- 1Department of Pharmacy, National Children's Medical Center, Children's Hospital of Fudan University, Shanghai, China
- 2Department of Respiratory Disease, National Children's Medical Center, Children's Hospital of Fudan University, Shanghai, China
Background: The purpose of this study was to evaluate the efficacy and safety of BAL in treating MPP.
Methods: From January 2013 to January 2019, 1,689 pediatric patients with MPP were analyzed retrospectively. Patients were subdivided into BAL group and non-BAL group according to whether they received BAL treatment within seven days after admission. The propensity score matching method matched patients' baseline characteristics (1:1). The primary outcomes were hospital stays and the cure rate. Secondary outcomes included mortality, co-infection, repeat hospitalization within 30 days, and total cost of treatment.
Results: After matching, 524 patients (BAL: 262; control: 262) were recorded. The BAL group had significantly shorter hospital stays (OR: 0.5, 95% CI: 0.4–0.7). Meanwhile, BAL did not significantly modify the cost, co-infection rate, and mortality. In subgroup analyses, the group with BAL intervention within three days had a significantly shorter hospital stay (OR: 0.4, 95% CI: 0.3–0.5) compared with the group with BAL intervention three days after admission.
Conclusions: Early BAL intervention is a better treatment than conventional drug therapy alone, and no significant complications were seen in this study. BAL intervention has an excellent clinical benefit. The earlier the intervention, the better the effect.
Introduction
Pneumonia is the third leading cause of death worldwide (1). More than 40% of community-acquired pneumonia (CAP) cases are due to Mycoplasma pneumoniae pneumonia (MPP), and 18% of children with MPP require hospitalization (2, 3). In the United States, one-quarter of hospitalized children with pneumonia older than five years are MPP infections (4). Mycoplasma has no cell wall, and Macrolide drugs, rather than penicillin or cephalosporin, are the preferred antidote (5, 6). Resistance to Macrolide drugs has been increasing worldwide, particularly in Asia, which amounts to 90%–100% (7, 8). Refractory MPP (RMPP) is a condition that does not improve after seven days of standard Macrolide therapy (9). In recent years, the increasing proportion of RMPP has posed a significant challenge to paediatricians (10). A South Korean study of 5,294 children with pneumonia showed RMPP rates of more than 20% (11). Patients with RMPP must be treated with tetracycline or fluoroquinolones (12, 13). Tigecycline can also be added when patients do not respond to fluoroquinolones (14). However, such multi-drug therapies may disrupt the gut microbial balance (15) and increase the risk of adverse drug reactions (16). In particular, these new drugs may be traced to life-long sequelae in children, including tetracycline teeth, cartilage dysplasia, etc (17).
Bronchoalveolar lavage (BAL) can shorten the course of pneumonia and improve the prognosis in neonates with severe pneumonia (18, 19). In combination with medication, BAL gets a better treatment than medication alone in children with RMPP (20). Clinical studies have shown that BAL can improve the recovery of lung injury in MPP patients with multipolar consolidation (21). The improvement of BAL in the initial stage of MPP is still unknown. This study was designed to evaluate BAL's efficacy in treating children with MPP from 2013 to 2019 at the National Children's Medical Center, in Shanghai, China.
Methods
Patients source
All the children in this cohort were infected with Mycoplasma pneumoniae pneumonia. From January 2013 to January 2019, the patients were admitted to a tertiary hospital with 1,689 pediatric beds in Shanghai, China. The IgM of mycoplasma pneumonia was more than 1: 160 in all pediatric patients. Or all were positive for mycoplasma by throat swab rapid culture results/DNA assay. With the spread of BAL technology, the number of patients admitted to the hospital between January 2013 and January 2019 who received early BAL active intervention has been increasing year by year, thus forming a treatment group. Some patients did not receive BAL active therapy and still received conventional drug therapy, so they created a control group. The exclusion criteria included: (1) severe pneumonia in patients with viral, bacterial, chlamydial, and other pathogens; (2) the patient had a history of chronic respiratory diseases such as asthma bronchitis, obstructive bronchitis, (3) congenital diseases such as immune system defect and severe hypofunction of heart, (4) a history of allergy to Macrolide drugs. The study protocol was subject to approval by the Institutional Review Board of the Pediatric Hospital affiliated with Fudan University (FELS2014–084). Due to the observational nature of the retrospective study, written informed consent was waived.
Treatment methods and evaluation
In this study, the patient's baseline characteristics, treatment implementation and clinical outcomes were collected by reviewing medical records. All the children received standard 7-day Macrolide treatment after admission: Azithromycin injection (Pfizer), IV, at a dose of 10 mg/kg d, once daily. The course of treatment is not greater than seven days.
In the BAL group, the parents of the children needed to consent to bronchoalveolar lavage, and the family members of the children signed the informed consent form. The contraindications of BAL in children included: (1) severe hypofunction of heart and lung; (2) severe arrhythmia: atrial, ventricular fibrillation and flutter, atrioventricular block; (3) high fever, (4) severe arrhythmia Severe hemorrhagic disease, Coagulopathy, severe pulmonary hypertension and possible massive hemoptysis; (5) severe malnutrition. The procedure of BAL was as follows: routine preoperative preparation, monitoring of vital signs, oxygen inhalation by face mask, local anaesthesia with 2% lidocaine, feeding fiberoptic bronchoscope from nasal cavity; Combined with preoperative imaging examination, the location of the lesion was selected, the distal end of the bronchoscope was fixed, and 37°C, 0.9% sodium chloride solution was injected into the Bronchus for bronchoalveolar lavage. 5–10 ml of lavage fluid was aspirated at negative pressure and repeated 2–4 times.
All the patients were assessed on discharge and were classified into four grades: cured, effective, ineffective, and dead, according to clinical manifestation, laboratory and imaging examination. Total recovery: the child's body temperature returned to normal, with no noticeable cough, shortness of breath or other clinical symptoms; inflammatory indicators were usual, and imaging examination suggested that the absorption of inflammatory shadow; effective: the child's body temperature is average, respiratory symptoms better than before; ineffective: the results showed that the clinical manifestation, laboratory examination and imaging examination of the patients were not improved or even worsened. The cure rate = [(number of cured cases + number of effective cases)/total number of cases] × 100%.
Statistical approach
We used the statistical package STATA15.0 as the statistical analysis software. Continuous variables between the two groups were expressed as mean ± standard deviation. Category variables are reported in percentages. Comparisons were made concerning constant variables using the Mann-Whitney U or Student's t-test and category variables using the chi-square test. P < 0.05 indicated that the difference was statistically significant. The Kaplan-Meier method was used to evaluate the total length of hospital stay in both groups, and odds ratios (OR) and 95% confidence intervals (CI) were calculated.
Two groups of subjects were matched 1:1 nearest neighbour using propensity score matching (PSM), with the calliper value set to 0.2. The variables for generating propensity score included age, sex, race, Comorbidities, d-dimer, and CRP level. The standard difference was utilized to evaluate the balance between the two groups. The difference of < 0.1 indicated that the balance of the variables between the two groups was better. In addition, subgroup analysis was performed to analyze the outcomes of BAL patients at different times of the intervention.
We chose six variables for PSM matching for three reasons. First, a previous study of the same type screened for five parameters, including age, sex, race, c-reactive protein, and comorbidities (22). Second, a previous study showed a gender difference in treatment outcomes for pneumonia (23). Meanwhile, the immunopathogenesis of MPP is regarded as age-dependent (24). Age may be the sole predisposing factor of MPP (25). Third, elevated D-dimer levels can be used as a predictor of RMPP and complications (26, 27).
Results
There were 262 cases in the BAL group and 989 cases in the control group. After 1∶1 matching, 262 patients were included in the analysis (Figure 1). The baseline characteristics of pre-matched patients are shown in Table 1. There were significant differences between the two groups in CRP, pneumonia complications (alveolar fluid), and race type (p < 0.05). These significant differences may be due to a lower rate of pneumonia complications in the control group than in the BAL group (9.7% vs. 22.2%). After PSM, there were no statistically significant differences in all demographic information between the two groups (p > 0.05).
Clinical outcome results are shown (Table 2): among the 262 patients, the mean length of hospital stay was 5.3 days in the BAL group and 7.4 days in the control group. As shown in Figure 2A, using BAL reduced the size of hospital stay by 2.1 days compared with the control group (p < 0.001). And there was a significant difference in hospital length of stay between the two groups before and after matching. In the matched cohort, the considerable difference in the incidence of concurrent infection between the BAL group and the control group disappeared after seven days of treatment. There were no significant differences between the two groups in the rate of repeated hospitalization within 30 days, the rate of death, and the rate of complicated infection. In addition, we performed a subgroup analysis of the BAL group (Figure 2B). Subgroup analysis showed that BAL intervention within three days of hospitalization significantly decreased the time to final discharge compared with the group after three days (p < 0.001). Notably, we significantly reduced RMPP conversion rates in all BAL groups before and after matching (p < 0.001). Compared with the control group, the BAL group had significantly shorter hospital stays (OR: 0.5,95% CI: 0.4–0.7). Meanwhile, BAL did not substantially modify the total cost, co-infection rate, and mortality. In subgroup analyses, the group with BAL intervention within three days had a significantly shorter hospital stay (OR: 0.4,95% CI: 0.3–0.5) compared with the group with BAL intervention three days after admission.
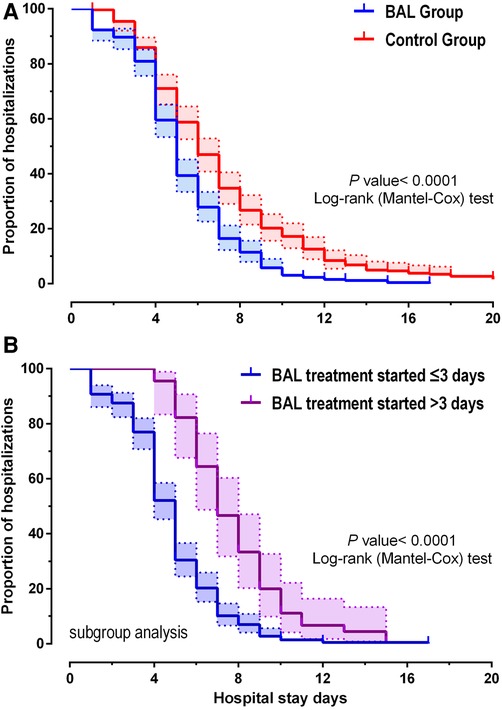
Figure 2. Kaplan-Meier plotter showing the discharge time for MMP patients (A). (B) shows the difference in length of stay between days 3 and 3 when the lavage was performed.
Discussion
BAL can improve pulmonary imaging of RMPP patients and shorten hospitalization time (20, 28). However, some clinical studies have shown that BAL can significantly prolong the hospital stay and increase the total cost (21). Why the contradictory results? We think that the key factor is the timing of BAL initiation, a difference that has not been strictly distinguished in previous studies. Usually, the paediatrician must confirm the diagnosis of RMPP after seven days of ineffective Azithromycin therapy before starting BAL (29).
In contrast, our study takes the lead in adopting BAL in patients with early MPP rather than RMPP. The results showed that BAL intervention within seven days considerably shortened hospital stay, regardless of whether they were later RMPP patients. Moreover, subgroup analyses showed a better treatment benefit when BAL was performed within three days of admission than after three days (Figure 2B). All these suggest that the earlier BAL is used, the better clinical results will be achieved.
It is worth pointing out that BAL can also reduce the incidence of RMPP (MPP to RMPP conversion rate) by 5.5 times (3.1% vs. 17.2%, p < 0.001). We were amazed by this dramatic improvement, and we speculate that there may be three reasons. First, BAL could decrease the abundance of MP in the lung. MP can cause epithelial cell damage and reduce the clearance of mucociliary cells (30). The degree of epithelial injury directly resulted from MPP's severity (31). The abundance of MP in the lung was positively correlated with the length of hospital stay, peak fever and serum c-reactive protein level (32, 33). The high load of MP in the lungs of pediatric patients was significantly correlated with the severity of pneumonia (34, 35). Second, BAL can clear away mucus plugs, improve child ventilation, and promote the rapid absorption of imaging large patchy shadows. Studies have shown that 75% of children with RMPP have mucus plug formation (36), and children with mucus plug have longer radiology clearance, signing up to 8 weeks (22). Third, BAL can eliminate cytokines, lipids and other inflammatory substances. For example, the up-regulation of multiple cytokines in bronchoalveolar lavage fluid exacerbates disease severity (37, 38). Increased glycerophospholipids, sphingolipids, and fatty acyl groups in the lungs lead to alveolar and endothelial cell death (39, 40).
While we support the positive efficacy of early BAL interventions, we are also concerned about the increased cost and rate of co-infection associated with BAL. Fortunately, we found no significant change in total hospital costs and co-infection rate in the BAL group. There are two possible causes for this. First, BAL in Shanghai costs about $20 per session. In contrast, the same single operation in the United States costs at least $400 (41). Second, patients with RMPP usually need multiple BAL treatments, which may prolong the hospital stay and increase the cost of treatment. On the contrary, our study shows that if BAL intervention is performed in advance, most patients need only one lavage. Therefore, we conclude that BAL's timely intervention strategies are cost-effective in reducing hospital length of stay without increasing total costs.
There are certain inadequacies in this study. Despite the use of the PSM method in this trial, some other probable factors are not considered, which may be biased. As a single-centre study, the small sample size has some limitations. Therefore, a larger scale of multicenter controlled trials is needed to confirm the BAL effect in the future.
Conclusion
Timely BAL treatment can postpone the progression of MPP and provide a larger therapeutic time window for treating MPP in children. BAL within seven days after hospitalization can shorten the healing time of MPP children and promote their recovery and early discharge. BAL intervention therapy had better efficacy, similar safety, and better clinical benefit. Therefore, early BAL can not only remove inflammatory substances but also reduce the abundance of mycoplasma in the lung and promote the recovery of pediatric patients.
Data availability statement
The raw data supporting the conclusions of this article will be made available by the authors, without undue reservation.
Ethics statement
The study protocol was subject to approval by the Institutional Review Board of the Pediatric Hospital affiliated with Fudan University (FELS2014-084). Due to the observational nature of the retrospective study, written informed consent was waived.
Author contributions
JML: evidence collection, manuscript preparation, and writing the manuscript's central part and manuscript revision. JQZ; GFW: evidence collection, manuscript editing and revising. ZPL; XBZ: ideas, reviewed the manuscript. All authors contributed to the article and approved the submitted version.
Funding
The presented work was financially supported by the China-WHO Biennial Collaborative Projects 2016–2017 (No. WPCHN1611348). National Natural Science Foundation (No. 81874325).
Conflict of interest
The authors declare that the research was conducted in the absence of any commercial or financial relationships that could be construed as a potential conflict of interest.
Publisher's note
All claims expressed in this article are solely those of the authors and do not necessarily represent those of their affiliated organizations, or those of the publisher, the editors and the reviewers. Any product that may be evaluated in this article, or claim that may be made by its manufacturer, is not guaranteed or endorsed by the publisher.
References
1. Chou CC, Shen CF, Chen SJ, Chen HM, Wang YC, Chang WS, et al. Recommendations and guidelines for the treatment of pneumonia in Taiwan. Journal of microbiology. Immunol and Infect. (2019) 52(1):172–99. doi: 10.1016/j.jmii.2018.11.004
2. Ferwerda A, Moll HA, de Groot R. Respiratory tract infections by Mycoplasma pneumoniae in children: a review of diagnostic and therapeutic measures. Eur J Pediatr. (2001) 160(8):483–91. doi: 10.1007/s004310100775
3. Metlay JP, Waterer GW, Long AC, Anzueto A, Brozek J, Crothers K, et al. Diagnosis and treatment of adults with community-acquired pneumonia. Am J Respir Crit Care Med. (2019) 200(7):E45–67. doi: 10.1164/rccm.201908-1581ST
4. Kutty PK, Jain S, Taylor TH, Bramley AM, Diaz MH, Ampofo K, et al. Mycoplasma pneumoniae among children hospitalized with community-acquired pneumonia. Clin Infect Dis. (2019) 68(1):5–12. doi: 10.1093/cid/ciy419
5. Qiu J-L, Huang L, Shao M-Y, Chai Y-N, Zhang H-J, Li X-F, et al. Efficacy and safety of azithromycin combined with glucocorticoid on refractory Mycoplasma pneumoniae pneumonia in children: a PRISMA-compliant systematic review and meta-analysis. Med (Baltimore). (2020) 99(22):e20121. doi: 10.1097/MD.0000000000020121
6. Lee H, Yun KW, Lee HJ, Choi EH. Antimicrobial therapy of macrolide-resistant Mycoplasma pneumoniae pneumonia in children. Expert Rev Anti-Infect Ther. (2018) 16(1):23–34. doi: 10.1080/14787210.2018.1414599
7. Cao B, Zhao CJ, Yin YD, Zhao F, Song SF, Bai L, et al. High prevalence of macrolide resistance in Mycoplasma pneumoniae isolates from adult and adolescent patients with respiratory tract infection in China. Clin Infect Dis. (2010) 51(2):189–94. doi: 10.1086/653535
8. Zhao F, Liu J, Xiao D, Liu L, Gong J, Xu J, et al. Pathogenic analysis of the bronchoalveolar lavage fluid samples with pediatric refractory Mycoplasma pneumoniae pneumonia. Front Cell Infect Microbiol. (2020) 10:553739. doi: 10.3389/fcimb.2020.553739
9. Tamura A, Matsubara K, Tanaka T, Nigami H, Yura K, Fukaya T. Methylprednisolone pulse therapy for refractory Mycoplasma pneumoniae pneumonia in children. Journal of Infect. (2008) 57(3):223–8. doi: 10.1016/j.jinf.2008.06.012
10. Tong L, Huang S, Zheng C, Zhang Y, Chen Z. Refractory Mycoplasma pneumoniae pneumonia in children: early recognition and management. J Clin Med. (2022) 11(10):2824. doi: 10.3390/jcm11102824
11. Choi YJ, Chung EH, Lee E, Kim C-H, Lee YJ, Kim H-B, et al. Clinical characteristics of macrolide-refractory Mycoplasma pneumoniae pneumonia in Korean children: a multicenter retrospective study. J Clin Med. (2022) 11(2):306. doi: 10.3390/jcm11020306
12. Hammerschlag MR. Mycoplasma pneumoniae infections. Curr Opin Infect Dis. (2001) 14(2):181–6. doi: 10.1097/00001432-200104000-00012
13. Tsai T-A, Tsai C-K, Kuo K-C, Yu H-R. Rational stepwise approach for Mycoplasma pneumoniae pneumonia in children. Journal of microbiology. Immunol and Infect. (2021) 54(4):557–65. doi: 10.1016/j.jmii.2020.10.002
14. Yuan X, Liu J, Bai C, Niu W, Wang J, Li P, et al. Tigecycline in the treatment of fulminant Mycoplasma pneumoniae pneumonia non-responsive to azithromycin and fluoroquinolone: a case report. Med (Baltimore). (2020) 99(28):e21128. doi: 10.1097/MD.0000000000021128
15. Qin N, Yin G, Chen L, Ping N, Zhao S, Qin H. Piperacillin combined with azithromycin in the treatment of childhood mycoplasma pneumoniae pneumonia and the influence on the intestinal microecology. Farmacia. (2021) 69(2):274–8. doi: 10.31925/farmacia.2021.2.12
16. Eschenauer GA, Xiao L, Waites KB, Crabb DM, Ratliff AE, Gandhi TN, et al. Macrolide-resistant Mycoplasma pneumoniae pneumonia in transplantation: increasingly typical? Transpl Infect Dis. (2020) 22(5):e13318. doi: 10.1111/tid.13318
17. Yang HJ. Benefits and risks of therapeutic alternatives for macrolide resistant Mycoplasma pneumoniae pneumonia in children [review]. Korean J Pediatr. (2019) 62(6):199–205. doi: 10.3345/kjp.2018.07367
18. Liu J, Zhao HR, Wei HL, Chen C, Qiu RX, Ren XL, et al. Efficacy of bronchoalveolar lavage as adjunct therapy in the treatment of neonatal severe pneumonia: a prospective case–control study. J Trop Pediatr. (2020) 66(5):528–33. doi: 10.1093/tropej/fmaa010
19. Liao CC, Tsai MH, Lai SH, Lai MY, Chu SM, Huang HR, et al. Safety and clinical application of nonbronchoscopic bronchoalveolar lavage in preterm neonates with clinical ventilator-associated pneumonia. Pediatr Neonatol. (2021) 62(2):195–201. doi: 10.1016/j.pedneo.2020.11.008
20. Li F, Zhu B, Xie G, Wang Y, Geng J. Effects of bronchoalveolar lavage on pediatric refractory mycoplasma pneumoniae pneumonia complicated with atelectasis: a prospective case-control study. Minerva Pediatr. (2021) 73(4):340–7. doi: 10.23736/S2724-5276.20.05538-3
21. Wang L, Xie Q, Xu S, Li H, Zhang L, Ai J, et al. The role of flexible bronchoscopy in children with Mycoplasma pneumoniae pneumonia. Pediatr Res. (2022):early access. doi: 10.1038/s41390-021-01874-z
22. Huang L, Huang X, Jiang W, Zhang R, Yan Y, Huang L. Independent predictors for longer radiographic resolution in patients with refractory <em>Mycoplasma pneumoniae</em> pneumonia: a prospective cohort study. BMJ Open. (2018) 8(12):e023719. doi: 10.1136/bmjopen-2018-023719
23. Barbagelata E, Cillóniz C, Dominedò C, Torres A, Nicolini A, Solidoro P. Gender differences in community-acquired pneumonia. Minerva Med. (2020) 111(2):153–65. doi: 10.23736/S0026-4806.20.06448-4
24. Kamizono S, Ohya H, Higuchi S, Okazaki N, Narita M. Three familial cases of drug-resistant Mycoplasma pneumoniae infection. Eur J Pediatr. (2010) 169(6):721–6. doi: 10.1007/s00431-009-1100-3
25. Xu D, Li S, Chen Z, Du L. Detection of Mycoplasma pneumoniae in different respiratory specimens. Eur J Pediatr. (2011) 170(7):851–8. doi: 10.1007/s00431-010-1360-y
26. Huang X, Li D, Liu F, Zhao D, Zhu Y, Tang H. Clinical significance of D-dimer levels in refractory Mycoplasma pneumoniae pneumonia. BMC Infect Dis. (2021) 21(1):14. doi: 10.1186/s12879-020-05700-5
27. Zheng Y, Hua L, Zhao Q, Li M, Huang M, Zhou Y, et al. The level of D-dimer is positively correlated with the severity of Mycoplasma pneumoniae pneumonia in children. Front Cell Infect Microbiol. (2021) 11:687391. doi: 10.3389/fcimb.2021.687391
28. Pei M, Jiang P, Wang T, Xia C, Hou R, Sun A, et al. Effect of bronchoalveolar lavage on the clinical efficacy, inflammatory factors, and immune function in the treatment of refractory pneumonia in children. Transl Pediatr. (2021) 10(4):921–8. doi: 10.21037/TP-21-89
29. Esposito S, Bosis S, Faelli N, Begliatti E, Droghetti R, Tremolati E, et al. Role of atypical bacteria and azithromycin therapy for children with recurrent respiratory tract infections. Pediatr Infect Dis J. (2005) 24(5):438–44. doi: 10.1097/01.inf.0000160949.99560.8d
30. Staugas R, Martin AJ. Secondary bacterial infections in children with proved Mycoplasma pneumoniae. Thorax. (1985) 40(7):546–8. doi: 10.1136/thx.40.7.546
31. Jiang W, Qian L, Liang H, Tian M, Liu F, Zhao D. Relationships between the varied ciliated respiratory epithelium abnormalities and severity of Mycoplasma pneumoniae pneumonia. Scand J Infect Dis. (2014) 46(7):486–92. doi: 10.3109/00365548.2014.885658
32. Dai W, Wang H, Zhou Q, Feng X, Lu Z, Li D, et al. The concordance between upper and lower respiratory microbiota in children with Mycoplasma pneumoniae pneumonia article. Emerging Microbes and Infections. (2018) 7(1):1–8. doi: 10.1038/s41426-018-0097-y
33. Chen J, Xi Z, Shi Y, Liu L, Wang L, Qian L, et al. Highly homogeneous microbial communities dominated by Mycoplasma pneumoniae instead of increased resistance to macrolide antibiotics is the characteristic of lower respiratory tract microbiome of children with refractory Mycoplasma pneumoniae pneumonia. Transl Pediatr. (2021) 10(3):604–15. doi: 10.21037/TP-20-404
34. Liu J, Zhao F, Lu J, Xu H, Liu H, Tang X, et al. High Mycoplasma pneumoniae loads and persistent long-term Mycoplasma pneumoniae DNA in lower airway associated with severity of pediatric Mycoplasma pneumoniae pneumonia. BMC Infect Dis. (2019) 19(1):1045. doi: 10.1186/s12879-019-4667-y
35. Nilsson AC, Björkman P, Welinder-Olsson C, Widell A, Persson K. Clinical severity of Mycoplasma pneumoniae (MP) infection is associated with bacterial load in oropharyngeal secretions but not with MP genotype. BMC Infect Dis. (2010) 10(1):1–8. doi: 10.1186/1471-2334-10-39
36. Yan Y, Wei Y, Jiang W, Hao C. The clinical characteristics of corticosteroid-resistant refractory Mycoplasma Pneumoniae pneumonia in children. Sci Rep. (2016) 6(1):39929. doi: 10.1038/srep39929
37. Yang M, Meng F, Gao M, Cheng G, Wang X. Cytokine signatures associate with disease severity in children with Mycoplasma pneumoniae pneumonia. Sci Rep. (2019) 9(1):17853. doi: 10.1038/s41598-019-54313-9
38. Gao M, Wang K, Yang M, Meng F, Lu R, Zhuang H, et al. Transcriptome analysis of bronchoalveolar lavage fluid from children with Mycoplasma pneumoniae pneumonia reveals natural killer and T cell-proliferation responses. Front Immunol. (2018) 9:1403. doi: 10.3389/fimmu.2018.01403
39. Li J, Luu LDW, Wang X, Cui X, Huang X, Fu J, et al. Metabolomic analysis reveals potential biomarkers and the underlying pathogenesis involved in Mycoplasma pneumoniae pneumonia. Emerg Microbes Infect. (2022) 11(1):593–605. doi: 10.1080/22221751.2022.2036582
40. Klement MLR, Öjemyr L, Tagscherer KE, Widmalm G, Wieslander Å. A processive lipid glycosyltransferase in the small human pathogen Mycoplasma pneumoniae: involvement in host immune response. Mol Microbiol. (2007) 65(6):1444–57. doi: 10.1111/j.1365-2958.2007.05865.x
41. Rossoff J, Locke M, Helenowski IB, Batra S, Katz BZ, Hijiya N. Cost analysis of bronchoalveolar lavage and respiratory tract biopsies in the diagnosis and management of suspected invasive fungal infection in children with cancer or who have undergone stem cell transplant article]. Pediatric Blood and Cancer. (2019) 66(5):e27598. doi: 10.1002/pbc.27598
Keywords: mycoplasma, pneumonia, medication, bronchoalveolar lavage, child, infection
Citation: Lu J, Zhang J, Wang G, Zhang X and Li Z (2023) Effects of bronchoalveolar lavage on Mycoplasma Pneumoniae pneumonia: A propensity score matched-cohort study. Front. Pediatr. 10:1066640. doi: 10.3389/fped.2022.1066640
Received: 11 October 2022; Accepted: 12 December 2022;
Published: 4 January 2023.
Edited by:
Guanhua Xue, Children's Hospital of Capital Institute of Pediatrics, ChinaReviewed by:
Yibing Cheng, Children's Hospital Affiliated of Zhengzhou University, ChinaXiaoxing You, University of South China, China
© 2023 Lu, Zhang, Wang, Zhang and Li. This is an open-access article distributed under the terms of the Creative Commons Attribution License (CC BY). The use, distribution or reproduction in other forums is permitted, provided the original author(s) and the copyright owner(s) are credited and that the original publication in this journal is cited, in accordance with accepted academic practice. No use, distribution or reproduction is permitted which does not comply with these terms.
*Correspondence: Xiaobo Zhang zhangxiaobo0307@163.com Zhiping Li zpli@fudan.edu.cn
Specialty Section: This article was submitted to Pediatric Infectious Diseases, a section of the journal Frontiers in Pediatrics