- 1Neonatal Unit, Homerton Healthcare NHS Foundation Trust, London, United Kingdom
- 2Neonatal Unit, Imperial College Healthcare NHS Trust and Imperial College London, London, United Kingdom
- 3University College London Great Ormond Street Institute of Child Health, London, England
- 4Barts and The London School of Medicine and Dentistry, Queen Mary University of London (QMUL), London, United Kingdom
Despite advances in neonatal care Necrotising Enterocolitis (NEC) continues to have a significant mortality and morbidity rate, and with increasing survival of those more immature infants the population at risk of NEC is increasing. Ischaemia, reperfusion, and inflammation underpin diseases affecting intestinal blood flow causing gut injury including Necrotising Enterocolitis. There is increasing interest in tissue biomarkers of gut injury in neonates, particularly those representing changes in intestinal wall barrier and permeability, to determine whether these could be useful biomarkers of gut injury. This article reviews current and newly proposed markers of gut injury, the available literature evidence, recent advances and considers how effective they are in clinical practice. We discuss each biomarker in terms of its effectiveness in predicting NEC onset and diagnosis or predicting NEC severity and then those that will aid in surveillance and identifying those infants are greatest risk of developing NEC.
Introduction
Necrotising Enterocolitis (NEC) remains one of the most significant complications of prematurity (1) with a high mortality and morbidity rate. It often presents acutely and causes rapid deterioration. For this reason, biomarkers of gut tissue injury have been examined for over 20 years with the hope of identifying non-invasive reliable markers that could predict the onset of NEC before clinical signs, allowing the opportunity for earlier interventions and improved clinical outcomes.
However, many of these biomarkers are non-specific or difficult and invasive to measure. In addition the main gastrointestinal diseases affecting preterm neonates are NEC and septic ileus; both trigger translocation of bacteria and luminal contents into the host related to intestinal permeability and impaired intestinal barrier function and it is often difficult to differentiate between them (2). Delays in diagnosis can result in surgery being performed when most of the gut is necrotic, resulting in short-bowel syndrome or withdrawal of care due to NEC totalis.
From a clinical perspective there are numerous approaches to finding a gut biomarker as a non-invasive measurement of gut injury rather than directly sampling the intestine, including stool, urine, and serum blood samples, as well as using clinical features known to predate NEC onset and newer emerging modalities such as Near Infrared Spectroscopy (NIRS) which reflects regional oxygenation (3) (Figure 1). Biomarkers range from non-specific biomarkers that form part of the proinflammatory and anti-inflammatory pathways of the immune system including C reactive protein and cytokines, non-specific gut biomarkers that can also be raised in other causes of inflammation such as calprotectin and volatile organic compounds and lastly more specific biomarkers that are associated with gut pathology which include fatty acid binding proteins (FABP) and trefoil factor-3 (TFF-3).
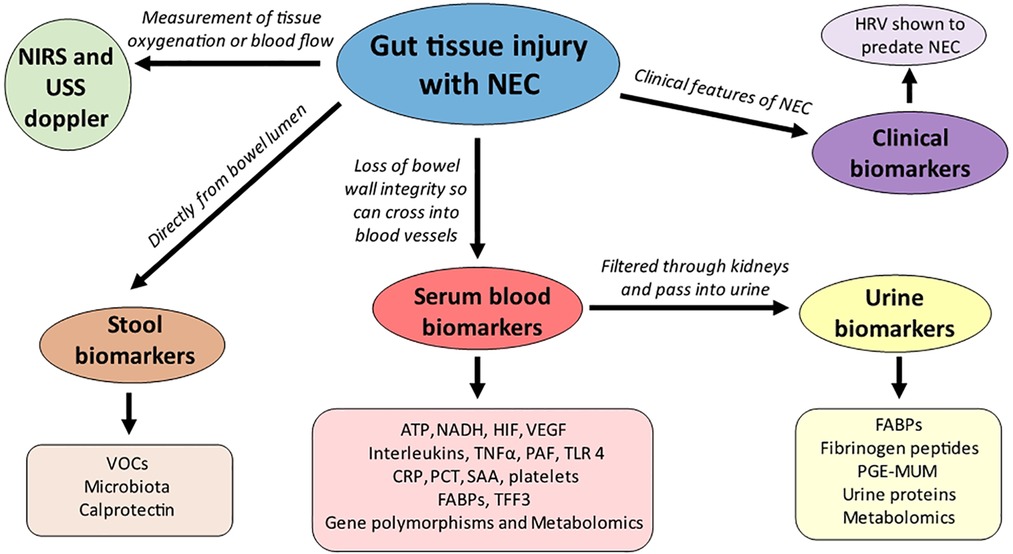
Figure 1. Non-invasive gut biomarkers and their origins (sample) of detection. ATP- adenosine triphosphate; CRP- C reactive protein; FABPs- fatty acid binding proteins; HIF-hypoxia inducible factor; NADH- nicotinamide adenine dinucleotide; NIRS- near infrared spectroscopy; PAF- platelet activating factor; PCT- procalcitonin; PGE-MUM- prostaglandin E2 Major urinary metabolite; SAA-serum amyloid A; TFF3- trefoil factor 3;TLR-4 toll like receptor 4; TNFα- tumour necrosis factor alpha; VEGF-vascular endothelial growth factor; VOCs-volatile organic compounds.
The ideal biomarker should have a modest sensitivity and high specificity in diagnosing NEC, would rapidly increase in response to disease onset, preferably prior to the onset of clinical signs, and decrease quickly in response to treatment and clinical improvement. In addition, the results should be available quickly to facilitate rapid decision making. Clearly for extreme preterm infants (those most at risk of NEC) minimising invasive and painful procedures, (e.g., venepuncture) is important, therefore another important criterion is to be non-invasive. Biomarkers of NEC are currently a hot topic in Neonatology as researchers endeavour to find ways which enable clinicians to detect this devastating condition earlier. There have been many reviews on this topic (4–10) which only serves to highlight just how important this topic is to the neonatal community. This narrative review focuses on the proposed tissue biomarkers but provides updates on more recently suggested biomarkers along with their benefits and pitfalls. We will examine recent advances, as well as newly proposed biomarkers, the “omics” era and clinical prediction models. We have aimed to discuss each biomarker in terms of the likelihood of it being used in clinical practice by examining their effectiveness in predicting NEC onset and diagnosis or predicting NEC severity or whether it will aid in surveillance and identifying those infants at greatest risk of developing NEC.
Endogenous/exogenous biomarkers representing hypoxia injury
Direct measurement of tissue oxygenation and hypoxia in vivo is not possible therefore various surrogate markers have been proposed including some biomarkers in serum, such as serum lactate, which may represent the change of state of hypoxia in intestinal tissues (11–14). Tissue hypoxia can also be determined in vivo by looking at biochemical markers at the cellular level including decreased adenosine triphosphate (ATP) and increased nicotinamide adenine dinucleotide (NADH) (15). In hypoxia, hypoxia-inducible factor (HIF) up-regulates the transcription of various cytokines, including erythropoietin and vascular endothelial growth factor (VEGF) and while these have been proposed as markers of hypoxia and gut injury (16, 17) they are difficult to measure and not used in routine in practice. Table 1 summarises the tissue biomarkers of hypoxia and inflammation which have been proposed as predictors of gut ischaemia and injury (18–26), including their clinical use, advantages, and disadvantages, as well as the evidence for their use and any current clinical applications.
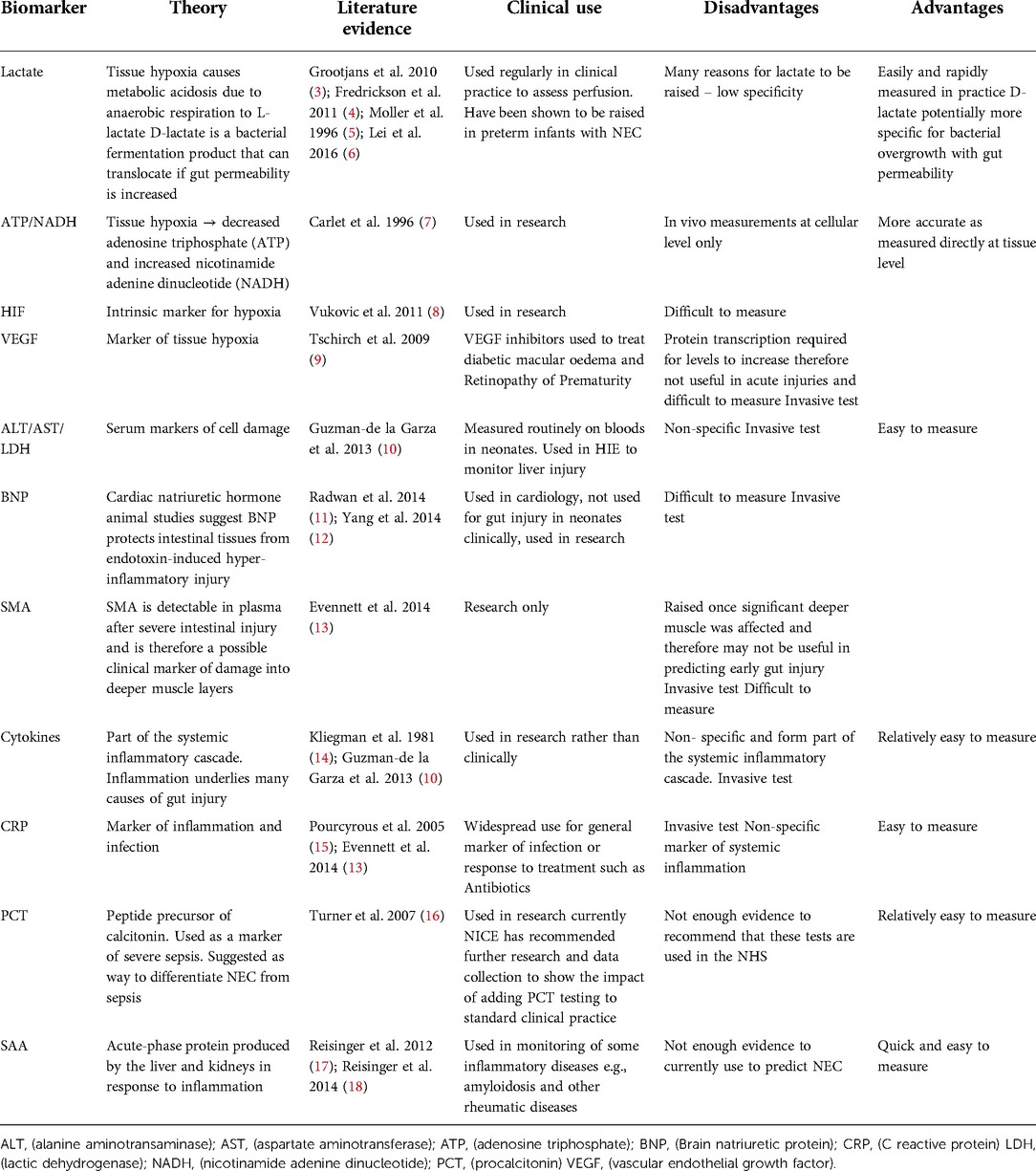
Table 1. Summary of the serological markers of ischaemia, hypoxia and inflammation which have been reported as potential biomarkers of gut inflammation/injury.
Inflammatory mediators as biomarkers of gut injury
Cytokines and cell surface receptors
Cytokines have an important modulatory role in intestinal inflammation and gut injury (22). Interleukins (IL) 1, 3, 6 and 8, tumour necrosis factor alpha (TNFα), platelet activating factor (PAF), expression of toll like receptor 4 (TLR 4) on cell surface have been linked with development of NEC. However, the inflammatory mediators are non-specific and form part of the systemic inflammatory cascade. Therefore their role as diagnostic tools in NEC is limited, particularly as the commonest differential diagnosis of NEC is septic ileus (18).
C-reactive protein (CRP)
The most used nonspecific biomarker is CRP, but the specificity is low, and it is not possible to differentiate NEC with sepsis, or other causes of inflammation, based on CRP alone. Pourcyrous et al. (23) evaluated the association between serum CRP and NEC. In their study fifty-five infants with stages II and III NEC had an abnormal CRP (out of 241 evaluated for GI symptoms), regardless of their blood culture results and this normalised within an average of 9 days unless complications developed. As suggested by the authors, this could advise treatment strategies in infants with suspected NEC in that if they have serial normal CRP values, antibiotics could be stopped, and feeds restarted. CRP becomes abnormal in stage 2 and 3 NEC meaning a raised CRP would be an indicator for treatment and a persistently raised CRP, despite adequate treatment, could suggest complications are developing. However its utility as a biomarker to diagnose NEC is limited because there could be several reasons for a raised CRP and Evennett et al. (27) found that although CRP is a sensitive marker for NEC, it is non–specific. Another limitation of using CRP as a biomarker for NEC is the lag of about 12–24 hours for a rise in CRP to occur after the onset of NEC. However, as it is a marker of active inflammation it does have a role in assessing the response to treatment and this is how CRP is used routinely in neonatal clinical practice currently.
Procalcitonin (PCT)
PCT is a peptide precursor of calcitonin, synthesised by the parafollicular C cells of the thyroid and involved in calcium homeostasis. PCT levels have been shown to rise within 3 hours in response to invasive infection (28). Turner et al. (24) reported low PCT levels during NEC in preterm infants; PCT levels were <1 ng/ml at presentation and <1.3 ng/ml thereafter in neonates with stage I and II NEC in comparison with 4.1 ng/ml in neonates with sepsis. It has been proposed as a potential biomarker to differentiate NEC from sepsis. Although there is not enough data for this to be implemented into routine practice, some centres are exploring its use in diagnosing sepsis through research studies.
Serum amyloid A (SAA)
Serum Amyloid A (SAA) is an acute-phase protein produced by the liver and kidneys in response to inflammation. Several studies have examined SAA levels and its association with NEC and suggested that it may be a potential marker for diagnosing severe or complicated NEC, especially if combined with other markers. Reisinger et al. (26) reported that levels of urinary SAA were significantly higher in complicated NEC; they demonstrated an optimised cut-off value of SAA of 40.7 ng/ml for the stage II and stage III NEC groups by Bells' modified criteria and a cut-off value between surgical and medical NEC was determined at 34.4 ng/ml with a sensitivity of 83% and specificity of 83%. This same group also reported that combining SAA levels with platelet count could improve sensitivity of identifying complicated or surgical NEC (94% sensitivity and 83% specificity). This suggests a potential role of SAA in diagnosing severe NEC, especially when combined with blood platelet counts. Given that mortality is higher with severe NEC or NEC requiring surgery, the ability to predict severe NEC to allow earlier and more aggressive therapeutic interventions would be important. However, SAA would need further validation before it could be used in clinical practice.
Combinations of inflammatory markers
Cetinkaya et al. (29) measured SAA, PCT and CRP in diagnosis and follow up in 152 preterm infants with NEC. They demonstrated that PCT had the highest specificity (98%) and PPV (97%), but lowest sensitivity (92%) and NPV (94%) of the 3 markers. Elfarargy et al. (30) conducted a case control study of 20 healthy neonates and 20 with NEC and reported that there was an increase in faecal calprotectin and serum levels of CRP, PCT and epithelial neutrophil activating peptide-78 (ENA-78), in the NEC group in comparison to the control group. These studies add weight to the suggestion that we should be using a combination of multiple different biomarkers to help identify NEC earlier.
More specific biochemical markers of gut tissue injury
The non-specificity of the above markers means they are unable to differentiate between sepsis, NEC, and other causes of gut inflammation. This has propelled researchers to focus on more specific gut tissue biomarkers. Table 2 summarises the gut tissue biomarkers proposed as possible predictors of NEC (25, 31–62) and we discuss their overall effectiveness below. Some of these are also affected by inflammation such as volatile organic compounds and calprotectin but are felt to be more specific than the inflammatory mediators above which is why we will discuss these biomarkers in this section.
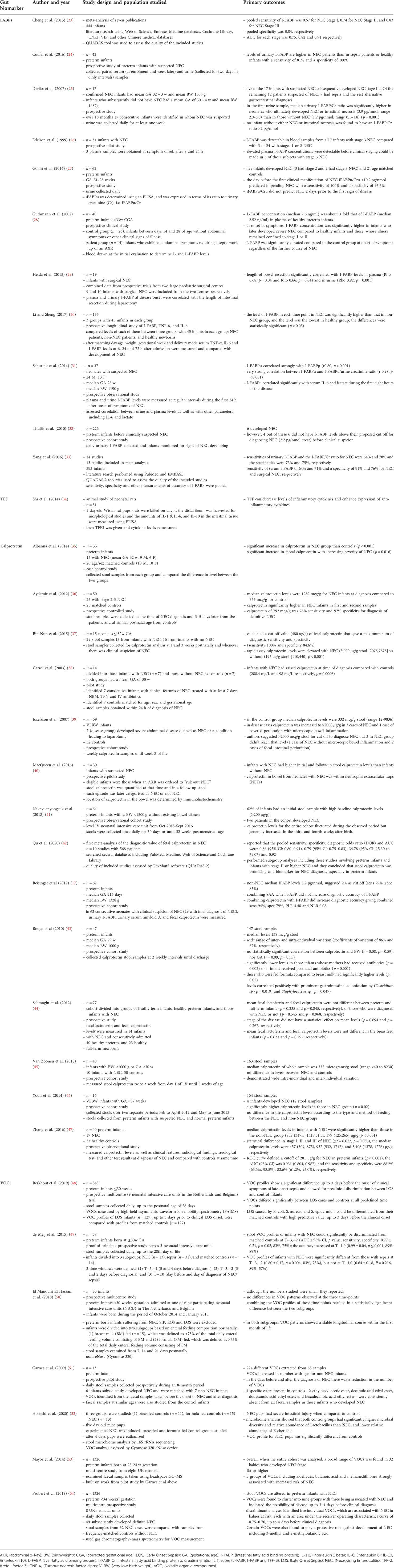
Table 2. Summary of the main studies reporting on gut tissue biomarkers (FABPs, calprotectin, VOC and TFF) as predictors of necrotising enterocolitis (NEC).
Fatty acid binding proteins (FABPs)
Intestinal FABP (I-FABP) and liver FABP (L-FABP) are both found in intestinal mucosa; I-FABP is relatively specific for enterocytes whereas L-FABP is present in many tissues. Both are released when cell membrane integrity is damaged. They are tissue specific inflammatory markers elevated during ischaemia, acting as markers of enterocyte and/or hepatocyte death, that can be measured in serum or urine by enzyme-linked immunosorbent assay (ELISA) (63–65).
FABPs effectiveness at diagnosing NEC
FABPs have been one of the most widely studied biomarkers for NEC. Table 2 lists the main studies and several have demonstrated raised I-FABP concentrations in neonates with NEC (32, 34, 36, 38) correlating with onset and resolution of symptoms (66). FABPs are felt to be more promising for diagnosing NEC as they are only released with specific gut damage rather than with generic neonatal sepsis (67). In further support of its use as gut biomarker, I-FABP has been shown to correlate significantly with serum IL-6 and lactate during the first 8 h of the disease, which are associated with development of NEC (39). However, there are concerns that they would not be able to predict the onset of NEC prior to clinical symptoms. Thuijls et al. (40) study of 226 neonates before clinical suspicion of NEC supported the evidence that urinary I-FABP levels are not suitable as a screening tool for NEC before the onset of clinical symptoms raising the suspicion for NEC. Although Gollin et al. reported that elevated urinary I-FABP was a sensitive and specific predictive biomarker for NEC one day prior to the development of clinical manifestations, it could not predict NEC two days prior to the first clinical signs developing (35), meaning there is limited lead time in terms of warning of NEC onset.
FABPs effectiveness at predicting disease severity
However, they are promising in terms of levels predicting disease severity. Even with a small number of infants, several studies have reported significantly higher I-FABP values related to severity of NEC (33, 34, 36, 40, 66). Guthmann et al. (36) reported that at the onset of symptoms I-FABP concentration was significantly higher in infants who later developed severe NEC and Heida et al. (37) demonstrated that the length of bowel resection in infants with surgical NEC was correlated with I-FABP levels in both plasma (p = 0.04) and urine (p = 0.001), supporting the hypothesis that increased I-FABP levels correspond with the extent of necrotic tissue.
Using urinary or serum FABPs
Although serum I-FABP levels have been shown to be increased in infants with NEC, blood is never an ideal specimen for surveillance purposes, especially in preterm infants, because blood-taking procedures are painful and invasive. Plasma I-FABP concentrations correlate strongly with urine I-FABP levels meaning either urine or blood levels can be used but, in neonates, non-invasive urine specimens would be more preferable (39). Thuijls et al. (40) proposed that urinary I-FABP is a better biomarker than plasma I-FABP for diagnosing NEC because FABPs have a short plasma half-life and are readily excreted in urine. Total bowel necrosis could also prevent the release of I-FABP into the circulation giving a negative plasma test, whereas urinary I-FABP reflects the accumulation of I-FABP over time and has a higher chance of achieving positive urinary test results. Conversely, some infants with NEC are anuric in which case blood sampling might be necessary.
Potential pitfalls in using FABPs as a biomarker for NEC
A potential problem with I-FABP for diagnosis of gut injury in NEC is that, although NEC frequently affects the ileum and jejunum, it can be localised to the colon, or rarely, the stomach, in which case an increase in I-FABP might not occur, as expression is lower in these regions; > 90% of all I-FABP expression is in the ileum and jejunum (33). Furthermore, there are limited studies reporting normal ranges of FABP in neonates, especially in preterm infants and particularly in those born at 22–23 weeks gestation who are at the greatest risk of developing NEC. This therefore limits their use in predicting NEC as the cut off value which equates to NEC has not been conclusively identified, although some authors have suggested various cut off levels (25). Most available studies involve control infants without NEC and these infants' FABP levels can be reviewed to compare normal ranges. Shores et al. (68) examined 112 infants (24–40 weeks gestational age) who had specimens in the first week of life, but only 19 premature infants (24–29 weeks gestational age) who had longitudinal specimens. They reported that infants had low levels of I-FABP during the first week of life, independent of gestational age, and levels increased longitudinally in premature infants. However, this is a very small study to base any conclusions on and much larger studies are needed to establish both the normative data for infants and the cut-off levels for diagnosing NEC.
Some studies have measured the ratio between I-FABP levels in the urine (I-FABPu) and the urine creatinine to compensate for variation in urine concentration and some did not (25, 40, 69). However, few have determined whether it is necessary to do this; Schurink et al. (39) in their study of 37 neonates with a median gestational age of 28 weeks found a strong correlation between I-FABP measured in plasma and urine (r0.80, p < 0.001) and a very strong correlation between I-FABPu and I-FABPu/urine creatinine ratio (r 0.98, p < 0.001) suggesting that calculating urinary IFABP/creatinine ratio may not be needed. Another factor to consider when comparing published levels of I-FABP in neonates is that different ELISA kits have been used by different studies and, therefore, it is difficult to compare, especially as there is no standardised I-FABP preparations. In addition, I-FABP is currently only available as a research test and might not be suitable for rapid result availability.
Summary
A recent meta-analysis by Yang et al. (41) reported that I-FABP levels in plasma and urine have a high specificity (91% and 73% respectively), but moderate sensitivity (64% and 64% respectively), which limits their value as a NEC biomarker. Cheng et al. in 2015 (31) completed a meta-analysis on serum I-FABP and found that it had moderate accuracy for diagnosing NEC with a pooled sensitivity of 0.67 for NEC Stage I, 0.74 for NEC Stage II, and 0.83 for NEC Stage III. Both analyses showed that serum I-FABP has a high specificity for the diagnosis of NEC, but it is limited by its sensitivity, hindering it as a gut biomarker in preterm infants.
Combining I-FABPs with other biomarkers
A recent review suggested that the diagnostic value of I-FABP can be improved by combining with other markers of intestinal injury (70) which adds weight to the theory that the best biomarker for NEC may well be a composite measure of many biomarkers in an algorithm. L-FABP, I-FABP and TFF3 are found in significantly higher levels in infants who develop confirmed NEC compared with those who do not (18, 40, 66, 67). Ng et al. (67) developed the LIT score which is the sum of combining the plasma level of each of these biomarkers – L-FABP, I-FABP and TFF3. Each biomarker is ranked 0–3 based on 3 cut offs to give a total LIT score of between 0 and 9. They reported significantly higher plasma levels of L-FABP, I-FABP and TFF3 and LIT score in patients with surgical NEC compared to nonsurgical cases and a significant association between these gut barrier biomarkers and mortality rate in the NEC group. With a median cut-off of 4.5 points, the LIT score identified surgical NEC with sensitivity and specificity of 83% and 100%, respectively.
FABPs have also been shown to correlate significantly with non-specific biomarkers of inflammation including IL-6 (39) and IL-8 (71). Recently, combining with non-invasive markers including Near Infrared Spectroscopy (NIRS) has shown promise. Kalteren et al. (72) showed that blood transfusions in preterm infants are associated with concomitant signs of oxidative stress and intestinal injury as measured by raised levels of urinary 8-isoprostane and I-FABP respectively, in parallel with lower variability in splanchnic oxygenation and one could surmise that combing these markers could help predict NEC with greater reliability and certainty.
Trefoil factors (TFF)
Trefoil factors 1–3 (TFF 1–3) are a family of polypeptides upregulated in response to gut mucosal injury which have a fundamental role in epithelial protection and repair. In NEC there is a down regulation of TFF3 expression leading to impaired mucosal regeneration (33, 36, 40, 66). Levels of TFF3 in plasma have been reported to be elevated in paediatric patients with sepsis (73), and in a hypoxia induced neonatal rat NEC model, TFF decreased inflammatory cytokines and enhanced expression of anti-inflammatory cytokines (42). This suggests those with lower levels of TFF would be at increased risk of developing NEC, but this is difficult and complex to measure and TFF have not been demonstrated to predict NEC onset in human studies.
Stool volatile organic compounds (VOC)
Faecal VOCs, products of microbial metabolism in the gut, are proposed as a gut tissue biomarker of gut injury and NEC (56, 57, 59, 74) and VOCs have demonstrated potential as non-invasive early diagnostic biomarkers in other gastrointestinal diseases which have a common feature with NEC in that the intestinal microbiome is felt to play a part in the pathogenesis, including neonatal sepsis (56), inflammatory bowel disease (IBD) (75), and colorectal cancer (76).
Garner at al (59). reported that VOCs increase in number as the neonate matures, likely reflecting the growing diversity of intestinal flora. Faecal VOC profiles of infants with NEC significantly differ from controls and were reported to be present from 2 to 4 days predating the onset of clinical symptoms and therefore, have been postulated to be a potential biomarker of pre-clinical NEC (57, 59). However as de-Meij (57) highlighted, there is overlap in the VOCs between NEC and sepsis; infant profiles were not always distinguishable. Furthermore, studies are small as very few recruited neonates developed NEC (Table 2). El Manouni El Hassani et al. (58) conducted a prospective multicentre study of preterm infants <30 weeks' gestation and found no difference in VOC levels between infants with NEC and sepsis.
Probert et al. (62) recently conducted a multicentre prospective study looking at VOCs as potential markers for NEC in infants <34w gestation. They reported faecal VOCs were altered in preterm infants and could indicate the possibility of disease 3–4 days before onset of clinical symptoms. Although this was the largest prospective study to date it still is insufficient to recommend routine VOC measurement to predict NEC. One major limitation is that different techniques have been used when studying VOCs and there is no guideline for the exact bioanalytical method, although headspace gas chromatography-mass spectrometry is thought to be the gold standard for quantitative analysis of VOCs. El Manouni el Hassani et al. (77) recently proposed a protocol for VOC analysis which would potentially allow for a standardised analysis of VOCs which could only serve to improve their use in clinical practice.
However there remain many challenges to overcome before implementing routine point of care testing of VOC for clinical diagnoses. The patients included in the human studies of VOC (Table 2) were of different gestational and chronological ages, located at different hospitals, and were on different enteral feeds, all of which are known to affect VOC measurement (60). El Manouni El Hassani et al. (77) demonstrated that there is a significant difference in VOC profiles over the first 21 days of life in the 15 infants <30w gestational age that they studied which would limit the diagnostic use of the eNOSE, as this device does not identify VOCs present, but instead gives an overview of the total number and mixture of VOC present in a sample (78). El-Metwally (79) demonstrated other confounding factors including differential environmental exposures or therapeutic interventions, which are commonplace in preterm infants who have varying antibiotic courses and incubator humidity settings, which will vary with preterm infant gestation, can affect VOC levels (80). Course et al. (78) in their systematic review examine in detail other issues with using VOC such as the measurement repeatability in neonates. One further potential limitation to routine VOC analysis by headspace gas chromatography-mass spectrometry is the specialised equipment necessary.
Stool calprotectin
Intestinal inflammation is characterised by the sequestration of neutrophils into the gut wall. Calprotectin is a neutrophil derived protein present in stool that is promising as a non-specific biomarker of gut injury because it is resistant to degradation and is stable in stool kept at room temperature (81). Table 2 reviews the studies that have examined the use of calprotectin in detecting NEC; the majority are of a small sample size and there is debate regarding its usefulness; some authors have reported raised calprotectin levels in infants whom have NEC (43, 44, 46, 48, 54, 55, 82), compared to well preterm infants, but others have reported no difference (52, 53).
Although calprotectin is in clinical use in some gastroenterological conditions, e.g., inflammatory bowel disease (83, 84), measuring calprotectin levels in NEC has no current practical clinical use, although a point-of-care test is available. Two recent studies have also questioned the utility of calprotectin in NEC, one of them using a point of care test (49, 53). In each of these, calprotectin was measured serially over time in preterm infants, and it was reported that calprotectin levels were much higher in preterm infants than in adults and that there are significant inter- and intra-individual variations in preterm infants during the first few weeks of life, limiting its utility in differentiating infants with NEC from those without. Furthermore, often neonates with NEC do not pass stool and this would limit the use of any stool biomarker in predicting NEC. More data is currently needed to determine whether a rise in calprotectin levels could predict NEC before clinical symptoms arise, and to decide on what cut off value should be used as various values have been proposed (Table 2). Interestingly, the previous cut off values for diagnosis of NEC that have been proposed range from 200 μg/g by Carroll et al. (46) to 2000 μg/g by Joseffson et al. (47). but in Van Zoonen's (53) study, all of these cut offs were within the range of concentrations observed in their control infants.
Pergialiotis et al. (85) conducted a systematic review of stool calprotectin levels as a non-invasive marker for NEC. This review included 13 studies with a total of 601 neonates and showed that the sensitivity and specificity of stool calprotectin as a diagnostic marker were 76%–100% and 39%–96.4%, respectively. Given it is a non-specific marker, combining it with other biomarkers is likely to increase its specificity, but as to what combination of biomarkers would be best is still unknown. Qu et al. (50) recently conducted a meta-analysis of the diagnostic value of fetal calprotectin in NEC looking at a total of 10 studies with 568 patients and reported that stool calprotectin was promising as a biomarker for NEC diagnosis, especially in preterm infants.
More longitudinal data in infants with NEC are necessary to determine whether acute rises in stool calprotectin levels before the onset of clinical symptoms can be confirmed as a diagnostic biomarker, either in isolation, or combined with other markers. However, it is unlikely that calprotectin could ever be used to predict NEC onset because it only rises in inflammation and once inflammation has occurred the NEC disease process has already started.
New urinary biomarkers
In urine, with the aid of liquid chromatography-mass spectrometry (LCMS), other biomarkers have been identified and validated (fibrinogen peptides: FGA1826, FGA1883, and FGA2659) and Sylvester et al. (86) demonstrated that they could discriminate surgical NEC from nonsurgical NEC with these peptides and perhaps more importantly they also demonstrated in their study of 555 infants suspected of having NEC that the integration of clinical parameters with urine biomarkers in an ensemble model resulted in the correct prediction of NEC outcomes in all cases tested. However, LCMS is expensive and time consuming and as such currently has no place in routine clinical practice, but this evidence supports the idea that combining multiple biomarkers with clinical features into an algorithm may well be the future of predicting NEC.
Prostaglandin E2 Major Urinary Metabolite (PGE-MUM) has been described as a potential urine biomarker and is advantageous as it is stable in urine. Previous studies have demonstrated that it is raised in intestinal mucosal inflammation and reflects ulcerative colitis severity in paediatric patients (87). Konishi et al. recently examined its effectiveness as a surrogate marker for NEC and reported that PGE-MUM levels were higher in those with NEC, and correlated with improved status of NEC, length of necrotic intestine, and Bell's staging criteria. This is promising as both a diagnostic biomarker and a biomarker of severity, especially as urine markers would be preferred as they are non-invasive and more reliable than stool which is affected by the presence of an ileus for example. However, PG-MUM is non-specific as metabolites of cyclooxygenase are upregulated in all inflammatory conditions and levels could be affected by chronic lung disease (88). This was also the first study to report PGE-MUM levels in NEC and was of a small study size, therefore before any conclusions can be made larger studies are needed.
Various urine proteins have been examined as candidates for possible biomarkers of NEC. Recently a panel of 7 such urine proteins was identified by LCMS and subsequently validated by enzyme-linked immunosorbent assay in a study of 119 preterm infants (85 NEC, 17 sepsis, 17 control) (89). The 7 urine protein studied were alpha-2-macroglobulin-like protein 1 (A2ML1), cluster of differentiation protein 14 (CD14), cystatin 3 (CST3), pigment epithelium-derived factor (PEDF), retinol-binding protein 4 (RET4), and vasolin (VASN). Various combinations of these proteins were examined; A2ML1, CD14, CST3, PEDF, RET4, and VASN combined produced an area under the curve (AUC) of 98.4% for distinguishing medical and surgical NEC demonstrating their potential as markers of disease severity with medical NEC being less severe. A panel consisting of CST3, PEDF, and RET4 produced an AUC of 98.2% for distinguishing NEC from sepsis, which is the main differential for NEC given they present quite similarly in the initial stages in preterm infants. This study also adds more weight to the theory that combining several biomarkers is likely to improve their effectiveness at diagnosing NEC itself or predicting disease severity.
Other markers focusing on intestinal wall barrier and permeability
Bischoff et al. explained the importance of the intestinal barrier (2) and recently there has been a move to look at the assessment of intestinal barrier function and permeability in humans by using intestinal permeability assays, and the assessment of biomarkers of epithelial integrity, including soluble adhesion molecules and Claudins. Claudins are tight junction proteins which are expressed in intestinal cells and therefore raised levels could imply intestinal epithelial cell damage. Only two studies have examined Claudins, and they involve a very small number of infants. Both studies found decreased claudin−2 expression in the intestinal tissue of infants with NEC (90) and a spike of increased excretion of claudin 3 in the urine (40). Thuijls et al. (40) examined urinary claudin-3 with urinary I-FABP and faecal calprotectin (FC) in 35 neonates suspected of having NEC and reported that median I-FABP, claudin-3 and FC levels were significantly higher in neonates with NEC than in neonates with other diagnosis. Blackwood et al. (90) examined urinary claudin-2 in six neonates and demonstrated raised urinary claudin-2 protein in those with NEC compared to those neonates without NEC (p < 0.001). However, these studies are too small to draw any conclusions regarding the effectiveness meaning there is currently not enough evidence to support Claudins as a biomarker of NEC as much larger studies are needed.
Citrulline is an amino acid not incorporated into proteins but produced by small intestinal enterocytes from glutamine and has been proposed as a marker of functional enterocyte mass. Loss of small bowel epithelial cell mass results in impaired intestinal permeability which is detected by reduced plasma citrulline levels. It has been demonstrated as a marker for chemotherapy-induced mucosal barrier injury in paediatric patients (91) but is not currently used routinely.
New and upcoming biomarkers – the omics era
With increasing advances in technology there is a new era of using metabolomics and proteomics to look for new biomarkers for NEC (92–94) and gene polymorphisms are also being investigated with several being identified as being associated with NEC or NEC severity (95–97).
Agakidou et al. (98) have already reviewed the data on prediction, diagnosis and prognosis of NEC using a metabolomics and proteomics approach and this data is limited. Metabolomics detects the direct result of a biochemical response to a stimulus and metabolites are the final products of gene transcription. One benefit of such an approach is that numerous samples can be used including urine and plasma/serum, but also other bodily fluids. Numerous metabolomics have been examined in experimental models of NEC and these are suggested to be used as potential biomarkers for NEC. Agakidou et al. (98) reviewed the literature evidence for these in their article and although there is evidence of altered metabolomics in infants with NEC, these are mostly involving only small studies and much larger studies are needed before any conclusions can be accurately drawn. Furthermore, accurate identification of metabolites requires confirmation with known standards which also limits their current use in clinical practice.
Genomics is the study of all genes in an organism and there is some data suggesting that there is a genetic predisposition to NEC. This has led to the study of the preterm infant genome looking at specific genes encoding factors known to be involved in the pathogenesis of NEC including variants in the genes encoding nucleotide binding and oligomerization domain like receptors, autophagy-related protein, angiotensinogen, IL8, low-molecular-weight kininogen protein, (KNG1) Acetyl-CoA Carboxylase Beta, and CAT- Catalase, genes regulating the pathways of the receptors TLR2, TLR4, and TREM1 (96, 97, 99–101), as well as rs1048719 polymorphism in the intron region of the GM2 activator (GM2A) gene, the rs2075783 polymorphism in the exon 1 region (95) and SIGIRR variants (97). However currently the accuracy of genomic biomarkers in diagnosing NEC has not been examined; it is not known whether these associations would have any clinical use and there is the disadvantage of the time taken for the results of any genetic polymorphism tests which may preclude them from being a biomarker for diagnosing NEC early. However, they could be used to test infants at birth to help in predicting those who are at higher risk of NEC so that for example higher risk feeding strategies could be implemented. In addition, assays for genetic markers are expensive, time consuming and not currently available for clinical use. It is well known that the pathogenesis of NEC is multifactorial, and a lot of the proposed genetic markers above could also reflect secondary inflammatory responses, meaning they may not be specific markers as these inflammatory markers are common to other pathologies which may mimic NEC clinically including its main differential, septic ileus. It is not known to what extent environmental factors could modify the impact of these gene polymorphisms which will also hinder their effectiveness as biomarkers of NEC.
Transcriptomics is the study of the transcriptome, i.e., the study of the mRNA within a cell or organism. Experimental and clinical studies as well as a meta-analysis on long-non-coding RNA (lncRNA), micro RNA (miRNA), and mRNA profiles in NEC demonstrated a role of lncRNA, miRNA, and mRNA in NEC (102–106), but further evaluation in larger studies is needed. In a very recent study Pan et al. (107) completed a retrospective analysis on preterm pigs with NEC and found 344 differentially expressed genes between those piglets with NEC and control piglets and the authors suggested that blood gene expression analysis could be used to help identify early biomarkers of NEC, but clearly more research is needed and most importantly we need to see if this evidence in animal studies translates into human studies.
Clinical prediction models
In addition, clinical factors have been explored such as heart rate variability (HRV) which reflects autonomic nervous system and tone; studies have shown that changes in HRV precede clinical symptoms of NEC by 2 days and the pattern of HRV change was also significantly associated with the clinical severity of NEC (stage II vs. stage III) (108). Doheny et al. conducted a prospective study in premature infants and found that low vagal tone (reflected by low HRV) in the first week of life in premature neonates may be a contributor for predicting the subsequent onset of NEC (109). However, HRV may be affected by many other conditions and so could not be used in isolation.
In 2015 Niemarkt et al. (9) reviewed the pathogenesis, diagnosis, and treatment of NEC and in this review discussed different clinical prediction models and biomarkers for NEC. Moss et al. (110) in their multicentre prospective, observational study examined clinical factors to see if any could predict severe NEC. Although they were able to identify 12 factors which were related to progressive NEC, they could not develop a clinical model to predict progression of NEC. Ji et al. (111) used a large multicentre prospective study to establish a clinical database and use machine learning taking into account clinical and laboratory results at the time of clinical presentation to create two NEC prediction models: the first to provide an automated diagnostic classification scheme and the second for risk-stratifying patients into low, intermediate and high NEC scores to determine the risk for disease progression and need of surgical intervention. If we could add biomarker data into such a model, then one would hope we could improve our diagnostic/predictive accuracy. Sylvester et al. (86) in their study looked at 27 clinical parameters and created a multivariate predictor of NEC progression. They reported that using clinical parameters alone resulted in a receiver-operator characteristic (ROC) curve with an area under the curve of 0.817 and left 40.1% of all patients in an “indeterminate” risk group. By adding in data from three urine peptide biomarkers (fibrinogen peptides: FGA1826, FGA1883 and FGA2659) they improved this ROC area under the curve to 0.856 and by combining clinical parameters with urine biomarkers their model correctly predicted NEC outcomes in all the cases the authors tested.
Novel application of future biomarkers
Although there has been a plethora of studies examining biomarkers for detecting NEC the perfect biomarker has remained elusive and the current available data to support their use routinely is insufficient (10). It has however been demonstrated that the combination of clinical parameters with biomarker analysis may significantly improve our ability to identify individuals at risk of developing NEC and that combining markers themselves will improve diagnostic sensitivity and specificity. Identifying those individuals at higher risk early after birth for example with using the suggested dried blood analysis of gene polymorphisms will give time to allow for therapeutic interventions which could reduce the risk of NEC developing, therefore further work is needed in this area.
In addition, there are newer biomarkers being suggested which might more accurately reflect intestinal injury such as Near Infrared Spectroscopy (NIRS) (112–117) and USS doppler studies (118) of the splanchnic oxygenation and blood flow respectively. These alternative non-invasive modalities could facilitate earlier detection of the onset of NEC; however, they also have their own limitations. Future work needs to combine the existing most promising biomarkers including FABP and newly identified ones which have been shown to predict NEC in small studies, such as urine proteins. Only with combining multiple biomarkers from multiple modalities ranging from NIRS, urine, and stool biomarkers, as well as clinical parameters, we may be able to finally make progress in detecting NEC earlier to allow for prompt intervention. Future research needs to focus on these novel markers being combined with those that are more established and aim to develop a practical point of care test that could be used easily and quickly in clinical practice.
Conclusion
Despite advances in neonatal care, the morbidity and mortality of NEC remain high; research must continue to look for ways to identify NEC sooner. Researchers have explored various tissue biomarkers to predict, diagnose and prognosticate NEC in newborn infants. Inflammatory mediators such as cytokines, CRP, PCT, SAA and combinations of these inflammatory markers have been investigated extensively but they are not useful in predicting or diagnosing NEC. However, CRP is used in clinical practice to measure the response to treatment of NEC.
Blood and urine FABPs, stool VOC and calprotectin are well known and studied gut tissue injury biomarkers. Of all the biomarkers evaluated for both predicting and diagnosing NEC the most promising are felt to be FABPs, however, their diagnostic accuracy is relatively low, most studies involve small numbers and are not randomised control trials. Researchers are investigating the usefulness of new biomarkers including urinary fibrinogen peptides and proteins, intestinal barrier function and permeability by using intestinal permeability assays, and the assessment of epithelial integrity including soluble adhesion molecules and Claudin. Further research on metabolomics and proteomics, and gene polymorphisms in predicting and diagnosing NEC is also needed to see if these could allow detection of those infants at the highest risk of developing NEC after birth. The ideal would be that these infants could then undergo greater surveillance for the possible onset of NEC and then subsequently combining this with the examination of multiple gut biomarkers aiming to detect the onset of NEC prior to its clinical manifestations.
Although no single biomarker has been identified, perhaps a combination of biomarkers would help. Further research is needed to see if we can develop a machine learning prediction and diagnostic model by inputting all the known biomarkers, combined with known clinical parameters suggestive of NEC to accurately predict NEC, which then improves outcomes. Using biomarkers in this way could facilitate the era of individualised medicine that we are approaching and mean that not only would be able to identify those at-risk neonates, but we could target them to receive maximal preventative therapies including utilising higher risk feeding regimens. Ultimately being able to identify NEC before clinical onset, would allow the opportunity for earlier intervention and the potential for improved clinical outcomes. Significantly improving the morbidity and mortality associated with NEC has so far proved elusive despite our best efforts, and the neonatal community must continue to strive to achieve this.
Author contributions
CH, JB and NA conceptualised the review article CH wrote the first draft of the manuscript. All authors contributed to manuscript revision. All authors contributed to the article and approved the submitted version.
Conflict of interest
The authors declare that the research was conducted in the absence of any commercial or financial relationships that could be construed as a potential conflict of interest.
Publisher's note
All claims expressed in this article are solely those of the authors and do not necessarily represent those of their affiliated organizations, or those of the publisher, the editors and the reviewers. Any product that may be evaluated in this article, or claim that may be made by its manufacturer, is not guaranteed or endorsed by the publisher.
References
1. Costeloe K, Hardy P, Juszczak E, Wilks M, Millar MR. Probiotics in preterm infants study collaborative G. Bifidobacteriu, breve BBG-001 in very preterm infants: a randomised controlled phase 3 trial. Lancet. (2016) 387:649–60. doi: 10.1016/S0140-6736(15)01027-2
2. Bischoff SC, Barbara G, Buurman W, Ockhuizen T, Schulzke JD, Serino M, et al. Intestinal permeability –a new target for disease prevention and therapy. BMC Gastroenterol. (2014) 14:189. doi: 10.1186/s12876-014-0189-7
3. Scheeren TW, Schober P, Schwarte LA. Monitoring tissue oxygenation by near infrared spectroscopy (NIRS): background and current applications. J Clin Monit Compu. (2012) 26:279–87. doi: 10.1007/s10877-012-9348-y
4. Garg BD, Sharma D, Bansal A. Biomarkers of necrotizing enterocolitis: a review of literature. J Matern Fetal Neonatal Med. (2018) 31(22):3051–64. doi: 10.1080/14767058.2017.1361925
5. Ng PC. An update on biomarkers of necrotizing enterocolitis. Seminars in Fetal and Neonatal Med. (2018) 23:380–6. doi: 10.1016/j.siny.2018.07.006
6. Wang K, Tao G, Sun Z, Sylvester KG. Recent potential noninvasive biomarkers in necrotizing enterocolitis. Gastroenterol Res Pract. (2019) 2019:8413698. doi: 10.1155/2019/8413698
7. Gunasekaran A, Devette C, Levin S, Chaaban H. Biomarkers of necrotizing enterocolitis: the search continues. Clin Perinatol. (2022) 49(1):181–94. doi: 10.1016/j.clp.2021.11.011
8. Ng PC. Biomarkers of necrotising enterocolitis. Semin Fetal Neonatal Med. (2014) 19:33–8. doi: 10.1016/j.siny.2013.09.002
9. Niemarkt HJ, de Meij TG, van de Velde ME, van der Schee MP, van Goudoever JB, Kramer BW, et al. Necrotizing enterocolitis: a clinical review on diagnostic biomarkers and the role of the intestinal microbiota. Inflamm Bowel Dis. (2015) 21(2):436–44. doi: 10.1097/MIB.0000000000000184
10. Terrin G, Stronati L, Cucchiara S, de Curtis M. Serum markers of necrotizing enterocolitis: a systematic review. J Pediatr Gastroenterol Nutr. (2017) 65(6):e120–32. doi: 10.1097/MPG.0000000000001588
11. Grootjans J, Thuijls G, Verdam F, Derikx JP, Lenaerts K, Buurman WA. Non-invasive assessment of barrier integrity and function of the human gut. World J Gastrointest Surg. (2010) 2:61–9. doi: 10.4240/wjgs.v2.i3.61
12. Fredrickson LK, Bell EF, Cress GA, Johnson KJ, Zimmerman MB, Mahoney LT, et al. Acute physiological effects of packed red blood cell transfusion in preterm infants with different degrees of anaemia. Arch Dis Child Fetal Neonatal Ed. (2011) 96(4):F249–53. doi: 10.1136/adc.2010.191023
13. Moller JC, Schwarz U, Schaible TF, Artlich A, Tegtmeyer FK, Gortner L. Do cardiac output and serum lactate levels indicate blood transfusion requirements in anemia of prematurity? Intensive Care Med. (1996) 22(5):472–6. doi: 10.1007/BF01712170
14. Lei G, Zhang J, Wang X, Chen M. Plasma D-lactate levels in necrotizing enterocolitis in premature infants. Iran J Pediatr. (2016) 26:e4403. doi: 10.5812/ijp.4403
15. Third European consensus conference in intensive care medicine. Tissue hypoxia: how to detect, how to correct, how to prevent. Societe de reanimation de langue francaise. The American thoracic society. European society of intensive care medicine. Am J Respir Crit Care Med. (1996) 154(5):1573–8. doi: 10.1164/ajrccm.154.5.8912783
16. Vukovic V, Haugland HK, Nicklee T, Morrison AJ, Hedley DW. Hypoxia-inducible factor -1 aplha is an intrinsic marker for hypoxia in cervical cancer xenografts. Am Assoc Cancer Res. (2001) 61(20):7394–8. PMID: 11606368
17. Tschirch E, Weber B, Koehne P, Guthmann F, von Gise A, Wauer RR, et al. Vascular endothelial growth factor as marker for tissue hypoxia and transfusion need in anemic infants: a prospective clinical study. Pediatr. (2009) 123(3):784–90. doi: 10.1542/peds.2007-2304
18. Guzmán-de la, Garza FJ, Ibarra-Hernández JM, Cordero-Pérez P, Villegas-Quintero P, Villarreal-Ovalle CI, et al. Temporal relationship of serum markers and tissue damage during acute intestinal ischemia/reperfusion. Clinics. (2013) 68(7):1034–8. doi: 10.6061/clinics/2013(07)23
19. Radwan H, Selem A, Ghazal K. Value of N-terminal pro brain natriuretic peptide in predicting prognosis and severity of coronary artery disease in acute coronary syndrome. J Saudi Heart Assoc. (2014) 26(4):192–8. doi: 10.1016/j.jsha.2014.04.004
20. Yang H, Song Z, Jin H, Cui Y, Hou M, Gao Y. Protective effect of rhBNP on intestinal injury in the canine models of sepsis. Int Immunopharmacol. (2014) 19(2):262–6. doi: 10.1016/j.intimp.2014.01.023
21. Evennett N, Cerigioni E, Hall NJ, Pierro A, Eaton S. Smooth muscle actin as a novel serologic marker of severe intestinal damage in rat intestinal ischemia-reperfusion and human necrotising enterocolitis. J Surg Res. (2014) 191(2):323–30. doi: 10.1016/j.jss.2014.04.020
22. Kliegman R, Fanaroff A. Neonatal enterocolitis: a nine year experience. Incidence, epidemiology and uncommon observations. Am J Dis Child. (1981) 135:603–67. doi: 10.1001/archpedi.1981.02130310009005
23. Pourcyrous M, Korones SB, Yang W, Boulden TF, Bada HS. C-reactive protein in the diagnosis, management, and prognosis of neonatal necrotizing enterocolitis. Pediatr. (2005) 116(5):1064–9. doi: 10.1542/peds.2004-1806
24. Turner D, Hammerman C, Rudensky B, Schlesinger Y, Wine E, Muise A, et al. Low levels of procalcitonin during episodes of necrotizing enterocolitis. Dig Dis Sci. (2007) 52:2972–6. doi: 10.1007/s10620-007-9763-y
25. Reisinger KW, Van der Zee DC, Brouwers HA, Kramer BW, van Heurn LW, Buurman WA et al. Noninvasive measurement of fecal calprotectin and serum amyloid A combined with intestinal fatty acid-binding protein in necrotizing enterocolitis. J Pediatr Surg. (2012) 47:1640–5. doi: 10.1016/j.jpedsurg.2012.02.027
26. Reisinger KW, Kramer BW, Van der Zee DC, Brouwers HA, Buurman WA, van Heurn E, et al. Non-invasive serum amyloid A (SAA) measurement and plasma platelets for accurate prediction of surgical intervention in severe necrotizing enterocolitis (NEC). PLoS One. (2014) 9(6):e90834. doi: 10.1371/journal.pone.0090834
27. Evennett N, Alexander N, Petrov M, Pierro A, Eaton S. A systematic review of serologic tests in the diagnosis of necrotizing enterocolitis. J Pediatr Surg. (2009) 44(11):2192–201. doi: 10.1016/j.jpedsurg.2009.07.028
28. Van Rossum AM, Wulkan RW, Oudesluys-Murphy AM. Procalcitonin as an early marker of infection in neonates and children. Lancet Infect Dis. (2004) 4(10):620–30. doi: 10.1016/S1473-3099(04)01146-6
29. Cetinkaya M, Ozkan H, Köksal N, Akaci O, Ozgür T. Comparison of the efficacy of serum amyloid A, C-reactive protein, and procalcitonin in the diagnosis and follow-up of necrotizing enterocolitis in premature infants. J Pediatr Surg. (2011) 46(8):1482–9. doi: 10.1016/j.jpedsurg.2011.03.069
30. Elfarargy MS, El Farargy MS, Atef MM, El-Deeb OS, Elsharaby RM, Elhady HAE. Early biomarkers in neonatal necrotizing enterocolitis: a pilot study. J Popul Ther Clin Pharmacol. (2019) 26(3):e1–8. doi: 10.15586/jptcp.v26i3.602
31. Cheng S, Yu J, Zhou M, Tu Y, Lu Q. Serologic intestinal-fatty acid binding protein in necrotizing enterocolitis diagnosis: a meta-analysis. BioMed Res Int. (2015) 2015:156704. doi: 10.1155/2015/156704
32. Coufal S, Kokesova A, Tlaskalova-Hogenova H, Snajdauf J, Rygl M, Kverka M. Urinary intestinal fatty acid-binding protein can distinguish necrotizing enterocolitis from sepsis in early stage of the disease. J Immunol Res. (2016) 2016:5727312. doi: 10.1155/2016/5727312
33. Derikx JP, Evennett NJ, Degraeuwe PL, Mulder TL, van Bijnen AA, van Heurn LW, et al. Urine based detection of intestinal mucosal damage in neonates with suspected necrotising enterocolitis. Gut. (2007) 56:1473–5. doi: 10.1136/gut.2007.128934
34. Edelson MB, Sonnino RE, Bagwell CE, Lieberman JM, Marks WH, Rozycki HJ. Plasma intestinal fatty acid binding protein in neonates with necrotizing enterocolitis: a pilot study. J Pediatr Surg. (1999) 34(10):1453–7. doi: 10.1016/S0022-3468(99)90102-1
35. Gollin G, Stadie D, Mayhew J, Slater L, Asmerom Y, Boskovic D, et al. Early detection of impending necrotizing enterocolitis with urinary intestinal fatty acid-binding protein. Neonatol. (2014) 106(3):195–200. doi: 10.1159/000362497
36. Guthmann F, Börchers T, Wolfrum C, Wustrack T, Bartholomäus S, Spener F. Plasma concentration of intestinal- and liver-FABP in neonates suffering from necrotizing enterocolitis and in healthy preterm neonates. Mol Cell Biochem. (2002) 239(1-2):227–34. doi: 10.1023/A:1020508420058
37. Heida FH, Hulscher JB, Schurink M, Timmer A, Kooi EM, Bos AF, et al. Intestinal fatty acid-binding protein levels in necrotizing enterocolitis correlate with extent of necrotic bowel: results from a multicenter study. J Pediatr Surg. (2015) 50(7):1115–8. doi: 10.1016/j.jpedsurg.2014.11.037
38. Li Z, Sheng Z. Significance of dynamic evolution of TNF-α, IL-6 and intestinal fatty acid-binding protein levels in neonatal necrotizing enterocolitis. Exp Ther Med. (2017) 15(2):1289–92. doi: 10.3892/etm.2017.5532
39. Schurink M, Scholten IG, Kooi EM, Hulzebos CV, Kox RG, Groen H, et al. Intestinal fatty acid-binding protein in neonates with imminent necrotizing enterocolitis. Neonatol. (2014) 106(1):49–54. doi: 10.1159/000358582
40. Thuijls G, Derikx JP, van Wijck K, Zimmermann LJ, Degraeuwe PL, Mulder TL, et al. Non-invasive markers for early diagnosis and determination of the severity of necrotizing enterocolitis. Ann Surg. (2010) 251(6):1174–80. doi: 10.1097/SLA.0b013e3181d778c4
41. Yang G, Wang Y, Jiang X. Diagnostic value of intestinal fatty-acid-binding protein in necrotizing enterocolitis: a systematic review and meta-analysis. Indian J Pediatr. (2016) 83(12-13):1410–9. doi: 10.1007/s12098-016-2144-9
42. Shi L, Zhou PH, Xi JL, Yu HG, Zhang BH. Recombinant human trefoil factor 3 ameliorates bowel injury: its anti-inflammatory effect on experimental necrotizing enterocolitis. Int J Pept. (2014) 2014:634135. doi: 10.1155/2014/634135
43. Albanna EA, Ahmed HS, Awad HA. Stool calprotectin in necrotizing enterocolitis. J Clin Neonatol. (2014) 3(1):16–9. doi: 10.4103/2249-4847.128721
44. Aydemir O, Aydemir C, Sarikabadayi YU, Emre Canpolat F, Erdeve O, Biyikli Z, et al. Fecal calprotectin levels are increased in infants with necrotizing enterocolitis. J Matern Fetal Neonatal Med. (2012) 25:2237–41. doi: 10.3109/14767058.2012.684172
45. Bin-Nun A, Booms C, Sabag N, Mevorach R, Algur N, Hammerman C. Rapid fecal calprotectin (FC) analysis: point of care testing for diagnosing early necrotizing enterocolitis. Am J Perinatol. (2015) 32(4):337–42. doi: 10.1055/s-0034-1384640
46. Carroll D, Corfield A, Spicer R, Cairns P. Faecal calprotectin concentrations and diagnosis of necrotising enterocolitis. Lancet. (2003) 361(9354):310–1. doi: 10.1016/S0140-6736(03)12333-1
47. Josefsson S, Bunn SK, Domellof M. Fecal calprotectin in very low birth weight infants. J Pediatr Gastroenterol Nutr. (2007) 44(4):407–13. doi: 10.1097/MPG.0b013e3180320643
48. MacQueen BC, Christensen RD, Yost CC, Lambert DK, Baer VL, Sheffield MJ, et al. Elevated fecal calprotectin levels during necrotizing enterocolitis are associated with activated neutrophils extruding neutrophil extracellular traps. J Perinatol. (2016) 36(10):862–9. doi: 10.1038/jp.2016.105
49. Nakayuenyongsuk W, Christofferson M, Stevenson DK, Sylvester K, Lee HC, Park KT. Point-of-Care fecal calprotectin monitoring in preterm infants at risk for necrotizing enterocolitis. J Pediatr. (2018) 196:98–103. doi: 10.1016/j.jpeds.2017.12.069
50. Qu Y, Xu W, Han J, Zhou W, Wu H. Diagnostic value of fecal calprotectin in necrotizing enterocolitis: a meta-analysis. Early Hum Dev Vol. (2020) 151:105170. doi: 10.1016/j.earlhumdev.2020.105170
51. Rougé C, Butel M-J, Piloquet H, Ferraris L, Legrand A, Vodovar M, et al.. Fecal calprotectin excretion in preterm infants during the neonatal period. PLoS One. (2010) 5:e11083. doi: 10.1371/journal.pone.0011083
52. Selimoğlu MA, Temel I, Yıldırım Ç, Özyaln F, Aktaş M, Karabiber H. The role of fecal calprotectin and lactoferrin in the diagnosis of necrotizing enterocolitis. Pediatr Crit Care Med. (2012) 13:452–4. doi: 10.1097/PCC.0b013e3182388ae9
53. van Zoonen AGJF, Hulzebos CV, Muller Kobold AC, Kooi EMW, Bos AF, Hulscher JBF. Serial fecal calprotectin in the prediction of necrotizing enterocolitis in preterm neonates. J Pediatr Surg. (2019) 54(3):455-9. doi: 10.1016/j.jpedsurg.2018.04.034
54. Yoon JM, Park JY, Ko KO, Lim JW, Cheon EJ, Kim HJ. Fecal calprotectin concentration in neonatal necrotizing enterocolitis. Korean J Pediatr. (2014) 57(8):351–6. doi: 10.3345/kjp.2014.57.8.351
55. Zhang M, Zhang X, Zhang J. Diagnostic value of fecal calprotectin in preterm infants with necrotizing enterocolitis. Clin Lab. (2016) 62(5):863–9.27349012
56. Berkhout DJC, van Keulen BJ, Niemarkt HJ, Bessem JR, de Boode WP, Cossey V, et al. Late-onset sepsis in preterm infants can be detected preclinically by fecal volatile organic compound analysis: a prospective, multicenter cohort study. Clin Infect Dis. (2019) 68(1):70–7. doi: 10.1093/cid/ciy383
57. de Meij TG, van der Schee MP, Berkhout DJ, van de Velde ME, Jansen AE, Kramer BW, et al. Early detection of necrotizing enterocolitis by fecal volatile organic compounds analysis. J Pediatr. (2015) 167(3):562–7. doi: 10.1016/j.jpeds.2015.05.044
58. El Manouni El, Hassani S, Niemarkt HJ, Said H, Berkhout DJC, van Kaam AH, et al. Fecal volatile organic compounds in preterm infants are influenced by enteral feeding composition. Sensors (Basel). (2018) 18(9):3037. doi: 10.3390/s18093037
59. Garner CE, Ewer AK, Elasouad K, Power F, Greenwood R, Ratcliffe NM, et al. Analysis of faecal volatile organic compounds in preterm infants who develop necrotising enterocolitis: a pilot study. J Pediatr Gastroenterol Nutr. (2009) 49(5):559–65. doi: 10.1097/MPG.0b013e3181a3bfbc
60. Hosfield BD, Pecoraro AR, Baxter NT, Hawkins TB, Markel TA. The assessment of fecal volatile organic compounds in healthy infants: electronic nose device predicts patient demographics and microbial enterotype. J Surg Res. (2020) 254:340–7. doi: 10.1016/j.jss.2020.05.010
61. Mayor A, Reade S, Aggio R, Khalid T, Probert C. PAEDIATRIC FAECAL VOC ANALYSIS: mETHOD OPTIMISATION. Gut. (2014) 63:A218–A218. Supplement: 1 Meeting Abstract: PTH-023. doi: 10.1136/gutjnl-2014-307263.469
62. Probert C, Greenwood R, Mayor A, Hughes D, Aggio R, Jackson RE, et al. Faecal volatile organic compounds in preterm babies at risk of necrotising enterocolitis: the DOVE study. Arch Dis in Childhood - Fetal and Neonatal Ed. (2020) 105(5):474–9. doi: 10.1136/archdischild-2019-318221
63. Kanda T, Fujii H, Tani T, Murakami H, Suda T, Sakai Y. Intestinal fatty acid-binding protein is a useful diagnostic marker for mesenteric infarction in humans. Gastroenterol. (1996) 110(2):339–43. doi: 10.1053/gast.1996.v110.pm8566578
64. Lieberman OM, Sacchettini J, Marks C, Marks WH. Human intestinal fatty acid binding protein: report of an assay with studies in Normal volunteers and intestinal ischemia. Surg. (1997) 121(3):335–42. doi: 10.1016/S0039-6060(97)90363-9
65. Gollin G, Marks C, Marks WH. Intestinal fatty acid binding protein in serum and urine reflects early ischemic injury to the small bowel. Surg. (1993) 113(5):545–51. PMID: 8488474
66. Schurink M, Kooi EM, Hulzebos CV, Kox RG, Groen H, Heineman E, et al. Intestinal fatty acid-binding protein as a diagnostic marker for complicated and uncomplicated necrotizing enterocolitis: a prospective cohort study. PLoS ONE. (2015) 10(3):e0121336. doi: 10.1371/journal.pone.0121336
67. Ng EW, Poon TC, Lam HS, Cheung HM, Ma TP, Chan KY, et al. Gut-associated biomarkers L-FABP, I-FABP, and TFF3 and LIT score for diagnosis of surgical necrotizing enterocolitis in preterm infants. Ann Surg. (2013) 92:776–80. doi: 10.1097/SLA.0b013e318288ea96
68. Shores DR, Fundora J, Go M, Shakeel F, Brooks S, Alaish SM, et al. Normative values for circulating intestinal fatty acid binding protein and calprotectin across gestational ages. BMC Pediatr. (2020) 20(1):250. doi: 10.1186/s12887-020-02142-5
69. Evennett NJ, Hall NJ, Pierro A, Eaton S. Urinary intestinal fatty acid-binding protein concentration predicts extent of disease in necrotizing enterocolitis. J Pediatr Surg. (2010) 251(45):735–40. doi: 10.1016/j.jpedsurg.2009.09.024
70. Ho SSC, Keenan JI, Day AS. The role of gastrointestinal-related fatty acid-binding proteins as biomarkers in gastrointestinal diseases. Dig Dis Sci. (2020) 65:376–90. doi: 10.1007/s10620-019-05841-x
71. Benkoe T, Reck C, Pones M, Weninger M, Gleiss A, Stift A, et al. Interleukin-8 predicts 60 day mortality in premature infants with necrotizing enterocolitis. J Pediatr Surg. (2014) 49(3):385–9. doi: 10.1016/j.jpedsurg.2013.05.068
72. Kalteren WS, Bos AF, Bergman KA, van Oeveren W, Hulscher JBF, Kooi EMW. The short-term effects of RBC transfusions on intestinal injury in preterm infants. Pediatr Res. (2022):1–7. doi: 10.1038/s41390-022-01961-9. [Epub ahead of print]
73. Žurek J, Kýr M, Vavřina M, Fedora M. Trefoil factor 3 as a marker of intestinal cell damage during sepsis. Open Med. (2015) 10(1):261–6. doi: 10.1515/med-2015-0020
74. Garner CE, Smith S, de Lacy Costello B, White P, Spencer R, Probert CS, et al. Volatile organic compounds from feces and their potential for diagnosis of gastrointestinal disease. Faseb j. (2007) 21(8):1675–88. doi: 10.1096/fj.06-6927com
75. van Gaal N, Lakenman R, Covington J, Savage R, de Groot E, Bomers M, et al. Faecal volatile organic compounds analysis using field asymmetric ion mobility spectrometry: non-invasive diagnostics in paediatric inflammatory bowel disease. J Breath Res. (2017) 12(1):016006. doi: 10.1088/1752-7163/aa6f1d
76. Bond A, Greenwood R, Lewis S, Corfe B, Sarkar S, O'Toole P, et al. Volatile organic compounds emitted from faeces as a biomarker for colorectal cancer. Aliment Pharmacol Ther. (2019) 49(8):1005–12. doi: 10.1111/apt.15140
77. El Manouni El Hassani S, Soers RJ, Berkhout DJC, Niemarkt HJ, Weda H, Nijsen T, et al. Optimized sample preparation for fecal volatile organic compound analysis by gas chromatography–mass spectrometry. Metabolomics. (2020) 16:112. doi: 10.1007/s11306-020-01735-6
78. Course C, Watkins WJ, Müller CT, Odd D, Kotecha S, Chakraborty M. Volatile organic compounds as disease predictors in newborn infants: a systematic review. J Breath Res. (2021) 15(2):024002. doi: 10.1088/1752-7163/abe283
79. El-Metwally D, Chain K, Stefanak MP, Alwis U, Blount BC, LaKind JS, et al. Urinary metabolites of volatile organic compounds of infants in the neonatal intensive care unit. Pediatr. Res. (2018) 83:1158–64. doi: 10.1038/pr.2018.52
80. Prazad P, Cortes DR, Puppala BL, Donovan R, Kumar S, Gulati A. Airborne concentrations of volatile organic compounds in neonatal incubators. J. Perinatol. (2008) 28:534–40. doi: 10.1038/jp.2008.75
81. Fagerhol MK. Calprotectin: a faecal marker of organic gastrointenstinal abnormality. Lancet. (2000) 356:1783–4. doi: 10.1016/S0140-6736(00)03224-4
82. Bin-Nun A, Booms C, Sabag N, Mevorach R, Algur N, Hammerman C. Rapid fecal calprotectin (FC) analysis: point of care testing for diagnosing early necrotizing enterocolitis. Am J Perinatol. (2014) 32(4):337–42. doi: 10.1055/s-0034-1384640
83. Sutherland AD, Gearry RB, Frizelle FA. Review of fecal biomarkers in inflammatory bowel disease. Dis Colon Rectum. (2008) 51(8):1283–91. doi: 10.1007/s10350-008-9310-8
84. D'Incà R, Dal Pont E, Di Leo V, Benazzato L, Martinato M, Lamboglia F, et al. Can calprotectin predict relapse risk in inflammatory bowel disease? Am J Gastroenterol. (2008) 103(8):2007–14. doi: 10.1111/j.1572-0241.2008.01870.x
85. Pergialiotis V, Konstantopoulos P, Karampetsou N, Koutaki D, Gkioka E, Perrea DN, et al. Calprotectin levels in necrotizing enterocolitis: a systematic review of the literature. Inflammation Res. (2016) 65(11):847–52. doi: 10.1007/s00011-016-0963-9
86. Sylvester KG, Ling XB, Liu GY, Kastenberg ZJ, Ji J, Hu Z, et al. A novel urine peptide biomarker-based algorithm for the prognosis of necrotising enterocolitis in human infants. Gut. (2014) 63(8):1284–92. doi: 10.1136/gutjnl-2013-305130
87. Hagiwara SI, Okayasu I, Fujiwara M, Matsuura M, Ohnishi H, Ito S, et al. Prostaglandin E-major urinary metabolite as a biomarker for pediatric ulcerative colitis activity. J Pediatr Gastroenterol Nutr. (2017) 64(6):955–61. doi: 10.1097/MPG.0000000000001477
88. Arai Y, Matsuura T, Matsuura M, Fujiwara M, Okayasu I, Ito S, Arihiro S, et al. Prostaglandin E-Major urinary metabolite as a biomarker for inflammation in ulcerative colitis: prostaglandins revisited. Digestion. (2016) 93(1):32–9. doi: 10.1159/000441665
89. Sylvester KG, Ling XB, Liu GY, Kastenberg ZJ, Ji J, Hu Z, et al. Urine protein biomarkers for the diagnosis and prognosis of necrotizing enterocolitis in infants. J Pediatr. (2014) 164(3):607–12. doi: 10.1016/j.jpeds.2013.10.091
90. Blackwood BP, Wood DR, Yuan CY, Nicolas JD, Griffiths A, Mestan K, et al. Urinary claudin-2 measurements as a predictor of necrotizing enterocolitis: a pilot study. J Neonatal Surg. (2015) 4(4):43. doi: 10.47338/jns.v4.457
91. van Vliet MJ, Tissing WJ, Rings EH, Koetse HA, Stellaard F, Kamps WA, et al. Citrulline as a marker for chemotherapy induced mucosal barrier injury in pediatric patients. Pediatr Blood Cancer. (2009) 53:1188–94. doi: 10.1002/pbc.22210
92. Wilcock A, Begley P, Stevens A, Whatmore A, Victor S. The metabolomics of necrotising enterocolitis in preterm babies: an exploratory study. J Matern Fetal Neonatal Med. (2016) 29(5):758–62. doi: 10.3109/14767058.2015.1017462
93. Morrow AL, Lagomarcino AJ, Schibler KR, Taft DH, Yu Z, Wang B, et al. Early microbial and metabolomic signatures predict later onset of necrotizing enterocolitis in preterm infants. Microbiome. (2013) 1(1):13. doi: 10.1186/2049-2618-1-13
94. Murgas Torrazza R, Li N, Young C, Kobeissy F, Chow M, Chen S, et al. Pilot study using proteomics to identify predictive biomarkers of necrotizing enterocolitis from buccal swabs in very low birth weight infants. Neonatol. (2013) 104(3):234–42. doi: 10.1159/000353721
95. Zhou W, Yuan W, Huang L, Wang P, Rong X, Tang J. Association of neonatal necrotizing enterocolitis with myeloid differentiation-2 and GM2 activator protein genetic polymorphisms. Mol Med Rep. (2015) 12(1):974–80. doi: 10.3892/mmr.2015.3499
96. Sampath V, Le M, Lane L, Patel AL, Cohen JD, Simpson PM, Garland JS, Hines RN. The NFKB1 (g.-24519delATTG) variant is associated with necrotizing enterocolitis (NEC) in premature infants. J Surg Res. (2011) 169:e51–57. doi: 10.1016/j.jss.2011.03.017
97. Sampath V, Menden H, Helbling D, Li K, Gastonguay A, Ramchandran R, et al. SIGIRR Genetic variants in premature infants with necrotizing enterocolitis. Pediatr. (2015) 135(6):e1530–4. doi: 10.1542/peds.2014-3386
98. Agakidou E, Agakidis C, Gika H, Sarafidis K. Emerging biomarkers for prediction and early diagnosis of necrotizing enterocolitis in the era of metabolomics and proteomics. Front Pediatr. (2020) 8:602255. doi: 10.3389/fped.2020.602255
99. Llanos AR, Moss ME, Pinzòn MC, Dye T, Sinkin RA, Kendig JW. Epidemiology of neonatal necrotising enterocolitis: a population-based study. Paediatr Perinat Epidemiol. (2002) 6:342–9. doi: 10.1046/j.1365-3016.2002.00445.x
100. Bhandari V, Bizzarro MJ, Shetty A, Zhong X, Page GP, Zhang H, et al. Neonatal genetics study group. Familial and genetic susceptibility to major neonatal morbidities in preterm twins. Pediatrics. (2006) 117:1901–6. doi: 10.1542/peds.2005-1414
101. Fawley J, Cuna A, Menden HL, McElroy S, Umar S, Welak SR, et al. Single-Immunoglobulin interleukin-1-related receptor regulates vulnerability to TLR4-mediated necrotizing enterocolitis in a mouse model. Pediatr Res. (2017) 83:164–74. doi: 10.1038/pr.2017.211
102. Chen H, Zeng L, Zheng W, Li X, Lin B. Increased expression of microRNA- 141-3p improves necrotizing enterocolitis of neonates through targeting MNX1. Front Pediatr. (2020) 8:385. doi: 10.3389/fped.2020.00385
103. Liu H, Wang YB. Systematic large-scale meta-analysis identifies microRNA-429/200a/b and microRNA-141/20°c clusters as biomarkers for necrotizing enterocolitis in newborn. Biosci Rep. (2019) 39:BSR20191503. doi: 10.1042/BSR20191503
104. Xu Y, Liu Y, Xie H, Zhou Y, Yan X, Chen W, et al. Profile analysis reveals endogenous RNAs regulate necrotizing enterocolitis progression. Biomed Pharmacother. (2020) 125:109975. doi: 10.1016/j.biopha.2020.109975
105. Wu Yu, Kathy C, Kam L, Lam T, Tam H, Lee Y, et al. Dysregulation of miR-431 and target gene FOXA1 in intestinal tissues of infants with necrotizing enterocolitis. FASEB J. (2019) 33:5143–52. doi: 10.1096/fj.201801470R
106. Jung K, Koh I, Kim JH, Cheong HS, Park T, Nam SH, et al. RNA-Seq for gene expression profiling of human necrotizing enterocolitis: a pilot study. Korean Med Sci. (2017) 32:817–24. doi: 10.3346/jkms.2017.32.5.817
107. Pan X, Muk T, Ren S, Nguyen DN, Shen RL, Gao F, et al. Blood transcriptomic markers of necrotizing enterocolitis in preterm pigs. Pediatr Res. (2022) 91(5):1113–20. doi: 10.1038/s41390-021-01605-4
108. Al-Shargabi T, Reich D, Govindan RB, Shankar S, Metzler M, Cristante C, et al. Changes in autonomic tone in premature infants developing necrotizing enterocolitis. Am J Perinatol. (2018) 35(11):1079–86. doi: 10.1055/s-0038-1639339
109. Doheny KK, Palmer C, Browning KN, Jairath P, Liao D, He F, et al. Diminished vagal tone is a predictive biomarker of necrotizing enterocolitis-risk in preterm infants. Neurogastroenterol and Motility. (2014) 26(6):832–40. doi: 10.1111/nmo.12337
110. Moss RL, Kalish LA, Duggan C, Johnston P, Brandt ML, Dunn JC, et al. Clinical parameters do not adequately predict outcome in necrotizing enterocolitis: a multi-institutional study. J Perinatol. (2008) 28(10):665–74. doi: 10.1038/jp.2008.119
111. Ji J, Ling XB, Zhao Y, Hu Z, Zheng X, Xu Z, et al. A data-driven algorithm integrating clinical and laboratory features for the diagnosis and prognosis of necrotizing enterocolitis. PLOS ONE. (2014) 9(2):e89860. doi: 10.1371/journal.pone.0089860
112. Howarth CN, Leung TS, Banerjee J, Eaton S, Morris JK, Aladangady N. Regional cerebral and splanchnic tissue oxygen saturation in preterm infants – longitudinal normative measurements. Early Hum Dev. (2022) 165:105540. doi: 10.1016/j.earlhumdev.2022.105540
113. Fortune PM, Wagstaff M, Petros AJ. Cerebro-splanchnic oxygenation ratio (CSOR) using near infrared spectroscopy may be able to predict splanchnic ischaemia in neonates. Intensive Care Med. (2001) 27(8):1401–7. doi: 10.1007/s001340100994
114. Zamora IJ, Stoll B, Ethun CG, Sheikh F, Yu L, Burrin DG, et al. Low abdominal NIRS values and elevated plasma intestinal faty acid-binding protein in a premature piglet model of necrotizing enterocolits in premture piglets. PLoS One. (2015) 10(10):e0125437. doi: 10.1371/journal.pone.0125437
115. Pavlek LR, Mueller C, Jebbia MR, Kielt MJ, Fathi O. Near-Infrared spectroscopy in extremely preterm infants. Front Pediatr. (2021) 8:624113. doi: 10.3389/fped.2020.624113
116. Schat TE, Schurink M, van der Laan ME, Hulscher JB, Hulzebos CV, Bos AF, et al. Near-Infrared spectroscopy to predict the course of necrotizing enterocolitis. PLoS ONE. (2016) 11(5):e0154710. doi: 10.1371/journal.pone.0154710
117. Le Bouhellec J, Prodhomme O, Mura T, Jacquot A, Combes C, Gamon L, et al. Near-Infrared spectroscopy: a tool for diagnosing necrotizing enterocolitis at onset of symptoms in preterm neonates with acute gastrointestinal symptoms? Am J Perinatol. (2021) 38(S 01):e299–308. doi: 10.1055/s-0040-1710033
Keywords: NEC, biomarker, gut oxygenation, tissue injury, ischaemia
Citation: Howarth C, Banerjee J, Eaton S and Aladangady N (2022) Biomarkers of gut injury in neonates – where are we in predicting necrotising enterocolitis?. Front. Pediatr. 10:1048322. doi: 10.3389/fped.2022.1048322
Received: 19 September 2022; Accepted: 7 November 2022;
Published: 28 November 2022.
Edited by:
Elisabeth M.W. Kooi, University Medical Center Groningen, NetherlandsReviewed by:
Jixin Yang, Tongji Hospital, ChinaJoachim Friedrich Kuebler, Hannover Medical School, Germany
© 2022 Howarth, Banerjee, Eaton and Aladangady. This is an open-access article distributed under the terms of the Creative Commons Attribution License (CC BY). The use, distribution or reproduction in other forums is permitted, provided the original author(s) and the copyright owner(s) are credited and that the original publication in this journal is cited, in accordance with accepted academic practice. No use, distribution or reproduction is permitted which does not comply with these terms.
*Correspondence: Claire Howarth Y2xhaXJlLmhvd2FydGhAbmhzLm5ldA==
Specialty Section: This article was submitted to Neonatology, a section of the journal Frontiers in Pediatrics