- 1Paediatric Intensive Care Unit, Queensland Children's Hospital, Brisbane, QLD, Australia
- 2Child Health Research Centre, The University of Queensland, Brisbane, QLD, Australia
- 3National Health and Medical Research Council (NHMRC) Clinical Trials Centre, University of Sydney, Sydney, NSW, Australia
- 4Queensland Paediatric Cardiac Service, Queensland Children's Hospital, Brisbane, QLD, Australia
- 5Department of Intensive Care Medicine and Neonatology, and Children's Research Center, University Children's Hospital of Zurich, University of Zurich, Zurich, Switzerland
Background: Infections represent one of the most common complications in patients managed on Extracorporeal Membrane Oxygenation (ECMO) and are associated with poorer outcomes. Clinical signs of infection in patients on ECMO are non-specific. We assessed the diagnostic accuracy of Procalcitonin (PCT), C-reactive protein (CRP) and White cell count (WCC) to diagnose infection on ECMO.
Methods: Retrospective single center observational study including neonates and children <18 years treated with ECMO in 2015 and 2016. Daily data on PCT, CRP and WCC were assessed in relation to microbiologically confirmed, and clinically suspected infection on ECMO using operating characteristics (ROC) curves.
Results: Sixty-five ECMO runs in 58 patients were assessed. CRP had the best accuracy with an area under the ROC curve (AUC) of 0.79 (95%-CI 0.66–0.92) to diagnose confirmed infection and an AUC of 0.72 (0.61–0.84) to diagnose confirmed and suspected infection. Abnormal WCC performed slightly worse with an AUC of 0.70 (0.59–0.81) for confirmed and AUC of 0.66 (0.57–0.75) for confirmed and suspected infections. PCT was non-discriminatory.
Conclusion: The diagnosis of infections acquired during ECMO remains challenging. Larger prospective studies are needed that also include novel infection markers to improve recognition of infection in patients on ECMO.
Highlights
- When comparing C-reactive protein (CRP), white cell count (WCC), and procalcitonin (PCT) to diagnose infection in 65 neonatal and pediatric ECMO runs, the performance of CRP was best.
- Combining infection markers did not lead to improved performance to diagnose sepsis, and PCT was non-discriminatory.
- This study can inform the design of prospective multicenter studies on novel infection markers and marker algorithms to improve recognition of infection in patients on ECMO.
Introduction
Extracorporeal Membrane Oxygenation (ECMO) has gained widespread acceptance as rescue treatment for refractory cardiovascular and respiratory failure in adults and children (1–4). The use of ECMO has extended to increasingly complex patient populations, such as patients with malignancies (5, 6) and patients with chronic medical comorbidities or those requiring extracorporeal cardiopulmonary resuscitation (ECPR) (7, 8). This complex patient group is subject to high risk of developing complications and more likely to be colonized with multi-drug resistant organisms (9).
Infections represent one of the most common complications in patients managed on ECMO (10). A recent review of the literature revealed an incidence of ECMO-associated infections between 10.1 and 116.2 per 1,000 ECMO days (11). Nosocomial infections acquired on ECMO have been described in up to 64% of adult and 30% of neonatal ECMO runs and are associated with increased ECMO duration, prolonged mechanical ventilation, prolonged hospital stay, and increased mortality; particularly in presence of invasive fungal infections (12).
Clinical signs of infection in patients on ECMO are non-specific and often masked, making a diagnosis of infection challenging (10). During ECMO, respiratory rate and temperature are usually artificially controlled, and ECMO perfusion, sedation, and cardiovascular drugs alter heart rate and blood pressure. In addition, ECMO-triggered systemic inflammation can mimic features of sepsis. A definite diagnosis of infection therefore relies on microbiological cultures which commonly take up to 48–72 h to analyse (13). Blood culture performance depends on bacterial load, and results can be falsely negative in patients exposed to prophylactic or therapeutic antibiotics (14). This diagnostic uncertainty may lead to unnecessary treatment with antibiotics and may promote antibiotic resistance (15). At the same time, missing a diagnosis of a nosocomial infection will delay initiation of effective antimicrobial treatment and potentially contribute to excess ECMO mortality and morbidity (16, 17).
Laboratory markers of infection have gained widespread acceptance to guide decisions to start and stop antibiotics in critical care (18). Extracorporeal circuits and shock-related acute phase responses lead to non-specific increases in infection markers, further confounding the diagnosis of infection in this population (19). In addition to the traditional infection markers White Cell Count (WCC) and C-reactive protein (CRP), a large randomized-controlled trial in adult general ICU patients demonstrated superiority of a Procalcitonin (PCT) guided approach (20). Similarly, PCT guided therapy in neonates with suspected early-onset sepsis allowed to reduce the duration of antimicrobial therapy (21, 22). While observational studies in critically ill children suggest superiority of PCR over CRP, adequately powered RCTs are lacking (23). In contrast, the available evidence supporting the use of infection markers on ECMO is very limited: Only four studies across 65 adult and 47 neonatal/pediatric patients reported on the accuracy of markers to diagnose infection in patients already on ECMO (19, 24–26).
We hypothesized that PCT would be superior to CRP and WCC to diagnose infection in neonates and children treated with ECMO. For this purpose, we assessed the diagnostic accuracy of daily measured PCT, CRP, and WCC to diagnose infection in 65 consecutive ECMO runs at our center.
Methods
We performed a retrospective, single center cohort study of all consecutive neonatal and pediatric ECMO runs at the Queensland Children's Hospital Pediatric Intensive Care Unit (PICU). Ethics approval was obtained by the institutional human research and ethics committee including waiver of consent (HREC/17/QRCH/8). This study was reported following the current Standards for Reporting Diagnostic accuracy studies (STARD) guideline (27).
We included ECMO cases in neonates, children and adolescents aged <18 years with initiation of ECMO between 01/01/2015 and 31/12/2016. All types of runs were included [venovenous (VV), and venoarterial (VA), extracorporeal cardiopulmonary resuscitation (ECPR), cardiac, respiratory]. Two authors independently reviewed the institutional patient data management system (Metavision, iMDsoft, USA) of all patients treated with ECMO during the study period to ascertain which patients had a microbiologically proven infection, suspected infection or no infection on each ECMO day.
The ECMO service provides centralized ECMO support for the state of Queensland and northern New South Wales including ECPR, cardiac, and respiratory ECMO for all neonatal and pediatric age groups. The default ECMO setup included centrifugal pumps, a shunt containing a filter for slow continuous ultrafiltration, in- and outlet pressure monitors, and continuous measurement of venous and arterial saturations, hemoglobin, and ECMO flow. Steroids are not routinely used. Antimicrobial prophyaxis using cephazolin is used for the first 24 h after cannulation, and in children with open chest for the duration of the chest remaining open.
Definitions
Microbiologically confirmed infection was defined as per Center for Diseases Control guideline definitions, characterized by positive bacterial or fungal growth of microbiological samples obtained in blood, urine, or endotracheal aspirate (28), and treated with intravenous antibiotics for at least 5 days or until death. Clinically suspected infection was defined as suspicion of infection documented in clinical notes in presence of negative cultures and treated with intravenous antibiotics for at least 5 days or until death.
We searched the electronic health records, radiology results and laboratory data for information on the focus of infection, to categorize them into Bloodstream Infection (BSI); Central Line Associated Blood Stream Infection (CLABSI); urinary tract infection (UTI); and Ventilator Associated Pneumonia (VAP). VAP was defined as clinical and radiological signs consistent with acute respiratory infection in presence of likely pathogenic microbiological organisms in sputum (10). Positive endotracheal cultures in the absence of clinical or radiological signs were considered as colonization. Patients with confirmed viral infections and patients who received prophylactic antibiotics in the absence of suspected or confirmed infections were considered non-infected. In episodes of confirmed infection, we defined day 0 as the day when a culture was obtained that later confirmed infection, while in episodes of suspected infection it was the day when intravenous antibiotics were started. We considered ECMO days from 48 h prior to day 0 until the end of antibiotic treatment as infected days (confirmed or suspected). Days outside this time frame, and days in ECMO runs where no infection was suspected or confirmed, were considered not infected.
During the study period, institutional practice as per the local ECMO guideline recommended obtaining daily surveillance cultures, PCT, CRP, and WCC. For the purpose of this study, daily laboratory and microbiology data (highest daily PCT, CRP, WCC; as well as microbiological cultures from blood, urine and endotracheal aspirate) were reviewed from the institutional ICU database from the day prior to ECMO to day of decannulation or death. Changes in CRP and PCT over 48 h were calculated to assess whether changes in biomarkers were more accurate in detecting infection than absolute values. Patient and ECMO characteristics including age, severity on presentation, surgical procedures performed, circuit changes, renal replacement therapy, inotrope use, daily maximum ECMO flow rates, PICU and hospital length of stay, and mortality, were extracted from the prospective institutional ECMO and ICU databases.
Statistical Analysis
We divided the ECMO runs into three groups according to the occurrence of infections: (1) patients with no infection during the whole ECMO run, (2) runs with a suspected infection, (3) runs with a confirmed infection. If a patient had both confirmed and suspected infection episodes during a run, this particular run was grouped under confirmed infection. Median and interquartile ranges (IQR) were used to describe continuous data. Given the small sample size and uncertain normality of data, non-parametric tests were used for group comparisons (Kruskal-Wallis test and Spearman Rank correlation for continuous data, Fisher's Exact test for proportions).
We used the biomarker measurements obtained on day 0 of each episode to estimate their accuracy in predicting a bacterial or fungal infection. If markers were not obtained at the same time as blood cultures, we used the highest marker value available within no more than 24 h of blood culture sampling. Given that both very low and very high WCC can be indicative of an infection, we applied age-dependent categorization of WCC based on the 2005 International Pediatric Sepsis Definition Consensus Conference (29).
We used receiver operating characteristics (ROC) analyses to compare the accuracy of markers by calculating the area under the ROC curve (AUC). As repeated measurements within ECMO runs may be correlated, the estimation of 95% confidence bands around ROC curves was adjusted for clustered data. For PCT and CPR, we determined the test cut-off values resulting in 80 and 90% sensitivity, respectively, which may serve as minimal thresholds for clinical practice. For completeness, we also used Youden's Index to find marker cut-offs yielding maximum combined sensitivity and specificity (30, 31). For each test cut-off, we calculated sensitivity, specificity, positive likelihood ratio (LR+), negative likelihood ratio (LR-), positive predictive value (PPV) and negative predictive value (NPV).
We further tested whether combining the two best performing markers would increase the diagnostic accuracy. In exploratory analyses, we tested for associations between infection markers and ECMO parameters, including ECMO type, patient age (dichotomized into neonates vs. older children), day of ECMO, peak daily lactate, and peak daily flow rate. We built multi-level regression models to assess the association of ECMO parameters with infection markers, adjusting for repeated measurements within ECMO runs.
All analyses used pairwise deletion, and no imputation of missing values was performed. A p-value < 0.05 was considered significant. Open source software R (32) (in particular pROC and clusteredROC packages), and SAS version 9.4 were used for analyses.
Results
Characteristics of Study Cohort
During the study period, 65 ECMO runs with an average run duration of 7.2 days (median 5 days, IQR 2–9) were performed in 58 patients. The cohort included 32 (49%) neonatal, 33 (51%) pediatric ECMO runs of which 12 (18%) were ECPR, 22 (34%) cardiac, and 31 (48%) respiratory, respectively (Table 1). Of these, 31 (48%) runs were with no infection, 13 (20%) with suspected infection, and 21 (32%) with confirmed infection (Table 1). In 20 of the 65 runs the patients died, resulting in a mortality of 31%.
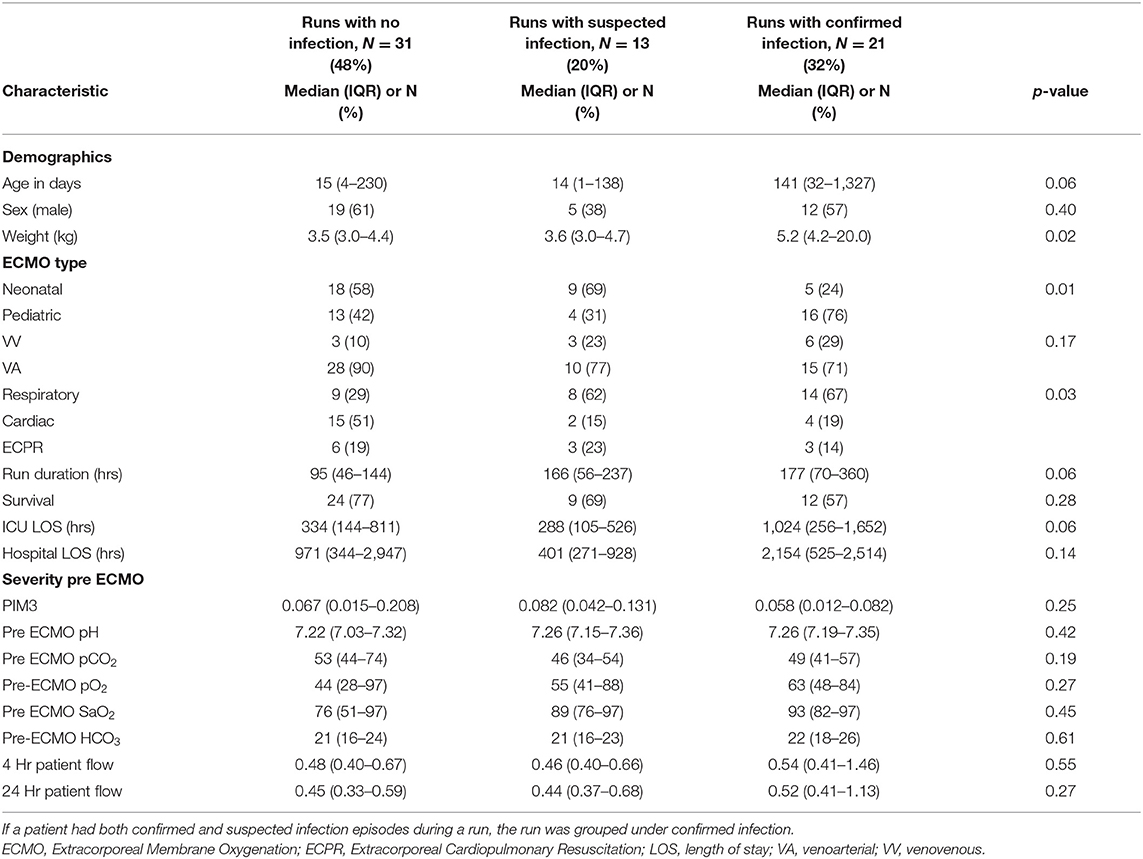
Table 1. Baseline characteristic of patients, grouped into Extracorporeal Membrane Oxygenation (ECMO) runs with no infection, with suspected infection, and with confirmed infection.
Out of 590 ECMO days, antibiotics were administered on 512 days (87%), including 155 days for prophylaxis, 122 days for suspected and 182 days for confirmed infection, and 53 days to treat a necrotizing enterocolitis. Surveillance blood cultures were taken on 492 run days (86%) out of 575 days where this information was available, with 17 (3.5%) blood cultures resulting positive. The most commonly identified organisms were Candida spp. and Stenotrophomonas spp., followed by Enterobacter spp., Coagulase-negative staphylococci (CoNS), and Klebsiella spp. The most common focus of infection was VAP, followed by UTI (Supplementary Table A). PCT was available for 252 days (43%), with a median of 5.8 mg/L (IQR 1.6–14.5 mg/L); CRP was available for 404 ECMO days (68%), median 63 mg/L (IQR 24–131 mg/L); and WCC was available for 557 ECMO days (94%), median 12.2*109/L (IQR 8.6–17.6*109/L).
Diagnostic Accuracy of Infection Markers
The diagnostic accuracy to identify confirmed infection was best for CRP with an AUC of 0.79 (95% CI 0.66–0.92), and it was worst for PCT with an AUC of 0.57 (95% CI 0.35–0.80, Table 2, Figures 1A,B) and. The accuracy of WCC was intermediate (AUC 0.70, 95% CI 0.59–0.81, Supplementary Figure 1). A change in PCT over 48 h was not significantly associated with an infection (AUC 0.68, 95% CI 0.49–0.86), neither was an increase in CRP over 48 h (AUC 0.65, 95% CI 0.47–0.84).
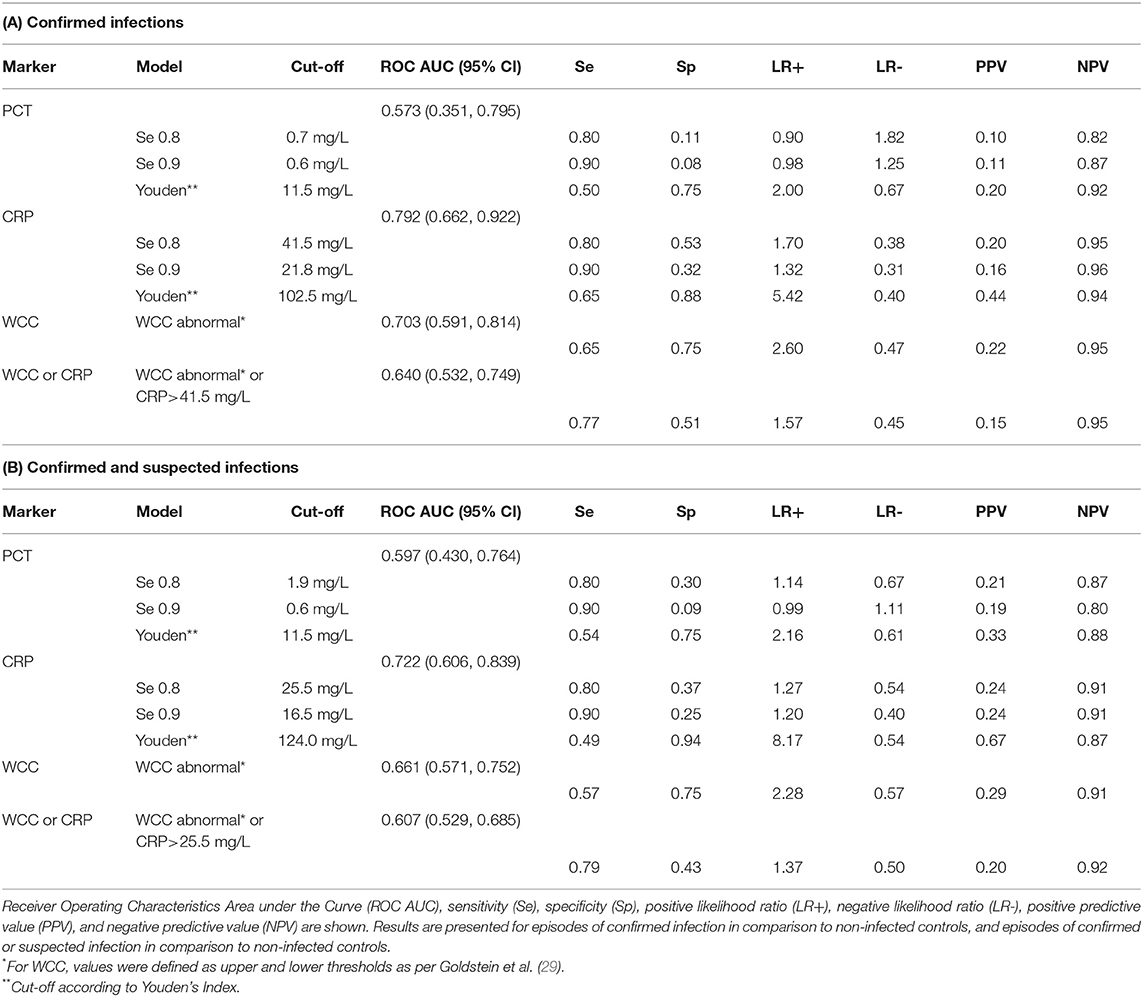
Table 2. Diagnostic accuracy of Procalcitonin (PCT), C-reactive protein (CRP), and White Cell Count (WCC) to diagnose infection on ECMO.
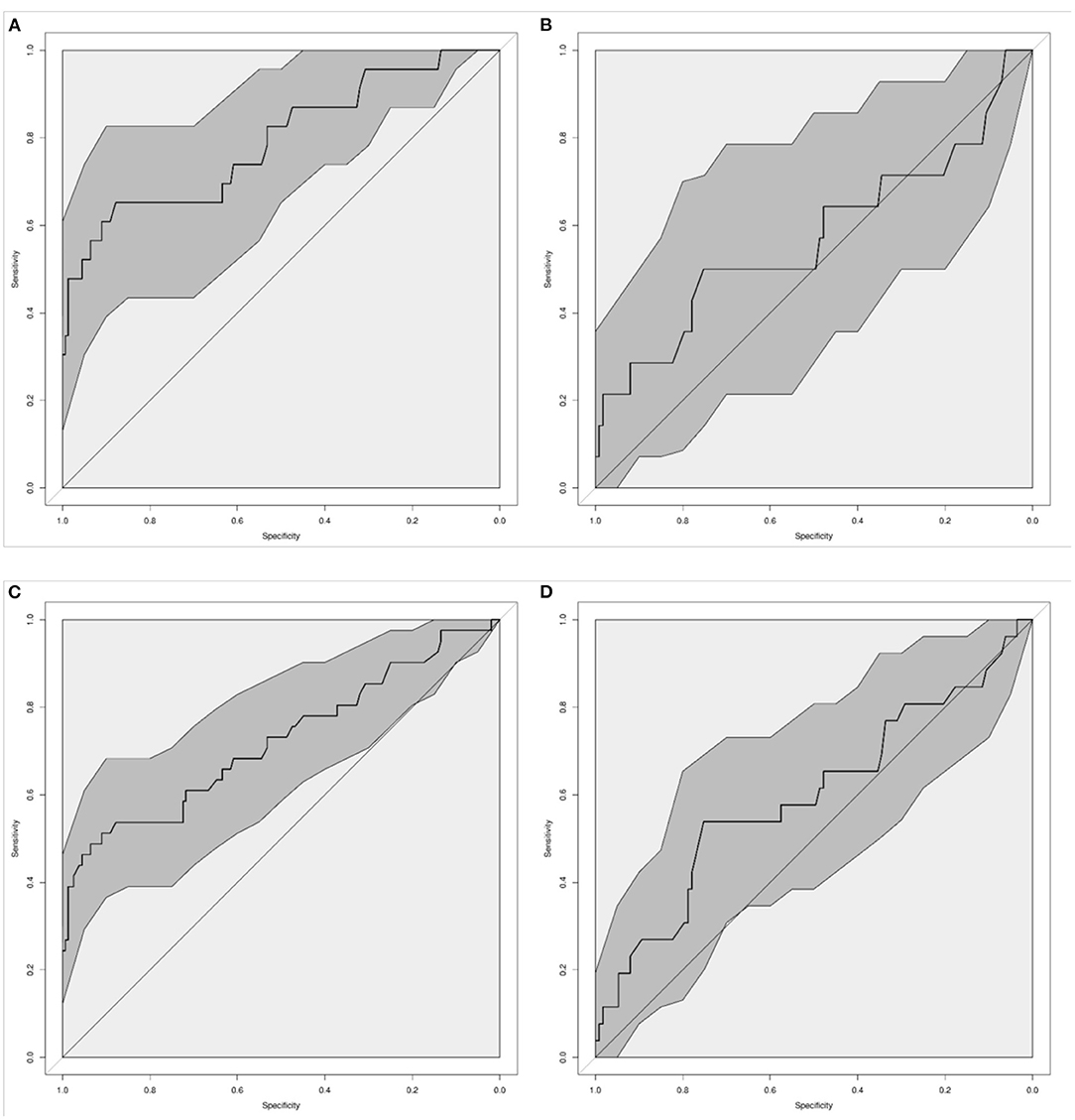
Figure 1. Diagnostic accuracy of C-Reactive Protein [CRP; (A,C)] and Procalcitonin [PCT; (B,D)] to diagnose infection in neonates and children on ECMO. Graphs show the Receiver-Operating Characteristic Area Under the Curve (AUC) for confirmed infection (A,B), and for confirmed and suspected infection (C,D). Dark gray shaded areas indicate 95%-Confidence bands.
We then calculated cut-offs where the markers reached a sensitivity of 80 and 90%, and the cut-offs according to Youden's Index (Table 2 and Supplementary Figure 2). For CRP, cut-offs of 41.5 mg/L and 21.8 mg/L were found to diagnose a confirmed infection with 80 and 90% sensitivity, which were accompanied by specificities of 53 and 32%. Applying Youden's Index resulted in a cut-off close to 100 mg/L, which was associated with a sensitivity of 65% and a specificity of 88%. The sensitivity of abnormal WCC to diagnose proven infection was 65%, with a specificity of 75%. Combining abnormal WCC with CRP ≥41.5 mg/L resulted in worse performance than CRP alone (AUC 0.64, 95% CI 0.53–0.75).
When assessing the diagnostic accuracy to identify confirmed and suspected infection, CRP again performed best with an AUC of 0.72 (95% CI 0.61–0.84) (Figures 1C,D). A CRP of ≥25.5 mg/L diagnosed proven or suspected infection with a sensitivity of 80%, with a specificity of 37%. The sensitivity of abnormal WCC to diagnose proven or suspected infection was 57%, with a specificity of 75%. The diagnostic accuracy decreased when combining WCC and CRP (Table 2).
Exploratory Analyses on Biomarker Associations With ECMO Run Characteristics
Using regression models, we then explored whether ECMO run characteristics were associated with biomarker levels (Supplementary Table B and Supplementary Figure 3). ECMO type and patient age were not associated with PCT, CRP or WCC. Both PCT and WCC decreased over time, with a weak but significant negative slope in the regression analysis. WCC was positively associated with peak daily lactate, while PCT and CRP increased with higher peak daily flow rates.
Discussion
Infections are one of the most common complications for patients on ECMO and contribute to higher morbidity and mortality (12). While the benefit of rapid identification and treatment of sepsis has been broadly recognized in critically ill patients (16, 33), accurate and timely diagnosis of infections on ECMO poses a major challenge, and the evidence to guide best practice in this area remains scarce. Due to profound activation of inflammatory pathways on patients requiring ECMO and aggravated by the extracorporeal circuit itself, it is unclear to what extent widely used infection (or inflammation) markers provide clinically meaningful diagnostic accuracy in this patient group (10). We assessed the diagnostic accuracy of PCT, CRP and WCC to diagnose bacterial infections in 65 neonatal and pediatric ECMO runs. While CRP performed best, its accuracy was only moderate with an AUC below 0.8. PCT did not discriminate reliably in our cohort. Neither changes in CRP or PCT, nor combinations of biomarkers improved the performance of the diagnostic approach.
Our findings contrast with self-reported practice in a recent survey of 147 intensivists from 91 ECMO centers across 25 countries, which identified that rising CRP or PCT were the most commonly used criteria to diagnose infection (34). Our study demonstrates that patients are exposed to antibiotics on almost 90 percent of ECMO days, indicating an urgent need for improved markers or infection prediction models. Previous studies in patients on ECMO showed that WCC, absolute neutrophil count, immature to total white cell ratio, platelet count, lactate and fibrinogen were not predictive of bloodstream infections (35–38). In adult general ICU patients with sepsis and septic shock, PCT has been shown to improve the diagnosis of bacterial infections compared to traditional infection markers such as WCC or CRP, allowing to enhance antimicrobial stewardship by reducing duration of antibiotic therapy with potential mortality benefit (20, 22). A recent literature review identified 59 full text articles reporting on infections on ECMO, of which only four investigated the role of infection markers (11). These previous studies on infection markers on ECMO were very small, including <30 runs, and yielded inconclusive results (19, 24, 25, 39): Rungatscher et al. reported that neither CRP or PCT were predictive of infection in 20 children on ECMO. In contrast, a recent report on 27 neonatal and pediatric ECMO runs where the proportion of positive cultures was very high (19% BSI) observed a sensitivity and specificity of a PCT threshold of 0.5 ng/ml to diagnose infection of 92%, and 43%, respectively. In two studies on 27 and 41 adult patients, a PCT cut-off of 2 ng/ml was reported as optimal to discriminate presence of infection vs. inflammation on ECMO, however was only predictive of infection on VA ECMO but not on VV ECMO. All studies were retrospective single center studies applying different definitions of infection, they reported on one or two markers rather than comparing CRP, PCT, and WCC, and none adjusted for repeated measurements.
Our findings are concordant with a number of studies showing that WBC have poor to moderate performance to discriminate infection in children (30, 37, 40, 41). In addition, it is possible that our approach to dichotomizing WBC (in order to capture both abnormal high, as well as abnormal low WBC) may have affected results. Of note, the diagnostic accuracy to diagnose infections on ECMO decreased when CRP and WBC were combined—which may be explained by overlaying areas of poor specificity with poor sensitivity. In addition, a multitude of non-infectious factors on ECMO may affect both high and low WBC counts, such as stress, steroids, massive transfusion, and chylus loss.
In our study, PCT was elevated in more than 75% of measurements to levels above the threshold commonly applied to diagnose infection in non-ECMO patients, and was unable to discriminate between confirmed or suspected infection and no infection. However, PCR values were available only for 43% of ECMO days, potentially biasing the findings. Nonspecific PCT increase has been observed in a range of settings such as ischemia, reperfusion injury or following cardiopulmonary bypass (24, 25, 42). This likely reflects the inflammatory response to the ECMO circuit immediately following cannulation and may be further aggravated by acute organ dysfunction, shock, and reperfusion injury. Exposure to non-epithelialized extracorporeal surfaces rapidly leads to systemic inflammation as a result of profound activation of cellular and humoral components of the innate immune system (43). We therefore hypothesize that the observed trend for PCT levels to decrease over time during ECMO runs may reflect the progression of the initial inflammatory response to ECMO. While infections are more commonly acquired after the first week on ECMO, our observations suggest that different infection marker thresholds may need to be considered depending on time spent on ECMO. However, our study was not powered to define infection marker thresholds specific for each week on ECMO, and the observed inter-patient variability was very high.
This study has several limitations. Due to the retrospective design, the definition of infection relied on a combination of microbiological findings, medical notes, and the initiation of antibiotic therapy for at least 5 days or until death. As clinical decision making was not blinded to infection markers, the reference standard was not totally independent of the index tests (incorporation bias), as requested by STARD (27). Moreover, the rate of confirmed BSI was low and the majority of infection episodes were classified as VAP. This may have impaired the quality of the reference standard, as differentiation of colonization and infection is challenging in pulmonary cultures. Aligned with CDC recommendations, positive endotracheal cultures in the absence of clinical or radiological signs were considered as colonization, however we cannot rule out that some of these findings resulted ultimatively in a true infection. We did not have access to colony forming unit counts. The application of VAP definitions to ECMO patients harbors further challenges as non-specific alterations on chest X-ray are common in patients with ultra long-protective ventilation on ECMO (10). Given the limitations of relying on microbiologically confirmed infections, additional analyses were performed on confirmed and suspected infections. In terms of index test measurements, while the institutional protocol during the study included CRP, PCT, and WCC in addition to blood cultures to be performed regularly, there was no protocol in place to define timing on the commencement or cessation of antibiotics at the institution, nor to define suspected infection prospectively. While WCC and CRP were performed almost daily, we identified fewer PCT values, which reduced the power of the study to analyze associations with PCT. Finally, we did not investigate immature to total ratio.
Our findings indicate that current infection markers are neither sensitive nor specific enough to reliably diagnose infection in neonates and children on ECMO. Difficulty in diagnosis combined with altered pharmacokinetics of medications on ECMO makes early effective treatment challenging. New biomarkers, such as host gene expression-based signatures, have been suggested to improve the guidance on antibiotic treatment decisions (44, 45). These markers have great potential to improve ECMO outcomes by facilitating earlier diagnosis of infection. Future research should develop and validate prediction models that estimate the risk of having a bacterial or fungal infection, based on all the available information including clinical signs and new infection markers. Implementation studies of these prediction models should then test whether the use of the models helps reduce unnecessary antibiotic exposure, resulting in improved antimicrobial stewardship at non-inferior safety.
In conclusion, while CRP performed best to diagnose infection for children on ECMO, its diagnostic accuracy was only moderate. Increases in WCC, and combinations of CRP and WCC or PCT, although commonly used in practice, did not result in improved diagnostic performance. Larger prospective studies are needed to test novel markers of infection to improve recognition of infection in patients on ECMO.
Data Availability Statement
The datasets presented in this article are not readily available because requests for additional analyses on the data need clearance from the institutional ethics board. Requests to access the datasets should be directed to the corresponding author.
Ethics Statement
The studies involving human participants were reviewed and approved by HREC/17/QRCH/8. Written informed consent to participate in this study was provided by the participants' legal guardian/next of kin.
Author Contributions
LJS designed the study, supervised analyses, interpreted the data, and wrote the first draft and the final manuscript. ID contributed to study design, collected all the data, performed initial analyses, and wrote with LJS the first draft. LPS and AS performed statistical analyses and contributed to manuscript writing. AM, EH, and NA were involved in study design, data interpretation, and manuscript writing. All authors read and approved the final manuscript.
Funding
LJS was supported by a Practitioner Fellowship of the National Health and Medical Research Council of Australia and New Zealand, and by the Children‘s Hospital Foundation, Brisbane, Australia. This study was performed at the Queensland Children's Hospital, Brisbane, Australia.
Conflict of Interest
The authors declare that the research was conducted in the absence of any commercial or financial relationships that could be construed as a potential conflict of interest.
Publisher's Note
All claims expressed in this article are solely those of the authors and do not necessarily represent those of their affiliated organizations, or those of the publisher, the editors and the reviewers. Any product that may be evaluated in this article, or claim that may be made by its manufacturer, is not guaranteed or endorsed by the publisher.
Supplementary Material
The Supplementary Material for this article can be found online at: https://www.frontiersin.org/articles/10.3389/fped.2021.824552/full#supplementary-material
Abbreviations
ARDS, acute respiratory distress syndrome; BSI, blood stream infection; CLABSI, central line associated blood stream infection; ECMO, extracorporeal membrane oxygenation; ECPR, extracorporeal cardiopulmonary resuscitation; SIRS, systemic inflammatory response syndrome; VA ECMO, venoarterial ECMO; VAP, ventilator associated pneumonia; VV ECMO, venovenous ECMO.
References
1. Sivarajan VB, Almodovar MC, Rodefeld MD, Laussen PC. Pediatric extracorporeal life support in specialized situations. Pediatr Crit Care Med. (2013) 14:S51–61. doi: 10.1097/PCC.0b013e318292e16e
2. Ramanathan K, Yeo N, Alexander P, Raman L, Barbaro R, Tan CS, et al. Role of extracorporeal membrane oxygenation in children with sepsis: a systematic review and meta-analysis. Crit Care. (2020) 24:684. doi: 10.1186/s13054-020-03418-z
3. Schlapbach LJ, Chiletti R, Straney L, Festa M, Alexander D, Butt W, et al. Defining benefit threshold for extracorporeal membrane oxygenation in children with sepsis-a binational multicenter cohort study. Crit Care. (2019) 23:429. doi: 10.1186/s13054-019-2685-1
4. Domico M, Ridout D, MacLaren G, Barbaro R, Annich G, Schlapbach LJ, et al. Extracorporeal membrane oxygenation for pertussis: predictors of outcome including pulmonary hypertension and leukodepletion. Pediatr Crit Care Med. (2018) 19:254–61. doi: 10.1097/PCC.0000000000001454
5. Smith S, Butt W, Best D, MacLaren G. Long-term survival after extracorporeal life support in children with neutropenic sepsis. Intensive Care Med. (2016) 42:942–3. doi: 10.1007/s00134-015-4163-9
6. Gow KW, Heiss KF, Wulkan ML, Katzenstein HM, Rosenberg ES, Heard ML, et al. Extracorporeal life support for support of children with malignancy and respiratory or cardiac failure: the extracorporeal life support experience. Crit Care Med. (2009) 37:1308–16. doi: 10.1097/CCM.0b013e31819cf01a
7. Sivarajan VB, Best D, Brizard CP, Shekerdemian LS, d'Udekem Y, Butt W. Duration of resuscitation prior to rescue extracorporeal membrane oxygenation impacts outcome in children with heart disease. Intensive Care Med. (2011) 37:853–60. doi: 10.1007/s00134-011-2168-6
8. Brodie D, Bacchetta M. Extracorporeal membrane oxygenation for ARDS in adults. N Engl J Med. (2011) 365:1905–14. doi: 10.1056/NEJMct1103720
9. Vodovar D, Marcade G, Rousseau H, Raskine L, Vicaut E, Deye N, et al. Predictive factors for extended-spectrum beta-lactamase producing Enterobacteriaceae causing infection among intensive care unit patients with prior colonization. Infection. (2014) 42:743–8. doi: 10.1007/s15010-014-0619-z
10. Abrams D, Grasselli G, Schmidt M, Mueller T, Brodie D. ECLS-associated infections in adults: what we know and what we don't yet know. Intensive Care Med. (2020) 46:182–91. doi: 10.1007/s00134-019-05847-z
11. MacLaren G, Schlapbach LJ, Aiken AM. nosocomial infections during extracorporeal membrane oxygenation in neonatal, pediatric, and adult patients: a comprehensive narrative review. Pediatr Crit Care Med. (2019) 21:283–90. doi: 10.1097/PCC.0000000000002190
12. Bizzarro MJ, Conrad SA, Kaufman DA, Rycus P Extracorporeal Extracorporeal Life Support Organization Task Force on Infections Extracorporeal Membrane Oxygenation. Infections acquired during extracorporeal membrane oxygenation in neonates, children, and adults. Pediatr Crit Care Med. (2011) 12:277–81. doi: 10.1097/PCC.0b013e3181e28894
13. Dierig A, Berger C, Agyeman PKA, Bernhard-Stirnemann S, Giannoni E, Stocker M, et al. Swiss pediatric sepsis, time-to-positivity of blood cultures in children with sepsis. Front Pediatr. (2018) 6:222. doi: 10.3389/fped.2018.00222
14. Buttery JP. Blood cultures in newborns and children: optimising an everyday test. Arch Dis Child Fetal Neonatal Ed. (2002) 87:F25–8. doi: 10.1136/fn.87.1.F25
15. Shallcross LJ, Davies DS. Antibiotic overuse: a key driver of antimicrobial resistance. Br J Gen Pract. (2014) 64:604–5. doi: 10.3399/bjgp14X682561
16. Kumar A, Roberts D, Wood KE, Light B, Parrillo JE, Sharma S, et al. Duration of hypotension before initiation of effective antimicrobial therapy is the critical determinant of survival in human septic shock. Crit Care Med. (2006) 34:1589–96. doi: 10.1097/01.CCM.0000217961.75225.E9
17. Weiss SL, Fitzgerald JC, Balamuth F, Alpern ER, Lavelle J, Chilutti M, et al. Delayed antimicrobial therapy increases mortality and organ dysfunction duration in pediatric sepsis. Crit Care Med. (2014) 42:2409–17. doi: 10.1097/CCM.0000000000000509
18. van Rossum AM, Wulkan RW, Oudesluys-Murphy AM. Procalcitonin as an early marker of infection in neonates and children. Lancet Infect Dis. (2004) 4:620–30. doi: 10.1016/S1473-3099(04)01146-6
19. Rungatscher A, Merlini A, De Rita F, Lucchese G, Barozzi L, Faggian G, et al. Diagnosis of infection in paediatric veno-arterial cardiac extracorporeal membrane oxygenation: role of procalcitonin and C-reactive protein. Eur J Cardio Thorac Surg. (2013) 43:1043–9. doi: 10.1093/ejcts/ezs524
20. de Jong E, van Oers JA, Beishuizen A, Vos P, Vermeijden WJ, Haas LE, et al. Efficacy and safety of procalcitonin guidance in reducing the duration of antibiotic treatment in critically ill patients: a randomised, controlled, open-label trial. Lancet Infect Dis. (2016) 16:819–27. doi: 10.1016/S1473-3099(16)00053-0
21. Geraerds AJLM, Van Herk W, Stocker M, El Helou S, Dutta S, Fontana MS, et al. Cost impact of procalcitonin-guided decision making on duration of antibiotic therapy for suspected early-onset sepsis in neonates. Crit Care. (2021) 25:367. doi: 10.1186/s13054-021-03789-x
22. Stocker M, van Herk W, El Helou S, Dutta S, Fontana MS, Schuerman F, et al. Procalcitonin-guided decision making for duration of antibiotic therapy in neonates with suspected early-onset sepsis: a multicentre, randomised controlled trial (NeoPIns). Lancet. (2017) 390:871–81. doi: 10.1016/S0140-6736(17)31444-7
23. Menon K, Schlapbach LJ, Akech S, Argent A, Biban P, Carrol ED, et al. Criteria for pediatric sepsis-A systematic review and meta-analysis by the pediatric sepsis definition taskforce. Crit Care Med. (2021) 50:21–36. doi: 10.1097/CCM.0000000000005294
24. Tanaka D, Pitcher HT, Cavarocchi NC, Diehl JT, Hirose H. Can procalcitonin differentiate infection from systemic inflammatory reaction in patients on extracorporeal membrane oxygenation? J Heart Lung Transplant. (2014) 33:1186–8. doi: 10.1016/j.healun.2014.08.015
25. Pieri M, Greco T, De Bonis M, Maj G, Fumagalli L, Zangrillo A, et al. Diagnosis of infection in patients undergoing extracorporeal membrane oxygenation: a case-control study. J Thorac Cardiovasc Surg. (2012) 143:1411–6. doi: 10.1016/j.jtcvs.2012.01.005
26. Kim DW, Cho HJ, Kim GS, Song SY, Na KJ, Oh SG, et al. Predictive value of procalcitonin for infection and survival in adult cardiogenic shock patients treated with extracorporeal membrane oxygenation. Chonnam Med J. (2018) 54:48–54. doi: 10.4068/cmj.2018.54.1.48
27. Bossuyt PM, Reitsma JB, Bruns DE, Gatsonis CA, Glasziou PP, Irwig L, et al. STARD 2015: an updated list of essential items for reporting diagnostic accuracy studies. BMJ. (2015) 351:h5527. doi: 10.1136/bmj.h5527
28. Horan TC, Andrus M, Dudeck MA. CDC/NHSN surveillance definition of health care-associated infection and criteria for specific types of infections in the acute care setting. Am J Infect Control. (2008) 36:309–32. doi: 10.1016/j.ajic.2008.03.002
29. Goldstein B, Giroir B, Randolph A International International Consensus Conference on Pediatric Sepsis. International pediatric sepsis consensus conference: definitions for sepsis and organ dysfunction in pediatrics. Pediatr Crit Care Med. (2005) 6:2–8. doi: 10.1097/01.PCC.0000149131.72248.E6
30. Schlapbach LJ, Graf R, Woerner A, Fontana M, Zimmermann-Baer U, Glauser D, et al. Pancreatic stone protein as a novel marker for neonatal sepsis. Intensive Care Med. (2013) 39:754–63. doi: 10.1007/s00134-012-2798-3
31. Gibot S, Bene MC, Noel R, Massin F, Guy J, Cravoisy A, et al. Combination biomarkers to diagnose sepsis in the critically ill patient. Am J Respir Crit Care Med. (2012) 186:65–71. doi: 10.1164/rccm.201201-0037OC
32. R Core Team. R: A Language and Environment for Statistical Computing R Foundation for Statistical Computing. Vienna (2019).
33. Evans IVR, Phillips GS, Alpern ER, Angus DC, Friedrich ME, Kissoon N, et al. Association between the new york sepsis care mandate and in-hospital mortality for pediatric sepsis. JAMA. (2018) 320:358–67. doi: 10.1001/jama.2018.9071
34. Farrell D, MacLaren G, Schlapbach LJ. Infections on extracorporeal life support in adults and children - a survey of international practice on prevention, diagnosis, and treatment. Pediatr Crit Care Med. (2019) 20:667–71. doi: 10.1097/PCC.0000000000001941
35. Kaczala GW, Paulus SC, Al-Dajani N, Jang W, Blondel-Hill E, Dobson S, et al. Bloodstream infections in pediatric ECLS: usefulness of daily blood culture monitoring and predictive value of biological markers. The British Columbia experience. Pediatr Surg Int. (2009) 25:169–73. doi: 10.1007/s00383-008-2299-1
36. Steiner CK, Stewart DL, Bond SJ, Hornung CA, McKay VJ. Predictors of acquiring a nosocomial bloodstream infection on extracorporeal membrane oxygenation. J Pediatr Surg. (2001) 36:487–92. doi: 10.1053/jpsu.2001.21609
37. Cruz AT, Mahajan P, Bonsu BK, Bennett JE, Levine DA, Alpern ER, et al. Accuracy of complete blood cell counts to identify febrile infants 60 days or younger with invasive bacterial infections. JAMA Pediatr. (2017) 171:e172927. doi: 10.1001/jamapediatrics.2017.2927
38. Mischler M, Orzulak FM, Hanks J. White blood cell count in the evaluation of the febrile infant: time to revisit the dogma? JAMA Pediatr. (2017) 171:e172796. doi: 10.1001/jamapediatrics.2017.2796
39. Tan VE, Moore WS, Chopra A, Cies JJ. Association of procalcitonin values and bacterial infections in pediatric patients receiving extracorporeal membrane oxygenation. Perfusion. (2018) 33:278–82. doi: 10.1177/0267659117743806
40. Thompson M, Van den Bruel A, Verbakel J, Lakhanpaul M, Haj-Hassan T, Stevens R, et al. Systematic review and validation of prediction rules for identifying children with serious infections in emergency departments and urgent-access primary care. Health Technol Assess. (2012) 16:1–100. doi: 10.3310/hta16150
41. Stocker M, van Herk W, El Helou S, Dutta S, Schuerman F, van den Tooren-de Groot RK, et al. C-reactive protein, procalcitonin, and white blood count to rule out neonatal early-onset sepsis within 36 hours: a secondary analysis of the neonatal procalcitonin intervention study. Clin Infect Dis. (2020) 73:e383–90. doi: 10.1093/cid/ciaa876
42. Zant R, Stocker C, Schlapbach LJ, Mayfield S, Karl T, Schibler A. Procalcitonin in the early course post pediatric cardiac surgery. Pediatr Crit Care Med. (2016) 17:624–9. doi: 10.1097/PCC.0000000000000751
43. Millar JE, Fanning JP, McDonald CI, McAuley DF, Fraser JF. The inflammatory response to extracorporeal membrane oxygenation (ECMO): a review of the pathophysiology. Crit Care. (2016) 20:387. doi: 10.1186/s13054-016-1570-4
44. Zimmerman JJ, Sullivan E, Yager TD, Cheng C, Permut L, Cermelli S, et al. Diagnostic accuracy of a host gene expression signature that discriminates clinical severe sepsis syndrome and infection-negative systemic inflammation among critically ill children. Crit Care Med. (2017) 45:e418–25. doi: 10.1097/CCM.0000000000002100
Keywords: C-reactive protein, extracorporeal membrane oxygenation, extracorporeal life support, infection, pediatric, procalcitonin, white cell count, diagnostic accuracy
Citation: Doo I, Staub LP, Mattke A, Haisz E, Seidler AL, Alphonso N and Schlapbach LJ (2022) Diagnostic Accuracy of Infection Markers to Diagnose Infections in Neonates and Children Receiving Extracorporeal Membrane Oxygenation. Front. Pediatr. 9:824552. doi: 10.3389/fped.2021.824552
Received: 29 November 2021; Accepted: 20 December 2021;
Published: 26 January 2022.
Edited by:
Joris Lemson, Radboud University Nijmegen, NetherlandsReviewed by:
Matteo Di Nardo, Bambino Gesù Children's Hospital (IRCCS), ItalyPeter Paul Roeleveld, Leiden University Medical Center, Netherlands
Copyright © 2022 Doo, Staub, Mattke, Haisz, Seidler, Alphonso and Schlapbach. This is an open-access article distributed under the terms of the Creative Commons Attribution License (CC BY). The use, distribution or reproduction in other forums is permitted, provided the original author(s) and the copyright owner(s) are credited and that the original publication in this journal is cited, in accordance with accepted academic practice. No use, distribution or reproduction is permitted which does not comply with these terms.
*Correspondence: Luregn J. Schlapbach, luregn.schlapbach@kispi.uzh.ch