gN antibodies (26, 29, 32). Comparison of neutralizing titers with anti-gB or anti-gM/gN titers among mothers (Figures 2A,C) or infants (Figures 2B,D) showed modest correlation of complement-fixing neutralizing titers with anti-gB titers for mothers (R = 0.0163) and infants (R = 0.0453), but no correlation with anti-gM/gN titers. Together, these data show that anti-gM/gN titers were low for mothers and infants, and that serum neutralizing capacity did not correlate with anti-gM/gN titers.
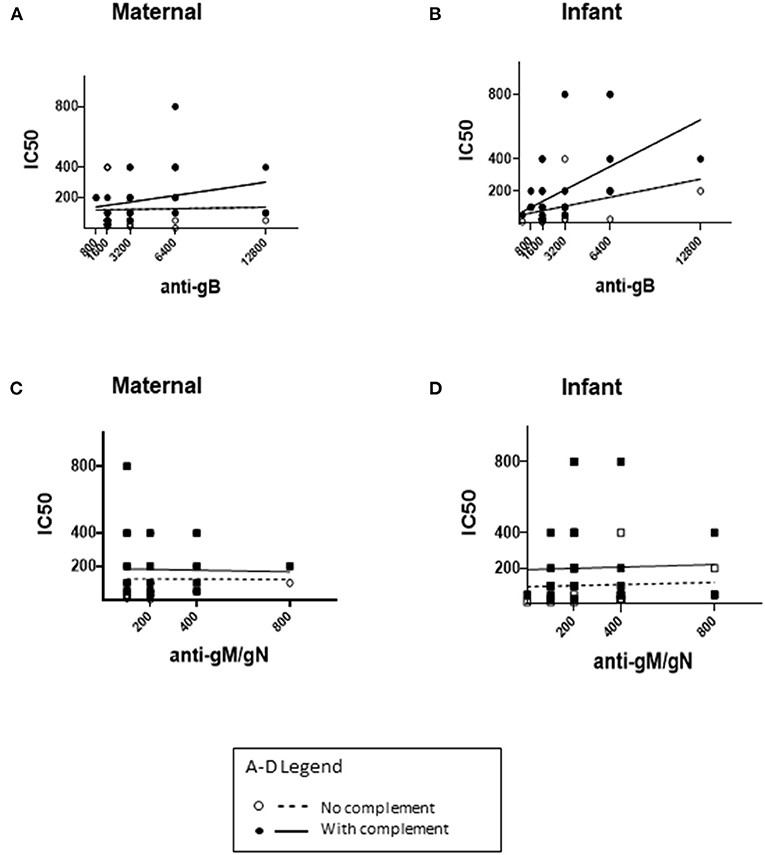
Figure 2. Neutralizing titers of maternal and infant sera. CMV neutralizing capacity of maternal third trimester sera and infant cord sera were tested using an in-vitro neutralizing assay, without or with guinea pig complement, and the IC50 titer was calculated for each sample. The IC50 titers without complement (dotted lines) and with complement (solid lines) were compared to anti-gB and anti-gM/gN titers in maternal (A,C) and infant (B,D) sera. (A,B) The x-axis range reflects anti-gB titers ranging from 1:200-1:12800. (C,D) The x-axis range reflects anti-gM/gN titers ranging from 0–1:800 (A–D). For each correlation plot, a single circle/square may represent more than one sample with the antibody titer/neutralizing IC50 titer shown in each graph.
Discussion
Congenital CMV (cCMV) infection can occur during nonprimary infection among mothers with preconceptional seroimmunity. A maternal vaccine against gB has been evaluated among seronegative women of childbearing age, but it has not been tested among the larger population of seropositive women who are also at risk to transmit CMV during nonprimary infection or viral reactivation. Antibodies against gB are present at high quantities among seropositive individuals and are associated with serum neutralizing capacity, suggesting that increasing anti-gB antibodies by vaccination may provide only incremental improvement in protection among seropositive women (33, 34). Our results confirm that anti-gB titers correlate to some extent with serum neutralizing capacity among mothers and their infants.
In contrast, anti-gM/gN antibody titers were lower than anti-gB titers among pregnant women and their infants independent of gestational age and did not correlate with serum neutralizing capacity. Transplacental transmission of anti-gM/gN antibodies to late preterm and full term infants was significantly lower than that of anti-gB antibodies. Human anti-gM/gN antibodies have been shown to neutralize CMV infection in-vitro and in a murine model but, similar to our findings, a recent study of non-pregnant CMV seropositive adults showed that anti-gM/gN titers did not correlate with in-vitro serum neutralizing activity (25, 26, 28). The reasons for the discrepancy between in-vitro and animal model data compared to clinical serologic responses are unclear, and it is unknown whether increasing human anti-gM/gN titers could improve serum CMV-neutralizing potential. As anti-gM/gN antibodies can be elicited in animal models after DNA immunization, it remains a possibility that vaccination could be used to enhance anti-gM/gN titers among human populations, including those with natural seroimmunity to HCMV infection, although the resulting impact upon serum neutralizing capacity is not yet defined.
A limitation of this study is that only one virus strain, AD169, was utilized for all assays. AD169 encodes gN genotype 1, so gN antibodies specific for genotypes 2–4 may not be detected in our ELISA assay. However, 70% of seropositive adults have anti-gN antibodies that react with epitopes present in all gN genotypes, whereas 30% of adults have genotype-specific anti-gN antibodies (20, 35). Our assay would detect the 70% of individuals with anti-gN antibodies common to all genotypes, as well as those with gN-1 specific antibodies, thus capturing the majority of anti-gN serologic responses in our cohort. Future studies could better define individual differences in gN genotype-specific titers in order to determine the potential utility of including all 4 gN genotypes in a gM/gN vaccine.
As this cohort was derived from a biorepository, the patients were not selected to represent the demographic of the general U.S. population. Previous research has demonstrated socioeconomic and racial/ethnic disparities in CMV seropositivity with 81.7% for Hispanics, 75.8% for African Americans, and 51.2% for Non-Hispanic whites in the United States (36–38). Similarly, cCMV rates are higher for nonwhites than whites and individuals with lower socioeconomic (SES) backgrounds compared with those of higher SES (39, 40). Our cohort of seropositive women were predominantly African American and non-Hispanic, with 77% on public insurance. These results align with the known SES and racial disparity in CMV seroprevalence in the US. However, the demographics of this cohort differs from the majority of the U.S. population, which could confer an unknown bias in our results and limit the generalizability of our findings to other demographic populations.
Finally, in this cohort, anti-CMV IgM was not detected among any of the cord blood samples (data not shown), indicating that no infants had congenital CMV infection. Congenital CMV transmission is reported among 0.5% of U.S. populations (41), so we would expect transmission to <1 infant (0.46) among 92 mothers. It is therefore not surprising that congenital CMV was not present in this cohort. However, consequently, no conclusions can be drawn regarding the protective benefit of anti-gB and ant-gM/gN antibodies against congenital CMV transmission among this study cohort.
In summary, among CMV seropositive pregnant women and their newborn infants, anti-gM/gN titers are lower than anti-gB titers and do not correlate with serum neutralizing capacity. Further study is needed to determine if higher human anti-gM/gN antibody titers may confer improved serum neutralizing capacity against CMV infection.
Data Availability Statement
The raw data supporting the conclusions of this article will be made available by the authors, without undue reservation.
Ethics Statement
The studies involving human participants were reviewed and approved by IRB-approved protocol (NCH IRB10-00035). Written informed consent to participate in this study was provided by the participants' legal guardian/next of kin.
Author Contributions
MT-B and MS designed the study. MT-B, KF, BG, RD, IK, and MS collected, processed, and analyzed the data. MT-B, AM, PS, and MS had major contributions to writing the manuscript. All authors participated in the revision of the manuscript.
Funding
This study was funded by the National CMV Foundation (MTB) and the Abigail Wexner Research Institute at Nationwide Children's Hospital (MS and PS). The Ohio Pediatric Research Network Pediatric Research Repository (OPRN PRR) was funded by the Abigail Wexner Research Institute at Nationwide Children's Hospital.
Conflict of Interest
The authors declare that the research was conducted in the absence of any commercial or financial relationships that could be construed as a potential conflict of interest.
Publisher's Note
All claims expressed in this article are solely those of the authors and do not necessarily represent those of their affiliated organizations, or those of the publisher, the editors and the reviewers. Any product that may be evaluated in this article, or claim that may be made by its manufacturer, is not guaranteed or endorsed by the publisher.
Acknowledgments
We would like to thank members of the OPRN Pediatric Research Repository, Rina Li, Hibo Abdi, Dr. Mark Klebanoff and Dr. Carl Backes who assisted with data procurement from the repository, and the mothers and babies who participated in the OPRN PRR.
References
1. Dollard SC, Grosse SD, Ross DS. New estimates of the prevalence of neurological and sensory sequelae and mortality associated with congenital cytomegalovirus infection. Rev Med Virol. (2007) 17:355–63. doi: 10.1002/rmv.544
2. Boppana SB, Ross SA, Novak Z, Shimamura M, Tolan RW Jr, Palmer AL, et al. Hearing multicenter screening, dried blood spot real-time polymerase chain reaction assays to screen newborns for congenital cytomegalovirus infection. JAMA. (2010) 303:1375–82. doi: 10.1001/jama.2010.423
3. Dahle AJ, Fowler KB, Wright JD, Boppana SB, Britt WJ, Pass RF. Longitudinal investigation of hearing disorders in children with congenital cytomegalovirus. J Am Acad Audiol. (2000) 11:283–90. doi: 10.1055/s-0042-1748054
4. Manicklal S, Emery VC, Lazzarotto T, Boppana SB, Gupta RK. The “silent” global burden of congenital cytomegalovirus. Clin Microbiol Rev. (2013) 26:86–102. doi: 10.1128/CMR.00062-12
5. Retzler J, Hex N, Bartlett C, Webb A, Wood S, Star C, et al. Economic cost of congenital CMV in the UK. Arch Dis Child. (2019) 104:559–63. doi: 10.1136/archdischild-2018-316010
6. Stagno S, Pass RF, Dworsky ME, Henderson RE, Moore EG, Walton PD, et al. Congenital cytomegalovirus infection: The relative importance of primary and recurrent maternal infection. N Engl J Med. (1982) 306:945–9. doi: 10.1056/NEJM198204223061601
7. Boppana SB, Rivera LB, Fowler KB, Mach M, Britt WJ. Intrauterine transmission of cytomegalovirus to infants of women with preconceptional immunity. N Engl J Med. (2001) 344:1366–71. doi: 10.1056/NEJM200105033441804
8. Leruez-Ville M, Magny JF, Couderc S, Pichon C, Parodi M, Bussieres L, et al. Risk Factors for Congenital Cytomegalovirus Infection Following Primary and Nonprimary Maternal Infection: A Prospective Neonatal Screening Study Using Polymerase Chain Reaction in Saliva. Clin Infect Dis. (2017) 65:398–404. doi: 10.1093/cid/cix337
9. Enders G, Daiminger A, Bader U, Exler S, Enders M. Intrauterine transmission and clinical outcome of 248 pregnancies with primary cytomegalovirus infection in relation to gestational age. J Clin Virol. (2011) 52:244–6. doi: 10.1016/j.jcv.2011.07.005
10. Cui X, Snapper CM. Development of novel vaccines against human cytomegalovirus. Hum Vaccin Immunother. (2019) 15:2673–83. doi: 10.1080/21645515.2019.1593729
11. Pass RF, Zhang C, Evans A, Simpson T, Andrews W, Huang ML, et al. Vaccine prevention of maternal cytomegalovirus infection. N Engl J Med. (2009) 360:1191–9. doi: 10.1056/NEJMoa0804749
12. Cui X, Meza BP, Adler SP, McVoy MA. Cytomegalovirus vaccines fail to induce epithelial entry neutralizing antibodies comparable to natural infection. Vaccine. (2008) 26:5760–6. doi: 10.1016/j.vaccine.2008.07.092
13. Nelson CS, Huffman T, Jenks JA, Cisneros de la Rosa E, Xie G, Vandergrift N, et al. HCMV glycoprotein B subunit vaccine efficacy mediated by nonneutralizing antibody effector functions. Proc Natl Acad Sci U S A. (2018) 115:6267–72. doi: 10.1073/pnas.1800177115
14. Wussow F, Chiuppesi F, Contreras H, Diamond DJ. Neutralization of human cytomegalovirus entry into fibroblasts and epithelial cells. Vaccines. (2017) 5:1–11. doi: 10.3390/vaccines5040039
15. Freed DC, Tang Q, Tang A, Li F, He X, Huang Z, et al. Pentameric complex of viral glycoprotein H is the primary target for potent neutralization by a human cytomegalovirus vaccine. Proc Natl Acad Sci U S A. (2013) 110:E4997–5005. doi: 10.1073/pnas.1316517110
16. Li L, Freed DC, Liu Y, Li F, Barrett DF, Xiong W, et al. A conditionally replication-defective cytomegalovirus vaccine elicits potent and diverse functional monoclonal antibodies in a phase I clinical trial. NPJ Vaccines. (2021) 6:79. doi: 10.1038/s41541-021-00342-3
17. Wussow F, Chiuppesi F, Martinez J, Campo J, Johnson E, Flechsig C, et al. Human cytomegalovirus vaccine based on the envelope gH/gL pentamer complex. PLoS Pathog. (2014) 10:e1004524. doi: 10.1371/journal.ppat.1004524
18. Vanarsdall AL, Chin AL, Liu J, Jardetzky TS, Mudd JO, Orloff SL, et al. HCMV trimer- and pentamer-specific antibodies synergize for virus neutralization but do not correlate with congenital transmission. Proc Natl Acad Sci U S A. (2019) 116:3728–33. doi: 10.1073/pnas.1814835116
19. Mach M, Kropff B, Dal Monte P, Britt W. Complex formation by human cytomegalovirus glycoproteins M (gpUL100) and N (gpUL73) N. J Virol. (2000) 74:11881–92. doi: 10.1128/JVI.74.24.11881-11892.2000
20. Pignatelli S, Dal Monte P, Rossini G, Chou S, Gojobori T, Hanada K, et al. Human cytomegalovirus glycoprotein N (gpUL73-gN) genomic variants: identification of a novel subgroup, geographical distribution and evidence of positive selective pressure. J Gen Virol. (2003) 84:647–55. doi: 10.1099/vir.0.18704-0
21. Kropff B, Burkhardt C, Schott J, Nentwich J, Fisch T, Britt W, et al. Glycoprotein N of human cytomegalovirus protects the virus from neutralizing antibodies. PLoS Pathog. (2012) 8:e1002999. doi: 10.1371/journal.ppat.1002999
22. Varnum SM, Streblow DN, Monroe ME, Smith P, Auberry KJ, Pasa-Tolic L, et al. Identification of proteins in human cytomegalovirus (HCMV) particles: the HCMV proteome. J Virol. (2004) 78:10960–6. doi: 10.1128/JVI.78.20.10960-10966.2004
23. Mach M, Kropff B, Kryzaniak M, Britt W. Complex formation by glycoproteins M and N of human cytomegalovirus: structural and functional aspects. J Virol. (2005) 79:2160–70. doi: 10.1128/JVI.79.4.2160-2170.2005
24. Krzyzaniak M, Mach M, Britt WJ. The cytoplasmic tail of glycoprotein M (gpUL100) expresses trafficking signals required for human cytomegalovirus assembly and replication. J Virol. (2007) 81:10316–28. doi: 10.1128/JVI.00375-07
25. Shibamura M, Yoshikawa T, Yamada S, Inagaki T, Nguyen PHA, Fujii H, et al. Association of human cytomegalovirus (HCMV) neutralizing antibodies with antibodies to the HCMV glycoprotein complexes. Virol J. (2020) 17:120. doi: 10.1186/s12985-020-01390-2
26. Shimamura M, Mach M, Britt WJ. Human cytomegalovirus infection elicits a glycoprotein M (gM)/gN-specific virus-neutralizing antibody response. J Virol. (2006) 80:4591–600. doi: 10.1128/JVI.80.9.4591-4600.2006
27. Pati SK, Novak Z, Purser M, Arora N, Mach M, Britt WJ, et al. Strain-specific neutralizing antibody responses against human cytomegalovirus envelope glycoprotein N. Clin Vaccine Immunol. (2012) 19:909–13. doi: 10.1128/CVI.00092-12
28. Wang H, Yao Y, Huang C, Chen Q, Chen J, Chen Z. Immunization with cytomegalovirus envelope glycoprotein M and glycoprotein N DNA vaccines can provide mice with complete protection against a lethal murine cytomegalovirus challenge. Virol Sin. (2013) 28:174–82. doi: 10.1007/s12250-013-3330-9
29. Li F, Freed DC, Tang A, Rustandi RR, Troutman MC, Espeseth AS, et al. Complement enhances in vitro neutralizing potency of antibodies to human cytomegalovirus glycoprotein (gB) B, and immune sera induced by gB/MF59 vaccination. NPJ Vaccines. (2017) 2:36. doi: 10.1038/s41541-017-0038-0
30. Britt WJ. Human cytomegalovirus: propagation, quantification, and storage. Curr Protoc Microbiol Chapter. (2010) 14:Unit 14E3. 10.100219780471729259.mc14e03s18
31. Spiller OB, Hanna SM, Devine DV, Tufaro F. Neutralization of cytomegalovirus virions: the role of complement. J Infect Dis. (1997) 176:339–47. doi: 10.1086/514050
32. Vanarsdall AL, Johnson DC. Human cytomegalovirus entry into cells. Curr Opin Virol. (2012) 2:37–42. doi: 10.1016/j.coviro.2012.01.001
33. Britt WJ, Vugler L, Butfiloski EJ, Stephens EB. Cell surface expression of human cytomegalovirus (HCMV) gp55-116 (gB): use of HCMV-recombinant vaccinia virus-infected cells in analysis of the human neutralizing antibody response. J Virol. (1990) 64:1079. doi: 10.1128/jvi.64.3.1079-1085.1990
34. Britt WJ. Maternal immunity and the natural history of congenital human cytomegalovirus infection. Viruses. (2018) 10:1–18. doi: 10.3390/v10080405
35. Burkhardt C, Himmelein S, Britt W, Winkler T, Mach M. Glycoprotein N subtypes of human cytomegalovirus induce a strain-specific antibody response during natural infection. J Gen Virol. (2009) 90:1951–61. doi: 10.1099/vir.0.010967-0
36. Staras SA, Dollard SC, Radford KW, Flanders WD, Pass RF, Cannon MJ. Seroprevalence of cytomegalovirus infection in the United States, 1988-1994. Clin Infect Dis. (2006) 43:1143–51. doi: 10.1086/508173
37. Dowd JB, Aiello AE, Alley DE. Socioeconomic disparities in the seroprevalence of cytomegalovirus infection in the US population: NHANES III. Epidemiol Infect. (2009) 137:58–65. doi: 10.1017/S0950268808000551
38. Cannon MJ, Schmid DS, Hyde TB. Review of cytomegalovirus seroprevalence and demographic characteristics associated with infection. Rev Med Virol. (2010) 20:202–13. doi: 10.1002/rmv.655
39. Fowler KB, Ross SA, Shimamura M, Ahmed A, Palmer AL, Michaels MG, et al. Racial and ethnic differences in the prevalence of congenital cytomegalovirus infection. J Pediatr. (2018) 200:196–201.e1. doi: 10.1016/j.jpeds.2018.04.043
40. Kenneson A, Cannon MJ. Review and meta-analysis of the epidemiology of congenital cytomegalovirus (CMV) infection. Rev Med Virol. (2007) 17:253–76. doi: 10.1002/rmv.535
Keywords: congenital cytomegalovirus infection, prematurity, glycoprotein antibodies, seroimmunity, vaccines
Citation: Talavera-Barber M, Flint K, Graber B, Dhital R, Kaptsan I, Medoro AK, Sánchez PJ and Shimamura M (2022) Antibody Titers Against Human Cytomegalovirus gM/gN and gB Among Pregnant Women and Their Infants. Front. Pediatr. 10:846254. doi: 10.3389/fped.2022.846254
Received: 30 December 2021; Accepted: 12 May 2022;
Published: 23 June 2022.
Edited by:
Marisa Marcia Mussi-Pinhata, University of São Paulo, BrazilReviewed by:
Alison J. Carey, Drexel University, United StatesRita Carsetti, Bambino Gesù Children's Hospital (IRCCS), Italy
Copyright © 2022 Talavera-Barber, Flint, Graber, Dhital, Kaptsan, Medoro, Sánchez and Shimamura. This is an open-access article distributed under the terms of the Creative Commons Attribution License (CC BY). The use, distribution or reproduction in other forums is permitted, provided the original author(s) and the copyright owner(s) are credited and that the original publication in this journal is cited, in accordance with accepted academic practice. No use, distribution or reproduction is permitted which does not comply with these terms.
*Correspondence: Maria Talavera-Barber, bWFyaWEuYmFyYmVyQGF2ZXJhLm9yZw==