- 1Division of Cardiology, Children’s Hospital of Philadelphia, Philadelphia, PA, United States
- 2Department of Pediatrics, University of Pennsylvania Perelman School of Medicine, Philadelphia, PA, United States
- 3Division of Gastroenterology, Hepatology, and Nutrition, Children’s Hospital of Philadelphia, Philadelphia, PA, United States
Background: Skeletal muscle deficits are associated with worse exercise performance in adults with pulmonary hypertension (PH) but the impact is poorly understood in pediatric PH.
Objective: To study muscle deficits, physical inactivity, and performance on cardiopulmonary exercise test (CPET) and exercise cardiac magnetic resonance (eCMR) in pediatric PH.
Methods: Youth 8–18 years participated in a prospective, cross-sectional study including densitometry (DXA) for measurement of leg lean mass Z-score (LLMZ), handheld dynamometer with generation of dominant and non-dominant handgrip Z-scores, Physical Activity Questionnaire (PAQ), CPET, and optional eCMR. CPET parameters were expressed relative to published reference values. CMR protocol included ventricular volumes and indexed systemic flow at rest and just after supine ergometer exercise. Relationships between LLMZ, PAQ score, and exercise performance were assessed by Pearson correlation and multiple linear regression.
Results: There were 25 participants (13.7 ± 2.8 years, 56% female, 64% PH Group 1, 60% functional class I); 12 (48%) performed both CPET and eCMR. Mean LLMZ (–0.96 ± 1.14) was associated with PAQ score (r = 50, p = 0.01) and with peak oxygen consumption (VO2) (r = 0.74, p = < 0.001), VO2 at anaerobic threshold (r = 0.65, p < 0.001), and peak work rate (r = 0.64, p < 0.01). Higher handgrip Z-scores were associated with better CPET and eCMR performance. On regression analysis, LLMZ and PAQ score were positively associated with peak VO2, while handgrip Z-score and PAQ score were positively associated with peak work rate.
Conclusion: Muscle mass and strength are positively associated with exercise performance in pediatric PH. Future studies should determine the effect of rehabilitation programs on muscle properties and exercise performance.
Introduction
Pediatric pulmonary hypertension (PH) is associated with various vascular, cardiac, pulmonary, and systemic conditions. Without treatment, the disease leads to right ventricular dysfunction, right ventricular failure, and death. While therapies have improved in recent years (1, 2), long-term outcomes remain poor. Patients report low quality of life in the face of significant morbidity and mortality risk (3, 4). Exercise intolerance is common in PH patients, significantly impacting quality of life and prognosis. While cardiopulmonary status affects exercise performance, the contribution of peripheral factors, including skeletal muscle dysfunction, is increasingly recognized (5). Skeletal muscle dysfunction is associated with worse 6-min walk distance (6 MWD) in adults with idiopathic pulmonary arterial hypertension (IPAH) (6). Similarly, we previously reported marked deficits in densitometry (DXA)-measured leg lean mass, a surrogate marker of skeletal muscle, in youth with PH in association with inactivity and worse 6 MWD (7). In other studies, skeletal muscle atrophy, impaired peripheral oxygen extraction, and reduced muscle contractility suggest that PH patients exhibit a generalized “myopathy” similar to patients with left heart failure (8–11). The skeletal muscle pump is critically important to augmentation of systemic venous return and pulmonary blood flow with erect exercise (12). Skeletal muscle dysfunction may critically hinder this mechanism in PH patients. Therefore, we sought to expand on our prior findings by characterizing leg lean mass Z-score (LLMZ) and muscle strength in a different cohort of pediatric PH patients and to explore the associations between muscle deficits, self-reported physical activity, and measures of exercise performance on cardiopulmonary exercise test (CPET) and exercise cardiac magnetic resonance (eCMR).
Materials and methods
Study population
Youth ages 8–18 years with World Symposium of PH (WSPH) diagnostic Groups 1–4 and functional class I or II were prospectively enrolled in a cross-sectional study from 2018 to 2021. Exclusion criteria included pregnancy, functional class III or IV, single ventricle physiology, moderate to severe chronic kidney disease (stage 3 or greater), severe hepatic impairment (transaminases > 2 times the upper limit of normal), pacemaker, defibrillator, or other metal that would interfer with imaging, and significant developmental delay or inability to comply with verbal English instructions in order to complete the study procedures. Fully informed, written consent was obtained from the parent/legal guardian of participants < 18 years and of participants 18 years of age. In addition, age-appropriate informed assent was obtained from participants < 18 years. This study was approved by the Children’s Hospital of Philadelphia Institutional Review Board.
Study procedures
Pulmonary hypertension history and standard of care testing
The medical record was queried for WSPH diagnostic group, World Health Organization (WHO) functional class, medications, and standard of care testing including last echocardiogram, 6-min walk test (within 3 months), cardiac catheterization data, and brain-type natriuretic peptide. Six-minute walk tests were performed according to American Thoracic Society guidelines (13).
Anthropometry and tanner stage
Weight was measured to the nearest 0.1 kg with a digital electronic stand-on scale. Height and sitting height were measured to the nearest 0.1 cm with a wall-mounted stadiometer in order to calculate leg length (leg length = height - sitting height). Tanner stage was determined via a validated self-assessment tool (14).
Heath-related quality of life
Participants and parents/guardians completed the Pediatric Cardiac Quality of Life Inventory, a reliable and validated instrument of disease specific quality of life for patients 8–18 years of age with congenital or acquired heart disease (15). Disease impact and psychosocial impact subscores were summed to generate a total score with higher scores (maximum 100 points) representing better health-related quality of life.
Physical activity questionnaire
Participants completed the Physical Activity Questionnaire (PAQ) for Older Children or Adolescents (PAQ-C and PAQ-A), 7-day recall instruments designed to assess moderate to vigorous physical activity (16–18). The 5-point scoring scale was used to calculate a final summary score from the means of scores for each question. Average scores > 3 are reported in healthy populations (16).
Vitamin D levels
Quantification of circulating 25 (OH) vitamin D was performed by HPLC tandem mass-spectrometry (19). Vitamin D deficiency was defined as serum level less than 20 ng/mL (20).
Body composition
Whole body lean and fat mass were measured with a Hologic Delphi densitometer (Bedford, Massachusetts, USA) in array mode (software V.12.4). Measurements were performed with standard supine positioning techniques with participants wearing scrubs to minimize scan variability. Urine pregnancy test was performed prior to DXA in female participants. Whole body lean mass was calculated as fat-free mass minus bone mineral content. Leg lean mass was used as a measure of skeletal muscle, given the previously reported concerns with the use of whole body lean mass as a representation of muscle mass (21). Calibration was performed daily with a hydroxyapatite phantom and weekly with a whole-body phantom. Coefficients of variation ranged from 1 to 4% (22).
Muscle strength testing
Bilateral forearm strength was measured with a handgrip dynamometer (Takei, Tokyo, Japan) (23). Hand dominance was determined by which hand the participant used to hold a pencil. The participant stood upright with the shoulder adducted holding the dynamometer, not touching the trunk. The handle was adjusted to the hand size of the participant, and no extra body movement was allowed during testing. For each hand, 3 maximal effort trials lasting 4–5 s interspersed with 60-s rests were carried out. The highest value was retained for analysis. Lower extremity strength (knee and ankle) was assessed using the Biodex Multi-Joint System 3 Pro (Biodex Medical Systems, Inc., Shirley, NY, USA) (24). For the knee, peak quadricep muscle torque (ft-lbs) was measured in knee flexion and extension. Participants sat with their thighs at an angle of 110° to the trunk. The trunk and both thighs were stabilized with belts. The tested knee was positioned at 90° flexion, and the mechanical axis of the dynamometer was aligned with the lateral epicondyle of the knee. Each participant performed 10 concentric contractions at 120°/s (flexion and extension) of both sides, and the highest value was recorded. For the ankle, peak calf muscle torque (ft-lbs) in dorsiflexion and plantarflexion were measured in triplicate with the foot placed in 20 degrees of plantar flexion, and the highest value was recorded (24, 25). Peak muscle torque was adjusted for patient age.
Cardiopulmonary exercise test
Patients exercised to their maximum ability on an electronically braked cycle ergometer (Ergometrics 800, Sensor-Medics, Yorba Linda, CA). A 12-lead electrocardiogram was obtained at rest in supine, sitting, and standing positions. Three minutes of pedaling in an unloaded state were followed by a ramp increase in work rate to achieve predicted peak work rate in 10–12 min of cycling time (26). Cardiac rhythm and pulse oximetry were monitored throughout the study. Blood pressure was measured at rest and every 3 min during exercise and recovery by auscultation. Metabolic data were obtained throughout the study and for the first 2 min of recovery on a breath-by-breath basis using a metabolic cart (SensorMedics V29, Yorba Linda, CA or similar). Ventilatory anaerobic threshold (VAT) was measured by the V-slope method (27). Peak oxygen consumption (VO2) and VO2 at VAT were normalized to the percentage expected for age, gender, and body size (28). O2 pulse was calculated by dividing peak VO2 by maximum heart rate. And expressed in milliliters per beat. A maximal test was defined as a respiratory exchange ratio ≥ 1.10 (29).
Exercise cardiac magnetic resonance
Participants ≥ 11 years of age could consent to a non-sedate, resting and exercise CMR protocol (30). The resting protocol consisted of a contiguous axial stack of static steady-state free precession images used for multiplanar anatomic reconstruction, both segmented and free-breathing real time cine short axis stacks, and through-plane retrospectively gated, respiratory-averaged phase-contrast MR across the superior and inferior vena cavae, branch pulmonary arteries, aortic valve, and descending aorta at the level of the diaphragm. After resting CMR image acquisition, the participant was slid partially out from the MR bore to perform lower limb exercise using an MR-compatible supine bicycle ergometer (Lode BV, Groningen, Netherlands). Heart rate was monitored continuously. An initial workload of 20 Watts was increased 20 Watts/minute to achieve the heart rate associated with VAT on the prior CPET. Exercise was suspended, the participant’s feet were removed from the ergometer pedals, and the participant was returned to isocenter for imaging (generally within 5–10 s). A free-breathing real time cine short axis stack was performed; this method has been previously validated against breath-held segmented short axis imaging (31, 32). As breath-holding is not uniform after exercise, respiratory-averaged segmented phase-contrast MR measurements of the aorta, superior vena cava, and descending aorta were performed. Descending aorta flow is substituted for inferior vena cava flow due to difficulty in maintaining inferior vena cava position at exercise (33). Flows and volumes were segmented using cvi42 software 5.13.7 (Circle Cardiovascular Imaging Inc.). Cardiac index was calculated as the product of stroke volume and heart rate, indexed to body surface area. Indexed systemic blood flow was calculated as the sum of superior vena cava and descending aorta flow, indexed to body surface area.
Statistical analysis
Growth and body composition variables were converted to Z-scores (standard deviation scores) as previously described (7, 21, 34). The 2,000 Centers for Disease Control and Prevention growth charts were used to calculate sex-specific Z-scores for height, weight, and body mass index relative to age (35). Data from > 2,000 healthy, typically developing children from multiple ethnic groups, ages 5–19 years, enrolled in the Bone Mineral Density in Childhood Study (BMDCS) (36, 37), a multicenter longitudinal DXA study, were used to compare participants’ growth Z-scores to a contemporary cohort. These reference data were also used to calculate sex- and race-specific LLMZ relative to age using the LMS method (38). Body composition measures are highly correlated with height and PH physiology is associated with impaired linear growth (39). Therefore, LLMZ was further adjusted for leg length Z-score (40).
Sex-specific reference curves for dominant and non-dominant handgrip were generated using data from the 2011–2012 and 2013–2014 releases of the US National Health and Nutrition Examination Survey (NHANES) using the LMS method (38) and implemented in R programming language using the Generalized Additive Models for Location, Scale, and Shape (GAMLSS library) in R (41).
Standard descriptive statistics [mean ± standard deviation or median (interquartile range)] were used to summarize baseline PH characteristics, LLMZ, muscle strength, quality of life scores, and CPET and eCMR data. Differences in body composition Z-scores between PH participants and the BMDCS reference data were assessed using one-sample Student’s t-test. Analyses within the PH group included correlations between LLMZ and continuous variables (e.g., indexed pulmonary vascular resistance) assessed by Pearson or Spearman correlation and comparisons of LLMZ according to categorical variables (e.g., diagnostic group or functional class). Associations between muscle mass and strength, physical activity, and exercise parameters were assessed by Pearson correlation and multiple linear regression. All analyses were conducted using Stata 16.1 with two-sided tests of hypotheses and a p-value < 0.05 as the criterion for clinical significance.
Results
Demographic and clinical characteristics of the 25 participants are displayed in Table 1. The cohort was 56% female and majority white. Mean height Z-score was –0.24 ± 1.24 while mean BMI Z-score was 0.19 ± 0.93. Most patients (64%) were classified as WSPH Group 1 (pulmonary arterial hypertension), but 24% of patients were classified as WSPH group 3 (PH due to lung disease). Of the 16 participants in WSPH Group 1, there were 10 with idiopathic or heritable PAH and 6 with PAH after repair of congenital heart disease. No participants with congenital heart disease had unrepaired cyanotic lesions or significant residual shunts. More patients were WHO functional class I but patients in functional class II enrolled as well. The most common medications were tadalafil and ambrisentan. Twenty-eight percent of the cohort met criteria for vitamin D deficiency. Mean brain natriuretic peptide and hemoglobin levels were within normal limits for our laboratory. Mean tricuspid annular plane systolic excursion Z-score was –2.5 ± 3.2 reflecting decreased right ventricular function. Participants achieved an average 6 MWD of 636 ± 113 meters reflecting good functional status. Cardiac catheterization data were available in 23 participants (90%) at median 1.42 years prior to the study visit. Mean pulmonary artery pressure was 33.1 ± 12.0 mm Hg and indexed pulmonary vascular resistance was 6.2 ± 3.0 indexed Wood units. PAQ scores were lower than reported for healthy populations. Average quality of life score was less than 70 for both participants and parents/guardians.
Skeletal muscle mass and strength data are shown in Table 2. Mean LLMZ was markedly decreased at –0.96 ± 1.14, equivalent to the 17th percentile. Dominant (D-HGZ) and non-dominant (ND-HGZ) were also very low with mean D-HGZ of –1.33 ± 1.37 equivalent to the 9th percentile.
Table 3 includes CPET and eCMR data. Baseline oxygen saturation was 97.7 ± 1.5% with decrease to 91.5 ± 8.3% (range 71–100) with CPET. On CPET, participants performed lower than predicted compared to healthy reference data. Percent predicted peak VO2 was approximately 71% while percent predicted VO2 at VAT was approximately 80%. Participants only achieved a peak work rate 59% predicted for demographics and body size. Reference data are not available for work rate at VAT. Resting and exercise CMR data are displayed. Right and left ventricular cardiac index and systemic blood flow increased with exercise.
LLMZ was associated with disease-specific factors. LLMZ was positively associated with hemoglobin (r = 0.44, p = 0.03) and negatively associated with brain type natriuretic peptide (r = –0.40, p = 0.05), mean pulmonary artery pressure (r = –0.54, p < 0.01), and indexed pulmonary vascular resistance (r = –0.50, p = 0.02) but was not associated with tricuspid annular plane systolic Z-score. LLMZ was higher in those with higher PAQ scores (r = 0.50, p = 0.01).
LLMZ, muscle strength, and PAQ score were associated with performance on CPET and eCMR (Table 4). LLMZ, both D-HGZ and ND-HGZ, and PAQ score were associated with percent predicted peak VO2, percent predicted VO2 at VAT, and percent predicted work (Figures 1–3). LLMZ was associated with O2 pulse on CPET (r = 0.41, p = 0.04) while D-HGZ was not. D-HGZ was associated with most recent 6 MWD (r = 0.44, p = 0.03). D-HGZ, ND-HGZ, and PAQ score were also associated with change in systemic flow from rest to exercise on eCMR, while LLMZ was not (r = 0.51, p = 0.1).
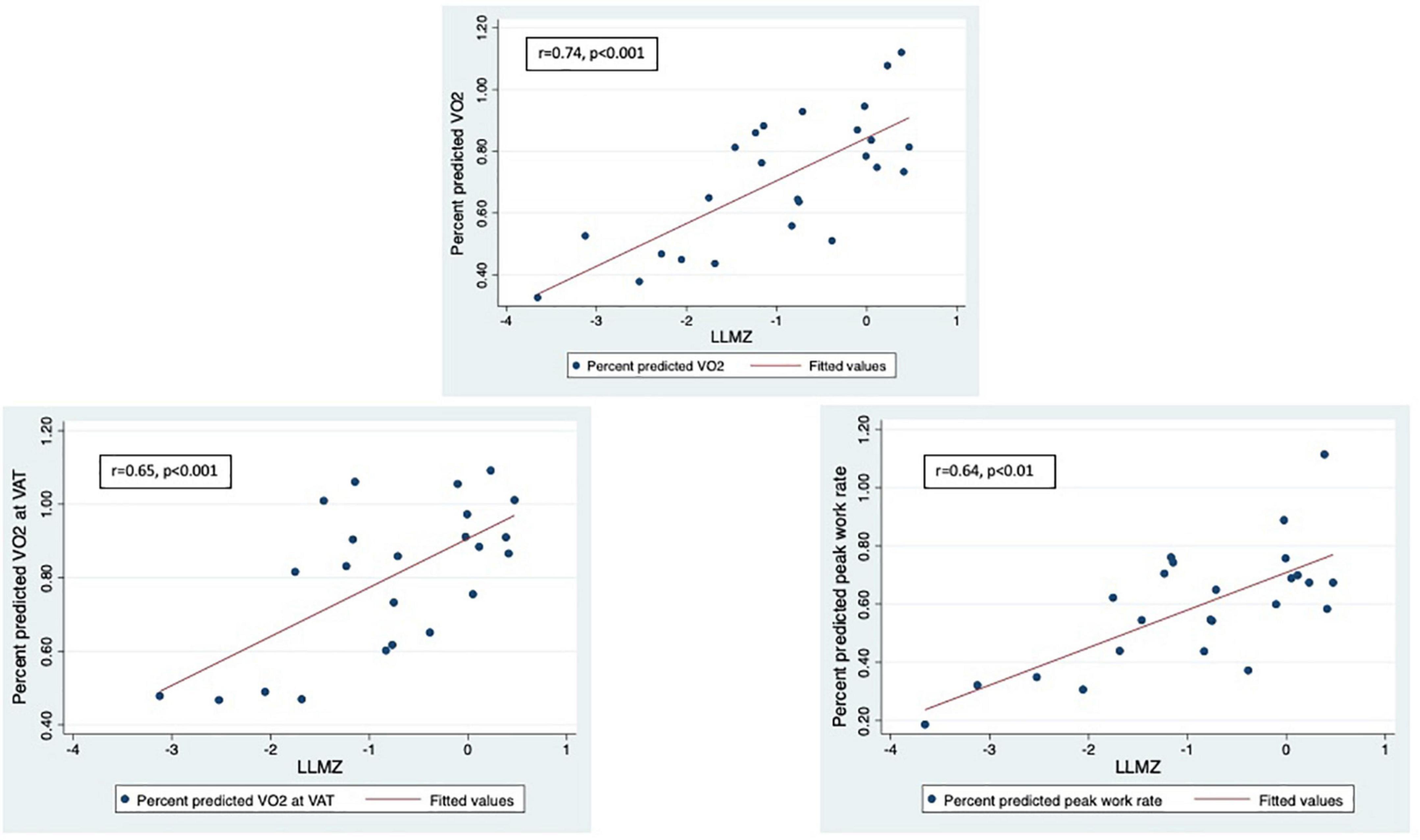
Figure 1. LLMZ, D-HGZ, and PAQ score were associated with percent predicted peak VO2, percent predicted VO2 at VAT, and percent predicted work. This figure demonstrates the associations between LLMZ and CPET parameters.
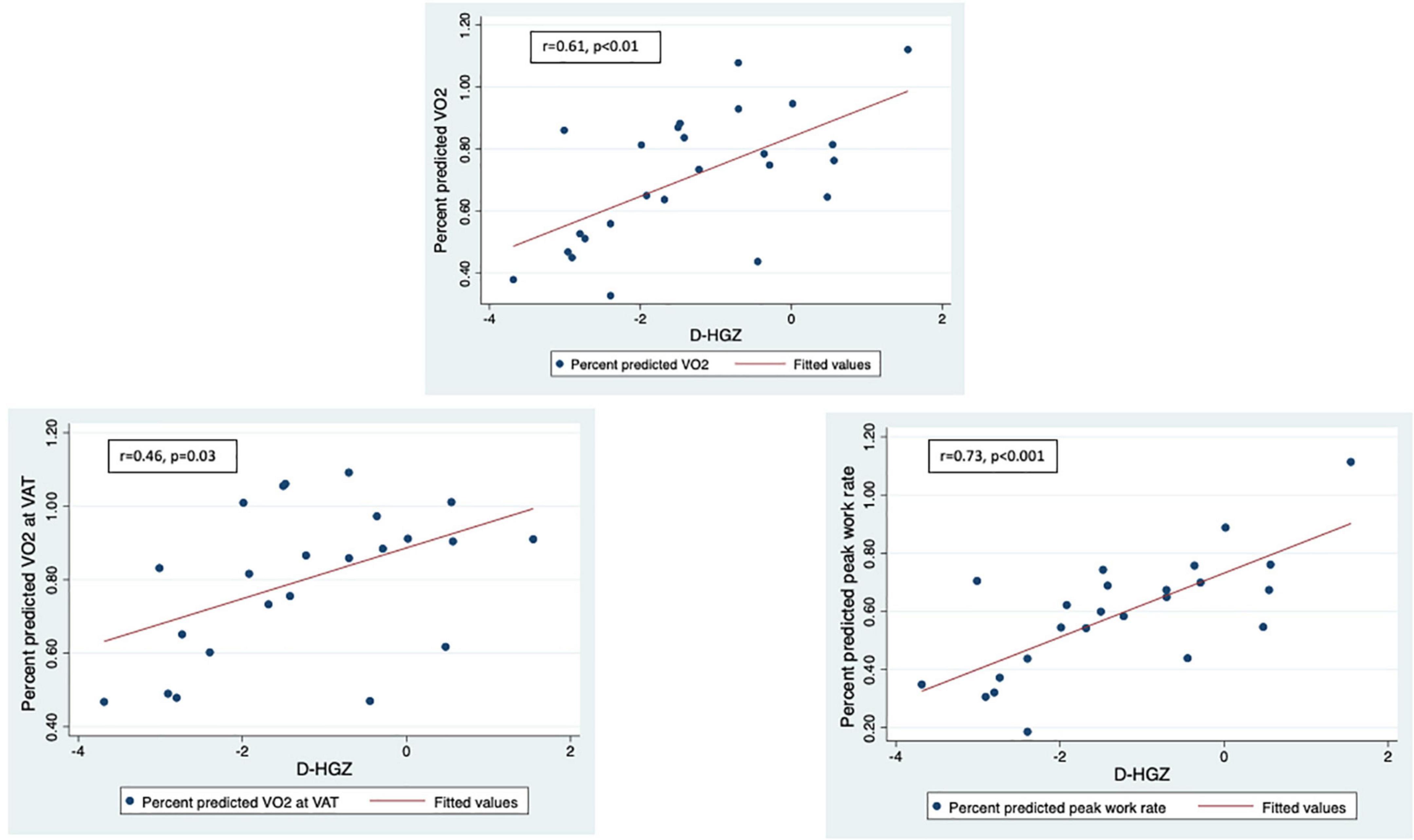
Figure 2. LLMZ, D-HGZ, and PAQ score were associated with percent predicted peak VO2, percent predicted VO2 at VAT, and percent predicted work. This figure demonstrates the associations between D-HGZ and CPET parameters.
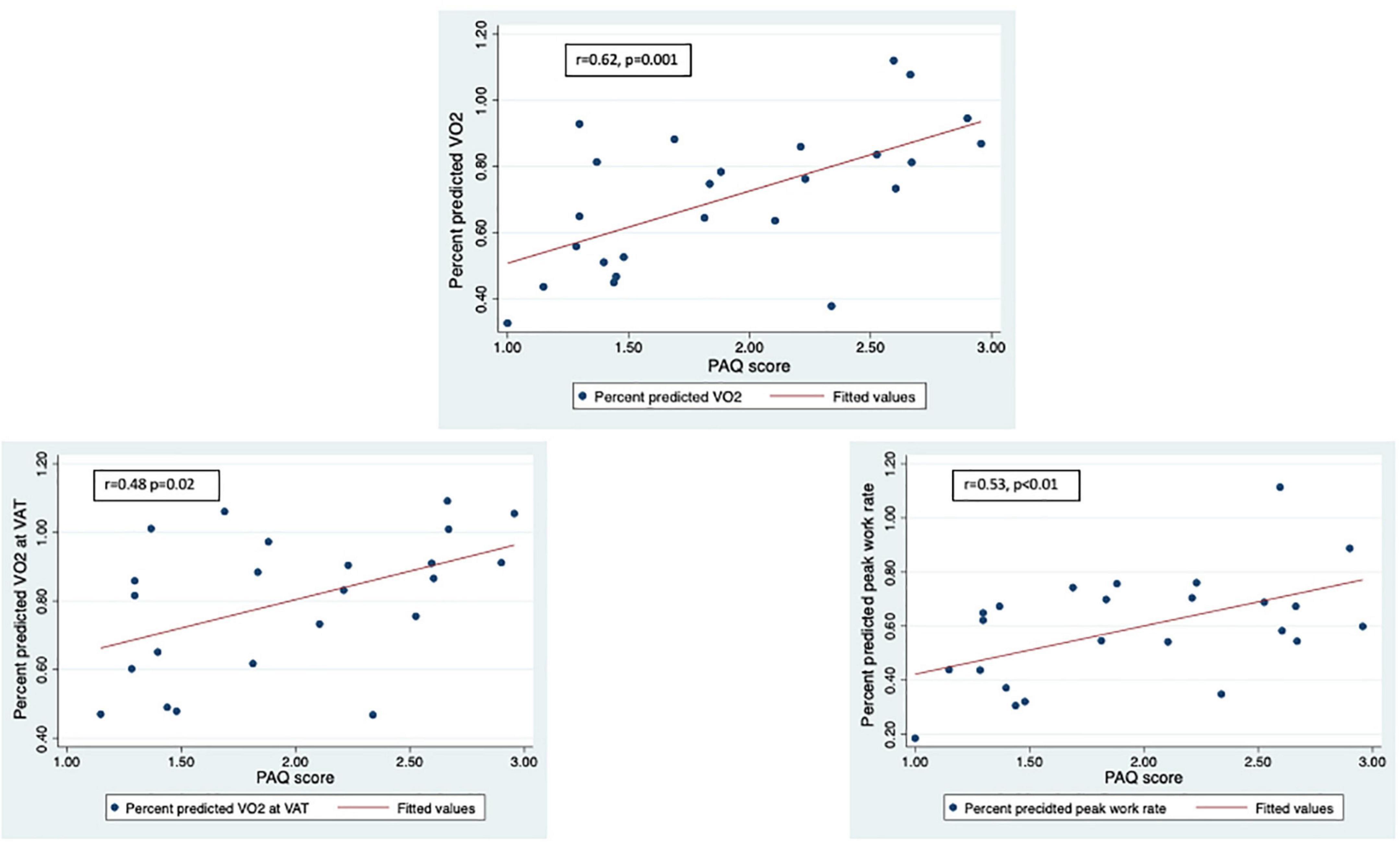
Figure 3. LLMZ, D-HGZ, and PAQ score were associated with percent predicted peak VO2, percent predicted VO2 at VAT, and percent predicted work. This figure demonstrates the associations between PAQ score and CPET parameters.
The associations between lower extremity strength by Biodex and exercise performance were variable. Ankle extension was associated with change in systemic flow from rest to exercise (r = 0.69, p = 0.04). Both knee extension and flexion were associated with peak work (not percent predicted) (r = 0.52, p = 0.02 for extension; r = 0.48, p = 0.03 for flexion), possibly because Z-scores are not available for the Biodex variables.
Results from multiple linear regression models testing the effect of skeletal muscle and physical activity variables on exercise performance are shown in Table 5. LLMZ and PAQ score were positively associated with peak VO2, while handgrip Z-score and PAQ score were positively associated with peak work rate. No factors were associated with the change in systemic flow from rest to exercise on eCMR.
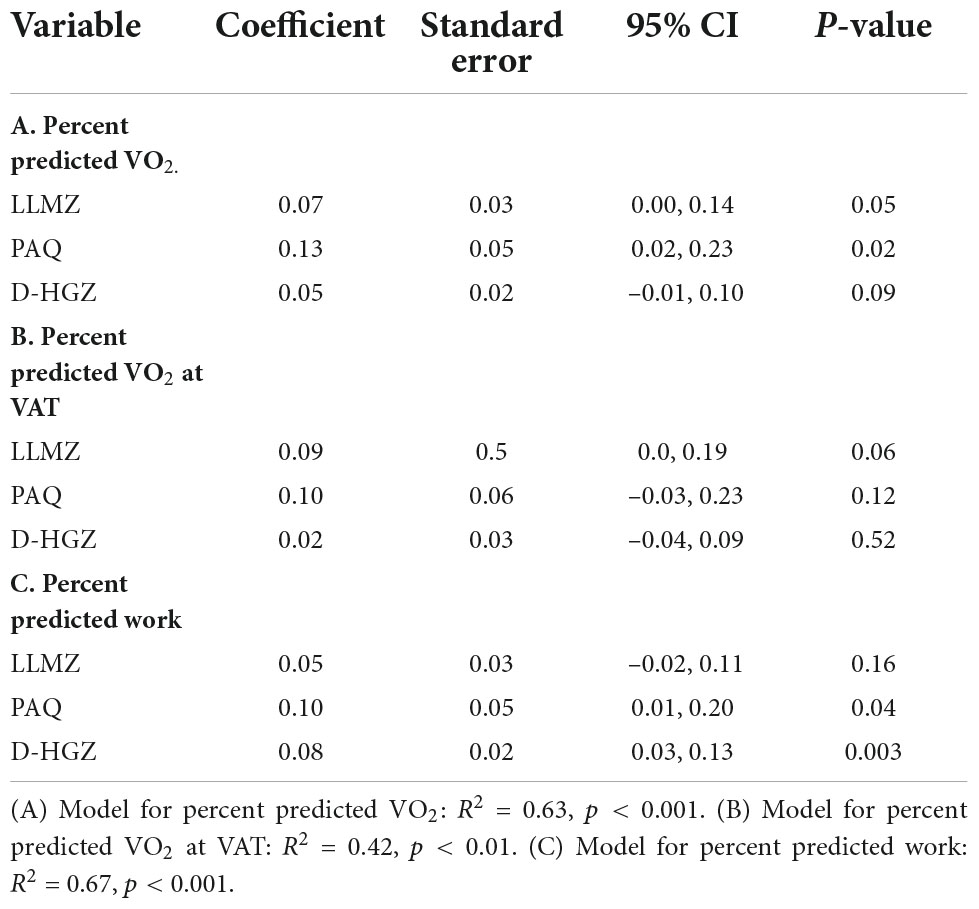
Table 5. Results from multiple linear regression models testing effect of skeletal muscle and physical activity variables on exercise performance.
There were no differences in LLMZ, PAQ, CPET, or eCMR findings in participants with Group 2 PH vs. other classifications. The study findings were unchanged when participants with Group 2 PH were excluded. There were some differences in LLMZ and exercise performance based on medication regimen. Participants on subcutaneous treprostinil had lower LLMZ (–2.02 ± 1.03 vs. –0.76 ± 1.06, p = 0.04), percent predicted VO2 (0.49 ± 0.11 vs. 0.75 ± 0.21, p = 0.02), percent predicted VO2 at VAT (0.54 ± 0.13 vs. 0.86 ± 0.17, p < 0.01), and percent predicted work (0.41 ± 0.10 vs. 0.63 ± 0.20, p = 0.05) compared to those not on subcutaneous treprostinil. Interestingly, participants on oral treprostinil demonstrated greater change in systemic flow with exercise [4.56 ± 1.56 (n = 3 with cCMR) vs. 2.02 ± 0.85 (n = 7 with eCMR), p < 0.01] and higher PAQ (2.3 ± 0.6 vs. 1.8 ± 0.6, p = 0.06), D-HGZ (–0.30 ± 1.62 vs. –1.67 ± 1.14, p = 0.03), and ND-HGZ (–0.02 ± 1.74 vs. –1.45 ± 1.28, p = 0.04) compared to those participants not on oral treprostinil. Participants on ambrisentan demonstrated lower percent predicted VO2 at VAT (0.73 ± 0.22 vs. 0.91 ± 0.13, p = 0.04) compared to those not on ambrisentan.
No adverse events occurred with exercise testing.
Discussion
In this study, we demonstrated marked deficits in skeletal muscle mass and strength in association with worse exercise performance on CPET and eCMR in youth with PH. Lower skeletal muscle mass was associated with physical inactivity. In regression models, skeletal mass, strength, and physical activity were positively associated with exercise performance on CPET. This study builds on our prior work in which lower LLMZ was associated with lower 6 MWD in youth with PH (7). These pilot data add to our appreciation of musculoskeletal abnormalities in pediatric PH and the potential impact of these deficits on exercise performance. Increased understanding of the peripheral determinants of exercise performance may identify targets for intervention trials.
The general “myopathy” seen in adult PH patients is an area of active investigation, but few studies have examined this issue in pediatric PH. PH is increasingly understood to be a systemic condition with metabolic, inflammatory, genetic, and epigenetic contributions (42). As one of the extra-cardiopulmonary manifestations of this condition, skeletal muscle dysfunction can be grouped into structural deficits, functional impairment, and molecular abnormalities with evidence from both human and animal studies (42). Structural deficits include reduced muscle fiber cross sectional area, lower proportion of type I fibers, decreased capillary density, lipid inclusion, and loss of mitochondrial structure (8, 10, 43). Functional impairment can manifest as reduced muscle strength, decreased muscular endurance, impaired oxygenation in the microcirculation, reduced type I fiber tension, and sarcomeric dysfunction (6, 8, 9, 44, 45). Molecular abnormalities have been described including reduced oxidative capacity, increased protein degradation and decreased protein synthesis, impaired angiogenesis, and impaired mitochondrial function (11, 46, 47). The spectrum of muscular abnormalities in children with PH is unknown. As pediatric PH often occurs in the context of congenital heart disease, developmental lung diseases, genetic differences, and other syndromic conditions, the findings may be even more severe. To our knowledge, no other investigators have explored decreased muscle mass and strength in children with PH. The data are essential to understanding the burden of disease across the lifespan and identifying appropriate timepoints for intervention. Future studies should explore the breath of these findings.
We demonstrated associations between muscle deficits and performance on CPET. CPET is a comprehensive assessment of a patient’s exercise performance that utilizes inspiratory and expiratory gas exchange to quantify peak oxygen consumption, carbon dioxide production, and minute ventilation (48). Peak VO2 is the most common indicator of a patient’s cardiorespiratory fitness. Adult patients with PH demonstrate decreased peak VO2, higher VE/VCO2 (ventilatory equivalents of carbon dioxide) indicating ventilatory inefficiency, lower arterial CO2 tension and end-tidal CO2 tension, lower O2 pulse (VO2/heart rate, a surrogate for stroke volume), and lower systemic oxygen saturation (49–51). Worse peak VO2 is associated with more symptoms of dyspnea and fatigue which limit quality of life. Both low peak VO2 and low 6 MWD are associated with mortality in adults with PAH and are incorporated into REVEAL 2.0 and European Society of Cardiology/European Respiratory Society risk stratification tools (52, 53). However, completion of a CPET requires significant developmental skills and reference values relative to outcomes are lacking in pediatric PH (54, 55). The lack of adverse events in this pilot study suggests that select older children and teenagers with PH can safely perform CPET and eCMR. Peak VO2 is included in pediatric PAH disease severity guidelines (56) and perhaps exercise parameters will be incorporated into novel pediatric risk stratification tools which are being developed. To our knowledge, this is the first study to express PH participants’ CPET performance relative to reference values from more than 1,800 healthy United States children published by members of our study team (28). Participants in our study generally performed at 60–80% predicted, suggesting children with PH already face marked limitations prior to progressive decline in adulthood. Additionally, this is the first report of eCMR in pediatric PH, although we have previously described eCMR in patients with single ventricle physiology after Fontan palliation (21). Higher PAQ and handgrip Z-scores were associated with greater change in systemic flow from rest to exercise, raising compelling questions regarding physical activity, strength, and the ability to augment systemic flow on exertion. Future, larger studies should also explore the correlation between eCMR performance and PH disease-specific outcomes.
While peak VO2 is a common outcome reported in research and clinical care, CPET measures of submaximal performance including VO2 at VAT and peak work rate relative to respiratory exchange ratio as well as measures of anaerobic strength may better reflect a patient’s daily activities and may also be targets for improvement. PH patients rapidly reach anaerobic threshold when performing activities of daily living such as doing laundry and folding clothes, cooking and setting the table, and walking outside with moderate effort (57, 58). These activities are often performed with short bursts of activity. The associations between muscle deficits, VO2 at VAT, and peak work rate in our study suggest that skeletal muscle deficits may impact everyday activity performance for PH patients and improvements in muscle mass or function could improve quality of life and other outcomes over longer periods. These findings are also consistent with our previous report associating lower LLMZ with lower 6 MWD, another test of submaximal exercise performance (7).
Cardiopulmonary rehabilitation improves symptoms and functional status in adult PH but the mechanisms underlying those improvements are incompletely understood. Some studies have demonstrated improvement in functional status and exercise performance without change in pulmonary hemodynamics. Data such as those from Bauer et al. (6) and Mainguy et al. (10) have supported development of exercise rehabilitation programs to improve muscle function and strength. In a separate study, Mainguy demonstrated increased 6 MWD, increased exercise duration on CPET, and improved VE/CO2 in association with decreased number of quadriceps type IIx fibers in 5 adult IPAH patients after a 12-week rehabilitation program (59). De Man also demonstrated increased quadriceps strength and endurance, increased quadriceps capillarization, and higher oxidative enzyme activity in 19 adult IPAH patients after a 12-week rehabilitation program (60). There is only one published report of exercise training in pediatric PH. Zöller et al. demonstrated increased treadmill running distance and improved VO2 at VAT after a 16-week home exercise program in 9 children with PH and functional class I and II (61). Skeletal muscle characterization was not part of that study. While PH patients perform less moderate to vigorous physical activity compared to healthy peers (62), no studies have tested interventions to improve activity. To our knowledge, no pediatric PH exercise trials have described changes in physical activity and/or skeletal muscle performance with training. Future studies should determine whether low skeletal muscle mass is a marker of disease severity or a modifiable determinant of exercise performance in youth with PH. It is not known whether increasing physical activity can augment skeletal muscle characteristics and improve exercise performance in youth with PH.
A primary limitation of this study is the lack of control group. It is not clear whether the relationships between LLMZ, PAQ score, and exercise performance are different in PH patients vs. healthy youth. Additionally, causal inferences cannot be made from these data given the study’s cross-sectional design. The small number of participants with certain medication regimens does not allow us to draw conclusions regarding relationships between medications, underlying disease status, and the study outcomes. The relationship between disease progression, muscle deficits, and exercise performance is also unknown. The study was also limited by lack of comparison reference data for lower extremity strength. The lack of association between leg strength and exercise performance may have been because we were unable to express strength relative to gender and body size. Finally, physical activity was measured by self-report. The analyses would have been strengthened by quantification of physical activity by wearable accelerometer. However, despite these limitations, this study has generated preliminary data for future observational studies and interventional trials.
Conclusion
Youth with PH demonstrate inactivity and marked skeletal muscle deficits in association with worse exercise performance on CPET and eCMR. Future studies should determine whether low skeletal muscle mass is a marker of disease severity or a modifiable target for exercise interventions. Interventions that improve skeletal muscle mass and function could improve exercise performance in this population.
Data availability statement
The raw data supporting the conclusions of this article will be made available by the authors, without undue reservation.
Ethics statement
The studies involving human participants were reviewed and approved by the Children’s Hospital of Philadelphia Institutional Review Board. Written informed consent to participate in this study was provided by the participants or their legal guardian/next of kin.
Author contributions
CA designed the study, recruited participants, supervised study procedures, analyzed and interpreted data, and wrote the manuscript. MM and SP supervised exercise testing procedures, interpreted exercise data, and reviewed the final manuscript. MH, KW, and MF supervised exercise cardiac magnetic resonance procedures, analyzed and interpreted the cardiac magnetic resonance data, and reviewed the final manuscript. BZ analyzed and interpreted anthropometry and densitometry data and reviewed the final manuscript. All authors contributed to the article and approved the submitted version.
Funding
This study was supported by the Acetelion Pharmaceuticals Young Investigator Program (CA).
Acknowledgments
We appreciate the contributions of study coordinators Sofia Saavedra, Alexis Mattei, and Dana Albizem, without whom this work would not be possible.
Conflict of interest
The authors declare that the research was conducted in the absence of any commercial or financial relationships that could be construed as a potential conflict of interest.
Publisher’s note
All claims expressed in this article are solely those of the authors and do not necessarily represent those of their affiliated organizations, or those of the publisher, the editors and the reviewers. Any product that may be evaluated in this article, or claim that may be made by its manufacturer, is not guaranteed or endorsed by the publisher.
Abbreviations
6 MWD, 6-min walk distance; BMDCS, Bone Mineral Density in Childhood Study; CPET, cardiopulmonary exercise test; DXA, densitometry; eCMR, exercise cardiac magnetic resonance; IPAH, idiopathic pulmonary arterial hypertension; LLMZ, leg lean mass Z-score; NHANES, National Health and Nutrition Examination Survey; PAQ, physical activity questionnaire; PH, pulmonary hypertension; VAT, ventilatory anaerobic threshold; VE/VCO2, ventilatory equivalents of carbon dioxide; VO2, oxygen consumption.
References
1. Barst RJ, Maislin G, Fishman AP. Vasodilator therapy for primary pulmonary hypertension in children. Circulation. (1999) 99:1197–208. doi: 10.1161/01.CIR.99.9.1197
2. Barst RJ, McGoon MD, Elliott CG, Foreman AJ, Miller DP, Ivy DD. Survival in childhood pulmonary arterial hypertension: insights from the registry to evaluate early and long-term pulmonary arterial hypertension disease management. Circulation. (2012) 125:113–22. doi: 10.1161/CIRCULATIONAHA.111.026591
3. Matura LA, McDonough A, Carroll DL. Cluster analysis of symptoms in pulmonary arterial hypertension: a pilot study. Eur J Cardiovasc Nurs. (2012) 11:51–61. doi: 10.1177/1474515111429649
4. Mullen MP, Andrus J, Labella MH, Forbes PW, Rao S, McSweeney JE, et al. Quality of life and parental adjustment in pediatric pulmonary hypertension. Chest. (2014) 145:237–44. doi: 10.1378/chest.13-0636
5. Tran DL, Lau EMT, Celermajer DS, Davis GM, Cordina R. Pathophysiology of exercise intolerance in pulmonary arterial hypertension. Respirology. (2018) 23:148–59. doi: 10.1111/resp.13141
6. Bauer R, Dehnert C, Schoene P, Filusch A, Bärtsch P, Borst MM, et al. Skeletal muscle dysfunction in patients with idiopathic pulmonary arterial hypertension. Respir Med. (2007) 101:2366–9. doi: 10.1016/j.rmed.2007.06.014
7. Avitabile CM, Saavedra S, Sivakumar N, Goldmuntz E, Paridon SM, Zemel BS. Marked skeletal muscle deficits are associated with 6-minute walk distance in paediatric pulmonary hypertension. Cardiol Young. (2021) 31:1426–33. doi: 10.1017/S1047951121000342
8. Breda AP, de Albuquerque ALP, Jardim C, Morinaga LK, Suesada MM, Fernandes CJ, et al. Skeletal muscle abnormalities in pulmonary arterial hypertension. PLoS One. (2014) 9:e114101. doi: 10.1371/journal.pone.0114101
9. Batt J, Ahmed SS, Correa J, Bain A, Granton J. Skeletal muscle dysfunction in idiopathic pulmonary arterial hypertension. Am J Respir Cell Mol Biol. (2014) 50:74–86. doi: 10.1165/rcmb.2012-0506OC
10. Mainguy V, Maltais F, Saey D, Gagnon P, Martel S, Simon M, et al. Peripheral muscle dysfunction in idiopathic pulmonary arterial hypertension. Thorax. (2010) 65:113–7. doi: 10.1136/thx.2009.117168
11. Tolle J, Waxman A, Systrom D. Impaired systemic oxygen extraction at maximum exercise in pulmonary hypertension. Med Sci Sports Exerc. (2008) 40:3–8. doi: 10.1249/mss.0b013e318159d1b8
12. Guyton AC, Douglas BH, Langston JB, Richardson TQ. Instantaneous increase in mean circulatory pressure and cardiac output at onset of muscular activity. Circ Res. (1962) 11:431–41. doi: 10.1161/01.RES.11.3.431
13. ATS Committee on Proficiency Standards for Clinical Pulmonary Function Laboratories. ATS statement: guidelines for the six-minute walk test. Am J Respir Crit Care Med. (2002) 166:111–7. doi: 10.1164/ajrccm.166.1.at1102
14. Morris M, Udry JR. Validation of a self-administered instrument to assess stage of adolescent development. J Youth Adolesc. (1980) 9:271–80. doi: 10.1007/BF02088471
15. Marino BS, Shera D, Wernovsky G, Tomlinson RS, Aguirre A, Gallagher M, et al. The development of the pediatric cardiac quality of life inventory: a quality of life measure for children and adolescents with heart disease. Qual Life Res. (2008) 17:613–26. doi: 10.1007/s11136-008-9323-8
16. Crocker PR, Bailey DA, Faulkner RA, Kowalski KC, McGrath R. Measuring general levels of physical activity: preliminary evidence for the physical activity questionnaire for older children. Med Sci Sports Exerc. (1997) 29:1344–9. doi: 10.1097/00005768-199710000-00011
17. Kowalski K, Crocker PRE, Faulkner RA. Validation of a physical activity questionnaire for older children. Pediatr Exerc Sci. (1997) 9:174–86. doi: 10.1123/pes.9.2.174
18. Kowalski K, Crocker PRE, Kowalski NP. Convergent validity of the physical activity questionnaire for adolescents. Pediatr Exerc Sci. (1997) 9:342–52. doi: 10.1123/pes.9.4.342
19. Saenger AK, Laha TJ, Bremmer DE, Sadrzadeh SM. Quantification of serum 25-hydroxyvitamin D(2) and D(3) using HPLC-tandem mass spectrometry and examination of reference intervals for diagnosis of vitamin D deficiency. Am J Clin Pathol. (2006) 125:914–20. doi: 10.1309/J32UF7GTQPWN25AP
20. Ross AC. The 2011 report on dietary reference intakes for calcium and vitamin D. Public Health Nutr. (2011) 14:938–9. doi: 10.1017/S1368980011000565
21. Avitabile CM, Goldberg DJ, Leonard MB, Wei ZA, Tang E, Paridon SM, et al. Leg lean mass correlates with exercise systemic output in young Fontan patients. Heart. (2018) 104:680–4. doi: 10.1136/heartjnl-2017-311661
22. Burnham JM, Shults J, Semeao E, Foster BJ, Zemel BS, Stallings VA, et al. Body-composition alterations consistent with cachexia in children and young adults with Crohn disease. Am J Clin Nutr. (2005) 82:413–20. doi: 10.1093/ajcn/82.2.413
23. Dougherty KA, Schall JI, Rovner AJ, Stallings VA, Zemel BS. Attenuated maximal muscle strength and peak power in children with sickle cell disease. J Pediatr Hematol Oncol. (2011) 33:93–7. doi: 10.1097/MPH.0b013e318200ef49
24. Lee DY, Wetzsteon RJ, Zemel BS, Shults J, Organ JM, Foster BJ, et al. Muscle torque relative to cross-sectional area and the functional muscle-bone unit in children and adolescents with chronic disease. J Bone Miner Res. (2015) 30:575–83. doi: 10.1002/jbmr.2375
25. Leggin BG, Neuman RM, Iannotti JP, Williams GR, Thompson EC. Intrarater and interrater reliability of three isometric dynamometers in assessing shoulder strength. J Shoulder Elbow Surg. (1996) 5:18–24. doi: 10.1016/S1058-2746(96)80026-7
26. Rowland T. Pediatric Laboratory Testing: Clinical Guidelines. Champaign, IL: Human Kinetics Publishers (1992).
27. Beaver WWK, Whipp B. A new method for detecting the anaerobic threshold by gas exchange. J Appl Physiol. (1986) 60:2020–7. doi: 10.1152/jappl.1986.60.6.2020
28. Burstein DS, McBride MG, Min J, Paridon AA, Perelman S, Huffman EM, et al. Normative values for cardiopulmonary exercise stress testing using ramp cycle ergometry in children and adolescents. J Pediatr. (2021) 229:60–9. doi: 10.1016/j.jpeds.2020.09.018
29. Paridon SM, Alpert BS, Boas SR, Cabrera ME, Caldarera LL, Daniels SR, et al. Clinical stress testing in the pediatric age group: a statement from the American heart association council on cardiovascular disease in the young, committee on atherosclerosis, hypertension, and obesity in youth. Circulation. (2006) 113:1905–20. doi: 10.1161/CIRCULATIONAHA.106.174375
30. Khiabani RH, Whitehead KK, Han D, Restrepo M, Tang E, Bethel J, et al. Exercise capacity in single-ventricle patients after Fontan correlates with haemodynamic energy loss in TCPC. Heart. (2015) 101:139–43. doi: 10.1136/heartjnl-2014-306337
31. Lee VS, Resnick D, Bundy JM, Simonetti OP, Lee P, Weinreb JC. Cardiac function: MR evaluation in one breath hold with real-time true fast imaging with steady-state precession. Radiology. (2002) 222:835–42. doi: 10.1148/radiol.2223011156
32. Kühl HP, Spuentrup E, Wall A, Franke A, Schröder J, Heussen N, et al. Assessment of myocardial function with interactive non-breath-hold real-time MR imaging: comparison with echocardiography and breath-hold Cine MR imaging. Radiology. (2004) 231:198–207. doi: 10.1148/radiol.2311021237
33. Wei Z, Whitehead KK, Khiabani RH, Tree M, Tang E, Paridon SM, et al. Respiratory effects on Fontan circulation during rest and exercise using real-time cardiac magnetic resonance imaging. Ann Thorac Surg. (2016) 101:1818–25. doi: 10.1016/j.athoracsur.2015.11.011
34. Avitabile CM, Leonard MB, Zemel BS, Brodsky JL, Lee D, Dodds K, et al. Lean mass deficits, vitamin D status and exercise capacity in children and young adults after Fontan palliation. Heart. (2014) 100:1702–7. doi: 10.1136/heartjnl-2014-305723
35. Ogden CL, Kuczmarski RJ, Flegal KM, Mei Z, Guo S, Wei R, et al. Centers for disease control and prevention 2000 growth charts for the United States: improvements to the 1977 National Center for Health Statistics version. Pediatrics. (2002) 109:45–60. doi: 10.1542/peds.109.1.45
36. Zemel BS, Kalkwarf HJ, Gilsanz V, Lappe JM, Oberfield S, Shepherd JA, et al. Revised reference curves for bone mineral content and areal bone mineral density according to age and sex for black and non-black children: results of the bone mineral density in childhood study. J Clin Endocrinol Metab. (2011) 96:3160–9. doi: 10.1210/jc.2011-1111
37. Kalkwarf HJ, Zemel BS, Gilsanz V, Lappe JM, Horlick M, Oberfield S, et al. The bone mineral density in childhood study: bone mineral content and density according to age, sex, and race. J Clin Endocrinol Metab. (2007) 92:2087–99. doi: 10.1210/jc.2006-2553
38. Cole TJ. The LMS method for constructing normalized growth standards. Eur J Clin Nutr. (1990) 44:45–60.
39. Ploegstra MJ, Ivy DD, Wheeler JG, Brand M, Beghetti M, Rosenzweig EB, et al. Growth in children with pulmonary arterial hypertension: a longitudinal retrospective multiregistry study. Lancet Respir Med. (2016) 4:281–90. doi: 10.1016/S2213-2600(16)00069-2
40. Zemel BS, Leonard MB, Kelly A, Lappe JM, Gilsanz V, Oberfield S, et al. Height adjustment in assessing dual energy x-ray absorptiometry measurements of bone mass and density in children. J Clin Endocrinol Metab. (2010) 95:1265–73. doi: 10.1210/jc.2009-2057
41. Stasinopoulos DM, Rigby RA. Generalized additive models for location scale and shape (GAMLSS) in R. J Statist Softw. (2007) 23:1–46. doi: 10.18637/jss.v023.i07
42. Nickel NP, Yuan K, Dorfmuller P, Provencher S, Lai YC, Bonnet S, et al. Beyond the lungs: systemic manifestations of pulmonary arterial hypertension. Am J Respir Crit Care Med. (2020) 20:148–57. doi: 10.1164/rccm.201903-0656CI
43. Potus F, Malenfant S, Graydon C, Mainguy V, Tremblay È, Breuils-Bonnet S, et al. Impaired angiogenesis and peripheral muscle microcirculation loss contribute to exercise intolerance in pulmonary arterial hypertension. Am J Respir Crit Care Med. (2014) 190:318–28. doi: 10.1164/rccm.201402-0383OC
44. Malenfant S, Potus F, Mainguy V, Leblanc E, Malenfant M, Ribeiro F, et al. Impaired skeletal muscle oxygenation and exercise tolerance in pulmonary hypertension. Med Sci Sports Exerc. (2015) 47:2273–82. doi: 10.1249/MSS.0000000000000696
45. Sithamparanathan S, Rocha MC, Parikh JD, Rygiel KA, Falkous G, Grady JP, et al. Skeletal muscle mitochondrial oxidative phosphorylation function in idiopathic pulmonary arterial hypertension: in vivo and in vitro study. Pulm Circ. (2018) 8:2045894018768290. doi: 10.1177/2045894018768290
46. Malenfant S, Potus F, Fournier F, Breuils-Bonnet S, Pflieger A, Bourassa S, et al. Skeletal muscle proteomic signature and metabolic impairment in pulmonary hypertension. J Mol Med. (2015) 93:573–84. doi: 10.1007/s00109-014-1244-0
47. McCullough DJ, Kue N, Mancini T, Vang A, Clements RT, Choudhary G. Endurance exercise training in pulmonary hypertension increases skeletal muscle electron transport chain supercomplex assembly. Pulm Circ. (2020) 10:2045894020925762. doi: 10.1177/2045894020925762
48. Guazzi M, Adams V, Conraads V, Halle M, Mezzani A, Vanhees L, et al. EACPR/AHA Joint Scientific Statement. Clinical recommendations for cardiopulmonary exercise testing data assessment in specific patient populations. Eur Heart J. (2012) 33:2917–27. doi: 10.1093/eurheartj/ehs221
49. Sun XG, Hansen JE, Oudiz RJ, Wasserman K. Exercise pathophysiology in patients with primary pulmonary hypertension. Circulation. (2001) 104:429–35. doi: 10.1161/hc2901.093198
50. Arena R, Lavie CJ, Milani RV, Myers J, Guazzi M. Cardiopulmonary exercise testing in patients with pulmonary arterial hypertension: an evidence-based review. J Heart Lung Transplant. (2010) 29:159–73. doi: 10.1016/j.healun.2009.09.003
51. Weatherald J, Farina S, Bruno N, Laveneziana P. Cardiopulmonary exercise testing in pulmonary hypertension. Ann Am Thorac Soc. (2017) 14(Suppl. 1):S84–92. doi: 10.1513/AnnalsATS.201610-788FR
52. Benza RL, Gomberg-Maitland M, Elliott CG, Farber HW, Foreman AJ, Frost AE, et al. Predicting survival in patients with pulmonary arterial hypertension: the REVEAL Risk Score Calculator 2.0 and comparison with ESC/ERS-based risk assessment strategies. Chest. (2019) 156:323–37. doi: 10.1016/j.chest.2019.02.004
53. Hoeper MM, Kramer T, Pan Z, Eichstaedt CA, Spiesshoefer J, Benjamin N, et al. Mortality in pulmonary arterial hypertension: prediction by the 2015 European pulmonary hypertension guidelines risk stratification model. Eur Respir J. (2017) 50:1700740. doi: 10.1183/13993003.00740-2017
54. Rosenzweig EB, Abman SH, Adatia I, Beghetti M, Bonnet D, Haworth S, et al. Paediatric pulmonary arterial hypertension: updates on definition, classification, diagnostics and management. Eur Respir J. (2019) 53:1801916. doi: 10.1183/13993003.01916-2018
55. Sun H, Stockbridge N, Ariagno RL, Murphy D, Nelson RM, Rodriguez W. Reliable and developmentally appropriate study end points are needed to achieve drug development for treatment of pediatric pulmonary arterial hypertension. J Perinatol. (2016) 36:1029–33. doi: 10.1038/jp.2016.103
56. Abman SH, Hansmann G, Archer SL, Ivy DD, Adatia I, Chung WK, et al. Pediatric pulmonary hypertension: guidelines from the American heart association and american thoracic society. Circulation. (2015) 132:2037–99. doi: 10.1161/CIR.0000000000000329
57. Malenfant S, Lebret M, Breton-Gagnon É, Potus F, Paulin R, Bonnet S, et al. Exercise intolerance in pulmonary arterial hypertension: insight into central and peripheral pathophysiological mechanisms. Eur Respir Rev. (2021) 30:200284. doi: 10.1183/16000617.0284-2020
58. Nayor M, Houstis NE, Namasivayam M, Rouvina J, Hardin C, Shah RV, et al. Impaired exercise tolerance in heart failure with preserved ejection fraction: quantification of multiorgan system reserve capacity. JACC Heart Fail. (2020) 8:605–17. doi: 10.1016/j.jchf.2020.03.008
59. Mainguy V, Maltais F, Saey D, Gagnon P, Martel S, Simon M, et al. Effects of a rehabilitation program on skeletal muscle function in idiopathic pulmonary arterial hypertension. J Cardiopulm Rehabil Prev. (2010) 30:319–23. doi: 10.1097/HCR.0b013e3181d6f962
60. de Man FS, Handoko ML, Groepenhoff H, van’t Hul AJ, Abbink J, Koppers RJ, et al. Effects of exercise training in patients with idiopathic pulmonary arterial hypertension. Eur Respir J. (2009) 34:669–75. doi: 10.1183/09031936.00027909
61. Zöller D, Siaplaouras J, Apitz A, Bride P, Kaestner M, Latus H, et al. Home exercise training in children and adolescents with pulmonary arterial hypertension: a pilot study. Pediatr Cardiol. (2017) 38:191–8. doi: 10.1007/s00246-016-1501-9
Keywords: pediatric pulmonary hypertension, skeletal muscle, exercise, densitometry, cardiac magnetic resonance imaging
Citation: Avitabile CM, McBride MG, Harris MA, Whitehead KK, Fogel MA, Paridon SM and Zemel BS (2022) Skeletal muscle deficits are associated with worse exercise performance in pediatric pulmonary hypertension. Front. Pediatr. 10:1025420. doi: 10.3389/fped.2022.1025420
Received: 22 August 2022; Accepted: 14 September 2022;
Published: 05 October 2022.
Edited by:
Michael Portman, University of Washington, United StatesReviewed by:
Nicolas Madsen, University of Texas Southwestern Medical Center, United StatesBrian Soriano, Seattle Children’s Hospital, United States
Copyright © 2022 Avitabile, McBride, Harris, Whitehead, Fogel, Paridon and Zemel. This is an open-access article distributed under the terms of the Creative Commons Attribution License (CC BY). The use, distribution or reproduction in other forums is permitted, provided the original author(s) and the copyright owner(s) are credited and that the original publication in this journal is cited, in accordance with accepted academic practice. No use, distribution or reproduction is permitted which does not comply with these terms.
*Correspondence: Catherine M. Avitabile, avitabilec@chop.edu