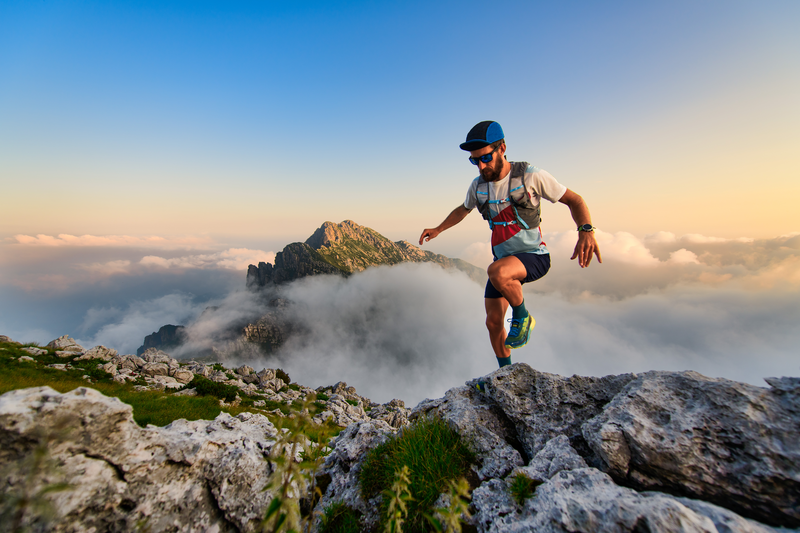
94% of researchers rate our articles as excellent or good
Learn more about the work of our research integrity team to safeguard the quality of each article we publish.
Find out more
MINI REVIEW article
Front. Pediatr. , 31 October 2022
Sec. Pediatric Nephrology
Volume 10 - 2022 | https://doi.org/10.3389/fped.2022.1051481
The kidney plays a fundamental role in acid-base homeostasis by reabsorbing the filtered bicarbonate and by generating new bicarbonate, to replace that consumed in the buffering of non-volatile acids, a process that leads to the acidification of urine and the excretion of ammonium (NH4+). Therefore, urine pH (UpH) and urinary NH4+ (UNH4+) are valuable parameters to assess urinary acidification. The adaptation of automated plasma NH4+ quantification methods to measure UNH4+ has proven to be an accurate and feasible technique, with diverse potential indications in clinical practice. Recently, reference values for spot urine NH4+/creatinine ratio in children have been published. UpH and UNH4+, aside from their classical application in the study of metabolic acidosis, have shown to be useful in the identification of incomplete distal renal tubular acidosis (dRTA), an acidification disorder, without overt metabolic acidosis, extensively described in adults, and barely known in children, in whom it has been found to be associated to hypocitraturia, congenital kidney abnormalities and growth impairment. In addition, a low UNH4+ in chronic kidney disease (CKD) is a risk factor for glomerular filtration decay and mortality in adults, even in the absence of overt metabolic acidosis. We here emphasize on the need of measuring UpH and UNH4+ in pediatric population, establishing reference values, as well as exploring their application in metabolic acidosis, CKD and disorders associated with incomplete dRTA, including growth retardation of unknown cause.
The kidney plays a fundamental role in acid-base homeostasis by regulating plasma bicarbonate (HCO3−) concentration, which constitutes the metabolic component of acid-base balance. This process is made up of two parts: the reabsorption of filtered HCO3− and the generation of new HCO3−, to replace that consumed by endogenous or exogenous acids (1). HCO3− is freely filtered at the glomerulus and then almost completely reabsorbed, making urine virtually free of HCO3− under normal conditions. This reabsorption takes place mostly (approximately 80%) in the proximal tubule, the distal segments of the nephron also playing a significant role in this process (1).
The production of new HCO3− occurs by excreting acids into urine (urinary acidification), since the addition of an alkali and the loss of acid are essentially equivalent in physiologic systems. The capacity of the nephron to excrete acids as free protons (H+) is limited (urinary H+ concentration is <0.1 mmol/L even at a urine pH of 4.5) (1). Instead, acid excretion occurs in the distal nephron by two means: excretion of titratable acids and excretion of ammonium (NH4+), so net acid excretion (NAE) in the urine is calculated as the sum of those components minus urinary HCO3− (which is negligible in fasting normal conditions).
Titratable acids refer to the excretion of H+ bound to urinary buffers (mainly dibasic/monobasic phosphate) (2) and, in normal conditions, it represents one-third to one-half of NAE. The rest of NAE corresponds to NH4+ generation and excretion. NH4+ is synthesized in the proximal tubule by the catabolism of glutamine, generating HCO3− in the process (1). In order to have a net gain of HCO3−, the NH4+ produced in the kidney must be excreted in the urine, allowing for the reabsorption of HCO3− into the bloodstream. If the NH4+ is not excreted, it returns to the liver, where it is metabolized to urea, consuming an equimolar quantity of HCO3− (1, 3). NH4+ excretion has a greater ability to increase under acid load conditions than titratable acid excretion −for example, the amount of phosphate in the urine is not significantly increased in chronic metabolic acidosis, whereas urinary NH4+ (UNH4+) increases several fold− (4) and, therefore, constitutes the most important mechanism of urinary acidification in response to a noxa or stressing condition.
Due to the importance of urinary acidification in maintaining acid-base balance, its evaluation is mandatory in some clinical situations. Although urine pH (UpH) is the simplest, most available parameter to assess urinary acidification, it does not always faithfully reflect NAE, since it merely indicates the maximum concentration of H+ that can be achieved in concrete circumstances. For instance, in cases of chronic metabolic acidosis, when renal ammoniagenesis is increased several fold and H+ ions are buffered by NH3, a relatively high UpH can be observed even when the urinary acidification capacity is preserved (3, 5, 6). Conversely, an appropriately low urine pH (<5.5) can occur when NH4+ excretion is compromised in cases such as hypoaldosteronism (6, 7). For these reasons, in order to perform a correct evaluation of urinary acidification, UpH should be taken in consideration simultaneously with UNH4+.
Nevertheless, due to historical technical difficulties, direct UNH4+ measurement is not usually performed in clinical laboratories as a routine test, and it is estimated by indirect methods, such as the urinary anion gap and the urinary osmolal gap (8, 9), which do not always correlate reliably with UNH4+ (10–15). To solve this problem, automated plasma NH4+ quantification methods, available in most laboratories, have been adapted and evaluated to analyze UNH4+, proving to be an accurate and simple technique for direct UNH4+ measurement (11, 16–18), also avoiding the inaccuracies resulting from urinary anion and osmolal gaps. These methods allow the use of direct UNH4+ quantification in clinical practice as a routine test to assess urinary acidification, reducing the number of tests, analysis time and sample volume, an issue of particular importance in children.
A potentiometric pH meter is the gold standard method for UpH measurement. In clinical practice, dipsticks are often used, which are much more available but less accurate, providing a value only up to the nearest 0.5 unit interval and being prone to perception bias when electronic readers are not used. Although dipsticks are useful in most situations, differences between dipstick and pH-meter readings can be as high as 0.4–0.5 units (19–21), even with electronic readers (22, 23). These differences might be clinically significant and thus lead to wrong clinical decisions in specific contexts (22).
UpH may be analyzed in freshly collected urine, or in a sample collected under mineral oil to minimize CO2 diffusion when measurement is delayed (especially when pH values ≥6.0 are expected) (24). However, collection under mineral oil can be omitted if the sample is stored at 4 °C in a regular disposable plastic syringe (capping the drawing needle and avoiding air bubbles) and the measurement is performed within 24 hours (25).
As UpH is a measure of H+ concentration, its value is dependent on urine concentration status. An extremely diluted urine may provide a falsely elevated UpH reading even when H+ excretion is normal, so an overnight thirst period is recommended before collecting the sample. It should also be taken in consideration that UpH follows a circadian rhythm, decreasing during the night, reaching its minimum before dawn and rising after common western meals (26–28). A low spontaneous UpH measured in a fasting spot morning urine is considered to rule out an acidification disorder without the need for more specific tests. In fact, fasting morning UpH has shown a better correlation with nadir UpH after an ammonium chloride (NH4Cl) load −the gold standard for the evaluation of urinary acidification− than 24-hour UpH (29). There is no consensus on the type of sample: the first vs. the second (usually after a period of 1–2 h after awakening) fasting morning urine sample. Chafe and Gault (30) reported that the first morning urine pH might be a better predictor of urinary acidification after an NH4Cl load than the second fasting urine. The morning rise of UpH, even in the absence of food or drink, has been well reported as a part of its circadian rhythm (26, 31, 32) and might account for this result. Consequently, the first morning urine, which is easier to collect and avoids the problems of a prolonged fasting period, could be the best option to evaluate UpH in continent children. Special considerations should be taken into account in cases of nocturnal enuresis and in infants who feed at night.
In the absence of metabolic acidosis and, outside the context of functional tests, UNH4+ is usually measured in 24-hour urine. A review on the available data on daily NH4+ excretion in adults under normal and various pathological conditions has recently been published (15). In children, there are very few data. Manz et al. (33) measured daily NAE in healthy children between 3 and 18 years old, but UNH4+ values were not reported.
24-hour urine collection is difficult to perform, requiring preservatives to avoid increases in UNH4+ until the time of analysis and bladder catheterization in young children and infants. Some studies have used spot urine samples in adults (34, 35), which are easier to collect, but UNH4+ concentration may not represent faithfully NH4+ excretion. To minimize the effect of variations in urine flow and concentration, a better parameter is the urine NH4+/creatinine ratio measured in the same sample.
Renal NH4+ excretion shows also a circadian rhythm (36) and increases following protein intake (37), so the best time for sample collection may be the first fasting morning urine. In fact, our group recently published urine NH4+/creatinine ratio reference values for children over 5 years old in spot morning urine, ranging between 776 and 8217 µmol/mmol (38). This may clear the path for applying direct spot UNH4+ measurement in daily clinical practice in children.
Traditionally, individual samples for UNH4+ quantification were collected in paraffin and shipped to the laboratory on ice (39), but we showed no significant differences between samples collected with or without paraffin (17), so samples can be collected in tubes without additives and sent to the laboratory without further preparation. UNH4+ concentrations have proven also to be stable up to 48 h at room temperature, up to 9 days at 4 °C and −20 °C, and for at least 2 years when stored at −80 °C (18). However, in order to avoid contamination and bacterial growth, they should be centrifuged, separated and then analyzed as soon as possible or frozen if the analysis is delayed.
Taking all the above into consideration, the first fasting morning urine, in our opinion, is the best sample to evaluate UpH and UNH4+/creatinine ratio simultaneously.
The main indication for evaluating urinary acidification in children is during the assessment and diagnosis of normal anion gap (hyperchloremic) metabolic acidosis, which can result, among other causes, from the loss of HCO3− from the gastrointestinal tract or kidney (proximal renal tubular acidosis –pRTA–), the addition of hydrochloric acid (HCl) or substances metabolized to HCl, or an impaired net renal acid excretion, as happens in distal renal tubular acidosis –dRTA– or in chronic kidney disease (CKD), when glomerular filtration rate (GFR) is significantly reduced (usually <20–25 ml/min/1.73 m2) (40).
The extrarenal (digestive) causes of hyperchloremic metabolic acidosis are the most common. In this context, when kidney function is preserved, UpH should be low and UNH4+ should rise. The increase in UNH4+ excretion is modest initially, but is maximized after 3–5 days if the stimulus persists (1, 3, 6). When more frequent extrarenal causes and CKD are excluded, an assessment of urinary acidification is indicated (40, 41). In these situations, an inappropriately high UpH with low UNH4+, coexisting with metabolic acidosis, indicate an impaired distal urinary acidification (42). If distal acidification is preserved, efforts should be directed to evaluate renal HCO3− wasting (40). Usually, it is not necessary to measure UpH and UNH4+ in most cases, since the underlying disorder is usually identifiable with a comprehensive history, physical examination and basic laboratory tests. However, the determination of UpH and UNH4+ in single spot urine can be a useful and simple tool in the assessment of normal anion gap metabolic acidosis, especially when the cause is not clear or an underlying kidney pathology is suspected (40, 41).
Another less frequent indication for UpH and UNH4+ measurement in pediatrics is during the realization of functional urinary acidification tests for the confirmatory diagnosis of dRTA, such as the oral NH4Cl load (43) and the furosemide + fludrocortisone test (29, 44, 45). In healthy subjects, UpH should decrease below 5.3 in both tests (although this precise value is disputed) and UNH4+ should rise up to 57 ± 14 (mean ± SD) µEq/min/1.73 m2 in infants aged 1–16 months and 80 ± 12 µEq/min/1.73 m2 in children aged 7–12 years (45) in the oral NH4Cl load.
However, dRTA in children is mostly primary, caused by genetic alterations (41, 42, 46, 47) and the increasing availability of genetic testing has partly relegated functional tests in this context.
Incomplete dRTA is a disorder defined by an inability to maximally acidify urine in the absence of spontaneous metabolic acidosis (47). It is a condition that has been reported mostly in adults, since its first description in 1959 (48). In these patients, the acidification defect is milder and NH4+ excretion is greater than in those with complete dRTA (49), a fact that may account for the absence of overt metabolic acidosis. The underlying mechanism that causes the impairment in urinary acidification is not well understood and may be dependent on the associated disorders (47).
Incomplete dRTA has been associated to recurrent calcium kidney stones in adults (50), nephrocalcinosis (50, 51), as well as to osteopenia or osteoporosis (52–54). In these cases, alkali therapy has shown to reduce stone formation and increase bone mass (54, 55). It has also been reported in sickle-cell disease (56), interstitial nephropathies (57) and autoimmune diseases [especially, Sjögren disease (58)].
The diagnosis of incomplete dRTA can be difficult to perform, since, by definition, serum acid-base balance parameters (including HCO3−) are normal. Incomplete dRTA can be suspected in case of a persistently high UpH, but its confirmation requires the measurement of UpH and UNH4+ in the same functional tests used to identify complete dRTA (42, 46, 47, 50). However, a low spontaneous UpH is usually considered to rule out an acidification defect without the need for functional tests (41, 47). Although, classically, the threshold value is 5.3 (48), cut-off points between 5.25 and 6.10 have been proposed and used. A list of publications on incomplete dRTA screening, along with the threshold values is provided in Table 1.
Table 1. Studies that analyze single spot urine pH (UpH) in the context of incomplete dRTA assessment.
Incomplete dRTA in children has scarcely been reported and its manifestations are not well established. Nevertheless, and, unlike in adults, it has been associated to congenital abnormalities in the kidney and urinary tract (68, 69), asymptomatic hypocitraturia (59), active nutritional rickets (70) and it also has been reported in heterozygous carriers of gene mutations responsible for primary dRTA (71) who did not present spontaneous metabolic acidosis. Furthermore, whether it is also a cause of growth retardation in patients with vesicoureteral reflux and posterior ureteral valves (68, 69) without other growth impairment causes, remains an open question. Interestingly, sustained bicarbonate therapy has resulted in growth improvement in some of these cases (72). Therefore, incomplete dRTA evaluation could have important implications in clinical practice for diagnosing causes of growth failure in children, when the cause cannot be identified with routine testing (47, 73).
In addition to the very limited number of published studies on incomplete dRTA in pediatric age, data on UpH values in children are scarce and mostly limited to timed urine samples (74–76). Skinner et al. analyzed in 1996 the first morning UpH in 322 healthy children and found that only one child out of eight had a UpH ≤ 5.4, the median value being 6.0 (77). These results suggest that the utility of fasting UpH in detecting acidification defects is limited, at least when considered in isolation, and point out to the need for more data on pediatric reference UpH values and their relationship with other associated urinary acidification parameters, such as osmolality, electrolytes and, especially, UNH4+.
Recent studies have emphasized the importance of measuring UNH4+ in CKD. An impaired net acid excretion (and, accordingly, low NH4+ secretion), with the consequent acid accumulation, contributes both to kidney injury (78) and to the pathogenesis of metabolic acidosis, which is a risk factor for progression of renal function deterioration and mortality (79, 80). Indeed, a low 24-hour UNH4+ level (<20 mmol/day) has been found to be a marker of poor outcome in adult CKD patients without overt metabolic acidosis (80).
Acid accumulation is a well-known risk factor for progression of renal failure and mortality in CKD (80, 81), even when it is insufficient to cause clinically apparent metabolic acidosis, the so-called “eubicarbonatemic” acidosis (78). Acid accumulation in CKD depends, among other factors, on the reduction of GFR, the dietary acid and the integrity of distal acidification mechanisms. Acid-related kidney injury is not limited to patients with reduced GFR and is favored by high-acid diets, such as rich in protein Western diets (78). The identification of eubicarbonatemic acidosis, in order to prescribe dietary interventions or alkali therapy, even in early CKD stages, could decrease acid accumulation and slow CKD progress in these patients (78). Although acid accumulation can be estimated by serum HCO3− levels after an oral HCO3− load (82), more practical and easier surrogate parameters are needed. In this context, reduced urinary NH4+ excretion might indicate risk for acid accumulation (78).
These data from adult CKD patients and the clinical implications of eubicarbonatemic acidosis need to be evaluated in children. Congenital kidney and urinary tract abnormalities, which are usually associated to tubulointerstitial injury, constitute a frequent cause of CKD in pediatric patients, especially in younger children (83). In these cases, where tubular function may be impaired already in early CKD stages, there might be a higher proportion of eubicarbonatemic acidosis than in adults, even when GFR is normal. This possibility and the potential clinical utility of UNH4+ quantification in pediatric CKD patients, need to be explored.
The identification of a urinary acidification disorder needs a high degree of suspicion and can be difficult, especially when there is not a coexisting metabolic acidosis and serum HCO3− is within range, as in incomplete dRTA or eubicarbonatemic acidosis in CKD. Thus, there might be an important number of undiagnosed cases of acidification defects, both in adults and children, which can benefit from early identification and treatment. Since a confirmatory diagnosis requires a functional test, an accurate screening method is necessary. Ideally, this test should be non-invasive and easy to perform, such as the collection of a spot urine sample.
UpH is easy to measure but it cannot be used alone as a screening tool. UNH4+ represents the most important part of net acid excretion and can provide more information on urinary acidification. Although traditionally not measured in clinical practice due to historical technical difficulties, which have been largely overcome, direct UNH4+ quantification has gained in importance in the past few years in different conditions.
In fact, UpH and UNH4+ measurement has shown to be feasible in clinical laboratories nowadays and its applications in clinical practice are starting to be discovered. The assessment of UpH and UNH4+ should become a part of the evaluation of metabolic acidosis as a simple but informative diagnostic tool. Furthermore, evidence in adult patients also points out to their utility in cases of eubicarbonatemic acidosis and in order to identify incomplete dRTA.
However, there are very few data on UpH and UNH4+ in pediatric population, so further research is needed to establish reference values in children, either in fasting conditions or in acidosis of nonrenal origin, and to explore the clinical applications of these measurements in metabolic acidosis, in CKD with a reduction of GFR and in incomplete dRTA associated disorders, including growth retardation of unknown cause. The recent availability of morning spot UNH4+/creatinine ratio reference values in children (38) may clear the path for future studies.
LD, LC, FS and HG contributed to the conception of this review. LD did the bibliographic search and wrote the first draft of the manuscript. LC, FS and HG wrote sections of the manuscript. All authors contributed to the article and approved the submitted version.
This study has been funded by Instituto de Salud Carlos III (ISCIII) through the project PI20/00922 and co-funded by the European Union.
The authors declare that the research was conducted in the absence of any commercial or financial relationships that could be construed as a potential conflict of interest.
All claims expressed in this article are solely those of the authors and do not necessarily represent those of their affiliated organizations, or those of the publisher, the editors and the reviewers. Any product that may be evaluated in this article, or claim that may be made by its manufacturer, is not guaranteed or endorsed by the publisher.
1. Hamm LL, Nakhoul N, Hering-Smith KS. Acid-base homeostasis. CJASN. (2015) 10(12):2232–42. doi: 10.2215/CJN.07400715
2. Hamm LL, Simon EE. Roles and mechanisms of urinary buffer excretion. Am J Physiol. (1987) 253(4):F595–F605. doi: 10.1152/ajprenal.1987.253.4.F595
3. Carlisle EJ, Donnelly SM, Halperin ML. Renal tubular acidosis (RTA): recognize the ammonium defect and pHorget the urine pH. Pediatr Nephrol. (1991) 5(2):242–8. doi: 10.1007/BF01095965
4. Lemann J, Bushinsky DA, Hamm LL. Bone buffering of acid and base in humans. Am J Physiol. (2003) 285(5):F811–32. doi: 10.1152/ajprenal.00115.2003
5. Carlisle EJ, Donnelly SM, Vasuvattakul S, Kamel KS, Tobe S, Halperin ML. Glue-sniffing and distal renal tubular acidosis: sticking to the facts. J Am Soc Nephrol. (1991) 1(8):1019–27. doi: 10.1681/ASN.V181019
6. Kamel KS, Halperin ML. Use of urine electrolytes and urine osmolality in the clinical diagnosis of fluid, electrolytes, and acid-base disorders. Kidney Int Rep. (2021) 6(5):1211–24. doi: 10.1016/j.ekir.2021.02.003
7. Batlle DC, Hizon M, Cohen E, Gutterman C, Gupta R. The use of the urinary anion gap in the diagnosis of hyperchloremic metabolic acidosis. N Engl J Med. (1988) 318(10):594–9. doi: 10.1056/NEJM198803103181002
8. Goldstein MB, Bear R, Richardson RM, Marsden PA, Halperin ML. The urine anion gap: a clinically useful index of ammonium excretion. Am J Med Sci. (1986) 292(4):198–202. doi: 10.1097/00000441-198610000-00003
9. Kim GH, Han JS, Kim YS, Joo KW, Kim S, Lee JS. Evaluation of urine acidification by urine anion gap and urine osmolal gap in chronic metabolic acidosis. Am J Kidney Dis. (1996) 27(1):42–7. doi: 10.1016/S0272-6386(96)90029-3
10. Sulyok E, Guignard JP. Relationship of urinary anion gap to urinary ammonium excretion in the neonate. Biol Neonate. (1990) 57(2):98–106. doi: 10.1159/000243169
11. Ha LY, Chiu WW, Davidson JS. Direct urine ammonium measurement: time to discard urine anion and osmolar gaps. Ann Clin Biochem. (2012) 49(Pt 6):606–8. doi: 10.1258/acb.2012.012013
12. Raphael KL, Gilligan S, Ix JH. Urine anion gap to predict urine ammonium and related outcomes in kidney disease. Clin J Am Soc Nephrol. (2018) 13(2):205–12. doi: 10.2215/CJN.03770417
13. Raphael KL, Ix JH. Correlation of urine ammonium and urine osmolal gap in kidney transplant recipients. Clin J Am Soc Nephrol. (2018) 13(4):638–40. doi: 10.2215/CJN.13311117
14. Uribarri J, Oh MS. The urine anion gap: common misconceptions. J Am Soc Nephrol. (2021) 32(5):1025–8. doi: 10.1681/ASN.2020101509
15. Uribarri J, Goldfarb DS, Raphael KL, Rein JL, Asplin JR. Beyond the urine anion gap: in support of the direct measurement of urinary ammonium. Am J Kidney Dis. (2022):S0272-6386(22)00768-5. doi: 10.1053/j.ajkd.2022.05.009 [Epub ahead of print]35810828
16. Katagawa K, Nagashima T, Inase N, Kanayama M, Chida M, Sasaki S, et al. Urinary ammonium measurement by the auto-analyzer method. Kidney Int. (1989) 36(2):291–4. doi: 10.1038/ki.1989.193
17. Cardo L, Gil-Peña H, García-García M, Fernández JC, Santos F, Álvarez FV. Implementation of an automated method for direct quantification of urinary ammonium. Clin Chem Lab Med. (2019) 57(8):e203–5. doi: 10.1515/cclm-2018-1250
18. Gruzdys V, Cahoon K, Pearson L, Raphael KL. Measurement of urinary ammonium using a commercially available plasma ammonium assay. Kidney 360. (2022) 3(5):926–932. doi: 10.34067/KID.0000262022
19. Desai RA, Assimos DG. Accuracy of urinary dipstick testing for pH manipulation therapy. J Endourol. (2008) 22(6):1367–70. doi: 10.1089/end.2008.0053
20. Grases F, Rodriguez A, Berga F, Costa-Bauza A, Prieto RM, Burdallo I, et al. A new device for simple and accurate urinary pH testing by the stone-former patient. SpringerPlus. (2014) 3(1):209. doi: 10.1186/2193-1801-3-209
21. Angerri O, Pascual D, Haro J, Fernández X, Chiganças V, Garganta R, et al. Comparative study between a medical device and reagent dipsticks in measuring pH. Arch Esp Urol. (2020) 73(6):546–53. PMID: 3263325032633250
22. Wockenfus AM, Koch CD, Conlon PM, Sorensen LD, Cambern KL, Chihak AJ, et al. Discordance between urine pH measured by dipstick and pH meter: implications for methotrexate administration protocols. Clin Biochem. (2013) 46(1–2):152–4. doi: 10.1016/j.clinbiochem.2012.10.018
23. Kwong T, Robinson C, Spencer D, Wiseman OJ, Karet Frankl FE. Accuracy of urine pH testing in a regional metabolic renal clinic: is the dipstick accurate enough? Urolithiasis. (2013) 41(2):129–32. doi: 10.1007/s00240-013-0546-y
24. Yi JH, Shin HJ, Kim SM, Han SW, Kim HJ, Oh MS. Does the exposure of urine samples to air affect diagnostic tests for urine acidification? Clin J Am Soc Nephrol. (2012) 7(8):1211–6. doi: 10.2215/CJN.03230312
25. Srivastava T, Kainer G. Collection under paraffin is not necessary for stability of urine pH over 24 h. Pediatr Nephrol. (2004) 19(2):169–71. doi: 10.1007/s00467-003-1334-7
26. Mills JN, Stanbury SW. Intrinsic diurnal rhythm in urinary electrolyte output. J Physiol. (1951) 115(1):18p–9p. PMID: 1488945014889450
27. Vahlensieck EW, Bach D, Hesse A. Circadian rhythm of lithogenic substances in the urine. Urol Res. (1982) 10(4):195–203. doi: 10.1007/BF00255944
28. Bilobrov VM, Chugaj AV, Bessarabov VI. Urine pH variation dynamics in healthy individuals and stone formers. Urol Int. (1990) 45(6):326–31. doi: 10.1159/000281730
29. Dhayat NA, Gradwell MW, Pathare G, Anderegg M, Schneider L, Luethi D, et al. Furosemide/fludrocortisone test and clinical parameters to diagnose incomplete distal renal tubular acidosis in kidney stone formers. CJASN. (2017) 12(9):1507–17. doi: 10.2215/CJN.01320217
30. Chafe L, Gault MH. First morning urine pH in the diagnosis of renal tubular acidosis with nephrolithiasis. Clin Nephrol. (1994) 41(3):159–62. PMID: 81873598187359
31. Barnett GD, Blume FE. Alkaline tides. J Clin Invest. (1938) 17(2):159–65. doi: 10.1172/JCI100939
32. Ayres JW, Weidler DJ, MacKichan J, Wagner JG. Circadian rhythm of urinary pH in man with and without chronic antacid administration. Eur J Clin Pharmacol. (1977) 12(6):415–20. doi: 10.1007/BF00561060
33. Manz F, Wentz A. Renal net acid excretion related to body surface area in children and adolescents. Pediatr Nephrol. (2000) 15(1–2):101–4. doi: 10.1007/s004670000424
34. Dyck RF, Asthana S, Kalra J, West ML, Massey L. A modification of the urine osmolal gap: an improved method for estimating urine ammonium. Am J Nephrol. (1990) 10(5):359–62. doi: 10.1159/000168150
35. Fujimaru T, Shuo T, Nagahama M, Taki F, Nakayama M, Komatsu Y. Assessing urine ammonium concentration by urine osmolal gap in chronic kidney disease. Nephrology. (2021) 26(10):809–13. doi: 10.1111/nep.13937
36. Cameron M, Maalouf NM, Poindexter J, Adams-Huet B, Sakhaee K, Moe OW. The diurnal variation in urine acidification differs between normal individuals and uric acid stone formers. Kidney Int. (2012) 81(11):1123–30. doi: 10.1038/ki.2011.480
37. Remer T. Influence of nutrition on acid-base balance - metabolic aspects. Eur J Nutr. (2001) 40(5):214–20. doi: 10.1007/s394-001-8348-1
38. Cardo L, Gil-Peña H, Álvarez FV, Santos F. Urinary ammonium: paediatric reference values. Acta Paediatr. (2021) 110(2):659–60. doi: 10.1111/apa.15544
39. Szmidt-Adjidé V, Vanhille P. [Urinary ammonium: validation of an enzymatic method and reliability with an indirect urine ammonium estimation]. Ann Biol Clin (Paris). (2008) 66(4):393–9. doi: 10.1684/abc.2008.0232
40. Kraut JA, Madias NE. Differential diagnosis of nongap metabolic acidosis: value of a systematic approach. Clin J Am Soc Nephrol. (2012) 7(4):671–9. doi: 10.2215/CJN.09450911
41. Trepiccione F, Walsh SB, Ariceta G, Boyer O, Emma F, Camilla R, et al. Distal renal tubular acidosis: ERKNet/ESPN clinical practice points. Nephrol Dial Transplant. (2021) 36(9):1585–96. doi: 10.1093/ndt/gfab171
42. Santos F, Ordóñez FA, Claramunt-Taberner D, Gil-Peña H. Clinical and laboratory approaches in the diagnosis of renal tubular acidosis. Pediatr Nephrol. (2015) 30(12):2099–107. doi: 10.1007/s00467-015-3083-9
43. Edelmann CM, Soriano JR, Boichis H, Gruskin AB, Acosta MI. Renal bicarbonate reabsorption and hydrogen ion excretion in normal infants. J Clin Invest. (1967) 46(8):1309–17. doi: 10.1172/JCI105623
44. Walsh SB, Shirley DG, Wrong OM, Unwin RJ. Urinary acidification assessed by simultaneous furosemide and fludrocortisone treatment: an alternative to ammonium chloride. Kidney Int. (2007) 71(12):1310–6. doi: 10.1038/sj.ki.5002220
45. Shavit L, Chen L, Ahmed F, Ferraro PM, Moochhala S, Walsh SB, et al. Selective screening for distal renal tubular acidosis in recurrent kidney stone formers: initial experience and comparison of the simultaneous furosemide and fludrocortisone test with the short ammonium chloride test. Nephrol Dial Transplant. (2016) 31(11):1870–6. doi: 10.1093/ndt/gfv423
46. Santos F, Gil-Peña H, Alvarez-Alvarez S. Renal tubular acidosis. Curr Opin Pediatr. (2017) 29(2):206–10. doi: 10.1097/MOP.0000000000000460
47. Alonso-Varela M, Gil-Peña H, Santos F. Incomplete distal renal tubular acidosis in children. Acta Paediatr. (2020) 109(11):2243–50. doi: 10.1111/apa.15269
48. Wrong O, Davies HE. The excretion of acid in renal disease. Q J Med. (1959) 28(110):259–313. PMID: 13658353.13658353
49. Caruana RJ, Buckalew VM. The syndrome of distal (type 1) renal tubular acidosis. Clinical and laboratory findings in 58 cases. Medicine (Baltimore). (1988) 67(2):84–99. doi: 10.1097/00005792-198803000-00002
50. Fuster DG, Moe OW. Incomplete distal renal tubular acidosis and kidney stones. Adv Chronic Kidney Dis. (2018) 25(4):366–74. doi: 10.1053/j.ackd.2018.05.007
51. Tannen RL, Falls WF Jr, Brackett NC Jr. Incomplete renal tubular acidosis: some clinical and physiological features. Nephron. (1975) 15(2):111–23. doi: 10.1159/000180502
52. Weger M, Deutschmann H, Weger W, Kotanko P, Skrabal F. Incomplete renal tubular acidosis in “primary” osteoporosis. Osteoporos Int. (1999) 10(4):325–9. doi: 10.1007/s001980050235
53. Weger W, Kotanko P, Weger M, Deutschmann H, Skrabal F. Prevalence and characterization of renal tubular acidosis in patients with osteopenia and osteoporosis and in non-porotic controls. Nephrol Dial Transplant. (2000) 15(7):975–80. doi: 10.1093/ndt/15.7.975
54. Sromicki JJ, Hess B. Abnormal distal renal tubular acidification in patients with low bone mass: prevalence and impact of alkali treatment. Urolithiasis. (2017) 45(3):263–9. doi: 10.1007/s00240-016-0906-5
55. Preminger GM, Sakhaee K, Skurla C, Pak CYC. Prevention of recurrent calcium stone formation with potassium citrate therapy in patients with distal renal tubular acidosis. J Urol. (1985) 134(1):20–3. doi: 10.1016/S0022-5347(17)46963-1
56. Oster JR, Lespier LE, Lee SM, Pellegrini EL, Vaamonde CA. Renal acidification in sickle-cell disease. J Lab Clin Med. (1976) 88(3):389–401. PMID: 85748574
57. Osther PJ, Hansen AB, Røhl HF. Renal acidification defects in medullary sponge kidney. Br J Urol. (1988) 61(5):392–4. doi: 10.1111/j.1464-410X.1988.tb06581.x
58. Jain A, Srinivas BH, Emmanuel D, Jain VK, Parameshwaran S, Negi VS. Renal involvement in primary Sjogren’s syndrome: a prospective cohort study. Rheumatol Int. (2018) 38(12):2251–62. doi: 10.1007/s00296-018-4118-x
59. Norman ME, Feldman NI, Cohn RM, Roth KS, McCurdy DK. Urinary citrate excretion in the diagnosis of distal renal tubular acidosis. J Pediatr. (1978) 92(3):394–400. doi: 10.1016/S0022-3476(78)80426-0
60. Konnak JW, Kogan BA, Lau K. Renal calculi associated with incomplete distal renal tubular acidosis. J Urol. (1982) 128(5):900–2. doi: 10.1016/S0022-5347(17)53268-1
61. Antón F M, Puig J G, Gaspar G, Martínez ME, Ramos T, Martínez Piñeiro JA. Renal tubular acidosis in recurrent renal stone formers. Eur Urol. (1984) 10(1):55–9. doi: 10.1159/000463513
62. Osther PJ, Hansen AB, Röhl HF. Renal acidification defects in patients with their first renal stone episode. Scand J Urol Nephrol Suppl. (1988) 110:275–8. PMID: 31874233187423
63. Osther PJ, Hansen AB, Røhl HF. Screening renal stone formers for distal renal tubular acidosis. Br J Urol. (1989) 63(6):581–3. doi: 10.1111/j.1464-410X.1989.tb05249.x
64. Gault MH, Chafe LL, Morgan JM, Parfrey PS, Harnett JD, Walsh EA, et al. Comparison of patients with idiopathic calcium phosphate and calcium oxalate stones. Medicine (Baltimore). (1991) 70(6):345–59. doi: 10.1097/00005792-199111000-00001
65. Pongchaiyakul C, Domrongkitchaiporn S, Stitchantrakul W, Chailurkit L, Rajatanavin R. Incomplete renal tubular acidosis and bone mineral density: a population survey in an area of endemic renal tubular acidosis. Nephrol Dial Transplant. (2004) 19(12):3029–33. doi: 10.1093/ndt/gfh534
66. Stitchantrakul W, Kochakarn W, Ruangraksa C, Domrongkitchaiporn S. Urinary risk factors for recurrent calcium stone formation in Thai stone formers. J Med Assoc Thai. (2007) 90(4):688–98. PMID: 1748712317487123
67. Arampatzis S, Röpke-Rieben B, Lippuner K, Hess B. Prevalence and densitometric characteristics of incomplete distal renal tubular acidosis in men with recurrent calcium nephrolithiasis. Urol Res. (2012) 40(1):53–9. doi: 10.1007/s00240-011-0397-3
68. Guizar JM, Kornhauser C, Malacara JM, Sanchez G, Zamora J. Renal tubular acidosis in children with vesicoureteral reflux. J Urol. (1996) 156(1):193–5. doi: 10.1016/S0022-5347(01)65995-0
69. Sharma AP, Sharma RK, Kapoor R, Kornecki A, Sural S, Filler G. Incomplete distal renal tubular acidosis affects growth in children. Nephrol Dial Transplant. (2007) 22(10):2879–85. doi: 10.1093/ndt/gfm307
70. Oduwole AO, Giwa OS, Arogundade RA. Relationship between rickets and incomplete distal renal tubular acidosis in children. Ital J Pediatr. (2010) 36(1):54. doi: 10.1186/1824-7288-36-54
71. Zhang J, Fuster DG, Cameron MA, Quiñones H, Griffith C, Xie X-S, et al. Incomplete distal renal tubular acidosis from a heterozygous mutation of the V-ATPase B1 subunit. Am J Physiol Renal Physiol. (2014) 307(9):F1063–71. doi: 10.1152/ajprenal.00408.2014
72. Sharma AP, Singh RN, Yang C, Sharma RK, Kapoor R, Filler G. Bicarbonate therapy improves growth in children with incomplete distal renal tubular acidosis. Pediatr Nephrol. (2009) 24(8):1509–16. doi: 10.1007/s00467-009-1169-y
73. Medeiros M, Enciso S, Hernández AM, García Hernández HR, Toussaint G, Pinto C, et al. Case report of renal tubular acidosis and misdiagnosed. Nefrologia. (2016) 36(3):323–5. doi: 10.1016/j.nefro.2015.10.012
74. Bergsland KJ, Coe FL, White MD, Erhard MJ, DeFoor WR, Mahan JD, et al. Urine risk factors in children with calcium kidney stones and their siblings. Kidney Int. (2012) 81(11):1140–8. doi: 10.1038/ki.2012.7
75. Rodriguez A, Saez-Torres C, Mir C, Casasayas P, Rodriguez N, Rodrigo D, et al. Effect of sample time on urinary lithogenic risk indexes in healthy and stone-forming adults and children. BMC Urol. (2018) 18(1):116. doi: 10.1186/s12894-018-0430-8
76. Mir C, Rodriguez A, Rodrigo D, Saez-Torres C, Frontera G, Lumbreras J, et al. Analysis of urine composition from split 24-h samples: use of 12-h overnight samples to evaluate risk factors for calcium stones in healthy and stone-forming children. J Pediatr Urol. (2020) 16(3):371.e1–e7. doi: 10.1016/j.jpurol.2020.02.011
77. Skinner R, Cole M, Pearson ADJ, Coulthard MG, Craft AW. Specificity of pH and osmolality of early morning urine sample in assessing distal renal tubular function in children: results in healthy children. Br Med J. (1996) 312(7042):1337–8. doi: 10.1136/bmj.312.7042.1337
79. Vallet M, Metzger M, Haymann J-P, Flamant M, Gauci C, Thervet E, et al. Urinary ammonia and long-term outcomes in chronic kidney disease. Kidney Int. (2015) 88(1):137–45. doi: 10.1038/ki.2015.52
80. Raphael KL, Carroll DJ, Murray J, Greene T, Beddhu S. Urine ammonium predicts clinical outcomes in hypertensive kidney disease. J Am Soc Nephrol. (2017) 28(8):2483–90. doi: 10.1681/ASN.2016101151
81. Raphael KL. Metabolic acidosis and subclinical metabolic acidosis in CKD. J Am Soc Nephrol. (2018) 29(2):376–82. doi: 10.1681/ASN.2017040422
82. Wesson DE. Assessing acid retention in humans. Am J Physiol. (2011) 301(5):F1140–2. doi: 10.1152/ajprenal.00346.2011
Keywords: urinary acidification, urine pH, urinary ammonium, spot urine sample, hyperchloremic metabolic acidosis, incomplete distal renal tubular acidosis
Citation: Díaz-Anadón L, Cardo L, Santos F and Gil-Peña H (2022) Evaluation of urinary acidification in children: Clinical utility. Front. Pediatr. 10:1051481. doi: 10.3389/fped.2022.1051481
Received: 22 September 2022; Accepted: 10 October 2022;
Published: 31 October 2022.
Edited by:
Orkun Tolunay, University of Health Sciences, Turkey© 2022 Díaz-Anadón, Cardo, Santos and Gil-Peña. This is an open-access article distributed under the terms of the Creative Commons Attribution License (CC BY). The use, distribution or reproduction in other forums is permitted, provided the original author(s) and the copyright owner(s) are credited and that the original publication in this journal is cited, in accordance with accepted academic practice. No use, distribution or reproduction is permitted which does not comply with these terms.
*Correspondence: Leire Cardo bGV5cmVjYXJkb0BnbWFpbC5jb20=
Specialty Section: This article was submitted to Pediatric Nephrology, a section of the journal Frontiers in Pediatrics
Disclaimer: All claims expressed in this article are solely those of the authors and do not necessarily represent those of their affiliated organizations, or those of the publisher, the editors and the reviewers. Any product that may be evaluated in this article or claim that may be made by its manufacturer is not guaranteed or endorsed by the publisher.
Research integrity at Frontiers
Learn more about the work of our research integrity team to safeguard the quality of each article we publish.