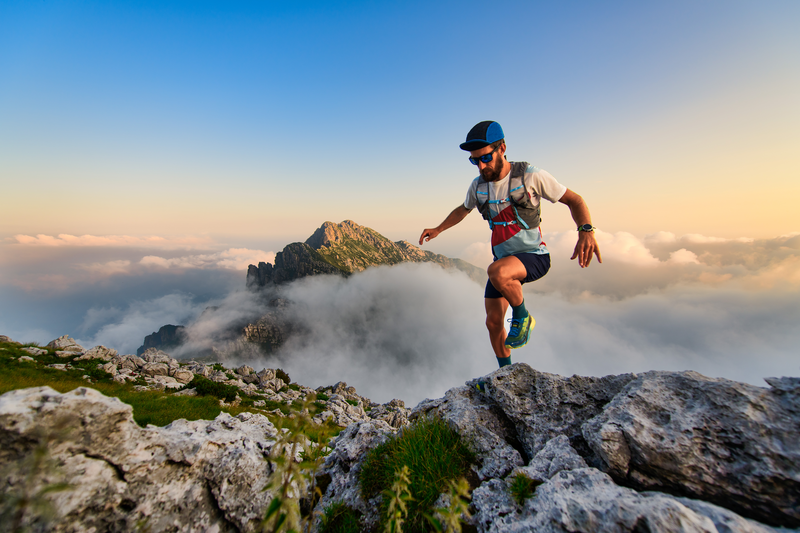
94% of researchers rate our articles as excellent or good
Learn more about the work of our research integrity team to safeguard the quality of each article we publish.
Find out more
MINI REVIEW article
Front. Pediatr. , 07 November 2022
Sec. Pediatric Immunology
Volume 10 - 2022 | https://doi.org/10.3389/fped.2022.1046100
This article is part of the Research Topic Neonatal Infections and the Developing Neonatal Immune System: Current Evidence and Research Gaps to Fill View all 9 articles
In utero exposure to maternally-derived antigens following chronic infection is associated with modulation of infants ‘immune response, differential susceptibility to post-natal infections and immune response toward vaccines. The maternal environment, both internal (microbiota) and external (exposure to environmental microbes) also modulates infant's immune response but also the clinical phenotype after birth. Vertical transmission of ubiquitous respiratory pathogens such as influenza and COVID-19 is uncommon. Evidence suggest that in utero exposure to maternal influenza and SARS-CoV-2 infections may have a significant impact on the developing immune system with activation of both innate and adaptive responses, possibly related to placental inflammation. Here in, we review how maternal respiratory infections, associated with airway, systemic and placental inflammation but also changes in maternal microbiota might impact infant's immune responses after birth. The clinical impact of immune modifications observed following maternal respiratory infections remains unexplored. Given the high frequencies of respiratory infections during pregnancy (COVID-19, influenza but also RSV and HMPV), the impact on global child health could be important.
Early immune responses in humans, both during the immediate post-natal period and infancy are shaped in utero and modulated by both maternal and environmental factors (1). As such, exposure to chronic maternal infections, in the absence of vertical transmission, is associated with induction of innate immune responses, pathogen-specific adaptive immune responses (sensitization) and decreased transfer rate of maternal antibodies. Those immune abnormalities are linked to modified susceptibility toward homologous pathogens (i.e., pathogen to which the infant was exposed in utero) or heterologous infections and modifications of immune response toward vaccine at birth or during infancy (2–7).
In utero exposure to non-pathogenic microbial environmental factors have also an impact on the clinical phenotype during infancy. This include differential susceptibility toward occurrence of immune mediated diseases such as atopic dermatitis or asthma (2, 8).
Influenza infection and influenza-like illness are relatively common during pregnancy worldwide, in both low & middle and high income countries (9–11). For example, incidence rates ranged from 0.7 to 0.9% per month of pregnancy in a large multi-centric studies in 3 middle income countries (10). In the pre-COVID-19 vaccine era, up to 10% of pregnant or recently pregnant women were diagnosed with COVID-19 at hospital admission for any reason (12).
Both maternal influenza and COVID-19 infections are associated with increased maternal morbidity and mortality but also increased risk of adverse neonatal outcomes such as low birthweight and premature birth (12–16). Vertical transmission with COVID-19 or influenza is uncommon (16).
Respiratory infections, such as influenza and COVID-19, are associated with lung, systemic and also placental inflammation that could impact post-natal immune responses. Infections-associated modulation of the maternal microbiota could also have an impact on neonates' microbiota and infants ‘immune responses.
The aim of this mini review is to summarize the possible mechanisms and current knowledge linking maternal respiratory infections with modulation of neonatal immunity and immune-related health outcomes.
Different studies across the world have demonstrated that the respiratory environment and specifically the microbial respiratory environment of the mother can impact fetal immunity and also the clinical phenotype during infancy. T-cells sensitization toward allergens found in maternal homes have been detected in cord blood (17). Exposure to a farming environment, contaminated by mold and bacteria species, during pregnancy has been associated with differential expression of Toll-like receptors (TLR) genes at birth (18). Children born to mothers living in farm environments have decreased risk of asthma and atopic dermatitis during childhood as compared to children born to mothers not living in such environment (19).
The factors associated with farm exposure and modulation susceptibility to atopic disease is likely multifactorial (19). Animal models suggest that microbial exposure through the maternal respiratory tract likely contribute to both immune and clinical risk modifications. In a mice model, maternal intra-nasal exposure during pregnancy to a nonpathogenic bacterium (Acinetobacter lwoffii) was protective against the development of experimental asthma in the progeny (20). This protection was related to modulation of both maternal and placental immune responses. Maternal exposure to A. lwoffii was associated with increased levels of maternal lung pro-inflammatory cytokines (TNF, IL-6 and IL-12p40) and TLR mRNA (TLR-2, 3, 6, 7 and 9). On the other hand, a decreased expression of placental TLR mRNA (TLR 6 and 7) was observed along with suppression of pro-inflammatory placental cytokines production (IL-1 and TNF). Different knock-out experiments using TLR deficient mice suggest a critical role of maternal TLR signaling in the protection against asthma in their progeny.
Overall these data indicate that prenatal exposure to a specific maternal microbial respiratory environment is associated with modification of immune responses at birth with a potential clinical impact during infancy.
Longitudinal cohort studies suggest that infant upper respiratory tract flora are acquired at least in part from their mothers (21). It has been suggested that the placenta is home to beneficial commensals (22) and that microbiota inhabit the fetal lung. Moreover, changes in microbiota composition during pregnancy influence fetal growth and development (23). Nowadays, the microbiome, defined as commensal living microorganisms, their genome, environment and the host interactions, is known to be responsible for the maintenance of homeostasis and tissue stability of different organs including the lung. The microbiome also impacts crucial event such as conventional dendritic cell maturation that will shape innate and adaptive immunity in early life (24). There is evidence that editing of the maternal gut microbiome with some beneficial bacterial strains during pregnancy may attenuate the features of allergic airways disease in the offspring through the stimulation of bone marrow hematopoiesis of dendritic cells (DC) precursors (25). A recent murine study demonstrates the role of maternal gut microbiome in regulating immunity to respiratory syncytial virus (RSV) infection in offspring. A prenatal Lactobacillus exposure through maternal supplementation led to decreased Th2 cytokines and lung inflammation following RSV infection in their neonates (26). Therefore, the interplay between maternal microbiota and the infant immunity may occur through microbiota editing or dysbiosis.
During chronic maternal infections with malaria or helminths, in utero sensitization occurs and is associated with modification of infant's susceptibility toward homologous infection during infancy (27, 28). As an example, placental malaria modifies the risk of malaria during infancy, possibly by the induction of a tolerant phenotype in exposed-infants (27).
In utero sensitization following maternal respiratory infection could possibly modulate the risk of infection in the post-natal period. Whether or not in utero sensitization to influenza occurs remains controversial: some historical studies have detected the presence of influenza-specific IgM (29, 30) in cord blood of exposed-newborns while a more recent study has not (11). Following maternal COVID-19 infection, no sign of SARS-CoV-2 sensitization has been reported by different studies, assessed by T-cells specific activation of IgM production in cord blood (31–33).
These observations indicate that in utero sensitization toward respiratory infections is uncommon following acute maternal respiratory infections, on the contrary to what is reported during maternal chronic infection or exposure to environmental antigens (2, 17).
During maternal infection, placental inflammation could occur following direct placental infection or systemic inflammation.
Since the beginning of the COVID-19 pandemic, various studies by different groups have assessed the impact of maternal COVID-19 infection on placental inflammatory responses by directly studying placenta from SARS-CoV-2 infected mothers or by using models of placental explants models (32, 34–37).
Ex vivo models of placental infection indicate that SARS-CoV-2 is able to replicate and propagates in human placenta (37). Placental SARS-CoV-2 RNA is detected at high frequency following late maternal infection during pregnancy. Subgenomic RNA was found in 22/52 (42%) of a cohort of mothers who were tested positive at delivery and was localized to the trophoblasts (35). However, no live virus was detected. Important immune cells infiltration is found in the placenta of infected mothers (34). SARS-CoV-2 infected placenta explants display strong inflammatory responses with increased expression of chemokines, inflammations pathways and apoptosis/necrosis related genes (35).
The impact of maternal influenza infection during pregnancy on placental pathology and inflammatory responses has been assessed in mice models. Following maternal influenza infection, no viral RNA is detected in the placenta or in the fetal tissue, in line with the absence of vertical transmission documented in humans. However, strong IFN responses are detected in the placenta (38) along with production of inflammatory chemokines (RANTES and G-CSF) (39).
In summary, these observations from both clinical and animal studies, indicate that maternal respiratory infections during pregnancy, in the absence of vertical transmission, induce strong inflammatory responses in the placenta with production of chemokines, leading to immune cell infiltrations.
Since the start of the COVID-19 pandemic, different human studies have been performed on the impact of maternal SARS-CoV-2 infection on neonatal immunity shedding light on the potential impact of a respiratory infection on neonatal immunity.
An important study that included three groups of mothers: non-SARS-CoV-2 infected, recovered infection and one with recent/ongoing infection found a gradient in the magnitude of the innate immune responses induced in cord blood. Those inflammatory responses included expansion of innate immune cells such as γδ T cells and NK cells and production of pro-inflammatory chemokines (CXCL8). Fetal immune cells show signs of activation as assessed by TNF-α and IFN-γ production. Immune activation is probably non-specific as no sign of SARS-CoV-2 sensitization, has been observed (31).
In a similar study that included 11 PCR-confirmed, mostly asymptomatic mothers, higher IL-8 production was found in cord blood of SARS-CoV-2-exposed neonates. Interestingly, exposure to SARS-CoV-2 in utero was associated with differential expression of genes involved in defense toward bacteria and fungus (32). Mild decrease of reactive oxygen species production by monocytes of SARS-CoV-2 exposed neonates was also reported.
Altogether, these data suggest that exposure to SARS-CoV-2 during pregnancy is associated with significant fetal immune activation, independent of vertical transmission and is probably related to placental inflammation more than transfer of viral byproducts. Importantly, those immune modifications have been reported in neonate born to asymptomatic mothers, suggesting that the long term impact could involve large numbers of neonates.
No human studies have however explored the impact of maternal influenza infection on fetal immunity; although different mice models provided insight on the potential impact of maternal influenza infection on fetal immunity. In one study, a downregulation of genes associated with Toll-like receptor signaling and T cell differentiation in fetuses was observed, suggesting a potential impact on infants post-natal immune responses (40). In another study that also used a model of maternal intra nasal influenza A virus (IAV) infection, in utero exposure to influenza was associated with reduced frequencies of NK and B cells in the lungs from offspring born to IAV-infected dams. Adoptive transfer experiments of alveolar macrophages from offspring born to uninfected-dams to offspring born to IAV-infected dams suggested an impairment of the function of alveolar macrophages secondary to in utero influenza infection. Indeed, offspring exposed to influenza in utero showed better recovery following influenza B virus infection after transfer of alveolar macrophages from offspring born to IAV-uninfected dams (41). Finally, elevated levels of the pro-inflammatory cytokine IL-1 was found in the cord blood of offspring born to influenza-infected dams (39).
In summary, evidences from both clinical and animal studies, indicate that exposure to respiratory infections during pregnancy, including mild disease, is associated with expansions and activation of innate immune cells, with a possible impact on the functionality of innate cells such as monocytes and lung macrophages.
The profound clinical impact during childhood of in utero exposure to maternal influenza infection has been reported since the 1,918 influenza pandemic (14, 42). Immediate post-natal health impact of maternal influenza infection include stillbirth, low birth weight and prematurity. Congenital heart defects and neurological and behavioral changes such as schizophrenia are also reported during childhood after maternal influenza infection. Large nationwide studies have also shown long-term effect of maternal influenza infection with increased morbidity during adulthood (43–45). Preliminary data suggest that in utero exposure to SARS-CoV-2 during pregnancy might impact neurodevelopmental outcomes at 1 year of age (46).
The impact of both maternal influenza and SARS-CoV-2 infection on the susceptibility to infections during infancy has not been assessed in humans. In a mice model of maternal influenza A infection, exposed neonates were more susceptible to heterologous infections challenges (influenza B and MRSA), possibly related to alveolar macrophages dysfunction (41). Studies are currently underway to assess the long-term health consequences of in utero SARS-CoV-2 exposure, including infectious risk during infancy.
We have reviewed the different mechanisms associated with acute maternal respiratory infections that could possibly impact infant's immune responses.
Previous research in the field of allergy and chronic maternal infections have highlighted the impact of maternal exposure to specific microbial respiratory environment and immune modulation related to chronic infections on infant's immune responses. Susceptibility toward infection and response to vaccines in infancy are modulated after exposure to chronic maternal infection, without vertical transmission while susceptibility toward immune-mediated diseases (asthma, atopic dermatitis) is impacted by the respiratory maternal environment (2, 8, 19).
In this mini-review, we show that part of these mechanisms are also observed during maternal respiratory infections, namely induction of innate immune responses and placental inflammation. On the other hand, in utero sensitization seems infrequent after exposure to maternal respiratory infections such as influenza and COVID-19.
Maternal microbiota editing may also have an impact on infants ‘immune responses. During pregnancy, disturbance in the mother microbiota either as a result of infection or stress linked to sexual hormones might influence infant health through several ways. Following lung-gut microbiota crosstalk, additional effects may be associated with upper respiratory infections during pregnancy. Alterations in the lung microbiota profile have been associated with several respiratory diseases such as pneumonia or viral infection (47). Dysbiosis of the lung and gut microbiomes have been also observed in severe COVID-19 patients (48, 49) and following influenza infection in mice and humans (50, 51). How prenatal events such as mother's infection influence the induction and training of a healthy maturation of the innate and adaptive immunity in early life still need further investigations. How the materno-fetal microbiota alterations associated with respiratory infections may affect the ontogeny of neonatal innate and adaptive immunity is underexplored and warrants further research.
Maternal respiratory infections such as influenza and COVID-19 are associated, beside upper and respiratory tract inflammatory responses, with systemic and placental inflammatory responses. Studies performed during the COVID-19 pandemic indicate that neonates exposed to respiratory infections have distinct innate but also adaptive responses, independently of virus-specific immune activation, as sensitization appears infrequent (31–33). Results from both humans and animal studies suggest that anti-microbial immune responses might be affected following exposure to viral respiratory infections during pregnancy (32, 40, 41). Differential expression of genes involved in anti-microbial responses (32) are reported following COVID-19 infection. Altered functionality of monocytes and alveolar macrophages are described following maternal respiratory infection, which might impact susceptibility toward post-natal bacterial infection, as shown in a mice model (41). Previous research in the field of chronic maternal HIV infection has highlighted the role of neonatal immune activation at birth on infectious risk during infancy. Indeed, HIV-exposed but uninfected infants born to mothers who initiated anti-retroviral therapy during pregnancy have higher risk of hospitalization for infection as compared to HIV-unexposed infants and this risk is related to activation of monocytes at birth (6). These observations warrant clinical studies assessing the risk of infections in early life following respiratory infections.
Modulation of placental TLR responses is associated with differential risk of atopic diseases during infancy. The impact of maternal influenza or COVID-19-induced placental inflammation on the risk of atopic diseases during infancy is undetermined.
Finally, responses to vaccines at birth or during infancy, might also be impacted following disturbances of both adaptive and innate immune responses, as previously shown following chronic maternal infections with protozoans or helminths (3, 7). Notably, decreased transfer of vaccine and pathogens-specific IgG are observed following chronic maternal infections (2). The mechanisms involved possibly include hypergammaglobulinemia (52) but also placental inflammation, with subsequent alteration of IgG transfer through the Fc neonatal receptor (2). Hypergammaglobulinemia has not been reported during acute maternal COVID-19 or influenza infection but placental inflammation could impact the transfer of maternal IgG. Given the increasing role of maternal immunization in the prevention of maternal and neonatal morbidity (53), more studies are required to assess the impact of both influenza and COVID-19 on the transfer of maternally-derived IgG specific for current and upcoming vaccines such as pertussis, tetanus, Group B streptococcus and RSV.
Most of the literature reviewed here was related to influenza and COVID-19. Other respiratory infections such as RSV and human metapneumovirus (HMPV) are highly prevalent worldwide and also reported during pregnancy as causes of acute respiratory illnesses (54–56). More research is needed to assess the impact of maternal RSV or HMPV infections on placental and infants ‘immune responses.
Maternal vaccination is recommended during pregnancy for both influenza and COVID-19; whether maternal vaccination is associated with dampening of inflammatory immune response following respiratory infection remain to be demonstrated.
N.D. drafted the first version of the manuscript. V.F. revised and edited the manuscript. All authors contributed to the article and approved the submitted version.
N.D. is a post-doctoral master specialist of the F.R.S-FNRS. This article is published with the support of the Fondation Universitaire of Belgium
The authors declare that the research was conducted in the absence of any commercial or financial relationships that could be construed as a potential conflict of interest.
All claims expressed in this article are solely those of the authors and do not necessarily represent those of their affiliated organizations, or those of the publisher, the editors and the reviewers. Any product that may be evaluated in this article, or claim that may be made by its manufacturer, is not guaranteed or endorsed by the publisher.
1. Apostol AC, Jensen KD, Beaudin AE. Training the fetal immune system through maternal inflammation—a layered hygiene hypothesis. Front Immunol. (2020) 11:123. doi: 10.3389/fimmu.2020.00123
2. Dauby N, Goetghebuer T, Kollmann TR, Levy J, Marchant A. Uninfected but not unaffected: chronic maternal infections during pregnancy, fetal immunity, and susceptibility to postnatal infections. Lancet Infect Dis. (2012) 12:330–40. doi: 10.1016/S1473-3099(11)70341-3
3. Dauby N, Alonso-Vega C, Suarez E, Flores A, Hermann E, Cordova M, et al. Maternal infection with trypanosoma cruzi and congenital chagas disease induce a trend to a type 1 polarization of infant immune responses to vaccines. PLoS Negl Trop Dis. (2009) 3:e571. doi: 10.1371/journal.pntd.0000571
4. Dauby N, Chamekh M, Melin P, Slogrove AL, Goetghebuer T. Increased risk of group B Streptococcus invasive infection in HIV-exposed but uninfected infants: a review of the evidence and possible mechanisms. Front Immunol. (2016) 7:505. doi: 10.3389/fimmu.2016.00505
5. Adler C, Haelterman E, Barlow P, Marchant A, Levy J, Goetghebuer T. Severe infections in HIV-exposed uninfected infants born in a European country. PLoS ONE. (2015) 10:e0135375. doi: 10.1371/journal.pone.0135375
6. Goetghebuer T, Smolen KK, Adler C, Das J, McBride T, Smits G, et al. Initiation of antiretroviral therapy before pregnancy reduces the risk of infection-related hospitalization in human immunodeficiency virus–exposed uninfected infants born in a high-income country. Clin Infect Dis. (2019) 68:1193–203. doi: 10.1093/cid/ciy673
7. LaBeaud AD, Malhotra I, King MJ, King CL, King CH. Do antenatal parasite infections devalue childhood vaccination? PLoS Negl Trop Dis. (2009) 3:e442. doi: 10.1371/journal.pntd.0000442
8. von Mutius E. The microbial environment and its influence on asthma prevention in early life. J Allergy Clin Immunol. (2016) 137:680–9. doi: 10.1016/j.jaci.2015.12.1301
9. Azziz-Baumgartner E, Veguilla V, Calvo A, Franco D, Dominguez R, Rauda R, et al. Incidence of influenza and other respiratory viruses among pregnant women: a multi-country, multiyear cohort. Intl J Gynecol & Obste. (2022) 158:359–67. doi: 10.1002/ijgo.14018
10. Dawood FS, Kittikraisak W, Patel A, Rentz Hunt D, Suntarattiwong P, Wesley MG, et al. Incidence of influenza during pregnancy and association with pregnancy and perinatal outcomes in three middle-income countries: a multisite prospective longitudinal cohort study. Lancet Infect Dis. (2021) 21:97–106. doi: 10.1016/S1473-3099(20)30592-2
11. Irving WL, James DK, Stephenson T, Laing P, Jameson C, Oxford JS, et al. Influenza virus infection in the second and third trimesters of pregnancy: a clinical and seroepidemiological study. BJOG. (2000) 107:1282–9. doi: 10.1111/j.1471-0528.2000.tb11621.x
12. Allotey J, Fernandez S, Bonet M, Stallings E, Yap M, Kew T, et al. Clinical manifestations, risk factors, and maternal and perinatal outcomes of coronavirus disease 2019 in pregnancy: living systematic review and meta-analysis. Br Med J. (2020) 370:m3320. doi: 10.1136/bmj.m3320
13. Mertz D, Geraci J, Winkup J, Gessner BD, Ortiz JR, Loeb M. Pregnancy as a risk factor for severe outcomes from influenza virus infection: a systematic review and meta-analysis of observational studies. Vaccine. (2017) 35:521–8. doi: 10.1016/j.vaccine.2016.12.012
14. Chandra S, Christensen J, Mamelund S-E, Paneth N. Short-Term birth sequelae of the 1918–1920 influenza pandemic in the United States: state-level analysis. Am J Epidemiol. (2018) 187:2585–95. doi: 10.1093/aje/kwy153
15. Mosby LG, Rasmussen SA, Jamieson DJ. 2009 Pandemic influenza A (H1N1) in pregnancy: a systematic review of the literature. Am J Obstet Gynecol. (2011) 205:10–8. doi: 10.1016/j.ajog.2010.12.033
16. Pietrasanta C, Artieri G, Ronchi A, Crippa B, Ballerini C, Crimi R, et al. SARS-Cov-2 infection and neonates: evidence-based data after 18 months of the pandemic. Pediatr Allergy Immunol. (2022) 33:96–8. doi: 10.1111/pai.13643
17. Miller RL, Chew GL, Bell CA, Biedermann SA, Aggarwal M, Kinney PL, et al. Prenatal exposure, maternal sensitization, and sensitization in utero to indoor allergens in an inner-city cohort. Am J Respir Crit Care Med. (2001) 164:995–1001. doi: 10.1164/ajrccm.164.6.2011107
18. Roduit C, Wohlgensinger J, Frei R, Bitter S, Bieli C, Loeliger S, et al. Prenatal animal contact and gene expression of innate immunity receptors at birth are associated with atopic dermatitis. J Allergy Clin Immunol. (2011) 127:179–85. doi: 10.1016/j.jaci.2010.10.010
19. Frei R, Heye K, Roduit C. Environmental influences on childhood allergies and asthma - the farm effect. Pediatr Allergy Immunol. (2022) 33:e13807. doi: 10.1111/pai.13807
20. Conrad ML, Ferstl R, Teich R, Brand S, Blümer N, Yildirim AO, et al. Maternal TLR signaling is required for prenatal asthma protection by the nonpathogenic microbe Acinetobacter lwoffii F78. J Exp Med. (2009) 206:2869–77. doi: 10.1084/jem.20090845
21. Ferretti P, Pasolli E, Tett A, Asnicar F, Gorfer V, Fedi S, et al. Mother-to-infant microbial transmission from different body sites shapes the developing infant gut microbiome. Cell Host Microbe. (2018) 24:133–45. doi: 10.1016/j.chom.2018.06.005
22. Aagaard K, Ma J, Antony KM, Ganu R, Petrosino J, Versalovic J. The placenta harbors a unique microbiome. Sci Transl Med. (2014);6(237):237ra65. doi: 10.1126/scitranslmed.3008599
23. Nuriel-Ohayon M, Neuman H, Koren O. Microbial changes during pregnancy, birth, and infancy. Front Microbiol. (2016) 7:1031. doi: 10.3389/fmicb.2016.01031
24. Köhler A, Delbauve S, Smout J, Torres D, Flamand V. Very early-life exposure to microbiota-induced TNF drives the maturation of neonatal pre-cDC1. Gut. (2021) 70:511–21. doi: 10.1136/gutjnl-2019-319700
25. Trompette A, Gollwitzer ES, Yadava K, Sichelstiel AK, Sprenger N, Ngom-Bru C, et al. Gut microbiota metabolism of dietary fiber influences allergic airway disease and hematopoiesis. Nat Med. (2014) 20:159–66. doi: 10.1038/nm.3444
26. Fonseca W, Malinczak C-A, Fujimura K, Li D, McCauley K, Li J, et al. Maternal gut microbiome regulates immunity to RSV infection in offspring. J Exp Med. (2021) 218:e20210235. doi: 10.1084/jem.20210235
27. Malhotra I, Dent A, Mungai P, Wamachi A, Ouma JH, Narum DL, et al. Can prenatal malaria exposure produce an immune tolerant phenotype?: a prospective birth cohort study in Kenya. PLoS Med. (2009) 6:e1000116. doi: 10.1371/journal.pmed.1000116
28. Lammie P, Hitch W, Walker E, Hightower A, Eberhard M. Maternal filarial infection as risk factor for infection in children. The Lancet. (1991) 337:1005–6. doi: 10.1016/0140-6736(91)92661-K
29. Ruben FL, Thompson DS. Cord blood lymphocyte in vitro responses to influenza A antigens after an epidemic of influenza A/port chalmers/73 (H3N2). Am J Obstet Gynecol. (1981) 141:443–7. doi: 10.1016/0002-9378(81)90609-8
30. Ruben FL, Winkelstein A, Sabbagha RE. In utero sensitization with influenza virus in man. Exp Biol Med. (1975) 149:881–3. doi: 10.3181/00379727-149-38918
31. Gee S, Chandiramani M, Seow J, Pollock E, Modestini C, Das A, et al. The legacy of maternal SARS-CoV-2 infection on the immunology of the neonate. Nat Immunol. (2021) 22:1490–502. doi: 10.1038/s41590-021-01049-2
32. Garcia-Flores V, Romero R, Xu Y, Theis KR, Arenas-Hernandez M, Miller D, et al. Maternal-fetal immune responses in pregnant women infected with SARS-CoV-2. Nat Commun. (2022) 13:1–20. doi: 10.1038/s41467-021-27745-z
33. Flannery DD, Gouma S, Dhudasia MB, Mukhopadhyay S, Pfeifer MR, Woodford EC, et al. Assessment of maternal and neonatal cord blood SARS-CoV-2 antibodies and placental transfer ratios. JAMA Pediatr. (2021) 175:594–600. doi: 10.1001/jamapediatrics.2021.0038
34. Lu-Culligan A, Chavan AR, Vijayakumar P, Irshaid L, Courchaine EM, Milano KM, et al. Maternal respiratory SARS-CoV-2 infection in pregnancy is associated with a robust inflammatory response at the maternal-fetal interface. Med. (2021) 2:591–610. doi: 10.1016/j.medj.2021.04.016
35. Argueta LB, Lacko LA, Bram Y, Tada T, Carrau L, Rendeiro AF, et al. Inflammatory responses in the placenta upon SARS-CoV-2 infection late in pregnancy. iScience. (2022) 25:104223. doi: 10.1016/j.isci.2022.104223
36. Debelenko L, Katsyv I, Chong AM, Peruyero L, Szabolcs M, Uhlemann A-C. Trophoblast damage with acute and chronic intervillositis: disruption of the placental barrier by severe acute respiratory syndrome coronavirus 2. Hum Pathol. (2021) 109:69–79. doi: 10.1016/j.humpath.2020.12.004
37. Fahmi A, Brügger M, Démoulins T, Zumkehr B, Esteves BIO, Bracher L, et al. SARS-CoV-2 can infect and propagate in human placenta explants. Cell Rep Med. (2021) 2:100456. doi: 10.1016/j.xcrm.2021.100456
38. Liong S, Oseghale O, To EE, Brassington K, Erlich JR, Luong R, et al. Influenza A virus causes maternal and fetal pathology via innate and adaptive vascular inflammation in mice. Proc Natl Acad Sci USA. (2020) 117:24964–73. doi: 10.1073/pnas.2006905117
39. Littauer EQ, Esser ES, Antao OQ, Vassilieva EV, Compans RW, Skountzou I. H1n1 influenza virus infection results in adverse pregnancy outcomes by disrupting tissue-specific hormonal regulation. PLoS Pathog. (2017) 13:e1006757. doi: 10.1371/journal.ppat.1006757
40. Van Campen H, Bishop JV, Abrahams VM, Bielefeldt-Ohmann H, Mathiason CK, Bouma GJ, et al. Maternal influenza A virus infection restricts fetal and placental growth and adversely affects the fetal thymic transcriptome. Viruses. (2020) 12:1003. doi: 10.3390/v12091003
41. Jacobsen H, Walendy-Gnirß K, Tekin-Bubenheim N, Kouassi NM, Ben-Batalla I, Berenbrok N, et al. Offspring born to influenza A virus infected pregnant mice have increased susceptibility to viral and bacterial infections in early life. Nat Commun. (2021) 12:1–14. doi: 10.1038/s41467-021-25220-3
42. Rasmussen SA, Jamieson DJ, Bresee JS. Pandemic influenza and pregnant women. Emerg Infect Dis. (2008) 14:95–100. doi: 10.3201/eid1401.070667
43. Helgertz J, Bengtsson T. The long-lasting influenza: the impact of fetal stress during the 1918 influenza pandemic on socioeconomic attainment and health in Sweden, 1968-2012. Demography. (2019) 56:1389–425. doi: 10.1007/s13524-019-00799-x
44. Mazumder B, Almond D, Park K, Crimmins EM, Finch CE. Lingering prenatal effects of the 1918 influenza pandemic on cardiovascular disease. J Dev Orig Health Dis. (2010) 1:26–34. doi: 10.1017/S2040174409990031
45. He J-R, Hirst JE, Tikellis G, Phillips GS, Ramakrishnan R, Paltiel O, et al. Common maternal infections during pregnancy and childhood leukaemia in the offspring: findings from six international birth cohorts. Int J Epidemiol. (2022) 51:769–77. doi: 10.1093/ije/dyab199
46. Edlow AG, Castro VM, Shook LL, Kaimal AJ, Perlis RH. Neurodevelopmental outcomes at 1 year in infants of mothers who tested positive for SARS-CoV-2 during pregnancy. JAMA Netw Open. (2022) 5:e2215787. doi: 10.1001/jamanetworkopen.2022.15787
47. Hanada S, Pirzadeh M, Carver KY, Deng JC. Respiratory viral infection-induced microbiome alterations and secondary bacterial pneumonia. Front Immunol. (2018) 9:2640. doi: 10.3389/fimmu.2018.02640
48. Ren Z, Wang H, Cui G, Lu H, Wang L, Luo H, et al. Alterations in the human oral and gut microbiomes and lipidomics in COVID-19. Gut. (2021) 70:1253–65. doi: 10.1136/gutjnl-2020-323826
49. Saleh J, Peyssonnaux C, Singh KK, Edeas M. Mitochondria and microbiota dysfunction in COVID-19 pathogenesis. Mitochondrion. (2020) 54:1–7. doi: 10.1016/j.mito.2020.06.008
50. Yildiz S, Mazel-Sanchez B, Kandasamy M, Manicassamy B, Schmolke M. Influenza A virus infection impacts systemic microbiota dynamics and causes quantitative enteric dysbiosis. Microbiome. (2018) 6:1–17. doi: 10.1186/s40168-017-0386-z
51. Al Khatib HA, Mathew S, Smatti MK, Eltai NO, Pathan SA, Al Thani AA, et al. Profiling of intestinal microbiota in patients infected with respiratory influenza A and B viruses. Pathogens. (2021) 10:761. doi: 10.3390/pathogens10060761
52. Okoko BJ, Wesumperuma LH, Ota MO, Pinder M, Banya W, Gomez SF, et al. The influence of placental malaria infection and maternal hypergammaglobulinemia on transplacental transfer of antibodies and IgG subclasses in a rural west african population. J Infect Dis. (2001) 184:627–32. doi: 10.1086/322808
53. Marchant A, Sadarangani M, Garand M, Dauby N, Verhasselt V, Pereira L, et al. Maternal immunisation: collaborating with mother nature. The Lancet Infect Dis. (2017) 17:e197–208. doi: 10.1016/S1473-3099(17)30229-3
54. Gonik B. The burden of respiratory syncytial virus infection in adults and reproductive-aged women. Glob health, Sci Pract. (2019) 7:515–20. doi: 10.9745/GHSP-D-19-00121
55. Lenahan JL, Englund JA, Katz J, Kuypers J, Wald A, Magaret A, et al. Human metapneumovirus and other respiratory viral infections during pregnancy and birth, Nepal. Emerg Infect Dis. (2017) 23:1341. doi: 10.3201/eid2308.161358
Keywords: COVID-19, SARS-CoV-2, influenza, pregnancy, fetal immunity, microbiota, placenta, inflammation
Citation: Dauby N and Flamand V (2022) From maternal breath to infant's cells: Impact of maternal respiratory infections on infants ‘immune responses. Front. Pediatr. 10:1046100. doi: 10.3389/fped.2022.1046100
Received: 16 September 2022; Accepted: 19 October 2022;
Published: 7 November 2022.
Edited by:
Carlo Pietrasanta, IRCCS Ca 'Granda Foundation Maggiore Policlinico Hospital, ItalyReviewed by:
Rita Carsetti, Bambino Gesù Children's Hospital (IRCCS), Italy© 2022 Dauby and Flamand. This is an open-access article distributed under the terms of the Creative Commons Attribution License (CC BY). The use, distribution or reproduction in other forums is permitted, provided the original author(s) and the copyright owner(s) are credited and that the original publication in this journal is cited, in accordance with accepted academic practice. No use, distribution or reproduction is permitted which does not comply with these terms.
*Correspondence: Nicolas Dauby bmljb2xhcy5kYXVieUB1bGIuYmU=
Specialty Section: This article was submitted to Pediatric Immunology, a section of the journal Frontiers in Pediatrics
Disclaimer: All claims expressed in this article are solely those of the authors and do not necessarily represent those of their affiliated organizations, or those of the publisher, the editors and the reviewers. Any product that may be evaluated in this article or claim that may be made by its manufacturer is not guaranteed or endorsed by the publisher.
Research integrity at Frontiers
Learn more about the work of our research integrity team to safeguard the quality of each article we publish.