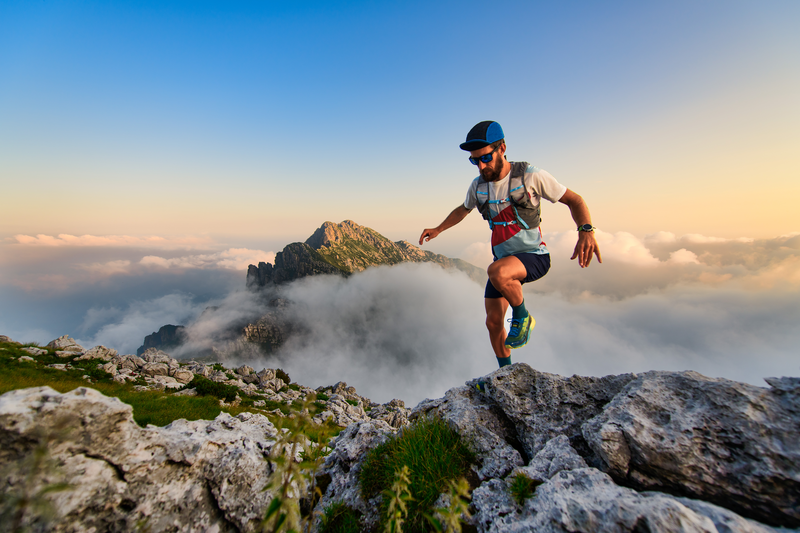
94% of researchers rate our articles as excellent or good
Learn more about the work of our research integrity team to safeguard the quality of each article we publish.
Find out more
REVIEW article
Front. Pediatr. , 21 October 2022
Sec. Pediatric Nephrology
Volume 10 - 2022 | https://doi.org/10.3389/fped.2022.1039024
This article is part of the Research Topic Insights in Pediatric Nephrology: 2022 View all 6 articles
Acute kidney injury (AKI) affects a large proportion of hospitalized children and increases morbidity and mortality in this population. Initially thought to be a self-limiting condition with uniformly good prognosis, we now know that AKI can persist and progress to acute kidney disease (AKD) and chronic kidney disease (CKD). AKI is presently categorized by stage of injury defined by increase in creatinine, decrease in eGFR, or decrease in urine output. These commonly used biomarkers of acute kidney injury do not change until the injury is well established and are unable to detect early stage of the disease when intervention is likely to reverse injury. The kidneys have the ability to compensate and return serum creatinine to a normal or baseline level despite nephron loss in the setting of AKI possibly masking persistent dysfunction. Though these definitions are important, classifying children by their propensity for progression to AKD and CKD and defining these risk strata by other factors besides creatinine may allow for better prognosis driven discussion, expectation setting, and care for our patients. In order to develop a classification strategy, we must first be able to recognize children who are at risk for AKD and CKD based on modifiable and non-modifiable factors as well as early biomarkers that identify their risk of persistent injury. Prevention of initial injury, prompt evaluation and treatment if injury occurs, and mitigating further injury during the recovery period may be important factors in decreasing risk of AKD and CKD after AKI. This review will cover presently used definitions of AKI, AKD, and CKD, recent findings in epidemiology and risk factors for AKI to AKD to CKD progression, novel biomarkers for early identification of AKI and AKI that may progress to CKD and future directions for improving outcome in children with AKI.
Acute kidney injury is a very common condition affecting about 5% of non-critically ill and 27% of critically ill children (1, 2). Children who have AKI have longer hospitalizations, prolonged need for mechanical ventilation, higher risk of developing CKD especially in the setting of repeated AKIs, and increased risk of mortality (1–6). The incidence of all causes of CKD as well as CKD after AKI are not well described and is likely underreported due to lack of routine screening (7). CKD is common in the US and it is associated with poor cardiovascular outcomes, anemia, dyslipidemias, altered growth and neurocognition in children which are all associated with poor health outcomes and quality of life in both children and adults (8, 9). AKI has long been recognized as a risk factor for CKD and recent literature has suggested that acute kidney disease (AKD), a term first introduced by the KDIGO group in 2012 and defined as persistent kidney dysfunction beyond 7 days and up to 3 months, may also be an independent risk factor for CKD (10, 11). Recent literature suggests that AKI, AKD, and CKD may be a continuation of the same ongoing disease process rather than separate entities; a graphic representation of this continuum is shown in Figure 1. The trajectory of kidney recovery after AKI and AKD is highly variable and therefore it is important to better understand what factors will increase a child's risk of non-recovery and to identify at risk children early. Studies have shown that children with rapid and sustained reversal of their kidney injury within 48 to 72 h tend to have better outcomes than those with persistent kidney injury irrespective of AKI stage (12–14). Persistent, as well as relapse of reversed kidney injury, is very common in hospitalized patients and is associated with longer hospital and ICU length of stay, need for kidney replacement therapy, and mortality compared to those with early sustained reversal of AKI (6). AKD has been found to be associated with new diagnosis of CKD as well as increased 1-year mortality in adults (15). Both AKI and AKD have been shown to be independent predictors of new CKD diagnosis and by better recognizing which children are at risk for progressing along the AKI-AKD-CKD path, providers can better prognosticate and implement kidney protective measures during this vulnerable period (16–20). Although AKI as a predictor of CKD has been well studied, it is more likely that a combination of injury severity, prolonged injury, and patient level risk factors play a role in progression of AKI to AKD and CKD. Recent discoveries in risk factors for AKI to AKD progression may provide insight into which children are at risk that may benefit from closer monitoring and care by a nephrologist. By better prognosticating which children may progress along this spectrum, nephrologists would better be able to discuss methods to mitigate further kidney injury and promote kidney recovery with families and providers.
Figure 1. Proposed model for continuum of acute kidney injury (AKI), acute kidney disease (AKD), and chronic kidney disease (CKD).
AKI has been well studied over the last several decades leading to significant changes in its definition and classification. The RIFLE criteria (Risk, Injury, Failure, Loss of Kidney Function, and End-stage Kidney Disease) were first proposed in 2002 the ADQI (Acute Disease Quality Initiative) group and published in 2004 (21). The RIFLE criteria were adapted for use in pediatrics (pRIFLE) and were able to accurately identify AKI in children (22). These criteria staged kidney injury severity based on serum creatinine, eGFR changes, and urine output and classified patients on two outcomes (loss of function and end-stage kidney disease). Although this criteria was able to identify patients with AKI well and to accurately prognosticate mortality it had limited evaluation in prospective studies (21). In 2005, the AKIN (Acute Kidney Injury Network) Working Group proposed a modification of the RIFLE criteria. The AKIN classification only considers a diagnosis of AKI once dehydration is corrected and urinary obstruction is ruled out. It relies on serum creatinine and urine output measurements over a 48-hour time frame and unlike the RIFLE criteria does not consider the eGFR. Much like the RIFLE criteria, the AKIN criteria was able to accurately identify patients with AKI but it had poor ability to predict in-hospital mortality (21). Most recently, the KDIGO (Kidney Disease Improving Global Outcomes) group proposed in 2007 and published in 2012 a new consensus classification system for AKI with the goal of harmonizing pediatric and adult AKI classifications (23). These definitions are now routinely used in both clinical practice and research. Table 1 shows the different criteria used by the RIFLE, pRIFLE, AKIN, and KDIGO groups for diagnosis of AKI.
AKD is a newer concept in the kidney disease lexicon and was proposed to better define the time period between AKI and CKD. The first concept was first suggested in 2012 and the first suggested definition and staging guidelines for AKD were recommended by the ADQI group in 2017. The ADQI group defined AKD as persistent stage 1 AKI or greater for >/= 7 days and staged it based on degree of serum creatinine increase from baseline (24). This definition was broadened in 2021 by the KDIGO group to include functional criteria for AKI as well as more subtle changes in eGFR and creatinine that may not meet the criteria for diagnosis of AKI (11). AKD can further be classified as AKD with or without AKI which includes those patients who don't meet creatinine or urine output criteria for AKI but do meet criteria for AKD (11, 24). Although no specific staging was presented in the consensus guidelines, it was proposed that AKD could be staged based on severity of eGFR decline as well as albuminuria (11). The ADQI and KDIGO definitions of AKD are outlined in Table 2.
Lastly, CKD is defined as an abnormality of kidney function defined by eGFR <60 ml/min/1.73 m2 or abnormal structure or function present for >90 days with implications on future health. Markers of kidney damage can include albuminuria, abnormal urine sediments, electrolyte or other abnormalities suggesting tubular disorder, abnormal kidney histology, abnormal kidney imaging, or history of kidney transplant. CKD, like AKI, is classified into stages by severity of the kidney dysfunction by eGFR and degree of albuminuria originally outlined by the Kidney Disease Outcomes Quality Initiative (KDOQI) group and most recently defined by the KDIGO group [Table 3] (25, 26).
The focus of the remainder of this review will be on the current data on the prevalence of AKD, and the risk factors for AKI-AKD and AKD-CKD progression. The review will also describe the state of knowledge on candidate biomarkers for disease progression following AKI and how we can leverage these biomarkers and clinical factors to identify children that are at risk of disease progression.
AKD is a relatively new concept and there are limited studies on the prevalence of AKD in pediatric populations. Table 4 outlines pediatric and adult studies of AKD epidemiology, risk factors, and outcomes. Pediatric studies in special populations have shown variable incidence of AKD ranging from 6.2% in children undergoing cardiopulmonary bypass, 13% in non-kidney solid organ transplant recipients, 15.6% in children with severe malaria infection, 35.3% in allogeneic hematopoietic stem cell recipients, and 42.3% in hospitalized children with AKI (16, 27–30). Adult studies have shown even more variability with incidence ranging from 3.8% in the general adult population of a single Canadian territory to 4.4%–74% in adults of special populations including those undergoing cardiac and non-cardiac procedure, chemotherapy, hematopoietic stem cell transplant recipients, those with AKI, heart failure, sepsis, cirrhosis, and SARS-CoV-2 infection (20). Hospitalized adults with AKI admitted to both the general floors as well as ICUs had high prevalence of AKD with most studies showing about one-fourth to half of all patients developing AKD (19, 31–40). Adults with cardiac diseases, post cardiac surgery, sepsis and cirrhosis all had high AKD prevalence suggesting hemodynamic status may play a significant role in sustained kidney injury and progression (17, 18, 37–46, 48). The wide variability in prevalence suggests there is a significant role for patient level and hospital acquired risk factors in the development of AKD after AKI.
Risk factors for AKD in children have not been widely studied. Few studies that have been published demonstrated a higher risk of AKD in children with higher stages of AKI with one study showing that stage of AKI predicted AKD in a graded manner (16, 27, 29). Though stage of AKI has been shown to be an important risk factor for progression to AKD, studies in adults and children have shown patient-level and acquired risk factors independently increased risk of AKD independent of AKI stage suggesting that patients with lower stages of AKI also deserve close evaluation for AKD risk. A study of children who have undergone hematopoietic stem cell transplant showed that older age, higher baseline eGFR and higher body mass index (BMI) were associated with increased risk of AKD (28). A study of a more general population of hospitalized children also showed that children with glomerulonephritis compared to other AKI etiologies were at higher risk for developing AKD after AKI suggesting that intrinsic kidney disease may also be an important predictor of progression to AKD (29). Although this is the only study assessing AKI etiology as a risk factor for AKD in pediatrics, a study in adults hospitalized with AKI showed similar results when evaluating intrinsic kidney injury and rates of AKD suggesting that AKI etiology is also an important consideration when evaluating a patient's prognosis for kidney recovery (31).
There have been more comprehensive studies of risk factors for AKD development in adults as outlined in Table 4. In hospitalized adults with AKI, age, gender, intrinsic kidney disease, AKI stage, oliguria, anuria, nephrotoxin exposure, early need for dialysis, BUN level, diabetes, anemia, and respiratory failure have been associated with AKD development (31–33). In adults admitted to an ICU setting age, male sex, African American race, sepsis, lower baseline eGFR and CKD, anemia, and lower ejection fraction have been shown to be risk factors for AKD (35, 39, 45). In the setting of cardiac disease including myocardial infarction, heart failure, and cardiac surgeries, increased risk of AKD was associated with age, female sex, AKI stage, diabetes, baseline CKD, hemoglobin, albumin, BNP, inotrope use, and outpatient loop diuretic use (17, 41, 43, 47). There are several established risk factors for developing AKD in adults undergoing non-cardiac procedures including age, intraoperative hypotension, and coronary artery disease (46, 52). In addition, other risk factors in adults hospitalized with COVID include hypertension, baseline CKD ≥ stage 3, low hemoglobin, low CRP, nephrotoxin exposure, and congestive heart failure have been associated with AKD (53–55). These studies demonstrate that there are some risk factors for AKD that are common to different populations but in general, each special population has its own unique patient-level risks. This highlights the need for comprehensive and large cohort studies to define the risk factors associated with AKD and develop a better understanding of how these risk factors play a role in prolonged kidney injury. No comprehensive studies have been completed yet that have assessed a wide range of patient-level risk factors for AKD in children with AKI.
Outcome studies in both adults and children have shown that AKD and not AKI stage is a significant risk factor for new onset CKD, need for kidney replacement therapy, and mortality further underscoring the importance of understanding patient level risk factors associated with development of CKD following AKI. The variability in AKD epidemiology suggests a significant relationship between AKI stage, patient level pre-existing risk factors, modifiable risk factors including medication exposures and hemodynamic status, as well as etiology of AKI. A risk prediction tool utilizing these different exposure patient-level and iatrogenic criteria may better allow for clinical risk stratification and identification of patients who are likely to develop AKD. This significant variability in prevalence of AKD as well as risk factors is shown in Table 4 and further emphasizes the fact that different clinical tools may be needed for separate populations.
The prevalence of CKD is significantly higher in adults with AKD than those without and prevalence has also been shown to increase with increasing AKD stage as defined by the ADQI group (17–19, 33, 38). One study demonstrated that AKD was associated with higher risk of new CKD, progression of pre-existing CKD, and ESKD (20). However, prevalence of CKD after AKD is not well studied in children with only one study showing the odds of CKD after AKD was 29-fold higher (16). More studies are needed in children to understand the risk of CKD after AKD and to assess which patient level risk factors increase the risk of progression from AKD to CKD.
Common biomarkers of AKI including creatinine, cystatin C, and urine output lack the ability for early kidney injury detection. Many other biomarkers have been studied with the goal of early identification of AKI but there have been minimal studies assessing their value as predictive markers for progression of AKD to CKD. A summary of well-studied candidate biomarkers is reviewed here and reviewed in Table 5.
Neutrophil gelatinase associated lipoprotein (NGAL) is a 25 kDa protein that is part of the lipocalin family. NGAL prevents iron uptake by bacteria allowing it to play a role in the innate immune system (56). Within the kidneys, NGAL is thought to be produced by the thick ascending loop of Henle and the intercalated cells of the collecting duct. Kidney damage is thought to lead to decreased reabsorption by the proximal tubule which may lead to increased urinary NGAL (57). Ischemic and toxic AKI highly upregulate NGAL expression and there is an increase in detectable levels as early as 2–3 h after injury and up to 5 days after initial injury (58, 59). The ability to use NGAL for early diagnosis of AKI was first studied by Mishra et al. in 2005 who noted that in 71 children undergoing cardiopulmonary bypass, a well-known risk factor for AKI, urine, and serum NGAL quickly rose within 2 h after the bypass procedure in children who later developed AKI. The rise in NGAL levels was rapid compared to the delayed rise in serum creatinine that occurred about 1–3 days after the procedure. NGAL was also found to be elevated, but to a lesser extent, in those without creatinine based AKI diagnosis, identifying possible subclinical injury (59). This highlighted the value of NGAL in the early diagnosis of AKI. Since then NGAL has been studied in children and adults that are critically ill, undergoing cardiac surgery, transplant, and presenting to the emergency department (60). Though NGAL has been shown to be a relatively good early predictor of kidney disease in these populations, there is significant variability in the AUC-ROC making it difficult to establish set cut offs for the diagnosis of kidney injury. There have been limited studies on the ability of NGAL to predict AKD with one study showing that higher concentration of NGAL was independently associated with longer duration of AKI (≥ 7 days) (61) and a second study showing that children with a NGAL level ≥300 ng/ml at the time of admission were more likely to develop AKD (27). Serum NGAL levels have been shown to be elevated in children and adults with CKD and that it may be a predictor of CKD progression (62, 63) possibly making it a good biomarker of persistent kidney damage. It is speculated that NGAL levels increase in proportion to tubular damage, neutrophil activation or inflamed vasculature which may explain elevated levels in CKD. Decreased clearance in the setting of CKD is hypothesized to prevent proximal tubule reabsorption of NGAL which may lead to increased serum levels (62). More studies are needed to assess the ability of early NGAL elevation to predict risk of AKD and CKD.
Kidney Injury Molecule-1 (KIM-1) is a 39 kDa transmembrane protein with an immunoglobulin domain and mucin domain that is expressed in the proximal tubule (64, 65). Expression of KIM-1 is upregulated in the setting of ischemia-reperfusion injury and the ectodomain of KIM-1 is shed from cells and can be detected in the urine and blood of patients with AKI within hours of kidney injury (66–71). KIM-1 has also been shown to be a predictor of AKI in the setting of nephrotoxin exposure (72, 73). Elevated urine KIM-1 levels can persist after a patient has had apparent recovery from AKI suggesting it is a marker of subclinical and ongoing injury (74). It is thought that KIM-1 has better diagnostic accuracy in pediatric patients than adults due to higher level of comorbidities in the latter as well as normal increase in KIM-1 levels with age (67, 75). Similar to NGAL, there are not well established set cut offs for KIM-1 for the diagnosis of kidney damage (68). KIM-1 is also a sensitive biomarker for CKD because it is thought to play a role in proinflammatory state and kidney fibrosis (76). The usefulness of KIM-1 in the diagnosis and prediction of AKD is unknown and further studies are warranted. A summary of KIM-1 and its potential role as an early biomarker of long-term injury is presented in Table 5.
Interleukin-18 (IL-18) is a proinflammatory cytokine (77). Within the kidney it is expressed in the tubular epithelial cells and is stored intracellularly as its inactive form, pro-IL-18 (78). Once cleaved by caspase-1, it is secreted as its biologically active form IL-18 which acts on the IL-18 receptor to cause downstream upregulation of pro-inflammatory gene transcription (78). IL-18 levels have been shown to be elevated prior to AKI development (79, 80) and are predictors of AKI progression in the setting of critical illness (81–84). Levels increase about 6 h after injury and peak at about 12 h (71). In children, IL-18 has been demonstrated to be a predictor of AKI in preterm infants but there are no well-established cutoffs for diagnosis of AKI (85–88). The AUC-ROC varies significantly and IL-18 has low sensitivity but high specificity indicating that some patients with AKI may not have elevated IL-18 levels (89). IL-18 can remain elevated for 41 months to 7 years in children with AKI post cardiac surgery and a study of adult patients showed that significantly elevated IL-18 levels were associated with longer term eGFR decline suggesting that it may be a marker of ongoing damage (90–92). Lastly, one study of adults admitted to a cardiac care unit showed that IL-18 was independently associated with development of AKD (45). In this study by Chen et al., patients with AKD had mean serum IL-18 levels of 454 ng/ml (SD 36 ng/ml) compared to 256 ng/ml (SD 15 ng/ml) in the non-AKD group and based on this, a possible cutoff value of 400 ng/ml was suggested as a marker for increased risk of AKD, this finding warrants further studies (45). IL-18 is also upregulated in CKD and may play a role in tubulointerstitial fibrosis by inducing tubuloepithelial cell injury and activation (93). These findings are summarized in Table 5. Given this finding of more elevated levels in those with AKD as well as its role as a marker of ongoing inflammation, IL-18 may be a good early predictor of who is at risk of persistent kidney disease.
Tissue inhibitor of metalloproteinases 2 (TIMP2) and insulin-like growth factor-binding protein 7 (IGFBP7) are involved in cell-cycle arrest preventing cells from dividing until damage can be repaired and therefore are markers of cell stress (94). They are upregulated in ischemia, sepsis, oxidative stress, and exposure to toxins (95). These biomarkers can be found in the urine within hours of kidney injury (96–98). The combination of these two biomarkers was shown to have improved risk stratification and predicts AKI progression in critically ill patients (81, 96, 99–101). When paired with a clinical model they have improved risk prediction for AKI (96). These biomarkers have been shown to be good predictors of AKI even in the setting of common comorbid conditions including CKD, congestive heart failure, and diabetes mellitus (102). In critically ill patients there was a seven fold increase in risk of AKI in patients with a [TIMP-2]× [IGFBP7] value of >0.3 ng/ml when compared to those with a score ≤0.3 ng/ml (99) making this a possible acceptable cut off for assessing for increased AKI risk in critically ill patients. The utility of this biomarker and relative cut offs for AKI risk prediction are variable in non-critically ill populations (103). Conflicting studies have shown variable utility of [TIMP-2]× [IGFBP7] in predicting persistent kidney injury beyond 3–5 days. A study of adults admitted to a single ICU demonstrated that the ([TIMP-2]× [IGFPB7]/1000) measured at hours 0, 4, 12, and 24 of ICU admission had poor ability to predict persistent (non-recovery at 5 days) compared to transient AKI (104). Another study of adults admitted to 11 different ICUs with sepsis demonstrated that patients with higher ([TIMP-2]× [IGFPB7]/1000) values early in their course were more likely to develop persistent AKI (non-recovery at 3 days) but that the short term changes in the biomarkers over the first 24 h failed to similarly identify at risk patients (105). Lastly, a retrospective cohort study of adults also admitted to an ICU who had ([TIMP-2]× [IGFPB7]/1000) and procalcitonin measured at the time of admission demonstrated that those with a positive double biomarker test had a higher risk of developing AKD (106). These studies done by Titeca-Beauport et al. and Godi et al. suggest that early, rather than sustained elevation or trajectory, of [TIMP-2]× [IGFBP7] may be a better early marker of AKD risk. Lastly, this biomarker in mouse models has also been shown to predict predisposition for repeat AKI episodes, and in a study of adults, elevated [TIMP-2]× [IGFBP7] at the time of AKI was predictive of CKD development even after complete recovery from AKI (107, 108). The possible utility of [TIMP-2]× [IGFBP7] for early risk stratification of at-risk patients is summarized in Table 5.
Tumor necrosis factor receptor-1 (TNFR-1) and tumor necrosis factor receptor-2 (TNFR-2) are soluble markers of neutrophil-endothelial cell interaction (109) and play a role in apoptosis (110). TNFR-1 is expressed in most cells and is activated by membrane bound and soluble TNFα whereas TNFR-2 is present only in endothelial cells, fibroblasts, neurons, myeloid cells, and T- and B-cell subsets and is only activated by membrane bound TNFα. As a result of cellular activation by TNFα, alternative splicing of mRNA occurs resulting in the production of soluble TNFR which is detectable in the blood (110). Serum TNFR-1 and -2 levels have been shown to be independent predictors of AKI, severe AKI, need for kidney replacement therapy, and mortality and elevated TNFR-1 has been shown to be a marker of slower kidney recovery (109, 111–115). In a study of patients with COVID-19, patients were found to have increasing levels of TNFR-1 and TNFR-2 with increasing stage of AKI (114). Lastly, TNFR-1 and-2 have been shown to be elevated in adults with diabetes associated proteinuria, hypertension, CKD and ESKD and more recently in non-diabetic adults as well (116–119). TNFR levels are associated with degree of interstitial fibrosis in IgA nephropathy likely related to TNFα release from mesangial cells and subsequent inflammation (120). This may indicate that elevated levels of soluble TNFR-1 and -2 in the setting of CKD may be indicators of ongoing inflammation within the kidney. No studies have shown a clear timing of detectable levels, peak levels, or cutoff value for identifying AKI or increased risk of AKD. Known data regarding these two biomarkers are summarized in Table 5. TNFR-1 and TNFR-2 have not been studied for its ability to predict AKD, but it may be a good candidate biomarker to monitor overtime to assess for ongoing inflammation and AKD risk.
Liver fatty acid binding protein (L-FABP) is found in the cytoplasm of proximal tubule epithelial cells and is involved in free fatty acid transportation (121). This segment of the nephron is highly dependent on fatty acid metabolism for energy production and normal cellular transport functions. L-FABP levels have been shown to be increased in the setting of kidney hypoxia and ischemia (121, 122). Mouse models have shown detectable increase in L-FABP levels within 2 h of ischemia-reperfusion or nephrotoxic medication exposure with peak levels at 3 h. From there L-FABP levels steadily decreased but remained detectable 24 h after reperfusion (123). L-FABP levels have been found to be elevated in adults with various etiologies of AKI as well as in children undergoing cardiac surgery in whom levels increase as early as 4 h after their procedure (124–126). Elevated levels have also been associated with increased risk of AKI non-survival and development of CKD (125, 127). Similar to TNFR-1 and -2, there are no established cutoff values though a urine value of greater than 17.4 micrograms/gram creatinine has been associated with more significant deterioration of kidney function in patients with CKD (127). Though it has not been studied for its ability to predict risk of AKD, it may represent a good biomarker as it may indicate ongoing abnormalities in metabolism and energy production; further studies to delineate its role in predicting AKD and CKD risk are needed.
Severe AKI and repeated AKIs have long been known to be a risk factor for CKD but recent literature suggests that AKD may be better predictor for disease progression. Limited studies in children and many studies in adults have shown that risk of AKD is dependent on stage and duration of AKI as well as a myriad of patient level risk factors. AKD itself increases the risk of new onset CKD, progression of CKD, development of ESKD, and mortality. By better understanding which pre-existing and iatrogenic factors place children with AKI at risk for longer duration of kidney injury we can help better prognosticate their likelihood of AKD and CKD after AKI. Early identification of at-risk children can allow for better kidney centered care and avoidance of repeated injuries and factors that promote irreversible kidney injury.
We propose that a combination of a clinical tool which identifies AKD and CKD risk by clinical factors in conjunction with biomarkers of early and prolonged kidney injury would best allow nephrologists to identify children who are at risk for persistent kidney injury. A proposed schematic for biomarker and clinical tool-based care is shown in Figure 2 with suggested follow up, counseling, and monitoring adapted and extrapolated from the recent ADQI meeting report (128). This model has been extrapolated to include monitoring for development of AKD as there are no specific guidelines or models in the literature for this. As shown in this review, there is significant heterogeneity in the risk of kidney disease progression as well as risk factors among both children and adults. In this setting, different clinical tools tailored to individual populations may be more suitable rather than one universal clinical risk prediction tool. We have suggested candidate biomarkers in this article which should be better studied for their ability to predict risk of progression to AKD and CKD. These biomarkers tend to be altered much sooner than classic markers of AKI including serum creatinine, urine output, and cystatin C. Many of these biomarkers have been shown to be persistently elevated long after AKI has resolved underscoring the importance of identifying molecular markers that can reveal subclinical and prolonged kidney dysfunction. There are limited studies that have shown that elevation of these biomarkers in the AKI period may aid in identification of children who are at risk for AKD. Further studies are needed to clarify if early changes in biomarkers can be used in prognostication.
Figure 2. Proposed schematic for biomarker and clinical tool-based prognostication for AKI-AKD-CKD progression and follow up nephrology care post AKI.
Although it is important to classify AKI by stage, it is also important to recognize that this alone does not explain a child's risk of developing CKD and its sequelae. Other factors must be taken into consideration when assessing the overall morbidity and mortality associated with a single or even repeated episodes of AKI. It may be more appropriate to consider how the child's future kidney health will be affected by their AKI episode and for this we must better understand the other risk factors that promote AKI-AKD-CKD progression. There are no well-studied therapeutic options for preventing kidney disease progression after AKI but by better understanding which children are at risk we may facilitate the study of disease modifying behaviors and therapies. Despite all that is known, more studies are needed for early identification of at-risk children so we may better mitigate adverse outcomes of AKI in this vulnerable population.
RAG and MP conceptualized the manuscript topic. MP wrote the manuscript. All authors contributed to the article and approved the submitted version.
MP was supported by National Institutes of Health Nephrology Training Grant (5T32DK007731-24).
The authors declare that the research was conducted in the absence of any commercial or financial relationships that could be construed as a potential conflict of interest.
All claims expressed in this article are solely those of the authors and do not necessarily represent those of their affiliated organizations, or those of the publisher, the editors and the reviewers. Any product that may be evaluated in this article, or claim that may be made by its manufacturer, is not guaranteed or endorsed by the publisher.
AKI, Acute kidney injury; AKD, Acute kidney disease; CKD, Chronic kidney disease; RIFLE, Risk, Injury, Failure, Loss of Kidney Function, and End-stage Kidney Disease; pRIFLE, Pediatric Risk, Injury, Failure, Loss of Kidney Function, and End-stage Kidney Disease; AKIN, Acute Kidney Injury Network; ADQI, Acute Disease Quality Initiative; KDIGO, Kidney Disease Improving Global Outcomes; KDOQI, Kidney Disease Outcomes Quality Initiative; eGFR, Estimated glomerular filtration rate; NGAL, Neutrophil gelatinase associated lipoprotein; KIM-1, Kidney Injury Molecule-1; IL-18, Interleukin-18; TIMP2, Tissue inhibitor of metalloproteinases 2; IGFBP7, Insulin-like growth factor-binding protein 7; TNFR-1, Tumor necrosis factor receptor 1; TNFR-2, Tumor necrosis factor receptor 2; L-FABP, Liver fatty acid binding protein
1. McGregor TL, Jones DP, Wang L, Danciu I, Bridges BC, Fleming GM, et al. Acute kidney injury incidence in noncritically ill hospitalized children, adolescents, and young adults: a retrospective observational study. Am J Kidney Dis. (2016) 67(3):384–90. doi: 10.1053/j.ajkd.2015.07.019
2. Kaddourah A, Basu RK, Bagshaw SM, Goldstein SL, AWARE Investigators. Epidemiology of acute kidney injury in critically ill children and young adults. N Engl J Med. (2017) 376(1):11–20. doi: 10.1056/NEJMoa1611391
3. Williams DM, Sreedhar SS, Mickell JJ, Chan JCM. Acute kidney failure: a pediatric experience over 20 years. Arch Pediatr Adolesc Med. (2002) 156(9):893–900. doi: 10.1001/archpedi.156.9.893
4. Sutherland SM, Ji J, Sheikhi FH, Widen E, Tian L, Alexander SR, et al. AKI In hospitalized children: epidemiology and clinical associations in a national cohort. Clin J Am Soc Nephrol. (2013) 8(10):1661–9. doi: 10.2215/CJN.00270113
5. Alkandari O, Eddington KA, Hyder A, Gauvin F, Ducruet T, Gottesman R, et al. Acute kidney injury is an independent risk factor for pediatric intensive care unit mortality, longer length of stay and prolonged mechanical ventilation in critically ill children: a two-center retrospective cohort study. Crit Care. (2011) 15(3):R146. doi: 10.1186/cc10269
6. Kellum JA, Sileanu FE, Bihorac A, Hoste EAJ, Chawla LS. Recovery after acute kidney injury. Am J Respir Crit Care Med. (2017) 195(6):784–91. doi: 10.1164/rccm.201604-0799OC
7. Harambat J, van Stralen KJ, Kim JJ, Tizard EJ. Epidemiology of chronic kidney disease in children. Pediatr Nephrol. (2012) 27(3):363–73. doi: 10.1007/s00467-011-1939-1
8. NAPRTCS. North American Pediatric Renal Trials and Collaborative Study (NAPRTCS) 2008 Annual Report. Rockville, MD: The EMMES Corporation; 2008.
9. Wong CJ, Moxey-Mims M, Jerry-Fluker J, Warady BA, Furth SL. CKid (CKD in children) prospective cohort study: a review of current findings. Am J Kidney Dis. (2012) 60(6):1002–11. doi: 10.1053/j.ajkd.2012.07.018
11. Lameire NH, Levin A, Kellum JA, Cheung M, Jadoul M, Winkelmayer WC, et al. Harmonizing acute and chronic kidney disease definition and classification: report of a kidney disease: improving global outcomes (KDIGO) consensus conference. Kidney Int. (2021) 100(3):516–26. doi: 10.1016/j.kint.2021.06.028
12. Brown JR, Kramer RS, Coca SG, Parikh CR. Duration of acute kidney injury impacts long-term survival after cardiac surgery. Ann Thorac Surg. (2010) 90(4):1142–8. doi: 10.1016/j.athoracsur.2010.04.039
13. Coca SG, King JT, Rosenthal RA, Perkal MF, Parikh CR. The duration of postoperative acute kidney injury is an additional parameter predicting long-term survival in diabetic veterans. Kidney Int. (2010) 78(9):926–33. doi: 10.1038/ki.2010.259
14. Kellum JA, Sileanu FE, Murugan R, Lucko N, Shaw AD, Clermont G. Classifying AKI by urine output versus serum creatinine level. J Am Soc Nephrol. (2015) 26(9):2231–8. doi: 10.1681/ASN.2014070724
15. Wang H, Lambourg E, Guthrie B, Morales DR, Donnan PT, Bell S. Patient outcomes following AKI and AKD: a population-based cohort study. BMC Med. (2022) 20(1):229. doi: 10.1186/s12916-022-02428-8
16. Patel M, Heipertz A, Joyce E, Kellum JA, Horvat C, Squires JE, et al. Acute kidney disease predicts chronic kidney disease in pediatric non-kidney solid organ transplant patients. Pediatr Transplant. (2021) 6:e14172. doi: 10.1111/petr.14172
17. Kofman N, Margolis G, Gal-Oz A, Letourneau-Shesaf S, Keren G, Rozenbaum Z, et al. Long-term renal outcomes and mortality following renal injury among myocardial infarction patients treated by primary percutaneous intervention. Coron Artery Dis. (2019) 30(2):87–92. doi: 10.1097/MCA.0000000000000678
18. Tonon M, Rosi S, Gambino CG, Piano S, Calvino V, Romano A, et al. Natural history of acute kidney disease in patients with cirrhosis. J Hepatol. (2021) 74(3):578–83. doi: 10.1016/j.jhep.2020.08.037
19. Lertussavavivat T, Kulvichit W, Peerapornratana S, Lumlertgul N, Bhumitrakul J, Tungsanga K, et al. The epidemiology and long-term outcomes of acute kidney disease in a resource-limited setting. J Nephrol. (2022) 1–10. doi: 10.1007/s40620-022-01328-7
20. James MT, Levey AS, Tonelli M, Tan Z, Barry R, Pannu N, et al. Incidence and prognosis of acute kidney diseases and disorders using an integrated approach to laboratory measurements in a universal health care system. JAMA Netw Open. (2019) 2(4):e191795. doi: 10.1001/jamanetworkopen.2019.1795
21. Lopes JA, Jorge S. The RIFLE and AKIN classifications for acute kidney injury: a critical and comprehensive review. Clin Kidney J. (2013) 6(1):8–14. doi: 10.1093/ckj/sfs160
22. Akcan-Arikan A, Zappitelli M, Loftis LL, Washburn KK, Jefferson LS, Goldstein SL. Modified RIFLE criteria in critically ill children with acute kidney injury. Kidney Int. (2007) 71(10):1028–35. doi: 10.1038/sj.ki.5002231
23. Khwaja A. KDIGO Clinical practice guidelines for acute kidney injury. Nephron Clin Pract. (2012) 120(4):c179–84. doi: 10.1159/000339789
24. Chawla LS, Bellomo R, Bihorac A, Goldstein SL, Siew ED, Bagshaw SM, et al. Acute kidney disease and renal recovery: consensus report of the acute disease quality initiative (ADQI) 16 workgroup. Nat Rev Nephrol. (2017) 13(4):241–57. doi: 10.1038/nrneph.2017.2
25. Kidney Disease: Improving Global Outcomes (KDIGO) CKD Workgroup. KDIGO 2012 Clinical practice guideline for the evaluation and management of chronic kidney disease. Kidney Inter Suppl. (2013) 3:1–150. doi: 10.1038/kisup.2012.73
26. National Kidney Foundation. KDOQI clinical practice guidelines for chronic kidney disease: evaluation, classification, and stratification. Am J Kidney Dis. (2002) 39:S1–266. doi: 10.1053/ajkd.2002.29865
27. Namazzi R, Batte A, Opoka RO, Bangirana P, Schwaderer AL, Berrens Z, et al. Acute kidney injury, persistent kidney disease, and post-discharge morbidity and mortality in severe malaria in children: a prospective cohort study. EClinicalMedicine. (2022) 44:101292. doi: 10.1016/j.eclinm.2022.101292
28. Daraskevicius J, Azukaitis K, Dziugeviciute-Tupko J, Peciulyte M, Planciunaite R, Vaitkeviciene G, et al. Phenotypes and baseline risk factors of acute kidney injury in children after allogeneic hematopoietic stem cell transplantation. Front Pediatr. (2020) 8:499. doi: 10.3389/fped.2020.00499
29. Deng Y-H, Yan P, Zhang N-Y, Luo X-Q, Wang X-F, Duan S-B. Acute kidney disease in hospitalized pediatric patients with acute kidney injury in China. Front Pediatr. (2022) 10:885055. doi: 10.3389/fped.2022.885055
30. LoBasso M, Schneider J, Sanchez-Pinto LN, Del Castillo S, Kim G, Flynn A, et al. Acute kidney injury and kidney recovery after cardiopulmonary bypass in children. Pediatr Nephrol. (2022) 37(3):659–65. doi: 10.1007/s00467-021-05179-5
31. Xiao Y-Q, Cheng W, Wu X, Yan P, Feng L-X, Zhang N-Y, et al. Novel risk models to predict acute kidney disease and its outcomes in a Chinese hospitalized population with acute kidney injury. Sci Rep. (2020) 10(1):15636. doi: 10.1038/s41598-020-72651-x
32. Zhao H, Liang L, Liu Z, Qiao Y, Liu D, Liu Z. Diabetes mellitus as a risk factor for progression from acute kidney injury to aute kidney disease: a specific prediction model. Diabetes Metab Syndr Obes. (2021) 14:2367–79. doi: 10.2147/DMSO.S307776
33. Hsu P-C, Liu C-H, Lee W-C, Wu C-H, Lee C-T, Su C-H, et al. Predictors of acute kidney disease severity in hospitalized patients with acute kidney injury. Biomedicines. (2022) 10(5):1081. doi: 10.3390/biomedicines10051081
34. Nagata K, Horino T, Hatakeyama Y, Matsumoto T, Terada Y, Okuhara Y. Effects of transient acute kidney injury, persistent acute kidney injury and acute kidney disease on the long-term renal prognosis after an initial acute kidney injury event. Nephrology (Carlton). (2021) 26(4):312–8. doi: 10.1111/nep.13831
35. Andonovic M, Traynor JP, Shaw M, Sim MAB, Mark PB, Puxty KA. Short- and long-term outcomes of intensive care patients with acute kidney disease. EClinicalMedicine. (2022) 44:101291. doi: 10.1016/j.eclinm.2022.101291
36. Rimes-Stigare C, Ravn B, Awad A, Torlén K, Martling C-R, Bottai M, et al. Creatinine- and cystatin C-based incidence of chronic kidney disease and acute kidney disease in AKI survivors. Crit Care Res Pract. (2018) 2018:7698090. doi: 10.1155/2018/7698090
37. Gameiro J, Carreiro C, Fonseca JA, Pereira M, Jorge S, Gouveia J, et al. Acute kidney disease and long-term outcomes in critically ill acute kidney injury patients with sepsis: a cohort analysis. Clin Kidney J. (2021) 14(5):1379–87. doi: 10.1093/ckj/sfaa130
38. Flannery AH, Li X, Delozier NL, Toto RD, Moe OW, Yee J, et al. Sepsis-associated acute kidney disease and long-term kidney outcomes. Kidney Med. (2021) 3(4):507–514.e1. doi: 10.1016/j.xkme.2021.02.007
39. Peerapornratana S, Priyanka P, Wang S, Smith A, Singbartl K, Palevsky PM, et al. Sepsis-associated acute kidney disease. Kidney Int Rep. (2020)5:839–50. doi: 10.1016/j.ekir.2020.03.005.32518866
40. He J, Lin J, Duan M. Application of machine learning to predict acute kidney disease in patients with sepsis associated acute kidney injury. Front Med (Lausanne). (2021) 8:792974. doi: 10.3389/fmed.2021.792974
41. Chen J-J, Lee T-H, Kuo G, Yen C-L, Chen S-W, Chu P-H, et al. Acute kidney disease after acute decompensated heart failure. Kidney Int Rep. (2022) 7(3):526–36. doi: 10.1016/j.ekir.2021.12.033
42. Hsu C-K, Wu I-W, Chen Y-T, Tsai T-Y, Tsai F-C, Fang J-T, et al. Acute kidney disease stage predicts outcome of patients on extracorporeal membrane oxygenation support. PLoS ONE. (2020) 15(4):e0231505. doi: 10.1371/journal.pone.0231505
43. Mizuguchi KA, Huang C-C, Shempp I, Wang J, Shekar P, Frendl G. Predicting kidney disease progression in patients with acute kidney injury after cardiac surgery. J Thorac Cardiovasc Surg. (2018) 155(6):2455–2463.e5. doi: 10.1016/j.jtcvs.2018.01.093
44. Matsuura R, Iwagami M, Moriya H, Ohtake T, Hamasaki Y, Nangaku M, et al. The clinical course of acute kidney disease after cardiac surgery: a retrospective observational study. Sci Rep. (2020) 10(1):6490. doi: 10.1038/s41598-020-62981-1
45. Chen Y-T, Jenq C-C, Hsu C-K, Yu Y-C, Chang C-H, Fan P-C, et al. Acute kidney disease and acute kidney injury biomarkers in coronary care unit patients. BMC Nephrol. (2020) 21(1):207. doi: 10.1186/s12882-020-01872-z
46. Shaw AD, Khanna AK, Smischney NJ, Shenoy AV, Boero IJ, Bershad M, et al. Intraoperative hypotension is associated with persistent acute kidney disease after noncardiac surgery: a multicentre cohort study. Br J Anaesth. (2022) 129(1):13–21. doi: 10.1016/j.bja.2022.03.027
47. Chen L, Hong L, Ma A, Chen Y, Xiao Y, Jiang F, et al. Intraoperative venous congestion rather than hypotension is associated with acute adverse kidney events after cardiac surgery: a retrospective cohort study. Br J Anaesth. (2022) 128(5):785–95. doi: 10.1016/j.bja.2022.01.032
48. Liu J, Li Q, Chen W, Huang H, Yu Y, Wang B, et al. Incidence and mortality of acute kidney disease following coronary angiography: a cohort study of 9223 patients. Int Urol Nephrol. (2022) 54:2433–40. doi: 10.1007/s11255-022-03110-x
49. Fernandes ARDS, de Brito GA, Baptista AL, Andrade LAS, Imanishe MH, Pereira BJ. The influence of acute kidney disease on the clinical outcomes of patients who received cisplatin, carboplatin, and oxaliplatin. Health Sci Rep. (2022) 5(1):e479. doi: 10.1002/hsr2.479
50. Rachman A, Shatri H, Salamat R. Correlation between higher cumulative dose of cisplatin for concurrent chemoradiation and acute kidney disease incidence among nasopharyngeal carcinoma patients: a comparative study. Int J Gen Med. (2021) 14:10527–39. doi: 10.2147/IJGM.S343644
51. Mima A, Tansho K, Nagahara D, Tsubaki K. Incidence of acute kidney disease after receiving hematopoietic stem cell transplantation: a single-center retrospective study. PeerJ. (2019) 7:e6467. doi: 10.7717/peerj.6467
52. Wu K-T, Chen C-Y, Chen B, Wang J-W, Lin P-C, Yen S-H. The incidence and risk factors of acute kidney disease after total knee arthroplasty with early postoperative volume supplement. Biomed Res Int. (2018) 2018:8718545. doi: 10.1155/2018/8718545
53. Marques F, Gameiro J, Oliveira J, Fonseca JA, Duarte I, Bernardo J, et al. Acute kidney disease and mortality in acute kidney injury patients with COVID-19. J Clin Med. (2021) 10(19):4599. doi: 10.3390/jcm10194599
54. Sindhu C, Prasad P, Elumalai R, Matcha J. Clinical profile and outcomes of COVID-19 patients with acute kidney injury: a tertiary centre experience from South India. Clin Exp Nephrol. (2022) 26(1):36–44. doi: 10.1007/s10157-021-02123-7
55. Sarwal A, Gomez E, Perez-Gutierrez V, Carlos A, Afzal A, Sivakumar M, et al. Renal recovery after acute kidney injury in a minority population of hospitalized COVID-19 patients: a retrospective cohort study. Medicine (Baltimore). (2022) 101(11):e28995. doi: 10.1097/MD.0000000000028995
56. Yang J, Goetz D, Li JY, Wang W, Mori K, Setlik D, et al. An iron delivery pathway mediated by a lipocalin. Mol Cell. (2002) 10(5):1045–56. doi: 10.1016/S1097-2765(02)00710-4
57. Schmidt-Ott KM, Mori K, Li JY, Kalandadze A, Cohen DJ, Devarajan P, et al. Dual action of neutrophil gelatinase-associated lipocalin. J Am Soc Nephrol. (2007) 18(2):407–13. doi: 10.1681/ASN.2006080882
58. Mishra J, Ma Q, Prada A, Mitsnefes M, Zahedi K, Yang J, et al. Identification of neutrophil gelatinase-associated lipocalin as a novel early urinary biomarker for ischemic renal injury. J Am Soc Nephrol. (2003) 14(10):2534–43. doi: 10.1097/01.asn.0000088027.54400.c6
59. Mishra J, Dent C, Tarabishi R, Mitsnefes MM, Ma Q, Kelly C, et al. Neutrophil gelatinase-associated lipocalin (NGAL) as a biomarker for acute renal injury after cardiac surgery. Lancet. (2005) 365(9466):1231–8. doi: 10.1016/S0140-6736(05)74811-X
60. Singer E, Markó L, Paragas N, Barasch J, Dragun D, Müller DN, et al. Neutrophil gelatinase-associated lipocalin: pathophysiology and clinical applications. Acta Physiol (Oxf). (2013) 207(4):663–72. doi: 10.1111/apha.12054
61. Coca SG, Nadkarni GN, Garg AX, Koyner J, Thiessen-Philbrook H, McArthur E, et al. First post-operative urinary kidney injury biomarkers and association with the duration of AKI in the TRIBE-AKI cohort. PLoS ONE. (2016) 11(8):e0161098. doi: 10.1371/journal.pone.0161098
62. Mitsnefes MM, Kathman TS, Mishra J, Kartal J, Khoury PR, Nickolas TL, et al. Serum neutrophil gelatinase-associated lipocalin as a marker of renal function in children with chronic kidney disease. Pediatr Nephrol. (2007) 22(1):101–8. doi: 10.1007/s00467-006-0244-x
63. Guo L, Zhu B, Yuan H, Zhao W. Evaluation of serum neutrophil gelatinase-associated lipocalin in older patients with chronic kidney disease. Aging Med (Milton). (2020) 3(1):32–9. doi: 10.1002/agm2.12098
64. Han WK, Bailly V, Abichandani R, Thadhani R, Bonventre JV. Kidney injury molecule-1 (KIM-1): a novel biomarker for human renal proximal tubule injury. Kidney Int. (2002) 62(1):237–44. doi: 10.1046/j.1523-1755.2002.00433.x
65. Ichimura T, Bonventre JV, Bailly V, Wei H, Hession CA, Cate RL, et al. Kidney injury molecule-1 (KIM-1), a putative epithelial cell adhesion molecule containing a novel immunoglobulin domain, is up-regulated in renal cells after injury. J Biol Chem. (1998) 273(7):4135–42. doi: 10.1074/jbc.273.7.4135
66. Huang Y, Don-Wauchope AC. The clinical utility of kidney injury molecule 1 in the prediction, diagnosis and prognosis of acute kidney injury: a systematic review. Inflamm Allergy Drug Targets. (2011) 10(4):260–71. doi: 10.2174/187152811796117735
67. Shao X, Tian L, Xu W, Zhang Z, Wang C, Qi C, et al. Diagnostic value of urinary kidney injury molecule 1 for acute kidney injury: a meta-analysis. PLoS ONE. (2014) 9(1):e84131. doi: 10.1371/journal.pone.0084131
68. Geng J, Qiu Y, Qin Z, Su B. The value of kidney injury molecule 1 in predicting acute kidney injury in adult patients: a systematic review and Bayesian meta-analysis. J Transl Med. (2021) 19(1):105. doi: 10.1186/s12967-021-02776-8
69. Han WK, Wagener G, Zhu Y, Wang S, Lee HT. Urinary biomarkers in the early detection of acute kidney injury after cardiac surgery. Clin J Am Soc Nephrol. (2009) 4(5):873–82. doi: 10.2215/CJN.04810908
70. Sabbisetti VS, Waikar SS, Antoine DJ, Smiles A, Wang C, Ravisankar A, et al. Blood kidney injury molecule-1 is a biomarker of acute and chronic kidney injury and predicts progression to ESRD in type I diabetes. J Am Soc Nephrol. (2014) 25(10):2177–86. doi: 10.1681/ASN.2013070758
71. Beker BM, Corleto MG, Fieiras C, Musso CG. Novel acute kidney injury biomarkers: their characteristics, utility and concerns. Int Urol Nephrol. (2018) 50(4):705–13. doi: 10.1007/s11255-017-1781-x
72. Sridharan K, Al Jufairi M, Al Segai O, Al Ansari E, Hashem Ahmed H, Husain Shaban G, et al. Biomarkers in neonates receiving potential nephrotoxic drugs. Eur Rev Med Pharmacol Sci. (2021) 25(22):7078–88. doi: 10.26355/eurrev_202111_27260
73. Desai RJ, Kazarov CL, Wong A, Kane-Gill SL. Kidney damage and stress biomarkers for early identification of drug-induced kidney injury: a systematic review. Drug Saf. (2022) 45:839–52. doi: 10.26355/eurrev_202111_27260.35831683
74. Cuesta C, Fuentes-Calvo I, Sancho-Martinez SM, Valentijn FA, Düwel A, Hidalgo-Thomas OA, et al. Urinary KIM-1 correlates with the subclinical sequelae of tubular damage persisting after the apparent functional recovery from intrinsic acute kidney injury. Biomedicines. (2022) 10(5):1106. doi: 10.3390/biomedicines10051106
75. Peng S, Liu N, Wei K, Li G, Zou Z, Liu T, et al. The predicted value of kidney injury molecule-1 (KIM-1) in healthy people. Int J Gen Med. (2022) 15:4495–503. doi: 10.2147/IJGM.S361468
76. Song J, Yu J, Prayogo GW, Cao W, Wu Y, Jia Z, et al. Understanding kidney injury molecule 1: a novel immune factor in kidney pathophysiology. Am J Transl Res. (2019) 11(3):1219–29.30972157
77. Dinarello CA, Novick D, Kim S, Kaplanski G. Interleukin-18 and IL-18 binding protein. Front Immunol. (2013) 4:289. doi: 10.3389/fimmu.2013.00289
78. Hirooka Y, Nozaki Y. Interleukin-18 in inflammatory kidney disease. Front Med (Lausanne). (2021) 8:639103. doi: 10.3389/fmed.2021.639103
79. Zdziechowska M, Gluba-Brzózka A, Poliwczak AR, Franczyk B, Kidawa M, Zielinska M, et al. Serum NGAL, KIM-1, IL-18, L-FABP: new biomarkers in the diagnostics of acute kidney injury (AKI) following invasive cardiology procedures. Int Urol Nephrol. (2020) 52(11):2135–43. doi: 10.1007/s11255-020-02530-x
80. Chui H, Caldwell J, Yordanova M, Cockovski V, Fredric D, Harel-Sterling M, et al. Tubular injury and cell-cycle arrest biomarkers to predict acute kidney injury in noncritically ill children receiving aminoglycosides. Biomark Med. (2020) 14(10):879–94. doi: 10.2217/bmm-2019-0419
81. Tao X, Chen C, Luo W, Zhou J, Tian J, Yang X, et al. Combining renal cell arrest and damage biomarkers to predict progressive AKI in patient with sepsis. BMC Nephrol. (2021) 22(1):415. doi: 10.1186/s12882-021-02611-8
82. Duff S, Irwin R, Cote JM, Redahan L, McMahon BA, Marsh B, et al. Urinary biomarkers predict progression and adverse outcomes ofacute kidney injury in critical illness. Nephrol Dial Transplant. (2021) 32:1668–78. doi: 10.1093/ndt/gfab263.
83. Al-Saegh RM A, Mohanad MA, Khudhair NJ, Al-Mukhtar MA R. Using urinary Interleukin-18 as a potential marker for early detection of acute kidney injury in intensive care unit. Saudi J Kidney Dis Transpl. (2021) 32(2):341–7. doi: 10.4103/1319-2442.335445
84. Dobilienė D, Masalskienė J, Rudaitis Š, Vitkauskienė A, Pečiulytė J, Kėvalas R. Early diagnosis and prognostic value of acute kidney injury in critically ill patients. Medicina (Kaunas). (2019) 55(8):506. doi: 10.3390/medicina55080506
85. Oncel MY, Canpolat FE, Arayici S, Alyamac Dizdar E, Uras N, Oguz SS. Urinary markers of acute kidney injury in newborns with perinatal asphyxia (.). Ren Fail. (2016) 38(6):882–8. doi: 10.3109/0886022X.2016.1165070
86. Li Y, Fu C, Zhou X, Xiao Z, Zhu X, Jin M, et al. Urine interleukin-18 and cystatin-C as biomarkers of acute kidney injury in critically ill neonates. Pediatr Nephrol. (2012) 27(5):851–60. doi: 10.1007/s00467-011-2072-x
87. Kadi FA, Yuniati T, Sribudiani Y, Rachmadi D. The association of rs187238, rs19465518 and rs1946519 IL-8 polymorphisms with acute kidney injury in preterm infants. Biomedicine. (2021) 11(4):43–50. doi: 10.37796/2211-8039.1231
88. Rumpel J, Spray BJ, Chock VY, Kirkley MJ, Slagle CL, Frymoyer A, et al. Urine biomarkers for the assessment of acute kidney injury in neonates with hypoxic ischemic encephalopathy receiving therapeutic hypothermia. J Pediatr. (2022) 241:133–140.e3. doi: 10.1016/j.jpeds.2021.08.090
89. Sampaio de Souza Garms D, Cardoso Eid KZ, Burdmann EA, Marçal LJ, Antonângelo L, Dos Santos A, et al. The role of urinary biomarkers as diagnostic and prognostic predictors of acute kidney injury associated with vancomycin. Front Pharmacol. (2021) 12:705636. doi: 10.3389/fphar.2021.705636
90. Sethi SK, Sharma R, Gupta A, Tibrewal A, Akole R, Dhir R, et al. Long-term renal outcomes in children with acute kidney injury post cardiac surgery. Kidney Int Rep. (2021) 6(7):1850–7. doi: 10.1016/j.ekir.2021.04.018
91. Cooper DS, Claes D, Goldstein SL, Bennett MR, Ma Q, Devarajan P, et al. Follow-up renal assessment of injury long-term after acute kidney injury (FRAIL-AKI). Clin J Am Soc Nephrol. (2016) 11(1):21–9. doi: 10.2215/CJN.04240415
92. Malhotra R, Katz R, Jotwani V, Ambrosius WT, Raphael KL, Haley W, et al. Urine markers of kidney tubule cell injury and kidney function decline in SPRINT trial participants with CKD. Clin J Am Soc Nephrol. (2020) 15(3):349–58. doi: 10.2215/CJN.02780319
93. Liang D, Liu H-F, Yao C-W, Liu H-Y, Huang-Fu C-M, Chen X-W, et al. Effects of interleukin 18 on injury and activation of human proximal tubular epithelial cells. Nephrology (Carlton). (2007) 12(1):53–61. doi: 10.1111/j.1440-1797.2006.00737.x
94. Pozzoli S, Simonini M, Manunta P. Predicting acute kidney injury: current status and future challenges. J Nephrol. (2018) 31(2):209–23. doi: 10.1007/s40620-017-0416-8
95. Ortega LM, Heung M. The use of cell cycle arrest biomarkers in the early detection of acute kidney injury. Is this the new renal troponin? Nefrologia. (2018) 38(4):361–7. doi: 10.1016/j.nefro.2017.11.013
96. Kashani K, Al-Khafaji A, Ardiles T, Artigas A, Bagshaw SM, Bell M, et al. Discovery and validation of cell cycle arrest biomarkers in human acute kidney injury. Crit Care. (2013) 17(1):R25. doi: 10.1186/cc12503
97. Meersch M, Schmidt C, Van Aken H, Rossaint J, Görlich D, Stege D, et al. Validation of cell-cycle arrest biomarkers for acute kidney injury after pediatric cardiac surgery. PLoS ONE. (2014) 9(10):e110865. doi: 10.1371/journal.pone.0110865
98. Meersch M, Schmidt C, Van Aken H, Martens S, Rossaint J, Singbartl K, et al. Urinary TIMP-2 and IGFBP7 as early biomarkers of acute kidney injury and renal recovery following cardiac surgery. PLoS ONE. (2014) 9(3):e93460. doi: 10.1371/journal.pone.0093460
99. Bihorac A, Chawla LS, Shaw AD, Al-Khafaji A, Davison DL, Demuth GE, et al. Validation of cell-cycle arrest biomarkers for acute kidney injury using clinical adjudication. Am J Respir Crit Care Med. (2014) 189(8):932–9. doi: 10.1164/rccm.201401-0077OC
100. Di Leo L, Nalesso F, Garzotto F, Xie Y, Yang B, Virzì GM, et al. Predicting acute kidney injury in intensive care unit patients: the role of tissue inhibitor of metalloproteinases-2 and insulin-like growth factor-binding protein-7 biomarkers. Blood Purif. (2018) 45(1–3):270–7. doi: 10.1159/000485591
101. Hoste EAJ, McCullough PA, Kashani K, Chawla LS, Joannidis M, Shaw AD, et al. Derivation and validation of cutoffs for clinical use of cell cycle arrest biomarkers. Nephrol Dial Transplant. (2014) 29(11):2054–61. doi: 10.1093/ndt/gfu292
102. Heung M, Ortega LM, Chawla LS, Wunderink RG, Self WH, Koyner JL, et al. Common chronic conditions do not affect performance of cell cycle arrest biomarkers for risk stratification of acute kidney injury. Nephrol Dial Transplant. (2016) 31(10):1633–40. doi: 10.1093/ndt/gfw241
103. Fan W, Ankawi G, Zhang J, Digvijay K, Giavarina D, Yin Y, et al. Current understanding and future directions in the application of TIMP-2 and IGFBP7 in AKI clinical practice. Clin Chem Lab Med. (2019) 57(5):567–76. doi: 10.1515/cclm-2018-0776
104. Daubin D, Cristol JP, Dupuy AM, Kuster N, Besnard N, Platon L, et al. Urinary biomarkers IGFBP7 and TIMP-2 for the diagnostic assessment of transient and persistent acute kidney injury in critically ill patients. PLoS ONE. (2017) 12(1):e0169674. doi: 10.1371/journal.pone.0169674
105. Titeca-Beauport D, Daubin D, Van Vong L, Belliard G, Bruel C, Alaya S, et al. Urine cell cycle arrest biomarkers distinguish poorly between transient and persistent AKI in early septic shock: a prospective, multicenter study. Crit Care. (2020) 24(1):280. doi: 10.1186/s13054-020-02984-6
106. Godi I, De Rosa S, Martino F, Bazzano S, Martin M, Boni E, et al. Urinary [TIMP-2]×[IGFBP7] and serum procalcitonin to predict and assess the risk for short-term outcomes in septic and non-septic critically ill patients. Ann Intensive Care. (2020) 10(1):46. doi: 10.1186/s13613-020-00665-9
107. Fuentes-Calvo I, Cuesta C, Sancho-Martínez SM, Hidalgo-Thomas OA, Paniagua-Sancho M, López-Hernández FJ, et al. Biomarkers of persistent renal vulnerability after acute kidney injury recovery. Sci Rep. (2021) 11(1):21183. doi: 10.1038/s41598-021-00710-y
108. Cho WY, Lim SY, Yang JH, Oh SW, Kim M-G, Jo S-K. Urinary tissue inhibitor of metalloproteinase-2 and insulin-like growth factor-binding protein 7 as biomarkers of patients with established acute kidney injury. Korean J Intern Med. (2020) 35(3):662–71. doi: 10.3904/kjim.2018.266
109. Liu KD, Glidden DV, Eisner MD, Parsons PE, Ware LB, Wheeler A, et al. Predictive and pathogenetic value of plasma biomarkers for acute kidney injury in patients with acute lung injury. Crit Care Med. (2007) 35(12):2755–61.18074478
110. Gough P, Myles IA. Tumor necrosis factor receptors: pleiotropic signaling complexes and their differential effects. Front Immunol. (2020) 11:585880. doi: 10.3389/fimmu.2020.585880
111. Iglesias J, Marik PE, Levine JS. Norasept II study investigators. Elevated serum levels of the type I and type II receptors for tumor necrosis factor-alpha as predictive factors for ARF in patients with septic shock. Am J Kidney Dis. (2003) 41(1):62–75. doi: 10.1053/ajkd.2003.50024
112. Morais BS, Teixeira AL, Maciel JF, Lima AA, Barbosa IG, Sanches MD. Soluble tumor necrosis factor receptors as predictors of 1-year mortality and renal dysfunction after liver transplantation. Transpl Immunol. (2016) 35:29–33. doi: 10.1016/j.trim.2016.01.006
113. Fernández-Juárez G, Villacorta Perez J, Luño Fernández JL, Martinez-Martinez E, Cachofeiro V, Barrio Lucia V, et al. High levels of circulating TNFR1 increase the risk of all-cause mortality and progression of renal disease in type 2 diabetic nephropathy. Nephrology (Carlton). (2017) 22(5):354–60. doi: 10.1111/nep.12781
114. Ferrando E S, Hanslin K, Hultström M, Larsson A, Frithiof R, Lipcsey M, et al. Soluble TNF receptors predict acute kidney injury and mortality in critically ill COVID-19 patients: a prospective observational study. Cytokine. (2022) 149:155727. doi: 10.1016/j.cyto.2021.155727
115. Murugan R, Wen X, Shah N, Lee M, Kong L, Pike F, et al. Plasma inflammatory and apoptosis markers are associated with dialysis dependence and death among critically ill patients receiving renal replacement therapy. Nephrol Dial Transplant. (2014) 29(10):1854–64. doi: 10.1093/ndt/gfu051
116. Forsblom C, Moran J, Harjutsalo V, Loughman T, Wadén J, Tolonen N, et al. Added value of soluble tumor necrosis factor-α receptor 1 as a biomarker of ESRD risk in patients with type 1 diabetes. Diabetes Care. (2014) 37(8):2334–42. doi: 10.2337/dc14-0225
117. Kamei N, Yamashita M, Nishizaki Y, Yanagisawa N, Nojiri S, Tanaka K, et al. Association between circulating tumor necrosis factor-related biomarkers and estimated glomerular filtration rate in type 2 diabetes. Sci Rep. (2018) 8(1):15302. doi: 10.1038/s41598-018-33590-w
118. Coca SG, Nadkarni GN, Huang Y, Moledina DG, Rao V, Zhang J, et al. Plasma biomarkers and kidney function decline in early and established diabetic kidney disease. J Am Soc Nephrol. (2017) 28(9):2786–93. doi: 10.1681/ASN.2016101101
119. Sarnak MJ, Katz R, Ix JH, Kimmel PL, Bonventre JV, Schelling J, et al. Plasma biomarkers as risk factors for incident CKD. Kidney Int Rep. (2022) 7(7):1493–501. doi: 10.1016/j.ekir.2022.03.018
120. Sonoda Y, Gohda T, Suzuki Y, Omote K, Ishizaka M, Matsuoka J, et al. Circulating TNF receptors 1 and 2 are associated with the severity of renal interstitial fibrosis in IgA nephropathy. PLoS ONE. (2015) 10(4):e0122212. doi: 10.1371/journal.pone.0122212
121. Noiri E, Doi K, Negishi K, Tanaka T, Hamasaki Y, Fujita T, et al. Urinary fatty acid-binding protein 1: an early predictive biomarker of kidney injury. Am J Physiol Renal Physiol. (2009) 296(4):F669–79. doi: 10.1152/ajprenal.90513.2008
122. Yamamoto T, Noiri E, Ono Y, Doi K, Negishi K, Kamijo A, et al. Renal L-type fatty acid–binding protein in acute ischemic injury. J Am Soc Nephrol. (2007) 18(11):2894–902. doi: 10.1681/ASN.2007010097
123. Negishi K, Noiri E, Doi K, Maeda-Mamiya R, Sugaya T, Portilla D, et al. Monitoring of urinary L-type fatty acid-binding protein predicts histological severity of acute kidney injury. Am J Pathol. (2009) 174(4):1154–9. doi: 10.2353/ajpath.2009.080644
124. Ferguson MA, Vaidya VS, Waikar SS, Collings FB, Sunderland KE, Gioules CJ, et al. Urinary liver-type fatty acid-binding protein predicts adverse outcomes in acute kidney injury. Kidney Int. (2010) 77(8):708–14. doi: 10.1038/ki.2009.422
125. Doi K, Noiri E, Maeda-Mamiya R, Ishii T, Negishi K, Hamasaki Y, et al. Urinary L-type fatty acid-binding protein as a new biomarker of sepsis complicated with acute kidney injury. Crit Care Med. (2010) 38(10):2037–42. doi: 10.1097/CCM.0b013e3181eedac0
126. Portilla D, Dent C, Sugaya T, Nagothu KK, Kundi I, Moore P, et al. Liver fatty acid-binding protein as a biomarker of acute kidney injury after cardiac surgery. Kidney Int. (2008) 73(4):465–72. doi: 10.1038/sj.ki.5002721
127. Kamijo A, Sugaya T, Hikawa A, Yamanouchi M, Hirata Y, Ishimitsu T, et al. Urinary liver-type fatty acid binding protein as a useful biomarker in chronic kidney disease. Mol Cell Biochem. (2006) 284(1–2):175–82. doi: 10.1007/s11010-005-9047-9
Keywords: acute kidney injury, acute kidney disease, chronic kidney disease, biomarkers, prognostic model
Citation: Patel M and Gbadegesin RA (2022) Update on prognosis driven classification of pediatric AKI. Front. Pediatr. 10:1039024. doi: 10.3389/fped.2022.1039024
Received: 7 September 2022; Accepted: 3 October 2022;
Published: 21 October 2022.
Edited by:
Michael L Moritz, University of Pittsburgh, United StatesReviewed by:
Aftab S Chishti, University of Kentucky, United States© 2022 Patel and Gbadegesin. This is an open-access article distributed under the terms of the Creative Commons Attribution License (CC BY). The use, distribution or reproduction in other forums is permitted, provided the original author(s) and the copyright owner(s) are credited and that the original publication in this journal is cited, in accordance with accepted academic practice. No use, distribution or reproduction is permitted which does not comply with these terms.
*Correspondence: Mital Patel TWl0YWwucGF0ZWwxNjZAZHVrZS5lZHU=
Specialty Section:This article was submitted to Pediatric Nephrology, a section of the journal Frontiers in Pediatrics
Disclaimer: All claims expressed in this article are solely those of the authors and do not necessarily represent those of their affiliated organizations, or those of the publisher, the editors and the reviewers. Any product that may be evaluated in this article or claim that may be made by its manufacturer is not guaranteed or endorsed by the publisher.
Research integrity at Frontiers
Learn more about the work of our research integrity team to safeguard the quality of each article we publish.