- Division of General and Thoracic Surgery, Translational Medicine Program, The Hospital for Sick Children, Toronto, ON, Canada
Necrotizing enterocolitis (NEC) is a gastrointestinal disease frequently prevalent in premature neonates. Despite advances in research, there is a lack of accurate, early diagnoses of NEC and the current therapeutic approaches remain exhausted and disappointing. In this review, we have taken a close look at the regenerative medical literature available in the context of NEC treatment. Stem cells from amniotic fluid (AFSC) administration may have the greatest protective and restorative effects on NEC. This review summarizes the potential protection and restoration AFSCs have on NEC-induced intestinal injury while comparing various components within AFSCs like conditioned medium (CM) and extracellular vesicles (EVs). In addition to therapeutic interventions that focus on targeting intestinal epithelial damage and regeneration, a novel discovery that AFSCs act in a Wnt-dependent manner provides insight into this mechanism of protection. Finally, we have highlighted the most important aspects that remain unknown that should be considered to guide future research on the translational application of AFSC-based therapy. We hope that this will be a beneficial frame of reference for the guidance of future studies and towards the clinical application of AFSC and/or its derivatives as a treatment against NEC.
Introduction
Necrotizing enterocolitis (NEC) is a gastrointestinal disease frequently prevalent in preterm neonates. Of those, 5%–12% of very-low birth weights are at an increased risk (1). Risk factors include intestinal immaturity, impaired microvascular circulation, abnormal intestinal microbiota, and a highly immunoreactive intestinal mucosa which may lead to necrosis (1–3). With advances in the management of preterm neonates, the overall incidence of NEC has decreased from 6.6% in 2007 to 3.9% in 2013 (4). However, there remains a difficulty in accurate, early diagnoses, and innovative treatment of NEC resulting in 27%–52% of very-low birth weight infants with NEC developing advanced NEC and requiring surgical intervention and approximately half of the infants developing neurodevelopmental damage (5). The mortality of advanced NEC (30%–50%) remains one of the highest in neonatology (4). The impact NEC has on the overall quality of life of patients and families is detrimental as diagnosis, treatment, long-term outcomes, and the cumbersome financial burden have remained stagnant throughout the past decade, despite improvements in neonatal intensive care. The cost of ongoing treatment has averaged to be $500 million to $1 billion per year in the United States (6).
In recent years, investigation into preventative actions have shown to be promising in reducing the incidence, morbidity and mortality of the disease (3). Breast milk is a significant factor in countering the occurrence of NEC (7). Research has identified components of the breast milk including milk-derived exosomes (8, 9) and human milk oligosaccharides (HMOs) (10) that regulate this beneficial effect. The use of prophylactic probiotics, prebiotics, and synbiotics administered to premature neonates is another plausible strategy to prevent the onset of NEC (11). Lastly, recent advances in remote ischemic conditioning (RIC) application may prevent the progression of NEC, avoiding irreversible damage to the neonate (12). However, the exact pathophysiology and “curative” treatment strategy remains unknown. Therefore, experimental and clinical research continue to investigate the pathogenesis of NEC and develop novel treatments to avoid disease progression and alleviate morbidity and mortality.
The pioneering of regenerative medicine has become a novel tool in understanding the pathogenesis of many diseases and development of treatment strategies (13). Their mechanism of action via cell surface markers and release of transcription factors grants self-renewal capabilities (14, 15). The administration of stem cells can regenerate tissue administered in experimental and clinical settings. In inflammatory bowel disease (IBD), evidence demonstrates successful autologous stem cell transplantation and diminished intestinal damage (16).
Recent research has suggested that stem cells, specifically amniotic fluid stem cells (AFSCs), can potentiate the treatment effects of experimental NEC. In this article, we will review the current evidence and discuss how promising regenerative medicine and stem cell therapy may be on the clinical translation of AFSC-based therapy against NEC. Moreover, we have highlighted aspects that remain unknown, which should be considered to guide future research of the translational application of AFSC-based therapy.
Discovery of amniotic fluid stem cell potential in NEC
Amniotic fluid in the context of NEC
During gestation, the fetus is encapsulated by amniotic fluid (AF), which is principally composed of water and solutes (17). These solutes include a variety of trophic factors, cytokines and growth factors that modify intestinal nutrient absorption and development, increase enterocyte proliferation, migration and differentiation, prevent apoptosis and promote mucosal restoration. Ultimately, these solutes reduce intestinal injury and aid in the protection against NEC (18). In addition, AF contains antimicrobial proteins and peptides (APPs) that support the microbial activity observed. Though often discarded as “biological waste”, due its properties described, AF has been hypothesized to protect the fetus from gastrointestinal impairment and supports stable growth and differentiation. It has been reported that Toll-like receptor 4 (TLR4) activation leads to mucosal injury via increased enterocyte apoptosis in NEC (19). Interestingly, in 2012, Good et al. demonstrated that AF inhibits TLR4 signalling in the neonatal intestinal epithelium (20). This study provides the foundation to amniotic fluid's future application in NEC prevention and treatment.
Discovery of amniotic fluid stem cell potential in NEC
Evidence of the potential of stem cell therapy against intestinal diseases has been a growing area of research including NEC. Adult stem cells, such as the mesenchymal stem cells (MSCs), were of the first to demonstrate protection from experimental NEC in rats in 2011 (21).
Moreover, De Coppi et al. discovered a stem cell derived from amniotic fluid in 2007 (22) (Figure 1). These cells demonstrated pluripotency through the confirmation of Oct-4, a marker for pluripotent human stem cells (23). The pluripotent nature of these cells include: the ability to differentiate into the three germ layers in vitro, to be cultured infinitely in an undifferentiated state, and to produce clonal lineages and teratomas in vivo (24, 25). Remarkably, AFSCs do not have the same detrimental effects as embryonic stem cells (ESCs) and adult stem cells (26). Moreover, they show greater immunosuppressive function and are capable of greater maintenance of pluripotency (27). Furthermore, AFSCs are not bound by the legal and ethical limitations that ESCs are subject to. They prove to be the more attractive stem cell for clinical use due to their greater differentiation capacity and plasticity, lower immunogenicity, and lack of tumorigenicity and ethical concerns (28).
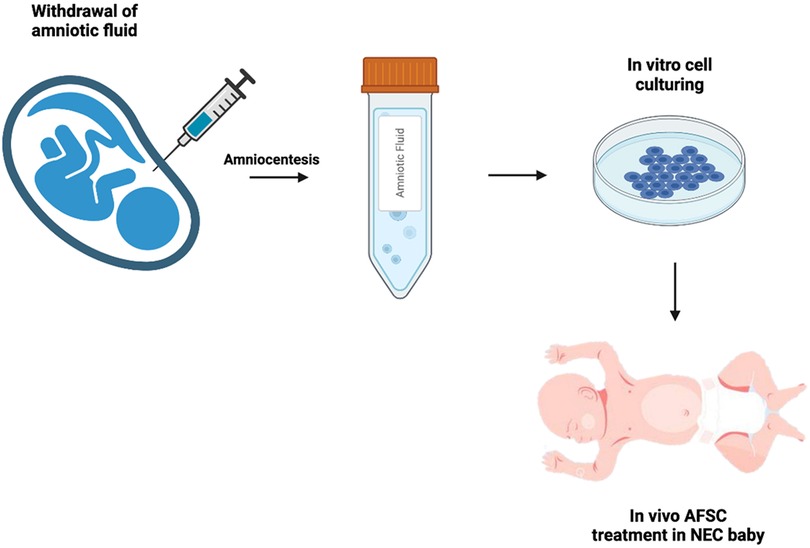
Figure 1. Schematic of AFSC collection and administration. Following amniocentesis, amniotic fluid stem cells are cultured from the amniotic fluid, then administered to the neonate suffering from NEC.
Extraordinarily, AFSC-based therapy against NEC was first discovered by Zani et al. in 2013 (25). AFSCs injected in experimental NEC successfully integrated into the bowel wall improving survival, clinical status, and gut structure and function. Moreover, it was shown that AFSCs function via a cyclooxygenase 2 (COX-2) dependent mechanism. These unique therapeutic effects and promising potential launched the focus on AFSC-based therapy against NEC.
Direct comparison of AFSC and other stem cells in NEC
Several experimental animal NEC studies have been conducted to identify the efficacy of different types of stem cells, such as amniotic fluid-derived stem cells (AFSC), bone marrow-derived MSC (BM-MSC), or enteric neural stem cells (E-NSC). McCulloh et al. compared these stem cell types and found that, generally, all demonstrate a reduction in the incidence and severity of NEC (29, 30). In addition, they found a similar rescue of barrier function in the injured intestine (29). However, the individual mechanisms of action remain unknown. AFSC are fibroblast-like, pluripotent stem cells with a great capacity for differentiation and immunomodulation (31). Their amniotic fluid origin renders them less tumorigenic and immunogenic compared to the other stem cells. BM-MSC function is dependent on modulation of stromal cells; however, these BM-MSC lack the potential to be effective in prolonging survival in the experimental NEC model (25, 32). E-NSC are stem cells that are a part of the enteric nervous system (ENS) that differentiate into neurons and glial cells (33). It has been proposed that ENS immaturity may predispose premature neonates to NEC, but with neuro-transplantation of E-NSCs, the injured ENS can be rebuilt.
Both AFSC and MSC have been investigated as potential treatment strategies in lowering NEC incidence and reducing the intestinal inflammation. However, AFSCs, not MSCs, administered prior to the onset of NEC have beneficial effects on prevention of intestinal epithelial injury (34), which is in line with a previous study comparing AFSCs and MSCs (30). In addition, AFSCs can “home” in on the intestinal epithelia while MSCs fail to do the same (25). Proteomics analysis of both AFSCs and MSCs suggested that AFSCs are primarily involved in cell development and growth, while MSCs are more immunomodulatory within the gut. These findings further support previous evidence that MSCs are effective in other inflammatory gastrointestinal diseases (35, 36). The protective effects of AFSCs in reducing intestinal damage were accompanied by increasing epithelial cell and intestinal stem cell proliferation, which results in epithelial regeneration. Remarkably, AFSCs were found to protect a healthy gastrointestinal tract with unseen damage compared to MSCs. These findings present AFSCs to be the more protective and attractive option for clinical use in NEC via stable intestinal proliferation, multipotency, and immunomodulatory abilities.
Mechanism of action: amniotic fluid stem cell potential in NEC
Upon retrieval of the AF from the pregnant mother donor via amniocentesis, the AFSCs are isolated in cultured medium then administered via an intraperitoneal injection. The AFSCs can integrate into the injured bowel acting on the damaged intestinal area due to its preferential attraction to areas of intestinal injury. There are various modes of action by AFSCs (Figure 2); to elucidate these effects we refer to the various elements of NEC pathophysiology. The development of NEC includes intestinal barrier dysfunction, ER stress associated apoptosis, inflammation, and decreased mucosal regeneration. AFSCs can restore lost function and rescue the injured intestine.
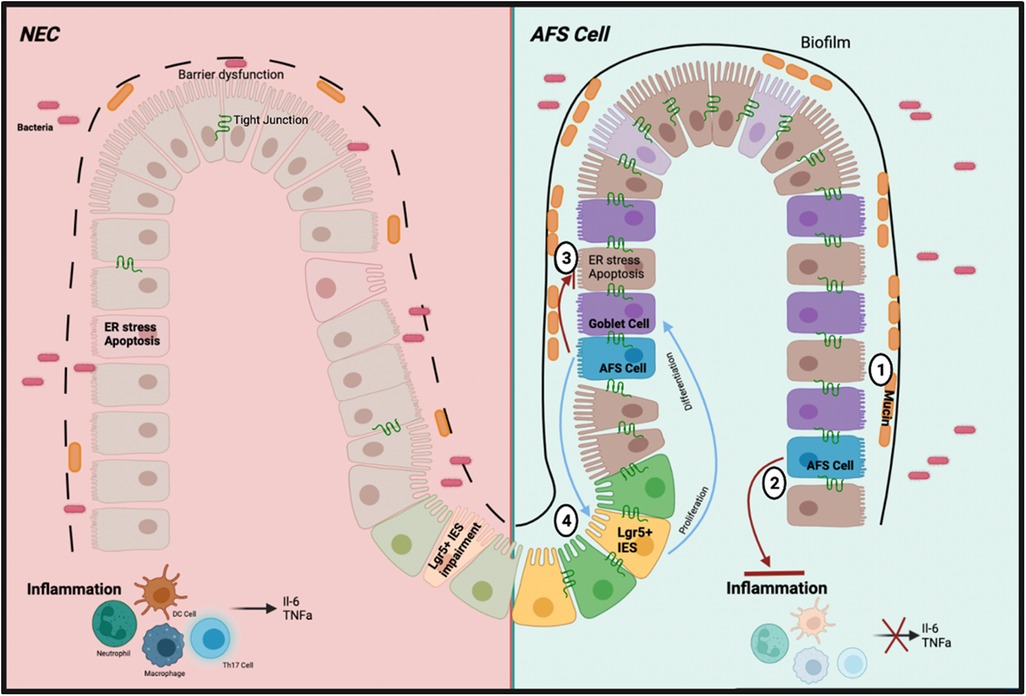
Figure 2. Schematic of the mechanism of action of AFSC in preclinical study. (1) Increased production of mucin levels by goblet cells due to increased differentiation (2) Inhibited inflammatory pathways and subsequent cytokines (3) Inhibited ER stress response and subsequent apoptosis (4) Restored function of ISCs to induce cell proliferation and differentiation.
Barrier dysfunction
The intestinal epithelial injury seen in NEC involves a weakened integrity of the epithelial barrier, thus allowing for the translocation of potentially harmful intestinal bacteria into circulation, leading to the inflammation observed (37). The epithelial barrier contains tight junctions (TJs) which serve as a blockade for control over the translocation of microbial products into the gut wall (38). Claudin 2 is an ileum TJ protein important in the paracellular transport of cations and water. A previous study found this TJ protein to be elevated in the urine and decreased in the intestinal tissue of neonatal NEC patients, creating selective pores within the epithelial wall contributing to the weakened integrity of the epithelial barrier. Moreover, Claudin 7, another TJ protein, is an important indicator of intestinal barrier injury and decreased expression is exhibited in NEC (39). With this knowledge, a recent study identified further insight into pathogenesis by experimentally attenuating disrupted intestinal barrier function during NEC-induced intestinal injury via administration of AFSCs (40).
Endoplasmic Reticulum (er) stress and its induced apoptosis
The ER stress response becomes activated upon accumulation of misfolded or unfolded proteins leading to decreased synthesis of functional proteins (41). To cope with ER stress, an adaptive response attempts to restore protein homeostasis and ER function via the unfolded protein response (UPR), governed by the ER stress central regulatory protein binding immunoglobulin protein (BiP). BiP controls three downstream ER transmembrane stress sensor proteins: inositol-requiring enzyme 1α (IRE1α), activating transcription factor 6 (ATF6) and pancreatic endoplasmic reticulum kinase (PERK). Furthermore, BiP activates these proteins with anti-apoptotic properties and promotes cell survival. However, prolonged ER stress activates the C/EBP homologous protein (CHOP), a part of both pro- and anti-apoptotic pathways which include encoding genes within the BCL2-family proteins. Since NEC is associated with increased intestinal epithelial cell and TJ apoptosis, inhibiting apoptotic signaling via CHOP activation may rescue barrier function (42).
It has been shown that ex vivo intestinal organoid model of NEC epithelial permeability and TJ disruption following NEC-induced injury were prevented by AFSCs (40). Claudin 2 expression was upregulated, while Claudin 7 was downregulated. In addition, the three ER stress sensor proteins (ATF6, IRE1α, PERK) were elevated in these AFSC-treated organoids, thus leading to the upregulation of activating transcription factor 4 (ATF4), which is important in the activation of CHOP and the pro-apoptotic pathway (40). Interestingly, the central controller, BiP, remained unaffected. Further, Li et al. analyzed whether the epithelial barrier restoration is dependent upon ER stress activity through administration of HA15, an inhibitor of the ER stress response, to the organoids (40). Any rescue observed by AFSCs was reverted to NEC injury phenotype, therefore AFSC-mediated intestinal barrier protection is dependent on the ER stress response. Remarkably, cell necrosis and apoptosis were reduced following AFSC treatment. Thus, AFSC-mediated anti-apoptotic effects are dependent on the ER stress response. In an in vivo NEC model, TJ function was restored, rescuing intestinal barrier function (40). Like the ex vivo model, AFSC-mediated ER stress was activated, while sequestering apoptotic effects. This differential effect of the ER stress response occurs because of different intestinal damage intensity points. When damage is at its highest, ER stress markers that would normally increase apoptosis decline. AFSCs proves to rescue intestinal permeability in experimental NEC via AFSC-induced ER stress without inducing apoptosis.
Immunological regulations
Intestinal inflammation is another common marker of NEC, which is closely correlated with barrier dysfunction, ER stress, and apoptosis. Upon intestinal injury, endotoxins released from intra-luminal bacteria bind to Toll-like receptor 4 (TLR4) present on intestinal epithelial cells (3). TLR4 expression is increased on intestinal epithelial cells in premature neonates (3) and recognize gram-negative lipopolysaccharide (LPS). Once bound to LPS, TLR4 activates pathogen-associated molecular pattern (PAMP) receptors further inducing the destruction of the gut barrier and allows for the observed intestinal permeability. The increased ability of bacterial translocation results in both a heightened innate and adaptive immune response, recruiting macrophages, dendritic cells, neutrophils, tumor necrosis factor-alpha (TNFα), IL-6 and other inflammatory cytokines. Additionally, the disruption of the balance between T helper 17 (Th17) cells and FoxP3+ regulatory T (Treg) cells further characterizes this inflammatory phenotype (43). In NEC, a decrease in Treg cells disrupts the equilibrium and increases proinflammatory Th17 cell response (44). However, when AFSCs are administered, TLR4-mediated signaling is inhibited (44) and inflammatory markers such as TNFα and IL-6 are significantly decreased (45).
Stem cell and regeneration ability
The rescue of intestinal stem cell (ISC) function and differentiation is paramount to intestinal epithelial rescue and impairment as a lack of cell proliferation and differentiation grants further destruction of epithelial integrity. Leucine-rich repeat-containing G-protein coupled receptor 5 (Lgr5) is a well-studied stem cell proliferation marker. During homeostasis, Lgr5+ ISCs located at the crypt base are responsible for mediating the constant renewal of the intestinal epithelium. These stem cells undergo self-renewal to maintain an undifferentiated ISC reservoir, and differentiation to give rise to major intestinal epithelial cell types, such as absorptive enterocytes, goblet cells, Paneth cells, tuft cells and enteroendocrine cells (46).
In premature infants there is a decrease in Lgr5+ ISCs which inhibits intestinal epithelial reconstitution and increases NEC susceptibility (47). AFSC medicated intestinal recovery via Wnt signaling can be attributed to canonical Wnt pathways as demonstrated by blocking Wnt signaling via inhibitors of Porcupine (Porcn) protein (44). It has been well established that transforming growth factor β1 (TGFβ1) activates the canonical Wnt signaling (48). TGF-β1 is downregulated in NEC and has been suggested to be used as a biomarker for early diagnosis and to assess disease severity (49). Intestinal stem cell Lgr5+ is upregulated in the embryonic intestinal specimens (50) and gastrointestinal cancer (51) exposed to an exogenous TGFβ1, which is in line with studies demonstrating that AFSC upregulated the expression of Lgr5 through the Wnt signalling pathway.
The administration of AFSCs rescued ISC function, the differentiation of goblet cells is readily increased, thus the creation of mucins to restore gut function and protection (44). Thus, AFSCs ultimately promote intestinal recovery by activating the Wnt/β-catenin signaling pathway to increase Lgr5+ ISCs, reduce inflammation and regenerate the intestinal epithelium (44).
AFSC derivatives in NEC
Although AFSCs improved safety, accessibility and ethical requirement of stem cell therapy, concerns continue to arise in clinical translation due to undesired differentiation, possible pro-tumorigenic and other detrimental effects (28). Thus, research has shifted its focus more to derivatives of the AFSC. For example, administration of the conditioned medium (CM) or extracellular vesicles (EVs) derived from AFSCs provide the same benefit as administering the stem cells themselves (52, 53).
Conditioned Medium (CM)
CM is essentially a cell-free “soup” of factors secreted by cells, including growth factors, cytokines, enzymes, nucleic acids and bioactive lipids (54). However, a gap in knowledge remained whether CM cultured with AFSCs would provide the same alleviation in experimental NEC.
A recent study demonstrated that administration of human AFSC conditioned medium (hAFSC-CM) improved NEC survival and reduced intestinal injury (45). Specifically, intestinal mucosal inflammation and epithelial apoptosis were significantly reduced. Remarkably, hAFSC-CM restored intestinal regeneration capabilities via increased expression of epithelial proliferation and intestinal stem cell markers. In addition, intestinal microvasculature and angiogenesis was restored.
Moreover, the secretome of the hAFSC-CM was assessed to gain a further understanding of its composition to explain the beneficial effects within in vivo models of NEC. Over 1500 proteins were found in the CM, mostly enriched by exosomes. In addition, several clusters included immunomodulation, cell cycle and stem cell regulation, which is in line with study results previously described. Interestingly, a large functional cluster of this secretome analysis was vesicle-mediated transport, which led to the interest in the function of these extracellular vesicles in the context of NEC.
Extracellular vesicles (EV)
The paracrine communication of AFSCs, as widely discussed in previous research findings, has now been accredited to the function of EVs (55–58). EVs are membrane-limited vesicles released from the outward budding of plasma membranes into the extracellular space with the capacity of intercellular communication and component exchange (59, 60). Some of these components include proteins and nucleic acids, reflecting the phenotype of parental cells (61). AFSC-CM, as previously described, has beneficial effects on experimental models of NEC, which include the paracrine function of these EVs. Recent studies went further and established that EVs alone were efficacious in the treatment of experimental NEC (9, 62).
Experimental NEC-induced intestinal damage was rescued via administration of human AFSC-derived EVs (hAFSC-EVs) (63). This study focused, however, on the most known affected intestinal area in experimental NEC, the ileum. In this experimental model, EVs improved ileal morphology, decreased markers ileal inflammation (IL-6; TNFα), increased ileal proliferation and regeneration by rescuing the stem cell niche, which is in line with their previous work on hAFSC-CM.
A recent study indicates that AFSC-EVs counteract NEC-induced intestinal injury through the stimulation of the Wnt/β-catenin signaling pathway (Figure 3) (44). It is known that intestinal stem cell (ISC) impairment is attributed to a lack of Wnt signaling in NEC pathogenesis (48). In an ex vivo NEC model, following hAFSC-EV administration, ISC and epithelial proliferation were increased via Wnt signaling in intestinal organoids (44). In an in vivo NEC model, Wnt-producing AFSCs administered to mouse pups were able to rescue epithelial injury, reduce inflammation and increase ISC and epithelial proliferation (44). However, Wnt-deficient AFSCs administered to mouse pups did not show these same results. Interestingly, AFSC-secreted factors were both able to increase ISC activity and Wnt signaling in a healthy and injured gut. Furthermore, when administering AFSC-EVs to both ex vivo and in vivo NEC models, similar results were observed as previously described. In addition, the authors concluded that timing is significant in EV-induced intestinal recovery, even though they were unable to prevent this injury (44).
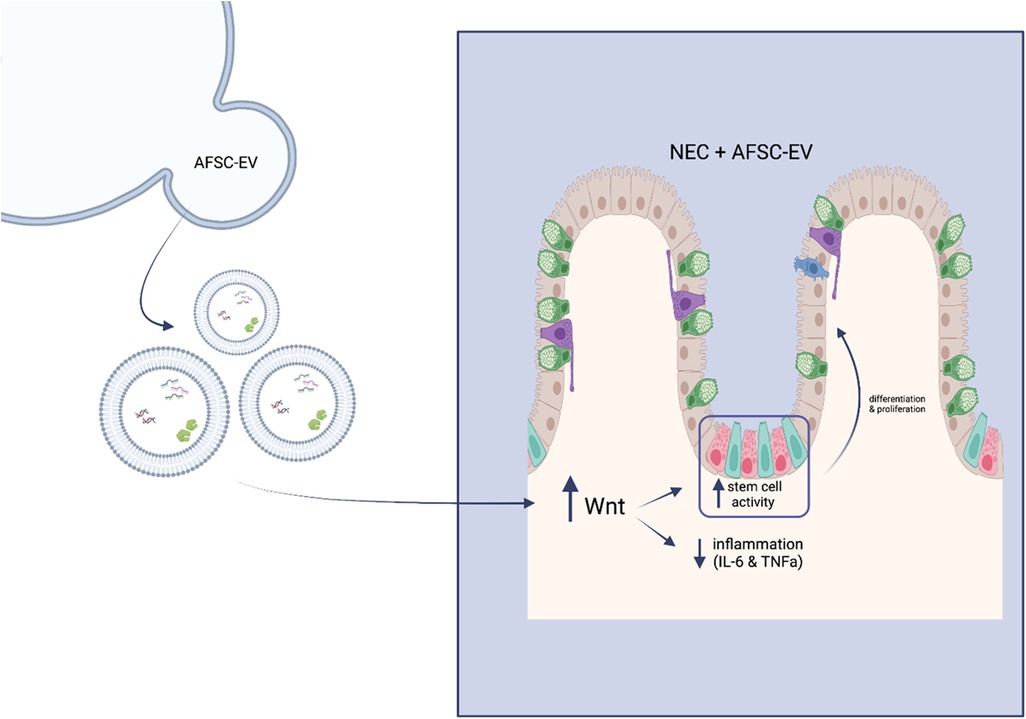
Figure 3. Schematic of mechanism of action of hAFSC-EVs. Wnt signalling becomes enhanced upon administration of hAFSC-EVs, which in turn increases stem cell activity and decreases inflammatory responses.
Challenges of clinical translation of AFSC-based therapy in NEC: What's next?
Overall, AFSC-based therapy has proven to be a novel therapeutic option in the context of experimental NEC. The pluripotent nature of these cells allows for their vast differentiation capabilities. In addition to its direct benefits to patients, the significant reduction of ethical issues of this less invasive tool in comparison to embryonic stem cells and other stem cell origins increases its attractiveness in tissue engineering and treatment of suffering neonates. Though there is a significant amount of evidence proving AFSCs to be valuable in the treatment of NEC, there remains a lack of clinical trials as indicated by our recent search in ClinicalTrials.gov.
Optimization of stem cell and stem cell derivative expansion is vital to clinical application. Moreover, various factors must be considered prior to the induction of experimental animal model techniques of AFSC administration, such as identifying: (1) the ideal gestational age to harvest AFSCs, (2) markers eliciting optimal cells, (3) an optimized standard for AFSC expansion (4) optimized dose concentrations of AFSC derivatives, (5) stem cell conditioning for enhanced applications, (6) proving that animal study results can be translated to a human setting without detrimental effects. In the following paragraphs, these factors are explained in more detail.
The general quality of AFSC cultures is significantly correlated with gestational age of the fetus when AF is harvested. In studies with experimental spina bifida patients, MSCs and neural SCs show to have been affected by gestational age (27). Recent studies with AFSCs show a similar trend, however gestational age is shown to affect paracrine signalling capabilities. For example, differential modulation of lymphocyte proliferation is most affected in the second trimester of pregnancy. Expression levels of HLA molecules and sensitivity to natural killer (NK) cell-mediated lysis are decreased, while T and NK cell proliferation is not efficiently inhibited but B cells are, which is not seen in first or third trimester cells (64). Immunomodulation, especially the suppression of inflammatory responses, is a meaningful attribute of AFSCs. Thus, the communication between lymphocytes and these cells must be optimized, and gestational age may allow for this to occur. While gestational age yields different effects, cell cultures stratified based on maternal age yielded no significant differences in the quality or repair capabilities (27).
On the quality of these cell cultures, AFSC morphology yield differential properties in protecting the neonate. There are two types of AFSCs: spindle-shaped and round-shaped. Those that are spindle-shaped possess greater neuroprotection to the developing fetal brain via analysis of CD90 and CD105. It is well studied that the consequences of NEC go on to affect neurodevelopment and brain morphology, therefore, these results support AFSC-mediated protection via the use of optimal cells (65). In addition, Oct-4 is a common biomarker of pluripotency capabilities and should be used when analyzing harvested hAFSC cultures. Optimizing the quality of cell cultures harvested via ideal gestational age and predictable cell markers will increase the confidence researchers have in the induction of AFSC cultures in clinical application. However, it remains unknown whether quality of these cell cultures is hindered when harvested from diseased sources. For example, harvesting AFSCs from a fetus with NEC. Baughn et al. studied whether the use of AFSCs harvested from patients with neural tube defects have differential quality or repair capabilities (27). Further research is needed to elucidate these implications, thus contributing to the lack of clinical AFSC induction.
As previously discussed, the use of CM and EVs are evidenced to be additional therapeutic tools. These EVs containing soluble bioactive factors and complex cargo have secretomes that differentially affect immune responses, including lymphocyte proliferation, and regeneration (64). EVs from different individuals contain various proteins that may not be present in their neighbour, thus elucidating minor differential effects. Therefore, results seen in many studies must consider how variance may play a significant factor in how clinical application is affected.
The culture medium in which cell expansion occurs has been optimized for in vitro use with animal models involving animal sera. The standardization of optimized 10%–15% fetal bovine serum (FBS) was studied in the clonal expansion of AFSC cultures, however, results yielded noncompliance with good manufacturing practice (GMP) (28, 66). Though AFSCs do not yield the same safety issues other stem cell lines do, it remains important to keep GMP-compliance when handling these cells to avoid all possibility of infectious disease transmission. For other stem cell lineages, xeno-free reagents have been used as animal sera substitute for clinical translation as xeno reagents induce immune rejection (66). However, platelet-derived products are now becoming the increasingly popular substitute. Platelets are rich in growth factors that modulate growth, repair and angiogenic capabilities. In addition, this type of media has the capacity to preserve cells involved in immunomodulation and the suppression of infectious disease transmission (28). Lyset, a commercial platelet-derived product, has been proven to be a safe alternative culture medium as it does not compromise the integrity and functionality of AFSCs in clinical translation (28).
In recent research, short-term small molecule treatments are a growing interest within the field of regenerative medicine. These molecules can improve stem cell characteristics to further reinforce their therapeutic potential and efficiency. A study assessed the effect of various concentrations and mixtures of small molecules known to participate in cell repair and regeneration on AFSCs, such as surface marker and pluripotency associated gene expression (67). HDAC inhibitors trichostatin A (TSA) and sodium butyrate (NaBut) promote somatic cell reprogramming, while multifunctional molecules retinoic acid (RA) and vitamin C (vitC) synergistically boost pluripotency. These small molecules in combination with each other have no cytotoxic effects but do increase gene expression patters of pluripotency markers and neurogenic transcription factors (Oct4, Nanog, Lin28a, Cmyc, Notch1, Sox2). Surface level markers are also affected (SSEA4, CD117, Tra-1-81, CD105) (67). Lastly, the effect on metabolic phenotype was significantly increased. Thus, it is plausible for the application of these small molecules as a pre-treatment strategy to maintain, even boost, AFSC functional efficiency.
To overcome these hurdles within this research field of AFSC-based therapy, key knowledge must further be elucidated prior to the implementation of randomized control trials in human neonates. Experimental NEC trials are currently completed in animal models, thus further investigation is required to investigate the effects of this therapy on human models, such as intestinal epithelial maturation, barrier function and innate immune responses. For example, working with human-derived organoids would be a great first step to preclinical human models (68).
Conclusion
To conclude, we have identified important bodies of knowledge that continue to lack within the scope of clinical translation of AFSC-based therapy. Future research must confirm whether this treatment strategy will benefit neonates suffering from NEC via preclinical trials. This review provides a beneficial frame of reference towards clinical application of AFSC and/or its derivatives as a novel treatment against NEC.
Author contributions
FB, YT, and BL: Substantial contributions to conception and design, Drafting or revising the article, Final approval of the version to be published. AP: Substantial contributions to conception and design. All authors contributed to the article and approved the submitted version.
Funding
BL is the recipient of a Restracomp Fellowship, The Hospital for Sick Children and Early Career Award Program grant from the Thrasher Research Fund (14503). AP is the recipient of a Canadian Institutes of Health Research (CIHR) Foundation Grant 353857.
Conflict of interest
The authors declare that the research was conducted in the absence of any commercial or financial relationships that could be construed as a potential conflict of interest.
Publisher's note
All claims expressed in this article are solely those of the authors and do not necessarily represent those of their affiliated organizations, or those of the publisher, the editors and the reviewers. Any product that may be evaluated in this article, or claim that may be made by its manufacturer, is not guaranteed or endorsed by the publisher.
References
1. Meister AL, Doheny KK, Travagli RA. Necrotizing enterocolitis: it's Not all in the gut. Exp Biol Med (Maywood). (2020) 245:85–95. doi: 10.1177/1535370219891971
2. Gupta A, Paria A. Etiology and medical management of NEC. Early Hum Dev. (2016) 97:17–23. doi: 10.1016/j.earlhumdev.2016.03.008
3. Alganabi M, Lee C, Bindi E, Li B, Pierro A. Recent advances in understanding necrotizing enterocolitis. F1000Res. (2019) 8:107. doi: 10.12688/f1000research.17228.1
4. Robinson JR, Rellinger EJ, Hatch LD, Weitkamp JH, Speck KE, Danko M, et al. Surgical necrotizing enterocolitis. Semin Perinatol. (2017) 41:70–9. doi: 10.1053/j.semperi.2016.09.020
5. Rees CM, Pierro A, Eaton S. Neurodevelopmental outcomes of neonates with medically and surgically treated necrotizing enterocolitis. Arch Dis Child Fetal Neonatal Ed. (2007) 92:F193–8. doi: 10.1136/adc.2006.099929
6. Neu J, Walker WA. Necrotizing enterocolitis. N Engl J Med. (2011) 364:255–64. doi: 10.1056/NEJMra1005408
7. O'Connor DL, Gibbins S, Kiss A, Bando N, Brennan-Donnan J, Ng E, et al. Effect of supplemental donor human milk compared with preterm formula on neurodevelopment of very low-birth-weight infants at 18 months: a randomized clinical trial. JAMA. (2016) 316:1897–905. doi: 10.1001/jama.2016.16144
8. Miyake H, Lee C, Chusilp S, Bhalla M, Li B, Pitino M, et al. Human breast milk exosomes attenuate intestinal damage. Pediatr Surg Int. (2020) 36:155–63. doi: 10.1007/s00383-019-04599-7
9. Pisano C, Galley J, Elbahrawy M, Wang Y, Farrell A, Brigstock D, et al. Human breast milk-derived extracellular vesicles in the protection against experimental necrotizing enterocolitis. J Pediatr Surg. (2020) 55:54–8. doi: 10.1016/j.jpedsurg.2019.09.052
10. Li B, Wu RY, Horne RG, Ahmed A, Lee D, Robinson SC, et al. Human milk oligosaccharides protect against necrotizing enterocolitis by activating intestinal cell differentiation. Mol Nutr Food Res. (2020) 64:e2000519. doi: 10.1002/mnfr.202000519
11. Johnson-Henry KC, Abrahamsson TR, Wu RY, Sherman PM. Probiotics, prebiotics, and synbiotics for the prevention of necrotizing enterocolitis. Adv Nutr. (2016) 7:928–37. doi: 10.3945/an.116.012237
12. Koike Y, Li B, Ganji N, Zhu H, Miyake H, Chen Y, et al. Remote ischemic conditioning counteracts the intestinal damage of necrotizing enterocolitis by improving intestinal microcirculation. Nat Commun. (2020) 11:4950. doi: 10.1038/s41467-020-18750-9
13. Kolios G, Moodley Y. Introduction to stem cells and regenerative medicine. Respiration. (2013) 85:3–10. doi: 10.1159/000345615
14. Dziadosz M, Basch RS, Young BK. Human amniotic fluid: a source of stem cells for possible therapeutic use. Am J Obstet Gynecol. (2016) 214:321–7. doi: 10.1016/j.ajog.2015.12.061
15. Sedrakyan S, Villani V, Da Sacco S, Tripuraneni N, Porta S, Achena A, et al. Amniotic fluid stem cell-derived vesicles protect from VEGF-induced endothelial damage. Sci Rep. (2017) 7:16875. doi: 10.1038/s41598-017-17061-2
16. Cassinotti A, Annaloro C, Ardizzone S, Onida F, Della Volpe A, Clerici M, et al. Autologous haematopoietic stem cell transplantation without CD34 + cell selection in refractory Crohn's Disease. Gut. (2008) 57:211–7. doi: 10.1136/gut.2007.128694
17. Murphy SV, Atala A. Amniotic fluid and placental membranes: unexpected sources of highly multipotent cells. Semin Reprod Med. (2013) 31:62–8. doi: 10.1055/s-0032-1331799
18. Dasgupta S, Jain SK. Protective effects of amniotic fluid in the setting of necrotizing enterocolitis. Pediatr Res. (2017) 82:584–95. doi: 10.1038/pr.2017.144
19. Leaphart CL, Cavallo J, Gribar SC, Cetin S, Li J, Branca MF, et al. A critical role for TLR4 in the pathogenesis of necrotizing enterocolitis by modulating intestinal injury and repair. J Immunol. (2007) 179:4808–20. doi: 10.4049/jimmunol.179.7.4808
20. Good M, Siggers RH, Sodhi CP, Afrazi A, Alkhudari F, Egan CE, et al. Amniotic fluid inhibits toll-like receptor 4 signaling in the fetal and neonatal intestinal epithelium. Proc Natl Acad Sci U S A. (2012) 109:11330–5. doi: 10.1073/pnas.1200856109
21. Tayman C, Uckan D, Kilic E, Ulus AT, Tonbul A, Murat Hirfanoglu I, et al. Mesenchymal stem cell therapy in necrotizing enterocolitis: a rat study. Pediatr Res. (2011) 70:489–94. doi: 10.1203/PDR.0b013e31822d7ef2
22. De Coppi P, Bartsch G Jr., Siddiqui MM, Xu T, Santos CC, Perin L, et al. Isolation of amniotic stem cell lines with potential for therapy. Nat Biotechnol. (2007) 25:100–6. doi: 10.1038/nbt1274
23. Prusa AR, Marton E, Rosner M, Bernaschek G, Hengstschlager M. Oct-4-expressing cells in human amniotic fluid: a new source for stem cell research? Hum Reprod. (2003) 18:1489–93. doi: 10.1093/humrep/deg279
24. Srivastava M, Ahlawat N, Srivastava A. Amniotic fluid stem cells: a new era in regenerative medicine. J Obstet Gynaecol India. (2018) 68:15–9. doi: 10.1007/s13224-017-1034-z
25. Zani A, Cananzi M, Fascetti-Leon F, Lauriti G, Smith VV, Bollini S, et al. Amniotic fluid stem cells improve survival and enhance repair of damaged intestine in necrotising enterocolitis via a COX-2 dependent mechanism. Gut. (2014) 63:300–9. doi: 10.1136/gutjnl-2012-303735
26. Volarevic V, Markovic BS, Gazdic M, Volarevic A, Jovicic N, Arsenijevic N, et al. Ethical and safety issues of stem cell-based therapy. Int J Med Sci. (2018) 15:36–45. doi: 10.7150/ijms.21666
27. Baughn C, Campion S, Elbabaa S. Amniotic fluid-derived stem cell potential for therapeutic and surgical use: a review of the literature. Prenat Diagn. (2022) 42:157–63. doi: 10.1002/pd.6087
28. Martinelli D, Pereira RC, Mogni M, Benelli R, Mastrogiacomo M, Coviello D, et al. A humanized system to expand in vitro amniotic fluid-derived stem cells intended for clinical application. Cytotherapy. (2016) 18:438–51. doi: 10.1016/j.jcyt.2015.11.020
29. McCulloh CJ, Olson JK, Wang Y, Vu J, Gartner S, Besner GE. Evaluating the efficacy of different types of stem cells in preserving gut barrier function in necrotizing enterocolitis. J Surg Res. (2017) 214:278–85. doi: 10.1016/j.jss.2017.03.026
30. McCulloh CJ, Olson JK, Zhou Y, Wang Y, Besner GE. Stem cells and necrotizing enterocolitis: a direct comparison of the efficacy of multiple types of stem cells. J Pediatr Surg. (2017) 52:999–1005. doi: 10.1016/j.jpedsurg.2017.03.028
31. Harrell CR, Gazdic M, Fellabaum C, Jovicic N, Djonov V, Arsenijevic N, et al. Therapeutic potential of amniotic fluid derived mesenchymal stem cells based on their differentiation capacity and immunomodulatory properties. Curr Stem Cell Res Ther. (2019) 14:327–36. doi: 10.2174/1574888X14666190222201749
32. Eaton S, Zani A, Pierro A, De Coppi P. Stem cells as a potential therapy for necrotizing enterocolitis. Expert Opin Biol Ther. (2013) 13:1683–9. doi: 10.1517/14712598.2013.849690
33. Wei J, Zhou Y, Besner GE. Heparin-binding EGF-like growth factor and enteric neural stem cell transplantation in the prevention of experimental necrotizing enterocolitis in mice. Pediatr Res. (2015) 78:29–37. doi: 10.1038/pr.2015.63
34. Li B, Lee C, Cadete M, O'Connell JS, Alganabi M, Lee D, et al. Amniotic fluid stem cell administration can prevent epithelial injury from necrotizing enterocolitis. Pediatr Res. (2022) 91:101–6. doi: 10.1038/s41390-021-01657-6
35. Okamoto R, Yajima T, Yamazaki M, Kanai T, Mukai M, Okamoto S, et al. Damaged epithelia regenerated by bone marrow-derived cells in the human gastrointestinal tract. Nat Med. (2002) 8:1011–7. doi: 10.1038/nm755
36. Tanaka F, Tominaga K, Ochi M, Tanigawa T, Watanabe T, Fujiwara Y, et al. Exogenous administration of mesenchymal stem cells ameliorates dextran sulfate sodium-induced colitis via anti-inflammatory action in damaged tissue in rats. Life Sci. (2008) 83:771–9. doi: 10.1016/j.lfs.2008.09.016
37. Bein A, Eventov-Friedman S, Arbell D, Schwartz B. Intestinal tight junctions are severely altered in NEC preterm neonates. Pediatr Neonatol. (2018) 59:464–73. doi: 10.1016/j.pedneo.2017.11.018
38. Blackwood BP, Wood DR, Yuan CY, Nicolas JD, Griffiths A, Mestan K, et al. Urinary claudin-2 measurements as a predictor of necrotizing enterocolitis: a pilot study. J Neonatal Surg. (2015) 4:43. doi: 10.47338/jns.v4.457
39. Liu J, Miyake H, Zhu H, Li B, Alganabi M, Lee C, et al. Fecal microbiota transplantation by enema reduces intestinal injury in experimental necrotizing enterocolitis. J Pediatr Surg. (2020) 55:1094–8. doi: 10.1016/j.jpedsurg.2020.02.035
40. Maghraby MK, Li B, Chi L, Ling C, Benmoussa A, Provost P, et al. Extracellular vesicles isolated from milk can improve gut barrier dysfunction induced by malnutrition. Sci Rep. (2021) 11:7635. doi: 10.1038/s41598-021-86920-w
41. Lau E, Lee C, Li B, Pierro A. Endoplasmic reticulum stress in the acute intestinal epithelial injury of necrotizing enterocolitis. Pediatr Surg Int. (2021) 37:1151–60. doi: 10.1007/s00383-021-04929-8
42. Rozpedek W, Pytel D, Mucha B, Leszczynska H, Diehl JA, Majsterek I. The role of the PERK/eIF2alpha/ATF4/CHOP signaling pathway in tumor progression during endoplasmic Reticulum stress. Curr Mol Med. (2016) 16:533–44. doi: 10.2174/1566524016666160523143937
43. Ma F, Hao H, Gao X, Cai Y, Zhou J, Liang P, et al. Melatonin ameliorates necrotizing enterocolitis by preventing Th17/treg imbalance through activation of the AMPK/SIRT1 pathway. Theranostics. (2020) 10:7730–46. doi: 10.7150/thno.45862
44. Li B, Lee C, O'Connell JS, Antounians L, Ganji N, Alganabi M, et al. Activation of wnt signaling by amniotic fluid stem cell-derived extracellular vesicles attenuates intestinal injury in experimental necrotizing enterocolitis. Cell Death Dis. (2020) 11:750. doi: 10.1038/s41419-020-02964-2
45. O'Connell JS, Li B, Zito A, Ahmed A, Cadete M, Ganji N, et al. Treatment of necrotizing enterocolitis by conditioned medium derived from human amniotic fluid stem cells. PloS One. (2021) 16:e0260522. doi: 10.1371/journal.pone.0260522
46. Leushacke M, Barker N. Lgr5 and Lgr6 as markers to study adult stem cell roles in self-renewal and cancer. Oncogene. (2012) 31:3009–22. doi: 10.1038/onc.2011.479
47. Hosfield B, Markel T, Li H, Shelley W, John Q. Lgr5 intestinal stem cells are more abundant with increasing age and exposure to breastmilk. Pediatrics. (2020) 146:664–5. doi: 10.1542/peds.146.1MA7.664
48. Gomart A, Vallee A, Lecarpentier Y. Necrotizing enterocolitis: lPS/TLR4-induced crosstalk between canonical TGF-beta/wnt/beta-catenin pathways and PPARgamma. Front Pediatr. (2021) 9:713344. doi: 10.3389/fped.2021.713344
49. Almonaem ERA, Almotaleb GSA, Alhameed MHA, El-Shimi OS. Utility of transforming growth factor beta-1 in diagnosis of neonatal necrotizing enterocolitis. J Neonatal Perinatal Med. (2022). doi: 10.3233/NPM-210973. [Epub ahead of print]35811541
50. Coletta R, Roberts NA, Randles MJ, Morabito A, Woolf AS. Exogenous transforming growth factor-beta1 enhances smooth muscle differentiation in embryonic mouse jejunal explants. J Tissue Eng Regen Med. (2018) 12:252–64. doi: 10.1002/term.2409
51. Liu XS, Lin XK, Mei Y, Ahmad S, Yan CX, Jin HL, et al. Regulatory T cells promote overexpression of Lgr5 on gastric cancer cells via TGF-beta1 and confer poor prognosis in gastric cancer. Front Immunol. (2019) 10:1741. doi: 10.3389/fimmu.2019.01741
52. Jun EK, Zhang Q, Yoon BS, Moon JH, Lee G, Park G, et al. Hypoxic conditioned medium from human amniotic fluid-derived mesenchymal stem cells accelerates skin wound healing through TGF-beta/SMAD2 and PI3K/akt pathways. Int J Mol Sci. (2014) 15:605–28. doi: 10.3390/ijms15010605
53. Chen L, Tredget EE, Wu PY, Wu Y. Paracrine factors of mesenchymal stem cells recruit macrophages and endothelial lineage cells and enhance wound healing. PLoS One. (2008) 3:e1886. doi: 10.1371/journal.pone.0001886
54. Bogatcheva NV, Coleman ME. Conditioned Medium of mesenchymal stromal cells: a new class of therapeutics. Biochemistry. (2019) 84:1375–89. doi: 10.1134/S0006297919110129
55. Thery C, Zitvogel L, Amigorena S. Exosomes: composition, biogenesis and function. Nat Rev Immunol. (2002) 2:569–79. doi: 10.1038/nri855
56. Simons M, Raposo G. Exosomes–vesicular carriers for intercellular communication. Curr Opin Cell Biol. (2009) 21:575–81. doi: 10.1016/j.ceb.2009.03.007
57. Ratajczak J, Miekus K, Kucia M, Zhang J, Reca R, Dvorak P, et al. Embryonic stem cell-derived microvesicles reprogram hematopoietic progenitors: evidence for horizontal transfer of mRNA and protein delivery. Leukemia. (2006) 20:847–56. doi: 10.1038/sj.leu.2404132
58. Baraniak PR, McDevitt TC. Stem cell paracrine actions and tissue regeneration. Regen Med. (2010) 5:121–43. doi: 10.2217/rme.09.74
59. van Niel G, D'Angelo G, Raposo G. Shedding light on the cell biology of extracellular vesicles. Nat Rev Mol Cell Biol. (2018) 19:213–28. doi: 10.1038/nrm.2017.125
60. Abels ER, Breakefield XO. Introduction to extracellular vesicles: biogenesis, RNA cargo selection, content, release, and uptake. Cell Mol Neurobiol. (2016) 36:301–12. doi: 10.1007/s10571-016-0366-z
61. Urabe F, Kosaka N, Ito K, Kimura T, Egawa S, Ochiya T. Extracellular vesicles as biomarkers and therapeutic targets for cancer. Am J Physiol Cell Physiol. (2020) 318:C29–39. doi: 10.1152/ajpcell.00280.2019
62. Matei AC, Antounians L, Zani A. Extracellular vesicles as a potential therapy for neonatal conditions: state of the art and challenges in clinical translation. Pharmaceutics. (2019) 11(8):404. doi: 10.3390/pharmaceutics11080404
63. O'Connell JS, Lee C, Farhat N, Antounians L, Zani A, Li B, et al. Administration of extracellular vesicles derived from human amniotic fluid stem cells: a new treatment for necrotizing enterocolitis. Pediatr Surg Int. (2021) 37:301–9. doi: 10.1007/s00383-020-04826-6
64. Beretti F, Zavatti M, Casciaro F, Comitini G, Franchi F, Barbieri V, et al. Amniotic fluid stem cell exosomes: therapeutic perspective. Biofactors. (2018) 44:158–67. doi: 10.1002/biof.1407
65. Biouss G, Antounians L, Li B, O'Connell JS, Seo S, Catania VD, et al. Experimental necrotizing enterocolitis induces neuroinflammation in the neonatal brain. J Neuroinflammation. (2019) 16:97. doi: 10.1186/s12974-019-1481-9
66. Wang J, Hao J, Bai D, Gu Q, Han W, Wang L, et al. Generation of clinical-grade human induced pluripotent stem cells in Xeno-free conditions. Stem Cell Res Ther. (2015) 6:223. doi: 10.1186/s13287-015-0206-y
67. Zentelyte A, Zukauskaite D, Jaceryte I, Borutinskaite VV, Navakauskiene R. Small molecule treatments improve differentiation potential of human amniotic fluid stem cells. Front Bioeng Biotechnol. (2021) 9:623886. doi: 10.3389/fbioe.2021.623886
Keywords: necrotizing enterocolitis (NEC), amniotic fluid stem cells (AFSCs), stem cell, translational research, preclinical study
Citation: Balsamo F, Tian Y, Pierro A and Li B (2022) Amniotic fluid stem cells: A novel treatment for necrotizing enterocolitis. Front. Pediatr. 10:1020986. doi: 10.3389/fped.2022.1020986
Received: 16 August 2022; Accepted: 17 November 2022;
Published: 1 December 2022.
Edited by:
Antonino Morabito, University of Florence, ItalyReviewed by:
Riccardo Coletta, University of Florence, ItalyMaria Grazia Scuderi, University of Catania, Italy
© 2022 Balsamo, Tian, Pierro and Li. This is an open-access article distributed under the terms of the Creative Commons Attribution License (CC BY). The use, distribution or reproduction in other forums is permitted, provided the original author(s) and the copyright owner(s) are credited and that the original publication in this journal is cited, in accordance with accepted academic practice. No use, distribution or reproduction is permitted which does not comply with these terms.
*Correspondence: Bo Li bo.li@sickkids.ca
Specialty Section: This article was submitted to Pediatric Surgery, a section of the journal Frontiers in Pediatrics