- 1Cansearch Research Platform for Pediatric Oncology and Hematology, Department of Pediatrics, Gynecology and Obstetrics, Faculty of Medicine, University of Geneva, Geneva, Switzerland
- 2Blood Transplant and Cell Therapies, Children's Hospital at Westmead, Sydney, NSW, Australia
- 3Department of Pediatric Hematology and Oncology, Comenius University, Bratislava, Slovakia
- 4Bone Marrow Transplantation Unit, National Institute of Children's Diseases, Bratislava, Slovakia
- 5Princess Maxima Center for Pediatric Oncology, Utrecht, Netherlands
- 6Division of Pediatric Oncology and Hematology, Department of Women, Child and Adolescent, University Geneva Hospitals, Geneva, Switzerland
- 7Speciality of Child and Adolescent Health, Faculty of Medicine and Health, The University of Sydney, Sydney, NSW, Australia
Total-body irradiation (TBI) based conditioning prior to allogeneic hematopoietic stem cell transplantation (HSCT) is generally regarded as the gold-standard for children >4 years of age with acute lymphoblastic leukaemia (ALL). Retrospective studies in the 1990's suggested better survival with irradiation, confirmed in a small randomised, prospective study in the early 2000's. Most recently, this was reconfirmed by the early results of the large, randomised, international, phase III FORUM study published in 2020. But we know survivors will suffer a multitude of long-term sequelae after TBI, including second malignancies, neurocognitive, endocrine and cardiometabolic effects. The drive to avoid TBI directs us to continue optimising irradiation-free, myeloablative conditioning. In chemotherapy-based conditioning, the dominant myeloablative effect is provided by the alkylating agents, most commonly busulfan or treosulfan. Busulfan with cyclophosphamide is a long-established alternative to TBI-based conditioning in ALL patients. Substituting fludarabine for cyclophosphamide reduces toxicity, but may not be as effective, prompting the addition of a third agent, such as thiotepa, melphalan, and now clofarabine. For busulfan, it's wide pharmacokinetic (PK) variability and narrow therapeutic window is well-known, with widespread use of therapeutic drug monitoring (TDM) to individualise dosing and control the cumulative busulfan exposure. The development of first-dose selection algorithms has helped achieve early, accurate busulfan levels within the targeted therapeutic window. In the future, predictive genetic variants, associated with differing busulfan exposures and toxicities, could be employed to further tailor individualised busulfan-based conditioning for ALL patients. Treosulfan-based conditioning leads to comparable outcomes to busulfan-based conditioning in paediatric ALL, without the need for TDM to date. Future PK evaluation and modelling may optimise therapy and improve outcome. More recently, the addition of clofarabine to busulfan/fludarabine has shown encouraging results when compared to TBI-based regimens. The combination shows activity in ALL as well as AML and deserves further evaluation. Like busulfan, optimization of chemotherapy conditioning may be enhanced by understanding not just the PK of clofarabine, fludarabine, treosulfan and other agents, but also the pharmacodynamics and pharmacogenetics, ideally in the context of a single disease such as ALL.
The Evolution of HSCT Conditioning for Paediatric ALL
Total body irradiation (TBI)-based conditioning prior to allogeneic haemopoietic stem cell transplantation (HSCT) is generally regarded as the gold standard for children ≥4 years of age with acute lymphoblastic leukaemia (ALL). TBI is a powerful anti-leukaemic modality that eradicates leukaemia in sanctuary sites and reduces the risk of relapse post-transplant (1, 2). Unfortunately, survivors suffer a multitude of long-term sequelae after TBI including second malignancies and neurocognitive, endocrine and cardiometabolic effects (3). TBI also requires access to irradiation facilities and sedation or anaesthetic in young children. The drive to avoid TBI has inspired an international effort to develop irradiation-free myeloablative conditioning regimens that provide equivalent disease-free survival (DFS) to TBI without the associated toxicity for children requiring HSCT for ALL. This review outlines the evolution of TBI-based conditioning for paediatric ALL, the development of chemotherapy-based conditioning (chemo-conditioning) alternatives that culminated in the For Omitting Radiation Under Majority age (FORUM) trial, and the latest published myeloablative chemo-conditioning protocols for ALL.
The Early Days of Chemo-Conditioning to Replace TBI
TBI conditioning prior to HSCT was pioneered by Thomas et al. in Seattle in 1970 (4). They added high-dose cyclophosphamide (120 mg/kg given over 2 days) to TBI in an effort to increase cytoreduction pre transplant and reduce relapse risk post-transplant. In a seminal report, they described the first 100 adult and paediatric patients with relapsed acute leukaemia who were transplanted in 1971–1975 following TBI-based conditioning (5). The combination of TBI and Cyclophosphamide was well-tolerated and was associated with long-term remission in 13% of patients, which was sustained in 8% (6). These results suggested that TBI-based conditioning for HSCT offered a survival advantage over chemotherapy in patients with end-stage disease, which prompted this approach to be trialled in the late 1970's in adult and paediatric patients with less-advanced leukaemia (7).
In the 1980's, attempts began to develop effective conditioning regimens that did not contain TBI, led by the John Hopkins group in Baltimore (8). They added the alkylating agent Busulfan to Cyclophosphamide to create the first chemo-conditioning regimen to be trialled. The addition of Busulfan aimed to provide equivalent myeloablation and leukaemia-free survival to TBI conditioning but with reduced toxicity. Chemo-conditioning with Busulfan 16 mg/kg and Cyclophosphamide 200 mg/kg or 120 mg/kg were used; both regimens induced long-term remission but the lower toxicity associated with Busulfan and Cyclophosphamide 120 mg/kg came at the cost of potentially increased relapse risk (9, 10). In paediatric HSCT, Busulfan and Cyclophosphamide 200 mg/kg is generally well-tolerated and so continues to be preferred over Busulfan and Cyclophosphamide 120 mg/kg as a conditioning regimen.
Early Trials Comparing TBI With Busulfan Plus Cyclophosphamide Predominantly in Adults
In the early 1990's, the first four prospective, randomised controlled trials comparing TBI-based conditioning and chemo-conditioning were published by groups in France (11, 12), Scandinavia (13), and Seattle (14). The studies involved predominantly adult patients, although a small number of children were included. The most common indication for HSCT was myeloid disease [acute myeloid leukaemia [AML] or chronic myeloid leukaemia (CML)]; a minority of patients in the Scandinavian trial had ALL or lymphoma (13). In all four trials, patients received Cyclophosphamide 120 mg/kg. Those randomised to the chemo-conditioning received Busulfan 16 mg/kg. In the TBI arms, regimens varied with most receiving 12 Gy in fractionated doses. When first published, at a relatively short follow-up of 24–42 months, DFS was superior in patients that received TBI-based conditioning vs. chemo-conditioning for AML in CR1 in the French multicentre study (72 vs. 47%, p < 0.01) (11) and for adults with advanced myeloid or lymphoid disease in the Scandinavian randomised controlled trial (68 vs. 54%, p = 0.05) (13). In contrast, chemo-conditioning with Busulfan-Cyclophosphamide achieved equivalent DFS to TBI-based conditioning in patients with CML in results published by the Seattle (14) and French group (12). A subsequent meta-analysis of these studies, and an additional randomised controlled trial comparing conditioning with Busulfan-Cyclophosphamide against that with TBI and etoposide, confirmed a non-statistically significant trend toward better overall survival (OS) and DFS with TBI-based conditioning (15).
This trend favouring TBI over chemo-conditioning, particularly in AML, was supported by the publication of the long-term data of the four trials. At a median follow-up of 10.8 years, Blaise et al. continued to show that TBI-Cyclophosphamide was associated with statistically significant higher DFS and OS and decreased relapse rates and transplant-related mortality compared with conditioning with Busulfan-Cyclophosphamide in patients with AML (TBI-Cyclophosphamide: 10-year OS 59%, DFS 55%; Busulfan-Cyclophosphamide: 10-year OS 43%, DFS 35%) (16). In the update of the Scandinavian study at 7 years of follow-up, OS was also higher in the TBI group (63% with TBI-Cyclophosphamide vs. 54% with Busulfan-Cyclophosphamide group) but this difference was not statistically significant (17). Similarly, when Socie et al. combined the data from the original four trials, a non-statistically significant 10% lower OS was observed in patients with AML who received conditioning with Busulfan-Cyclophosphamide compared with in those who received TBI-Cyclophosphamide [projected 10-year survival: 51% for Busulfan-Cyclophosphamide vs. 63% for TBI-Cyclophosphamide, 95% confidence interval (CI) 52–74%]. No statistically significant difference in OS or DFS was observed among patients with CML, as in the original studies (18).
Studies Comparing TBI With Busulfan Plus Cyclophosphamide in Children
In 2000, Davies et al. published a large study conducted in paediatric patients comparing TBI-based and chemo-conditioning regimens. This retrospective International Bone Marrow Transplant Registry (IBMTR) analysis included children with ALL who received a matched sibling HSCT after TBI/Cyclophosphamide or oral Busulfan-Cyclophosphamide. The incidence of relapse was similar between arms, suggesting that chemo-conditioning with Busulfan may not be inferior to TBI in preventing relapse. However, the higher non-relapse mortality (NRM) in the Busulfan arm led to TBI-based conditioning being associated with a superior leukaemia-free survival over Busulfan-based conditioning (50 vs. 35%, respectively; p = 0.005) (19).
The IBMTR study was shortly followed by publication of the first randomised controlled trial in paediatric patients comparing TBI-based and chemo-conditioning regimens: the Paediatric Blood and Marrow Transplant Consortium (PBMTC) study (20). This small study compared outcomes with chemo-conditioning with Busulfan, etoposide, Cyclophosphamide and anti-thymocyte globulin to those with TBI-based conditioning including Cyclophosphamide, etoposide +/- anti-thymocyte globulin. Relapse rates were similar between groups, yet NRM rates were higher in the Busulfan-Cyclophosphamide group. Bunin et al. concluded that “significant concerns regarding late effects, particularly secondary cancers, continue to make conditioning without radiation a potential attractive option, but additional studies are required to develop a safe, effective regimen.”
Despite these data, many centres replaced TBI-based protocols with Busulfan-based conditioning, particularly for myeloid diseases. However, over the ensuing decade, TBI retained its central role in conditioning for ALL. This was reinforced by evidence within in the literature. For example, a study looking at patients with ALL in CR2 concluded that TBI followed by HSCT compared to chemotherapy alone reduced the rate of relapse for children with early first relapse (21).
At the same time, there was continued recognition of the long-term burden following TBI, including an increased risk of breast cancer (22) and thyroid cancer (23). Moreover, the association between an increased risk of second solid cancers and age at the time of TBI was reported (24).
An important point is that in all the above studies, the Busulfan preparation used was oral, not intravenous (IV). The highly variable absorption rate and bioavailability of Busulfan, adding to its variable clearance, led to the development of the IV Busulfan formulation (25, 26). IV Busulfan enables better control of the cumulative exposure to Busulfan through therapeutic drug monitoring (TDM) (27, 28). In a retrospective trial that included paediatric ALL patients, Bartelink et al. reported an improved event-free survival (EFS) (83 vs. 30%, respectively; p < 0.001) and OS (83 vs. 53%, respectively; p = 0.016) accompanied with a decrease risk of veno-occlusive disease (VOD) under TDM-guided IV Busulfan compared with fixed-dose oral Busulfan (27). Although most centres have moved to the IV route, oral administration of Busulfan in paediatric HSCT is still used. Of note, a retrospective registry-based study on 460 transplanted children with leukaemia showed similar outcomes for both IV and oral formulations of Busulfan, but it was suggested that this was likely due to the routine use of Busulfan TDM (29).
The FORUM Trial of TBI vs. Chemo-Conditioning
With recognition of the life-long consequences of irradiation in young children, a convergence of shared thoughts and ideas led to the creation of the protocol that became the FORUM international, randomised controlled trial (Clinicaltrials.gov identifier: NCT02670564). The rationale included the following points:
1. Some patients relapse after TBI-based conditioning.
2. The use of oral Busulfan was being replaced by IV Busulfan, supporting more consistent bioavailability, more predictable pharmacokinetics (PK) and lower incidence of acute toxicity.
3. Recognition of the importance of measurable residual disease (MRD), particularly at the time of HSCT, for identifying patients with a poorer prognosis even with TBI-based HSCT (30, 31).
4. The use of haploidentical donors for second or third, and more recently first, HSCT was increasing; these transplants had often used less-aggressive conditioning than first or second remission transplants using matched related or unrelated donors. Despite the less intensive conditioning, the good overall results suggested that the greater immune reactivity of the mismatched donor might favour a graft-versus-leukaemia effect (32, 33).
5. A non-significant trend in favour of disease control by TBI in early follow-up might be offset in later follow-up by benefits of chemo-conditioning in terms of hard endpoints such as rates of secondary malignancies and other multiple benefits, such as a reduced risk of cataracts as well as fewer growth, neurocognition and dental effects.
The FORUM trial compared TBI (12 Gy) plus etoposide vs. chemo-conditioning with Fludarabine and Thiotepa combined with either Busulfan or Treosulfan (by country preference) in paediatric patients with ALL in CR who were between the ages of 4 and 21 years at HSCT. Twenty-one countries were involved in this large, prospective, Phase III study. The original intention was to recruit 1,000 patients over 5 years; however, the trial was stopped in March 2019 after 417 patients had been randomised due to early results indicating superiority of the TBI arm. The early results of FORUM were published in 2021 and confirmed that TBI conditioning was superior to chemo-conditioning, with a 16% higher 2-year OS (91 vs. 75%, respectively; p < 0.0001) and reduced cumulative risk of relapse (12 vs. 33%, respectively; p < 0.0001). Treatment-related mortality (TRM) was similar between the groups (34).
With FORUM showing a clear early benefit favouring TBI, we have to rethink how conditioning therapy in childhood ALL might otherwise be improved. Options include:
• Optimising the use of Busulfan-based conditioning with PK and genomics
• Optimising the use of Treosulfan
• Optimising the whole conditioning regimen
• Introducing newer agents, such as clofarabine (Clo), into conditioning regimens and establishing how we can introduce a new combination into frontline HSCT therapy.
We now explore each of these themes in turn.
Optimising the Use of Busulfan-Based Conditioning With Pharmacokinetics and Genomics
Definition and Refinement of the Optimal Busulfan Target Exposure
Busulfan with TDM is recommended in paediatric HSCT for several reasons. Firstly, Busulfan has a demonstrated exposure-response relationships and narrow therapeutic window, so small variations in exposure can result in poor clinical outcomes. Secondly, despite the improved predictability of PK obtained using IV formulations, due to the bypass of the unpredictable absorption phase, the inter-individual and intra-individual PK variability in Busulfan elimination and exposure remain substantial. The American Society for Blood and Marrow Transplantation recommends TDM-based dose adjustments for paediatric patients receiving myeloablative Busulfan-based conditioning therapy (35).
The association between Busulfan exposure and outcomes in paediatric patients with varying malignant diagnoses, including ALL, has been reported in many studies (Table 1) (36–55). The therapeutic window for Busulfan recommended by the European Medicines Agency (EMA) is AUC6h 900–1,500 μM.min (daily AUC of 14.8–24.6 mg.h/L) (56, 57). This target was originally derived from studies in adult HSCT patients using oral Busulfan. Exposure higher than 1,500 μM.min has been associated with increased toxicities such as sinusoidal obstruction syndrome (SOS) and acute graft-versus-host disease (GvHD) (47, 58, 59), while exposures lower than 900 μM.min were associated with increased graft rejection and disease relapse (52, 60). This therapeutic window has been confirmed to be safe and efficacious in various studies of paediatric patients, including those with ALL (52, 61, 62). Nguyen et al. developed a dosing nomogram designed to reach this therapeutic target in paediatric patients, which the EMA has since recommended (57). One retrospective study in 138 patients, including 13 paediatric patients with ALL, investigated the impact of narrowing the EMA-recommended typical Busulfan therapeutic window to a local target AUC6h 980–1,250 μM.min (daily AUC 16.1–20.5 mg.h/L). The efficacy (EFS and OS) and safety (SOS) outcomes evaluated in this study cohort were not improved using a narrower therapeutic window, suggesting that the EMA therapeutic window of 900–1,500 μM.min (daily AUC of 14.8–24.6 mg.h/L) is the most appropriate for children (53).
Another target for Busulfan dosing is based on steady-state concentration (Css). Css values can be expressed as AUC values by multiplying the Css value by the inter-dose interval. The reported optimal Css window of Busulfan is 600–900 ng/mL, corresponding to a daily AUC of 14.4 −21.6 mg.h/L (43, 44), which is only slightly lower than another narrowed therapeutic window recommended by the US Food and Drug Administration (FDA) (daily AUC 14.8–22.2 mg.h/L) (63). A recent meta-analysis by Feng et al. showed that the typical lower cutoff of 900 μM.min (daily AUC 14.8 mg.h/L) was strongly associated with the risk of graft failure (AUC ≥900 μM.min vs. <900 μM.min: Relative risk (RR) 3.666; CI 1.419–9.467), while the FDA cutoff (1,350 μM.min; daily AUC 22.2 mg.h/L) was more strongly associated with the risk of SOS than the EMA target (AUC ≤ 1,350 μM.min vs. >1,350 μM.min: RR 0.370; CI 0.205–0.666) (64). This study suggested that the FDA upper AUC cutoff (1,350 μM.min, daily AUC 22.2 mg.h/L) is safer in paediatric patients in terms of protection from SOS.
Much of the discussion about the Busulfan exposure metric has been superseded with the international harmonisation process to adopt uniform units of mg.L.h (65), as used in the largest retrospective study to date on the association between Busulfan exposure and outcomes in paediatric patients (36). Of the 674 patients enrolled in that study by Bartelink and colleagues, 41% were diagnosed with malignancies but only 5% had ALL (36). Based on EFS as the main criteria, the researchers found the optimal therapeutic window to be 78–101 mg.h/L, corresponding to a daily AUC of 19.5–25.3 mg.h/L. This target was shown to be optimal regardless of patients' malignant diagnoses. This new therapeutic target is included within the EMA target, with a slightly higher upper range (25.3 vs. 24.6 mg.h/L, respectively). However, it is higher than the FDA target, which was reported to be associated with a decreased SOS risk (64). This therapeutic window proposed by Bartelink et al. was also associated with acceptable acute toxicity (defined as acute GvHD and SOS) and occurrence of chronic GvHD. In response to a letter to the editor by Paci et al. (66), Bartelink et al. demonstrated that EFS was significantly reduced when targeting the lower end of the EMA threshold (AUC 59–78 mg.h/L) (41). The different studies show that there is still no consensus on the optimal cumulative exposure to Busulfan for paediatric patients due to heterogeneous data. Future well-designed, prospective investigations should further establish the optimal target window of Busulfan. However, it is widely agreed that TDM-guided dose adjustment of Busulfan is required to reach the desired target exposure in the paediatric HSCT setting, especially in neonates and small children for whom Busulfan PK is more unpredictable (67).
Studies have also shown that HSCT outcomes are not only associated with cumulative exposure to Busulfan but also with per-dose exposure. The AUC or Css of the first dose of Busulfan has been reported to be associated with toxicities of Busulfan as well as transplant outcomes. As shown in Table 1, a study from Ansari et al. reported that a first-dose Css <600 ng/mL (AUC6h <3.6 mg.h/L) was associated with improved OS and EFS, a lower NRM and a lower incidence of relapse and acute GvHD of grade II to IV compared to patients with Css > 600 ng/mL (38). The other toxicities reported (SOS, lung toxicities, and haemorrhagic cystitis) showed trends of lower incidence in patients receiving Busulfan with a first-dose Css <600 ng/mL (AUC6h <3.6 mg.h/L) compared to patients with Css > 600 ng/mL. A similar association between this exposure cut off and better NRM, OS, and EFS was later demonstrated in a larger multicentre population (39). In the latter study, the association between exposure and treatment-related toxicity (TRT) risk, comprising acute GvHD of grade I–IV, was shown to depend on glutathione S-transferase A1 (GSTA1) metabolic capacity (39).
Another study reported the association between SOS with the per-dose PK parameters of Busulfan in 293 patients including 125 with haematological malignancies (50). In the univariate analysis based on logistic regression, the maximal concentration after Busulfan infusion ended, and the first-dose AUC, but not the cumulative AUC, were associated with the occurrence of SOS. In the same study, engraftment only significantly associated with cumulative AUC. Interestingly, a study by Kerl et al. reported an increased risk of SOS with AUC6h >1,500 μM.min (daily AUC >24.6 mg.h/L) in patients receiving Busulfan four times daily but not in patients receiving once daily Busulfan (48). These studies provide evidence that per-dose exposure to Busulfan could impact the outcomes and incidence of toxicity in paediatric patients. Accurately targeted first doses of Busulfan before TDM is performed should enable clinicians to avoid the toxicities and poor outcomes related to higher per-dose exposure. A planned future analysis of Busulfan PK data from the FORUM trial will enable better understanding of the association between Busulfan exposure and outcomes in a homogenous cohort of paediatric ALL patients. A similar analysis will be performed of Busulfan exposure in AML patients in the ongoing Myechild01 trial (Clinicaltrials.gov identifier: NCT02724163). The target Busulfan exposure in FORUM and TDM adjustment settings were not harmonised; rather, they depended on the local clinical practise in each transplantation centre (34). The upcoming analysis of the FORUM PK data will enable the researchers to explore a potentially heterogeneous Busulfan exposure among patients and its relationship to patient outcomes. This heterogeneity in patient exposure could partly explain the inferiority of Busulfan-based regimens to TBI, and the analysis of the Busulfan PK data from FORUM will explore this.
Busulfan Administration Schedule
In HSCT, Busulfan was originally administered during 4 days of conditioning, four times daily (every 6 h). A once daily oral or IV Busulfan schedule has been reported to be safe and efficacious in paediatric patients (27, 68–72). One study in paediatric patients receiving IV Busulfan compared SOS risk between once-daily and four-times-daily dosing, finding a similar risk with each schedule (48). However, an association between exposure and SOS was only observed in patients receiving Busulfan four times a day, probably due to the presence of other risk factors. More recently, Philippe et al. showed that the risk of SOS was associated with the maximum concentration (Cmax) of Busulfan. While the cumulative AUC should be equivalent between once-daily and four-times-daily dosing, the Cmax obtained with once-daily dosing is systematically higher than that obtained with four-times-daily dosing.
The study by Philippe et al. included 11 patients who received once-daily or twice-daily Busulfan, among which nine (81.8%) patients experienced SOS (50). In contrast, other studies in paediatric patients have observed a lower occurrence of SOS in paediatric patients who received once-daily IV Busulfan dosing (69, 70). Further studies should address the comparison between once-daily and four-times-daily IV Busulfan dosing in paediatric patients, in terms of efficacy and toxicity outcomes.
The once-daily Busulfan dosing schedule has many advantages. Xhaard et al. showed that once-daily Busulfan dosing was associated with better patient comfort related to reduced nausea and vomiting and less infusions (73). Once-daily dosing was perceived by healthcare professionals to be safer and less error prone, in addition to reducing workload and allowing smoother treatment management. In addition, once-daily Busulfan dosing reduces transplantation-related costs (74). Dividing the total Busulfan dose over 16 doses (four times a day schedule) provides more opportunity for dose adjustments, which may make it easier to target the desired cumulative exposure. Four times daily regimen have enabled to adjust the dose of Bu from the third dose onwards during the 1st day of Bu (depending on access to a biomedical analysis laboratory), which is not feasible with once daily dosing. However, TDM-guided dose adjustment from the 2nd day of Busulfan infusion is feasible with once-daily dosing and allows cumulative exposure to be readily estimated (75). The less commonly used twice daily Bu schedule (every 12 h administration, eight doses) allows dose adjustments from the 2nd day of Bu treatment, whilst reducing the workload associated with the four times daily dosing.
Getting the First Dose of Busulfan Right: First Dose Personalization
When the use of TDM accounts for the interindividual PK variability of Busulfan, so allowing you to target the desired cumulative AUC, why is it important to individualise the first dose? Relying solely on TDM for dose adjustment has some limitations as well as having time-constrained limits on how quickly and how often dose adjustments can be made. Studies have highlighted the per-dose therapeutic window of Busulfan and the necessity to target early in administration the desired therapeutic window (39, 50, 66, 76). The personalization of the first dose of Busulfan should minimise the risk of overexposure and any associated acute toxicity. In combination with efficient TDM, this strategy could enable control of cumulative Busulfan exposure throughout conditioning treatment, which may optimise the outcomes. Because engraftment is associated with cumulative underexposure to Busulfan (50), first dose under-exposure seems to be less critical as it could be accounted for via TDM-guided dose adjustment. Even so, first dose underexposure could lead to the need for substantial dose augmentation, thus reaching a toxic Cmax associated with SOS occurrence (50). This is particularly of concern in the case of once-daily dosing, where plasma concentrations reached are high and dose modifications are more considerable to correct the desired exposure in only four administered doses. Dividing the first dose into two half doses counteracts this risk and has been used successfully for many years in some centres (77).
The two strategies that can be implemented to personalise the first dose of Busulfan are the “test dose strategy” and the “first dose strategy.” The test dose strategy consists of the administration of a small dose of Busulfan ≥2 days before the start of the typical 4-day Busulfan conditioning course. This is particularly useful when the laboratory performing the Busulfan PK analysis is not on-site. The Busulfan PK obtained from the test dose is used to modify the first full dose according to the predicted PK and the chosen target exposure (78, 79). The first dose strategy consists of the personalization of the first dose according to the demographic and clinical attributes of the patient (age, weight, etc.). This strategy is based on dosing nomograms or algorithms derived from population PK studies. The advantage of this strategy is that it better considers each patient's individual characteristics for the recommendation of accurate first doses. As shown in Table 2, body size metrics (actual body weight, body surface area, fat-free mass, etc.) are covariates consistently reported to explain Busulfan PK variability in paediatric patients and are used for dose calculations (38, 57, 63, 66, 67, 80, 82–100).
Several studies have also included an age-based metric to describe the ontogeny and maturation of Busulfan clearance. Such a model has been shown to result in accurate PK predictions and selection of the first dose in paediatric patients (75, 101–103).
For both the test dose and first dose strategies, intraindividual (i.e., inter-day) PK variability of Busulfan mandates that repeat PK testing is needed to assess the cumulative AUC over the course of therapy (78–80, 82, 104). In this way, personalised first doses coupled with efficient TDM permits the desired Busulfan exposure to be targeted. More importantly, repeat measurements used for TDM allow the cumulative exposure to be measured: this can inform future studies, particularly as additional drugs are added to the backbone of a Busulfan-based conditioning, so optimising the outcome and minimising the risk of toxicities related to under- or over-exposure.
The Role of Pharmacogenomics in the Pharmacokinetics and Pharmacodynamics of Busulfan-Based Chemoconditioning
In recent years, in an effort to accurately predict Busulfan PK in paediatric patients, the influence of biomarkers explaining Busulfan PK became an area of interest. Table 3 summarises the studies on the association between pharmacogenetic markers and Busulfan PK in paediatric HSCT patients (39, 71, 82, 83, 89, 97, 98, 102, 105–107, 109–120).
As Busulfan is mainly metabolised by glutathione-S-transferases (GSTs) (121, 122), clinical investigations on the influence of genetic polymorphisms related to GST activity on Busulfan PK were initiated in the early 2000's (116). Table 3 shows that Busulfan PK is mainly associated with haplotypes of the promoter regions of GSTA1 (18 studies) and GSTM1 (7 studies). The association between GSTP1 and GSTT1 with Busulfan PK is scarce, probably due to their less important role in Busulfan metabolism compared with A1 and M1 isoforms (123). GSTA1-*B haplotypes have been associated with decreased Busulfan clearance, implying an increased exposure to Busulfan. This is due to decreased GSTA1 expression with *B haplotypes (39, 124). Initially, *A and *B haplotypes of GSTs were determined using one single nucleotide polymorphism (SNP) (either 52G/A rs3957356 or -69C/T rs3957357, in linkage disequilibrium) (113, 116, 117). The association of these haplotypes with Busulfan PK are still being studied (97). More recently, GSTA1 haplotypes have been shown to be more complex, requiring the genotyping of at least four SNPs of the GSTA1 promoter (39, 82, 124). In fact, sub-haplotypes within *A and *B have significantly different gene expression potentials. Within *A haplotypes, the *A1 sub-haplotype has a decreased expression potential than *A2 and *A3 haplotypes. The *A2 haplotype has been associated with a significantly increased clearance and thus lower Busulfan exposure (108). Within *B haplotypes, which are all associated with poor Busulfan metabolism, patients carrying the sub-haplotype *B1b have significantly decreased Busulfan metabolism and clearance compared with other *B haplotypes (39).
These different gene expression potentials have enabled the classification of patients into three (82, 89, 102, 124) or four (39) groups according to their capacity to metabolise Busulfan. GSTA1 polymorphisms have been also associated with the clinical outcome of HSCT (SOS, acute GvHD, transplant-related mortality, engraftment, and survival) (39, 97, 107, 108). These associations are likely to be related to differing exposure to Busulfan according to the GSTA1 haplotype. More recently, genetic polymorphisms explaining the metabolising capacity of GSTA1 have been detected as a significant covariate influencing Busulfan clearance: two recent models included as significant covariates GSTA1 metabolic groups associated with Busulfan metabolic capacity, based on GSTA1 sub-haplotypes (82, 89). Predictions based on these models have enabled researchers to accurately achieve Busulfan AUC within the Busulfan EMA therapeutic window in around 80% of the patients from an independent cohort of which 13% of patients had ALL (82). The addition of GSTA1 metabolic capacity to the model seems to have improved the accuracy of first dose selection.
The pharmacogenomic-based models are likely to enable accurate targeting of Busulfan exposure from the beginning of Busulfan conditioning, limiting the need for dose adjustments. A prospective validation is still required for the implementation of this dosing recommendation, although the model was validated in an external cohort. In addition, the feasibility of the implementation of GSTA1 genotyping in routine clinical practise needs to be assessed. These aspects are being addressed in the current BuGenes01 multicentre, prospective randomised trial (Clinicaltrials.gov identifier: NCT04822532), in which paediatric patients undergoing HSCT will be randomised to either a pharmacogenomic-based first dose algorithm or the best-performing dosing algorithm currently used (86). Personalising the first dose of Busulfan in paediatric patients should enable researchers to appraise the unpredictability of Busulfan PK, thus limiting large dose adjustments that could subsequently overexpose these patients (86).
GST polymorphisms have also been associated with poor HSCT outcomes and TRT, as shown in Table 3. These associations were reported in patients carrying GST haplotypes expressing poor metabolising phenotypes, for example GSTA1*B, GSTM1-null, and GSTP1 313*G haplotypes. Polymorphisms of GSTA1, GSTM1, and GSTP1 were reported as risk factors for SOS (39, 107, 116, 125) and acute GvHD (39, 107, 108, 111), while GSTA1 and GSTM1 have been associated with combined TRTs (39, 107, 108). GSTM1 was associated with graft rejection and mortality within 30 days post-transplant (111), while GSTA1 was associated with neutrophil recovery and survival (97). Whether these associations are solely due to the influence of GST polymorphisms on Busulfan PK is questionable. In a study by Ansari et al., increased TRT was associated with GSTA1 polymorphisms in multivariate logistic regression even when Busulfan exposure was accounted for (39). GSTA1 seems to have a direct influence on the transplant outcomes in addition to influencing Busulfan PK. Furthermore, the same study demonstrated that, in patients within or below the therapeutic window (Css 600 – 900 ng/ml, corresponding to daily AUC of 14.4 −21.6 mg.h/L), GSTA1 haplotypes expressing poor metabolic capacity were associated with higher TRT risk (HR 4.4; p < 0.0005) (39). This association was not observed in patients overexposed to Busulfan (Css >900 ng/mL) for whom TRT rates were very high irrespective of the GSTA1 genotype. This suggests that when patients are within therapeutic exposures, the influence of the poor metabolising capacity of GSTA1 on TRT occurrence is independent of PK. GST polymorphisms could therefore influence toxicities and outcomes of HSCT independently of Busulfan exposure. This aspect should be further explored in future studies of patients receiving Busulfan.
Other genetic markers for Busulfan conditioning toxicities have been reported. In paediatric patients, CYP2B6, CTH, MTHFR, HPSE, UGT2B10, and KIAA1715 were reported as risk factors for SOS (126). The risk related to the combined presence of these markers remains to be studied further. Interestingly, CTH c.1364 TT, a gene coding for cystathionase (an enzyme that participates in the glutathione synthesis pathway), was reported to be associated with SOS risk in combination with GSTA1*B*B (reduced function) (127). The data from the pharmacogenomic add-on study of the FORUM study will address this question. Recent studies have reported that polymorphisms of MGMT (128), ERC1, PLEK, NOP9, and SPRED1 were associated with increased GvHD risk (129) in paediatric HSCT, both studies included ALL patients. Donor polymorphisms of genes encoding interleukins (ILs), such as IL-6, interferon γ (IFNγ), and IL-7Rα, have also been associated with GvHD in studies including adult and paediatric patients receiving HSCT, both studies including ALL diagnoses (130, 131). The inclusion of these genetic variants in prognostic models for TRTs could be useful to guide personalised interventions. Combined with other known risk factors for SOS, genetic markers for increased risk of SOS could aid the selection of reduced toxicity chemo-conditioning regimens (e.g., those composed of maximum of two alkylating agents, or/and Fludarabine based), and the administration of defibrotide prophylaxis. Furthermore, the presence of markers of increased GvHD risk could contribute to the choice of GvHD prophylaxis.
Optimising the Use of Treosulfan
Unlike Busulfan, Treosulfan is a prodrug—to gain cytotoxic activity it has to undergo non-enzymatic pH and temperature dependent transformation to biologically active metabolites—which takes place spontaneously under physiological conditions, without involvement of hepatic metabolism. These epoxy derivates of Treosulfan mediate DNA alkylation and interstrand cross-linking (132, 133).
Due to its strong antineoplastic, myeloablative and immunosuppressive properties as well as favourable toxicity profile, the use of Treosulfan in paediatric HSCT conditioning has grown rapidly. In 2019 it was authorised by the EMA for use as a conditioning treatment in adults and children from 1 month of age.
Much of the early literature on Treosulfan-based conditioning comes from its use in non-malignant disease. High rates of engraftment and low non-regimen-related toxicity have translated into good survival rates (134–136). Commonly encountered regimen-related toxicities include skin toxicity and mild mucosal toxicity (137, 138). Importantly for use in malignant disease, there is a low rate of VOD (137–139); specifically, there is a much lower rate compared with Busulfan in high-risk beta thalassaemia patients (30 vs. 78%, respectively) (140).
An additional and major potential long-term benefit of Treosulfan-based conditioning is that it may be less gonadotoxic than Busulfan (141). Higher rates of spontaneous puberty and menarche and lower luteinizing hormone levels in patients receiving Treosulfan vs. Busulfan all suggest less damage to the gonad; there is hope that this will translate to fertility and pregnancies in the future.
A summary of the use of Treosulfan in malignant disease can be found in Table 4 (34, 142–145).
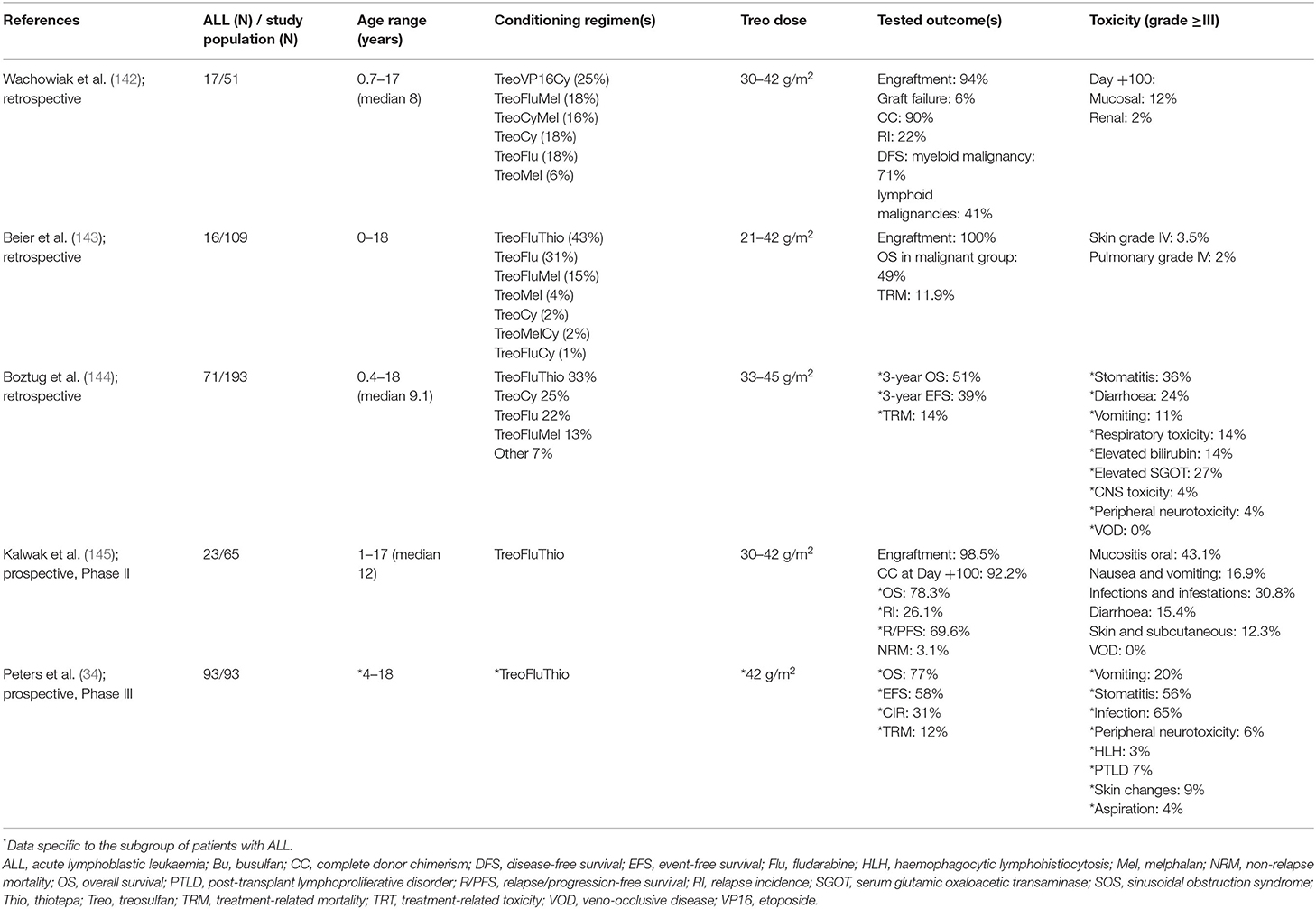
Table 4. Summary of studies assessing the use of treosulfan conditioning in children with malignant diseases.
Toxicity of Treosulfan-Based Conditioning
Prior to the FORUM study, published experience of Treosulfan use in patients with ALL was scarce. Wachowiak et al. retrospectively evaluated 51 children with high risk or advanced haematological malignancies (17 with ALL) transplanted between 2000 and 2005 with Treosulfan-containing conditioning regimens and found no early regimen-related fatal toxicity and a NRM of 16% at 4 years (142). In a retrospective analysis of 109 children transplanted using Treosulfan-based conditioning between 2003 and 2009, approximately half of children had malignancy and 16 had ALL. Treosulfan was combined with agents such as Fludarabine, Thiotepa, and Melphalan. Skin toxicity was frequent but mild with Treosulfan, mucosal toxicity was reduced compared with Busulfan, VOD occurred in 3%, and seizures in 4% of patients (143). Boztug et al.'s retrospective study of 193 children and adolescents with malignant haematological disorders who received HSCT after Treosulfan-based conditioning therapy included 71 with ALL. In accordance with previous studies, toxicity of Treosulfan was low and mainly gastrointestinal in this study. VOD and neurological toxicity were rare. No association of toxicity with type of disease or Treosulfan dose was found. TRM was at 14% (144).
In a Phase II, prospective, multicentre study conducted by Kalwak et al., Treosulfan-Fludarabine-Thiotepa conditioning was investigated in 65 children with a haematological malignancy (3 ALL, 29 AML, 10 myelodysplastic syndrome and 3 juvenile myelomonocytic leukaemia). Treosulfan was dosed by body surface area (BSA), with those patients ≤ 0.5 m2 receiving 10 g/m2/day; those >0.5–1.0 m2 12 g/m2/day and those >1.0 m2 14 g/m2/day for 3 days. Overall, 98.5% of patients achieved engraftment, with complete donor chimerism in 92.6% at 12 months. The most frequently reported toxicities of grade 3–4 were oral mucositis (43.1%), infections (30.8%), nausea and vomiting (16.9%), skin and subcutaneous tissue disorders (12.3%), and hepatic VOD (1.4%). NRM was estimated to be low, at 3.1% (145).
To date, only preliminary results of the Treosulfan arm in the FORUM trial have been published: the most frequent early grade 3–4 toxicities included infections (65%) and stomatitis (56%), while skin toxicity of grade 3–4 was present in 9% of patients. Of concern, neither the Treosulfan nor Busulfan arm compared favourably with TBI with regards to TRM in the modified as-treated population (12, 6, and 3%, respectively; p = 0.1103). Analysing the two chemotherapy groups together, the higher TRM compared to the TBI arm (9 vs. 2%, p = 0.027) contributed to the lower overall survival, triggering the cessation of randomisation to the chemotherapy arms (34). This raises concerns of duplicating what was seen in the PBMTC study (20), with a more intensive and thus toxic combination of chemotherapy agents not comparing favourably with the well-known early toxicity profile of TBI.
Outcome Data for Treosulfan-Based Conditioning in Paediatric ALL HSCT
Prior to the FORUM trial, children with ALL receiving Treosulfan-based conditioning therapy prior to HSCT were reported in cohorts together with non-malignant disorders (143) or with other (myeloid) malignancies (142, 145). The numbers of paediatric ALL patients included in trials did not exceed 71 in retrospective cohorts (144) or 23 in prospective trials (145). The more robust outcome data for Treosulfan-based conditioning come from studies with myeloid malignancies in adults (146–148).
In the retrospective study of Wachowiak et al. referred to above, the estimated 4-year probability of DFS was 71% for those with myeloid malignancies and 41% in the 20 patients with lymphoid malignancies (predominately ALL), with an acceptable relapse incidence of 24% at 4 years (142). Beier et al., in a cohort including 16 patients with ALL and 11 with AML, reported a 3-year EFS of 49% and with predominant cause of death being relapse (143). In the European Society for Bone and Marrow Transplantation (EBMT) Paediatric Diseases Working Party retrospective analysis of Treosulfan-based conditioning for Haematological malignancy, the 3-year EFS was 45% and disease-related mortality 32% for the 71 ALL patients (144). The addition of an additional alkylator (either Thiotepa or Melphalan) to the Treosulfan-Fludarabine backbone resulted in significantly better OS.
One should bear in mind that these early retrospective studies selected patients who were felt to be at high risk for regimen-related toxicity, especially pulmonary and hepatic (VOD) toxicity associated with standard of care myeloablative regimes (TBI or Busulfan based). In 23 prospectively studied paediatric ALL patients given Treosulfan-Fludarabine-Thiotepa, Kalwak et al. estimated the relapse/progression incidence to be 26.1%, the relapse/progression free survival to be 69.9% and OS to be 78.3% at 36 months (145). Outcomes were comparable across each of the BSA-based Treosulfan doses (10, 12, and 14g/m2).
The most valuable knowledge on the efficacy of Treosulfan-Fludarabine-Thiotepa conditioning before HSCT for paediatric ALL comes from the 99 patients with ALL randomised to this regimen in the FORUM trial (34). Outcomes in the Treosulfan arm in the modified as-treated population-−58% EFS, 77% OS, 31% cumulative incidence of relapse and 12% TRM at 2 years—were significantly lower than the TBI arm (85% EFS, 91% OS, 12% cumulative incidence of relapse and 3% TRM at 2 years), clearly not supporting the use of an unadjusted Treosulfan regimen for patients eligible for TBI.
Treosulfan Pharmacokinetics and Outcome
One difference between the Busulfan and Treosulfan arms in the FORUM study is that we know a significant proportion of patients in the Busulfan arm will have had PK analysis performed, with subsequent TDM. In contrast, we do not expect any of the Treosulfan-assigned patients to have had TDM. A fundamental question remains unanswered: is there a meaningful relationship between drug exposure and clinical outcome for Treosulfan and will optimization of dose and TDM improve the EFS vs. TBI when compared with the non-TDM-targeted Treosulfan usage in FORUM?
We know that, like most of the drugs we use in conditioning, there is high inter-patient variability in exposure to Treosulfan (149). To date, most of the PK data for Treosulfan was collected in patients with non-malignant disease. Van der Stoep et al. performed a prospective multicentre study in 77 children undergoing HSCT (84.4% of whom had non-malignant disease), focussing on the PK profile of Treosulfan. Their results showed that there is a relationship between Treosulfan exposure and early toxicity. Patients with higher exposure (AUC >1,650 mg.h/L) had an increased risk of developing grade 2 or higher mucositis and skin toxicity. No correlation between Treosulfan exposure and the early clinical outcome parameters (engraftment, acute GvHD or donor chimerism) was found (149). A prospective study in two UK centres looked at Treosulfan PK and PD in children undergoing allogeneic HSCT mainly for primary immunodeficiency after Treosulfan-Fludarabine conditioning. An association between high AUC and mortality as well as low AUC and poor engraftment was shown (150).
Mohanan et al. studied 87 patients with thalassaemia major undergoing allogeneic HSCT. Treosulfan clearance of <7.97 L/h/m2 was significantly associated with poor OS and EFS; where as high Treosulfan clearance (>7.97 L/h/m2) and low AUC (<1,828 mg.h/L) showed a trend toward better OS (151).
Thus, it can be postulated that there is likely to be an association of outcome and toxicity parameters with Treosulfan exposure, yet perhaps the improved safety profile of Treosulfan over Busulfan makes this more difficult to establish until we have available larger studies on more uniform populations. In most protocols, Treosulfan is administered over 3 consecutive days in doses of 10–14 g/m2/day, with the dose adjusted according to age or body weight. Despite the dose reduction to 10 g/m2 in infants, admittedly with a variety of diagnoses, including many with non-malignant disease, Treosulfan exposure remained higher compared with older children receiving 14 g/m2 (149). We may find that it is in these younger (and so smaller) patients where Treosulfan TDM has a role. In order to identify and quantify sources of variability in drug concentration and to predict concentrations in individual patients, PK models have been developed (152–154). Clearly, the currently available data are not sufficient to inform a practise guideline for TDM of Treosulfan in paediatric ALL—the relationship of Treosulfan exposure to leukaemia-free survival has not been described. A number of clinical trials incorporating Treosulfan PK evaluation are underway that may provide additional insights. In particular, the PK data on Treosulfan from the FORUM trial are eagerly awaited.
Introducing Clofarabine into Conditioning Regimens
Clofarabine is a second-generation purine nucleoside analogue that was designed to improve outcomes and minimise toxicity in the treatment of acute leukaemia. It inhibits DNA synthesis and repair and also disrupts the mitochondrial membrane resulting in programmed cell death. It has been studied widely in the setting of relapsed/refractory ALL over the past decade and was approved for the use in refractory or relapsed ALL in children by the FDA in 2004.
It has an acceptable toxicity profile with more frequent adverse reactions including febrile neutropenia, nausea/anorexia, cytokine-release–like events, skin rash and hand-foot syndrome (155–157). This safety profile supports the feasibility of combining Clofarabine with other effective agents based on pharmacological properties and mechanisms of action. In particular, the combination of Clofarabine, Cyclophosphamide and etoposide for conditioning has been studied in children with relapsed or refractory ALL undergoing HSCT and has been found to be well-tolerated, with overall response rates of 28–67% (158–160).
Use of Clofarabine in HSCT Conditioning
One advantage of Clofarabine is that it is not associated with the neurotoxicity seen with other similar nucleoside analogues. In order to reduce toxicity but sustain efficacy, studies both in vitro and in vivo have been done where nucleoside analogues replace alkylating agents. In vitro cell line studies showed the clear synergistic cytotoxicity of Clofarabine and Fludarabine, which was further enhanced by adding Busulfan. This finding led to the combination of Clofarabine, Fludarabine, and Busulfan being investigated by the MD Anderson group (161, 162).
In that randomised controlled trial, 51 adult patients with high-risk myeloid leukaemias were randomised to receive Clofarabine-Fludarabine-Busulfan conditioning across four treatment arms that differed with respect to the Clo and Fludarabine dosing used. Initial findings were encouraging with regard to safety and antileukemic activity (162). Longer follow up of this expanded cohort (n = 70) confirmed the safety, OS and PFS advantage of the arms with higher Clofarabine doses and lower Fludarabine doses (163).
The same group studied Clofarabine and Busulfan in 107 adults undergoing HSCT for ALL (164, 165). With a median follow up of 3.3 years, 2-year leukaemia-free survival was 51% (being best in CR1 patients, at 62%), and NRM was 6% at day 100 and 18% at 2 years. These outcomes compare favourably with reports of adult patients with ALL in CR1 treated with myeloablative TBI-based regimens.
There are few data published on the use of Clofarabine for HSCT conditioning in paediatric patients. A retrospective analysis in paediatric AML using a common backbone of induction chemotherapy followed by three different chemotherapy conditioning regimens suggested that Clofarabine-Fludarabine-Busulfan had good anti-leukaemic activity with low NRM. In comparison, Busulfan-Cyclophosphamide was associated with higher relapse incidence, while Busulfan-Cyclophosphamide-Melphalan was associated with higher incidence of acute GVHD (166).
In a cohort of 60 paediatric ALL patients undergoing HSCT after Clofarabine-Fludarabine-Busulfan conditioning, the 2-year estimated EFS probability was 72.0% ± 6.0, with significantly lower EFS observed in patients with MRD positivity prior to HSCT. Two-year TRM probability was low at only 5.0% ± 2.8 and no VOD was seen.
At the time of writing, there were no ongoing clinical studies of Clofarabine use in HSCT conditioning regimens.
Optimising the Entire Conditioning Regimen
We have tried to address the issues around optimising the PK and PD of the individual alkylators in the conditioning regimen, but it is equally important to address the impact of the entire package on efficacy and toxicity.
Substituting Alkylating Agents
For Busulfan, acute and chronic toxicities remain a matter of concern even when Busulfan target exposures are strictly controlled (50, 167). As shown by several studies, the use of multiple alkylating agents in conditioning regimens is a predictor of acute toxicity in paediatric patients (36, 37). For this reason and based on adult experience, the nucleoside analogue Fludarabine—an inhibitor of DNA, RNA and protein synthesis—has been introduced as an immunosuppressive agent in the replacement of Cyclophosphamide in paediatric transplantation. The majority of data comparing Fludarabine-Busulfan to Busulfan-Cyclophosphamide conditioning regimens come from adult patients, although some of these studies included children and adolescents. The meta-analysis by Ben-Barouch et al. included studies with paediatric ALL patients (168). The authors reported that a lower risk of NRM was associated with Fludarabine-Busulfan vs. Busulfan-Cyclophosphamide, while OS was similar between the two regimens. The same study found that Fludarabine-Busulfan was associated with lower risk of SOS than Busulfan-Cyclophosphamide. However, when only considering randomised controlled trials, the SOS risk was similar between the two regimens. A higher risk of microbiological infections was associated with the Busulfan-Cyclophosphamide regimen. Other assessed outcomes (GvHD, relapse, engraftment and mucositis) were similar between the two regimens. The meta-analysis concluded that Fludarabine-Busulfan and Busulfan-Cyclophosphamide regimens have similar efficacy, but Fludarabine-Busulfan regimens are slightly more favourable in terms of toxicity profile.
Two important studies have compared Busulfan-Cyclophosphamide and Fludarabine-Busulfan regimens in paediatric HSCT. In the first, Bartelink et al. compared the data of patients prospectively recruited 64 patients (9 ALL) who received Fludarabine-Busulfan conditioning with retrospective data of 50 (5 ALL) patients who received Busulfan-Cyclophosphamide. ALL patients received melphalan (Mel) in addition to Busulfan-Cyclophosphamide. Much like the picture in adults, EFS and OS were similar between conditioning groups, while the risk of TRT such as SOS, chronic GvHD, acute lung toxicity and viral reactivations were lower in patients who received Fludarabine-Busulfan (169). Rates of acute GvHD were similar between the two groups. As shown by more recent data, the use of three alkylating agents is correlated with the occurrence of acute toxicity compared to patients with two or one alkylating agent (36). Mel-containing conditioning regimens were also associated with acute toxicity risk (37). The use of Mel could therefore have contributed to the observed higher toxicity in Busulfan-Cyclophosphamide-Melphalan group in the study by Bartelink et al. A sub-analysis of that study that excluded ALL patients (for whom Mel was indicated), showed less toxicity in patients receiving Fludarabine-Busulfan compared with Busulfan-Cyclophosphamide. The comparison between outcomes of ALL patients receiving Busulfan-Cyclophosphamide-Melphalan vs. Fludarabine-Busulfan was not reported by the authors (169). The second study, by Harris et al., compared Fludarabine-Busulfan and Busulfan-Cyclophosphamide using retrospective data from 1,781 transplanted children. Post-relapse survival was inferior in patients receiving Fludarabine-Busulfan vs. Busulfan-Cyclophosphamide, leading to an inferior OS in those patients (170). In contrast to the Bartelink et al. study, this study showed no difference in transplant-related toxicity and TRM between conditioning groups (170). This suggests that one may still consider the addition of a third agent, but on the backbone of Busulfan-Fludarabine rather than Busulfan-Cyclophosphamide.
In contrast to Busulfan-Cyclophosphamide, there is evidence of a PK drug–drug interaction between Busulfan and Fludarabine. Two studies have shown a significantly decreased clearance of Busulfan when co-administered with Fludarabine (82, 171). As the effect sizes related to Busulfan co-administration reported in these studies were fairly small, the clinical significance of this interaction is likely to be minimal. As Busulfan has a narrow therapeutic window, even this small effect size should be considered for accurate dose individualisation of Busulfan. Furthermore, Busulfan-related toxicities in patients co-administered Busulfan and Fludarabine are also exposure dependent. A higher inter-dose variability was reported in patients receiving a Fludarabine co-administered with Busulfan, than that observed with Busulfan-Cyclophosphamide (172). TDM is therefore important to control for this increased PK variability observed when Busulfan is used alongside Fludarabine in conditioning regimens.
Pharmacokinetics of Fludarabine
There is a small but emerging literature on Fludarabine PK in Paediatric Transplantation. Retrospective data suggested high levels were associated with more toxicity, particularly in the setting of renal impairment (173). A more recent prospective multicentre study again showed that renal impairment predictably increased AUC. In this paediatric study, it is likely that many of the patients had reduced intensity grafts, some received fludarabine alone and so the low TRM made it difficult to demonstrate if there was an relationship between exposure and TRM (174). Another paediatric study also found no association between exposure and clinically important end-points (175).
More interestingly, there has been a first attempt to look at the impact of the pharmacokinetics of Fludarabine in combination with Busulfan (176). Rather than a multivariate analysis of the impact of the PK of both Fludarabine and Busulfan independently and then looking for any interaction, the paper describes the impact of Fludarabine PK within a retrospective cohort of patients who were all given a set dose of 160 mg/m2 of Fludarabine combined with what is described as a targeted dose exposure of Busulfan. In fact, although an AUC of 90 mg.h/L was targeted, the mean exposure to Buslfan achieved was 96.1, with a wide range of AUC from 59 to 120 mg.h/L. Within this large series of adult and paediatric patients, including some leukaemias, and with a consequent much higher rate of TRM (28%) than the purely paediatric studies quoted above, the authors found that higher exposure associated with more toxicity and lower levels associated with more rejections. They suggested that an optimal cumulative exposure could be targeted by refinement of the current surface area based dosing, or measured as part of a TDM strategy. Give the variability in the exposure to Busulfan, which was not explored in this retrospective study, this is an illustration of the way forward.
Pharmacokinetics of the “Serotherapy”
The chemotherapy drugs used in transplant conditioning are not given in isolation. Additional immunosuppression, depending on donor type and cell source, is added in, typically in the form of agents such as Anti-T cell polyclonal antibodies or monoclonal antibodies, such as Alemtuzumab. Their use is considered in a separate chapter of this issue.
Adding to Busulfan
The Busulfan-based protocol used in the FORUM study added Thiotepa (10 mg/kg divided into two doses) to the Busulfan and Fludarabine. This combination is based upon protocols mainly studied in adult patients (177, 178), umbilical cord blood transplantation (179–181), haploidentical HSCT (180, 182), and reduced intensity regimens (183). The rationale behind the addition of Thiotepa was to improve the engraftment rates in adult umbilical cord blood transplanted patients, which was insufficient under a Fludarabine-Busulfan regimen (179, 184, 185). The original protocols used only 3 days of Busulfan at 3.2 mg/mL daily, thus a lower cumulative dose than myeloablative regimens. In FORUM, this protocol was used as the Busulfan-based conditioning arm but with the standard 4 days of conditioning and myeloablative target exposures suggested. In adult AML, intensifying Fludarabine-Busulfan-Thiotepa conditioning with full myeloablative doses of Busulfan resulted in significantly lower relapse [hazard ratio (HR) 0.47; p = 0.005] but higher NRM (HR 2.69; p < 0.001) compared with a myeloablative Fludarabine-Busulfan regimen (178). Leukaemia-free survival and OS was similar between the two regimens. Fludarabine-Busulfan-Thiotepa has been reported also to result in a lower relapse rate (HR 0.6; p = 0.02) and similar OS compared with Busulfan-Cyclophosphamide in adult AML patients (177, 178). Fludarabine-Busulfan-Thiotepa had not been studied in the conventional matched donor setting in ALL paediatric patients prior to the FORUM study. It remains unknown if this combination results in optimal outcomes in paediatric ALL and should be tested against other Busulfan-based regimens in paediatric ALL patients is therefore needed.
Adding to Treosulfan
As described in Section Optimizing the Use of Treosulfan above, the favourable toxicity profile of Treosulfan, combined with its limited activity when combined with Fludarabine alone, led to the addition of a third agent, often Thiotepa or Melphalan.
Pharmacokinetics of the Whole Conditioning Regimen
When using potentially toxic drugs at high doses for a short period of time, after gaining as much PK and PD information as possible from investigations of each single drug, it becomes important to look at the impact of the agents in combination. For ALL, we have added Thiotepa to Fludarabine partnered with Busulfan or Treosulfan, or used Clofarabine. We then have to consider the impact of the serotherapy used. It is naïve to believe that the complex relationship between disease and disease status, type of donor and cell source used after giving multi-agent chemotherapy combined with serotherapy will have a simple relationship to even complex descriptors of any one of the conditioning agents used. For the next phase of our international PK/PD effort, we should attempt to share data to integrate information regarding each element of the conditioning. In this way, we can move closer to our goal of optimising conditioning for each individual patient.
Conclusion: Where Do We Go From Here?
Although only initial results are available from the FORUM trial (34), these give us some clear insights that can help to determine where we should go to from here:
• TBI was superior to both Treosulfan-based and Busulfan-based chemo-conditioning.
• This superiority extended across all sub-group analyses, regardless of age, phenotype, MRD status, donor type, remission status, timing, and type of relapse.
• TRM was higher in the chemo-conditioning arms compared with the TBI arm (p = 0.027) and tended to be higher with Treosulfan-based vs. Busulfan-based conditioning.
This clearly indicates that any attempt to non-specifically increase dosing for chemo-conditioning would result in a similar, dismal outcome to that observed 20 years ago in the PBMTC Study (20).
In addition to HSCT following TBI-based conditioning being effective therapy for those over 4 years of age with ALL (whether or not they have precursor B-cell lymphoblastic leukaemia), alternative therapies including chimeric antigen receptor (CAR) T-cell therapy have become available. Whether the availability of CAR-T cells will influence the choice of a chemotherapy-based vs. TBI-based conditioning is outside the scope of this review.
For patients under 4 years of age (or indeed potentially those under 3 years of age—a subject of debate) requiring HSCT, the life-long adverse effects of irradiation will drive the majority of paediatric transplanters to persist in optimising and using chemo-conditioning. Therefore, going forward, this is the group where we need to refine chemo-conditioning regimens. Although the three-drug combination of Busulfan-Fludarabine-Thiotepa has been used in significant numbers of patients, it worth noting that most of these patients were not paediatric patients with ALL and did not receive a matched donor graft (177, 179, 182, 183, 186). Furthermore, the dosing of Busulfan used in these published studies was three-quarters of the standard dose and we have not finished analysing the impact of Busulfan dose in the context of the FORUM study. This work will allow us to study the impact of various levels of exposure to Busulfan in children with ALL and determine whether factors such as cumulative dose given, cumulative exposure, method of dosing (such as once vs. multiple times per day) and/or pharmacogenomics will allow us to optimise individualised Busulfan dosing. Such dosing could then be carried forward into future prospective studies aiming to provide the best anti-leukaemic control with the least toxicity.
At the same time, analysis of the Treosulfan PK in the Treosulfan arm of the FORUM trial may suggest a way of optimising delivery of Treosulfan-based conditioning regimens. Particularly in the youngest patients, it is likely that TDM of Treosulfan will be indicated (149).
We also have to consider the possibility that further clinical data may emerge from new chemotherapy combinations, such as those containing Clo, that have good enough clinical outcomes to support such regimens being evaluated as one arm of future prospective studies.
Given the recent closure of randomisation to chemo-conditioning vs. TBI in the massive international effort of FORUM, it is likely to be some years before investigators are prepared to take on and/or can assemble the necessary resources to conduct another large prospective randomised study in paediatric ALL. As the number of patients <4 years old with ALL is limited, a study in this population would require a truly global effort in order to evaluate chemo-conditioning and could perhaps be conducted as part of an expanded “Interfant” collaborative protocol. Even with a global effort, numbers will mean a non-randomised study is more feasible, but can be based around further analysis of the detailed results of the Busulfan and Treosulfan arms of the FORUM trial and design an optimised chemotherapy-based alternative to TBI for conditioning.
Author Contributions
All authors listed have made a substantial, direct, and intellectual contribution to the work and approved it for publication.
Funding
KB and MA were supported by the Cansearch Foundation.
Conflict of Interest
The authors declare that the research was conducted in the absence of any commercial or financial relationships that could be construed as a potential conflict of interest.
The handling editor declared a shared consortium with the authors at time of review.
Publisher's Note
All claims expressed in this article are solely those of the authors and do not necessarily represent those of their affiliated organizations, or those of the publisher, the editors and the reviewers. Any product that may be evaluated in this article, or claim that may be made by its manufacturer, is not guaranteed or endorsed by the publisher.
References
1. Hill-Kayser CE, Plastaras JP, Tochner Z, Glatstein E. TBI during BM and SCT: review of the past, discussion of the present and consideration of future directions. Bone Marrow Transplant. (2011) 46:475–84. doi: 10.1038/bmt.2010.280
2. Thomas ED, Storb R, Buckner CD. Total-body irradiation in preparation for marrow engraftment. Transplant Proc. (1976) 8:591–3.
3. Baker KS, Bresters D, Sande JE. The burden of cure: long-term side effects following hematopoietic stem cell transplantation (HSCT) in children. Pediatr Clin North Am. (2010) 57:323–42. doi: 10.1016/j.pcl.2009.11.008
4. Thomas E, Storb R, Clift RA, Fefer A, Johnson FL, Neiman PE, et al. Bone-marrow transplantation (first of two parts). N Engl J Med. (1975) 292:832–43. doi: 10.1056/NEJM197504172921605
5. Thomas ED, Buckner CD, Banaji M, Clift RA, Fefer A, Flournoy N, et al. One hundred patients with acute leukemia treated by chemotherapy, total body irradiation, and allogeneic marrow transplantation. Blood. (1977) 49:511–33. doi: 10.1182/blood.V49.4.511.511
6. Thomas ED. A history of haemopoietic cell transplantation. Br J Haematol. (1999) 105:330–9. doi: 10.1111/j.1365-2141.1999.01337.x
7. Johnson FL. Marrow transplantation in the treatment of acute childhood leukemia. Historical development and current approaches. Am J Pediatr Hematol Oncol. (1981) 3:389–95.
8. Santos GW. Busulfan and cyclophosphamide versus cyclophosphamide and total body irradiation for marrow transplantation in chronic myelogenous leukemia–a review. Leuk Lymphoma. (1993) 11:201–4. doi: 10.3109/10428199309047886
9. Santos GW, Tutschka PJ, Brookmeyer R, Saral R, Beschorner WE, Bias WB, et al. Marrow transplantation for acute nonlymphocytic leukemia after treatment with busulfan and cyclophosphamide. N Engl J Med. (1983) 309:1347–53. doi: 10.1056/NEJM198312013092202
10. Tutschka PJ, Copelan EA, Klein JP. Bone marrow transplantation for leukemia following a new busulfan and cyclophosphamide regimen. Blood. (1987) 70:1382–8. doi: 10.1182/blood.V70.5.1382.1382
11. Blaise D, Maraninchi D, Archimbaud E, Reiffers J, Devergie A, Jouet JP, et al. Allogeneic bone marrow transplantation for acute myeloid leukemia in first remission: a randomized trial of a busulfan-Cytoxan versus Cytoxan-total body irradiation as preparative regimen: a report from the Group d'Etudes de la Greffe de Moelle Osseuse. Blood. (1992) 79:2578–82. doi: 10.1182/blood.V79.10.2578.bloodjournal79102578
12. Devergie A, Blaise D, Attal M, Tigaud JD, Jouet JP, Vernant JP, et al. Allogeneic bone marrow transplantation for chronic myeloid leukemia in first chronic phase: a randomized trial of busulfan-cytoxan versus cytoxan-total body irradiation as preparative regimen: a report from the French Society of Bone Marrow Graft (SFGM). Blood. (1995) 85:2263–8. doi: 10.1182/blood.V85.8.2263.bloodjournal8582263
13. Ringden O, Ruutu T, Remberger M, Nikoskelainen J, Volin L, Vindelov L, et al. A randomized trial comparing busulfan with total body irradiation as conditioning in allogeneic marrow transplant recipients with leukemia: a report from the Nordic Bone Marrow Transplantation Group. Blood. (1994) 83:2723–30. doi: 10.1182/blood.V83.9.2723.2723
14. Clift RA, Buckner CD, Thomas ED, Bensinger WI, Bowden R, Bryant E, et al. Marrow transplantation for chronic myeloid leukemia: a randomized study comparing cyclophosphamide and total body irradiation with busulfan and cyclophosphamide. Blood. (1994) 84:2036–43. doi: 10.1182/blood.V84.6.2036.2036
15. Hartman AR, Williams S, Dillon J. Survival, disease-free survival and adverse effects of conditioning for allogeneic bone marrow transplantation with busulfan/cyclophosphamide vs total body irradiation: a meta-analysis. Bone Marrow Transplant. (1998) 22:439–43. doi: 10.1038/sj.bmt.1701334
16. Blaise D, Maraninchi D, Michallet M, Reiffers J, Jouet JP, Milpied N, et al. Long-term follow-up of a randomized trial comparing the combination of cyclophosphamide with total body irradiation or busulfan as conditioning regimen for patients receiving HLA-identical marrow grafts for acute myeloblastic leukemia in first complete remission. Blood. (2001) 97:3669–71. doi: 10.1182/blood.V97.11.3669
17. Ringdén O, Remberger M, Ruutu T, Nikoskelainen J, Volin L, Vindeløv L, et al. Increased risk of chronic graft-versus-host disease, obstructive bronchiolitis, and alopecia with Busulfan versus total body irradiation: long-term results of a randomized trial in allogeneic marrow recipients with leukemia. Blood. (1999) 93:2196–201. doi: 10.1182/blood.V93.7.2196
18. Socie G, Clift RA, Blaise D, Devergie A, Ringden O, Martin PJ, et al. Busulfan plus cyclophosphamide compared with total-body irradiation plus cyclophosphamide before marrow transplantation for myeloid leukemia: long-term follow-up of 4 randomized studies. Blood. (2001) 98:3569–74. doi: 10.1182/blood.V98.13.3569
19. Davies SM, Ramsay NK, Klein JP, Weisdorf DJ, Bolwell B, Cahn JY, et al. Comparison of preparative regimens in transplants for children with acute lymphoblastic leukemia. J Clin Oncol. (2000) 18:340–7. doi: 10.1200/JCO.2000.18.2.340
20. Bunin N, Aplenc R, Kamani N, Shaw K, Cnaan A, Simms S. Randomized trial of busulfan vs. total body irradiation containing conditioning regimens for children with acute lymphoblastic leukemia: a Pediatric Blood and Marrow Transplant Consortium study. Bone Marrow Transplant. (2003) 32:543–8. doi: 10.1038/sj.bmt.1704198
21. Eapen M, Raetz E, Zhang MJ, Muehlenbein C, Devidas M, Abshire T, et al. Outcomes after HLA-matched sibling transplantation or chemotherapy in children with B-precursor acute lymphoblastic leukemia in a second remission: a collaborative study of the Children's Oncology Group and the Center for International Blood and Marrow Transplant Research. Blood. (2006) 107:4961–7. doi: 10.1182/blood-2005-12-4942
22. Friedman DL, Rovo A, Leisenring W, Locasciulli A, Flowers ME, Tichelli A, et al. Increased risk of breast cancer among survivors of allogeneic hematopoietic cell transplantation: a report from the FHCRC and the EBMT-Late Effect Working Party. Blood. (2008) 111:939–44. doi: 10.1182/blood-2007-07-099283
23. Sanders JE, Hoffmeister PA, Woolfrey AE, Carpenter PA, Storer BE, Storb RF, et al. Thyroid function following hematopoietic cell transplantation in children: 30 years' experience. Blood. (2009) 113:306–8. doi: 10.1182/blood-2008-08-173005
24. Rizzo JD, Curtis RE, Socie G, Sobocinski KA, Gilbert E, Landgren O, et al. Solid cancers after allogeneic hematopoietic cell transplantation. Blood. (2009) 113:1175–83. doi: 10.1182/blood-2008-05-158782
25. Andersson BS, Gajewski J, Donato M, Giralt S, Gian V, Wingard J, et al. Allogeneic stem cell transplantation (BMT) for AML and MDS following iv busulfan and cyclophosphamide (iv BuCy). Bone Marrow Transplant. (2000) 25:S35–S8. doi: 10.1038/sj.bmt.1702351
26. Andersson BS, Kashyap A, Gian V, Wingard JR, Fernandez H, Cagnoni PJ, et al. Conditioning therapy with intravenous busulfan and cyclophosphamide (IV BuCy2) for hematologic malignancies prior to allogeneic stem cell transplantation: a phase II study. Biol Blood Marrow Transplant. (2002) 8:145–54. doi: 10.1053/bbmt.2002.v8.pm11939604
27. Bartelink I, Bredius R, Ververs T, Raphael M, Vankesteren C, Bierings M, et al. Once-daily intravenous busulfan with therapeutic drug monitoring compared to conventional oral busulfan improves survival and engraftment in children undergoing allogeneic stem cell transplantation. Biol Blood Marrow Transplant. (2008) 14:88–98. doi: 10.1016/j.bbmt.2007.09.015
28. Veal GJ, Nguyen L, Paci A, Riggi M, Amiel M, Valteau-Couanet D, et al. Busulfan pharmacokinetics following intravenous and oral dosing regimens in children receiving high-dose myeloablative chemotherapy for high-risk neuroblastoma as part of the HR-NBL-1/SIOPEN trial. Eur J Cancer. (2012) 48:3063–72. doi: 10.1016/j.ejca.2012.05.020
29. Kato M, Takahashi Y, Tomizawa D, Okamoto Y, Inagaki J, Koh K, et al. Comparison of intravenous with oral busulfan in allogeneic hematopoietic stem cell transplantation with myeloablative conditioning regimens for pediatric acute leukemia. Biol Blood Marrow Transplant. (2013) 19:1690–4. doi: 10.1016/j.bbmt.2013.09.012
30. Bader P, Hancock J, Kreyenberg H, Goulden NJ, Niethammer D, Oakhill A, et al. Minimal residual disease (MRD) status prior to allogeneic stem cell transplantation is a powerful predictor for post-transplant outcome in children with ALL. Leukemia. (2002) 16:1668–72. doi: 10.1038/sj.leu.2402552
31. Sutton R, Shaw PJ, Venn NC, Law T, Dissanayake A, Kilo T, et al. Persistent MRD before and after allogeneic BMT predicts relapse in children with acute lymphoblastic leukaemia. Br J Haematol. (2015) 168:395–404. doi: 10.1111/bjh.13142
32. Chen X, Hale GA, Barfield R, Benaim E, Leung WH, Knowles J, et al. Rapid immune reconstitution after a reduced-intensity conditioning regimen and a CD3-depleted haploidentical stem cell graft for paediatric refractory haematological malignancies. Br J Haematol. (2006) 135:524–32. doi: 10.1111/j.1365-2141.2006.06330.x
33. Lang P, Teltschik HM, Feuchtinger T, Muller I, Pfeiffer M, Schumm M, et al. Transplantation of CD3/CD19 depleted allografts from haploidentical family donors in paediatric leukaemia. Br J Haematol. (2014) 165:688–98. doi: 10.1111/bjh.12810
34. Peters C, Dalle JH, Locatelli F, Poetschger U, Sedlacek P, Buechner J, et al. Total body irradiation or chemotherapy conditioning in childhood all: a multinational, randomized, non-inferiority phase III study. J Clin Oncol. (2021) 39:295–307. doi: 10.1200/JCO.20.02529
35. Palmer J, McCune JS, Perales M-A, Marks D, Bubalo J, Mohty M, et al. Personalizing busulfan-based conditioning: considerations from the american society for blood and marrow transplantation practice guidelines committee. Biol Blood Marrow Transplant. (2016) 22:1915–25. doi: 10.1016/j.bbmt.2016.07.013
36. Bartelink IH, Lalmohamed A, van Reij EML, Dvorak CC, Savic RM, Zwaveling J, et al. Association of busulfan exposure with survival and toxicity after haemopoietic cell transplantation in children and young adults: a multicentre, retrospective cohort analysis. Lancet Haematol. (2016) 3:e526–e36. doi: 10.1016/S2352-3026(16)30114-4
37. Bartelink IH, Bredius RGM, Belitser SV, Suttorp MM, Bierings M, Knibbe CAJ, et al. Association between busulfan exposure and outcome in children receiving intravenous busulfan before hematologic stem cell transplantation. Biol Blood Marrow Transplant. (2009) 15:231–41. doi: 10.1016/j.bbmt.2008.11.022
38. Ansari M, Theoret Y, Rezgui MA, Peters C, Mezziani S, Desjean C, et al. Association between busulfan exposure and outcome in children receiving intravenous busulfan before hematopoietic stem cell transplantation. Ther Drug Monit. (2014) 36:93–9. doi: 10.1097/FTD.0b013e3182a04fc7
39. Ansari M, Curtis PH-D, Uppugunduri CRS, Rezgui MA, Nava T, Mlakar V, et al. GSTA1 diplotypes affect busulfan clearance and toxicity in children undergoing allogeneic hematopoietic stem cell transplantation: a multicenter study. Oncotarget. (2017) 8:90852–67. doi: 10.18632/oncotarget.20310
40. Baker KS, Bostrom B, DeFor T, Ramsay NK, Woods WG, Blazar BR. Busulfan pharmacokinetics do not predict relapse in acute myeloid leukemia. Bone Marrow Transplant. (2000) 26:607–14. doi: 10.1038/sj.bmt.1702590
41. Bartelink IH, Lalmohamed A, Long-Boyle JR, Boelens JJ. Busulfan after HSCT in children and young adults – authors' reply. Lancet Haematol. (2017) 4:e103–e4. doi: 10.1016/S2352-3026(17)30018-2
42. Benadiba J, Ansari M, Krajinovic M, Vachon MF, Duval M, Teira P, et al. Pharmacokinetics-adapted Busulfan-based myeloablative conditioning before unrelated umbilical cord blood transplantation for myeloid malignancies in children. PLoS ONE. (2018) 13:e0193862. doi: 10.1371/journal.pone.0193862
43. Bolinger AM, Zangwill AB, Slattery JT, Glidden D, DeSantes K, Heyn L, et al. An evaluation of engraftment, toxicity and busulfan concentration in children receiving bone marrow transplantation for leukemia or genetic disease. Bone Marrow Transplant. (2000) 25:925–30. doi: 10.1038/sj.bmt.1702371
44. Bolinger AM, Zangwill AB, Slattery JT, Risler LJ, Sultan DH, Glidden DV, et al. Target dose adjustment of busulfan in pediatric patients undergoing bone marrow transplantation. Bone Marrow Transplant. (2001) 28:1013–8. doi: 10.1038/sj.bmt.1703264
45. Copelan EA, Bechtel TP, Avalos BR, Elder PJ, Ezzone SA, Scholl MD, et al. Busulfan levels are influenced by prior treatment and are associated with hepatic veno-occlusive disease and early mortality but not with delayed complications following marrow transplantation. Bone Marrow Transplant. (2001) 27:1121–4. doi: 10.1038/sj.bmt.1703047
46. Esteves I, Santos FPS, Ribeiro AAF, Seber A, Sugawara EK, Sobrinho J, et al. Targeted-dose of busulfan: Higher risk of sinusoidal obstructive syndrome observed with systemic exposure dose above 5,000 μMol min. A historically controlled clinical trial. Hematol Oncol. (2020) 38:773–81. doi: 10.1002/hon.2789
47. Grochow LB, Jones RJ, Brundrett RB, Braine HG, Chen T-L, Saral R, et al. Pharmacokinetics of busulfan: correlation with veno-occlusive disease in patients undergoing bone marrow transplantation. Cancer Chemother Pharmacol. (1989) 25:55–61. doi: 10.1007/BF00694339
48. Kerl K, Diestelhorst C, Bartelink I, Boelens J, Trame MN, Boos J, et al. Evaluation of effects of busulfan and DMA on SOS in pediatric stem cell recipients. Pediatr Blood Cancer. (2014) 61:306–11. doi: 10.1002/pbc.24827
49. Ljungman P, Hassan M, Békássy AN, Ringdén O, Oberg G. High busulfan concentrations are associated with increased transplant-related mortality in allogeneic bone marrow transplant patients. Bone Marrow Transplant. (1997) 20:909–13. doi: 10.1038/sj.bmt.1700994
50. Philippe M, Neely M, Rushing T, Bertrand Y, Bleyzac N, Goutelle S. Maximal concentration of intravenous busulfan as a determinant of veno-occlusive disease: a pharmacokinetic-pharmacodynamic analysis in 293 hematopoietic stem cell transplanted children. Bone Marrow Transplant. (2019) 54:448–57. doi: 10.1038/s41409-018-0281-7
51. Zwaveling J, Bredius RG, Cremers SC, Ball LM, Lankester AC, Teepe-Twiss IM, et al. Intravenous busulfan in children prior to stem cell transplantation: study of pharmacokinetics in association with early clinical outcome and toxicity. Bone Marrow Transplant. (2005) 35:17–23. doi: 10.1038/sj.bmt.1704707
52. McCune JS, Gooley T, Gibbs JP, Sanders JE, Petersdorf EW, Appelbaum FR, et al. Busulfan concentration and graft rejection in pediatric patients undergoing hematopoietic stem cell transplantation. Bone Marrow Transplant. (2002) 30:167–73. doi: 10.1038/sj.bmt.1703612
53. Philippe M, Goutelle S, Guitton J, Fonrose X, Bergeron C, Girard P, et al. Should busulfan therapeutic range be narrowed in pediatrics? Experience from a large cohort of hematopoietic stem cell transplant children. Bone Marrow Transplant. (2016) 51:72–8. doi: 10.1038/bmt.2015.218
54. Schechter T, Finkelstein Y, Doyle J, Verjee Z, Moretti M, Koren G, et al. Pharmacokinetic disposition and clinical outcomes in infants and children receiving intravenous busulfan for allogeneic hematopoietic stem cell transplantation. Biol Blood Marrow Transplant. (2007) 13:307–14. doi: 10.1016/j.bbmt.2006.10.026
55. Bouligand J, Boland I, Valteau-Couanet D, Deroussent A, Kalifa C, Hartmann O, et al. In children and adolescents, the pharmacodynamics of high-dose busulfan is dependent on the second alkylating agent used in the combined regimen (melphalan or thiotepa). Bone Marrow Transplant. (2003) 32:979–86. doi: 10.1038/sj.bmt.1704275
56. European Medicines Agency,. Busilvex: Summary of Product Characteristics. Amsterdam (2018). Available online at: https://www.ema.europa.eu/en/medicines/human/EPAR/busilvex (accessed November 1, 2021).
57. Nguyen L, Fuller D, Lennon S, Leger F, Puozzo CIV. Busulfan in pediatrics: a novel dosing to improve safety/efficacy for hematopoietic progenitor cell transplantation recipients. Bone Marrow Transplant. (2004) 33:979–87. doi: 10.1038/sj.bmt.1704446
58. Grochow LB. Busulfan disposition: the role of therapeutic monitoring in bone marrow transplantation induction regimens. Semin Oncol. (1993) 20(4Suppl.4):18–25.
59. Dix SP, Wingard JR, Mullins RE, Jerkunica I, Davidson TG, Gilmore CE, et al. Association of busulfan area under the curve with veno-occlusive disease following BMT. Bone Marrow Transplant. (1996) 17:225–30.
60. Slattery JT, Sanders JE, Buckner CD, Schaffer RL, Lambert KW, Langer FP, et al. Graft-rejection and toxicity following bone marrow transplantation in relation to busulfan pharmacokinetics. Bone Marrow Transplant. (1995) 16:31–42.
61. McCune JS, Slattery JT. Pharmacological considerations of primary alkylators. Cancer Treat Res. (2002) 112:323–45. doi: 10.1007/978-1-4615-1173-1_16
62. Slattery JT, Sanders JE, Buckner CD, Schaffer RL, Lambert KW, Langer FP, et al. Graft-rejection and toxicity following bone marrow transplantation in relation to busulfan pharmacokinetics. Bone Marrow Transplant. (1995) 16:31–42.
63. Booth BP, Rahman A, Dagher R, Griebel D, Lennon S, Fuller D, et al. Population pharmacokinetic-based dosing of intravenous busulfan in pediatric patients. J Clin Pharmacol. (2007) 47:101–11. doi: 10.1177/0091270006295789
64. Feng X, Wu Y, Zhang J, Li J, Zhu G, Fan D, et al. Busulfan systemic exposure and its relationship with efficacy and safety in hematopoietic stem cell transplantation in children: a meta-analysis. BMC Pediatr. (2020) 20:176. doi: 10.1186/s12887-020-02028-6
65. McCune JS, Quinones CM, Ritchie J, Carpenter PA, van Maarseveen E, Yeh RF, et al. Harmonization of busulfan plasma exposure unit (BPEU): a community-initiated consensus statement. Biol Blood Marrow Transplant. (2019) 25:1890–7. doi: 10.1016/j.bbmt.2019.05.021
66. Paci A, Poinsignon V, Broutin S, Mir O, Vassal G. Busulfan after HSCT in children and young adults. Lancet Haematol. (2017) 4:e103. doi: 10.1016/S2352-3026(16)30189-2
67. Savic RM, Cowan MJ, Dvorak CC, Pai S-Y, Pereira L, Bartelink IH, et al. Effect of weight and maturation on busulfan clearance in infants and small children undergoing hematopoietic cell transplantation. Biol Blood Marrow Transplant. (2013) 19:1608–14. doi: 10.1016/j.bbmt.2013.08.014
68. Shaw PJ, Nath C, Berry A, Earl JW. Busulphan given as four single daily doses of 150 mg/m2 is safe and effective in children of all ages. Bone Marrow Transplant. (2004) 34:197–205. doi: 10.1038/sj.bmt.1704560
69. Zwaveling J, den Hartigh J, Lankester AC, Guchelaar HJ, Egeler RM, Maarten Bredius RG. Once-daily intravenous busulfan in children prior to stem cell transplantation: study of pharmacokinetics and early clinical outcomes. Anticancer Drugs. (2006) 17:1099. doi: 10.1097/01.cad.0000231482.15277.48
70. González-Vicent M, Molina B, Pérez A, Díaz MA. Once-daily intravenous busulfan for 47 pediatric patients undergoing autologous hematopoietic stem cell transplantation: a single center study. J Pediatr Hematol Oncol. (2012) 34:180–3. doi: 10.1097/MPH.0b013e3182431e1b
71. Lee JW, Kang HJ, Lee SH Yu K-S, Kim NH, Yuk YJ, et al. Highly variable pharmacokinetics of once-daily intravenous busulfan when combined with fludarabine in pediatric patients: phase I clinical study for determination of optimal once-daily busulfan dose using pharmacokinetic modeling. Biol Blood Marrow Transplant. (2012) 18:944–50. doi: 10.1016/j.bbmt.2011.11.025
72. Lee JW, Kang HJ, Kim S, Lee SH Yu K-S, Kim NH, et al. Favorable outcome of hematopoietic stem cell transplantation using a targeted once-daily intravenous busulfan-fludarabine-etoposide regimen in pediatric and infant acute lymphoblastic leukemia patients. Biol Blood Marrow Transplant. (2015) 21:190–5. doi: 10.1016/j.bbmt.2014.09.013
73. Xhaard A, Rzepecki P, Valcarcel D, Santarone S, Fürst S, Serrano D, et al. Optimization of health-care organization and perceived improvement of patient comfort by switching from intra-venous BU four-times-daily infusions to a once-daily administration scheme in adult hematopoietic stem cell recipients. Bone Marrow Transplant. (2014) 49:509–12. doi: 10.1038/bmt.2013.220
74. Singhal S, Kim T, Jenkins P, Bassett B, Tierney DK, Rezvani AR. Costs and outcomes with once-daily versus every-6-hour intravenous busulfan in allogeneic hematopoietic cell transplantation. Biol Blood Marrow Transplant. (2020) 26:145–9. doi: 10.1016/j.bbmt.2019.09.008
75. Lawson R, Paterson L, Fraser CJ, Hennig S. Evaluation of two software using Bayesian methods for monitoring exposure and dosing once-daily intravenous busulfan in paediatric patients receiving haematopoietic stem cell transplantation. Cancer Chemother Pharmacol. (2021). 88:379–91. doi: 10.1007/s00280-021-04288-0
76. Azab AK, Runnels JM, Pitsillides C, Moreau AS, Azab F, Leleu X, et al. CXCR4 inhibitor AMD3100 disrupts the interaction of multiple myeloma cells with the bone marrow microenvironment and enhances their sensitivity to therapy. Blood. (2009) 113:4341–51. doi: 10.1182/blood-2008-10-186668
77. Willcox A, Wong E, Nath C, Janson B, Harrison SJ, Hoyt R, et al. The pharmacokinetics and pharmacodynamics of busulfan when combined with melphalan as conditioning in adult autologous stem cell transplant recipients. Ann Hematol. (2018) 97:2509–18. doi: 10.1007/s00277-018-3447-x
78. Kletzel M, Jacobsohn D, Duerst R. Pharmacokinetics of a test dose of intravenous busulfan guide dose modifications to achieve an optimal area under the curve of a single daily dose of intravenous busulfan in children undergoing a reduced-intensity conditioning regimen with hematopoietic stem cell transplantation. Biol Blood Marrow Transplant. (2006) 12:472–9. doi: 10.1016/j.bbmt.2005.12.028
79. Brooks KM, Jarosinski P, Hughes T, Kang E, Shah NN, Gall JBL, et al. Test dose pharmacokinetics in pediatric patients receiving once-daily IV busulfan conditioning for hematopoietic stem cell transplant: a reliable approach? J Clin Pharmacol. (2018) 58:332–9. doi: 10.1002/jcph.1049
80. Bartelink IH, Boelens JJ, Bredius RG, Egberts AC, Wang C, Bierings MB, et al. Body weight-dependent pharmacokinetics of busulfan in paediatric haematopoietic stem cell transplantation patients: toward individualized dosing. Clin Pharmacokinet. (2012) 51:331–45. doi: 10.2165/11598180-000000000-00000
81. Bartelink IH, Van Kesteren C, Boelens JJ, Egberts TCG, Bierings MB, Cuvelier GDE et al. Predictive performance of a busulfan pharmacokinetic model in children and young adults. Ther Drug Monit. (2012) 34:574–83. doi: 10.1097/FTD.0b013e31826051bb
82. Ben Hassine K, Nava T, Theoret Y, Nath CE, Daali Y, Kassir N, et al. Precision dosing of intravenous busulfan in pediatric hematopoietic stem cell transplantation: results from a multicenter population pharmacokinetic study. CPT Pharmacometrics Syst Pharmacol. (2021) 10:1043–56. doi: 10.1002/psp4.12683
83. Choi B, Kim MG, Han N, Kim T, Ji E, Park S, et al. Population pharmacokinetics and pharmacodynamics of busulfan with GSTA1 polymorphisms in patients undergoing allogeneic hematopoietic stem cell transplantation. Pharmacogenomics. (2015) 16:1585–94. doi: 10.2217/pgs.15.98
84. Diestelhorst C, Boos J, McCune JS, Hempel G. Population pharmacokinetics of intravenous busulfan in children: revised body weight-dependent NONMEM® model to optimize dosing. Eur J Clin Pharmacol. (2014) 70:839–47. doi: 10.1007/s00228-014-1692-z
85. Kawazoe A, Funaki T, Kim S. Population pharmacokinetic analysis of busulfan in Japanese pediatric and adult HCT patients. J Clin Pharmacol. (2018) 58:1196–204. doi: 10.1002/jcph.1120
86. McCune JS, Bemer MJ, Barrett JS, Scott Baker K, Gamis AS, Holford NHG. Busulfan in infant to adult hematopoietic cell transplant recipients: a population pharmacokinetic model for initial and Bayesian dose personalization. Clin Cancer Res. (2014) 20:754–63. doi: 10.1158/1078-0432.CCR-13-1960
87. Langenhorst JB, Boss J, van Kesteren C, Lalmohamed A, Kuball J, Egberts ACG, et al. A semi-mechanistic model based on glutathione depletion to describe intra-individual reduction in busulfan clearance. Br J Clin Pharmacol. (2020) 86:1499–509. doi: 10.1111/bcp.14256
88. Long-Boyle JR, Savic R, Yan S, Bartelink I, Musick L, French D, et al. Population pharmacokinetics of busulfan in pediatric and young adult patients undergoing hematopoietic cell transplant: a model-based dosing algorithm for personalized therapy and implementation into routine clinical use. Ther Drug Monit. (2015) 37:236–45. doi: 10.1097/FTD.0000000000000131
89. Nava T, Kassir N, Rezgui MA, Uppugunduri CRS, Huezo-Diaz Curtis P, Duval M, et al. Incorporation of GSTA1 genetic variations into a population pharmacokinetic model for IV busulfan in paediatric hematopoietic stem cell transplantation: GSTA1-based busulfan population pharmacokinetic model in children. Br J Clin Pharmacol. (2018) 84:1494–504. doi: 10.1111/bcp.13566
90. Neely M, Philippe M, Rushing T, Fu X, van Guilder M, Bayard D, et al. Accurately achieving target busulfan exposure in children and adolescents with very limited sampling and the BestDose software. Ther Drug Monit. (2016) 38:332–42. doi: 10.1097/FTD.0000000000000276
91. Philippe M, Neely M, Bertrand Y, Bleyzac N, Goutelle SA. Nonparametric method to optimize initial drug dosing and attainment of a target exposure interval: concepts and application to busulfan in pediatrics. Clin Pharmacokinet. (2017) 56:435–47. doi: 10.1007/s40262-016-0448-6
92. Poinsignon V, Faivre L, Nguyen L, Neven B, Broutin S, Moshous D, et al. New dosing nomogram and population pharmacokinetic model for young and very young children receiving busulfan for hematopoietic stem cell transplantation conditioning. Pediatr Blood Cancer. (2020) 67:e28603. doi: 10.1002/pbc.28603
93. Rhee SJ, Lee JW Yu KS, Hong KT, Choi JY, Hong CR, et al. Pediatric patients undergoing hematopoietic stem cell transplantation can greatly benefit from a novel once-daily intravenous busulfan dosing nomogram. Am J Hematol. (2017) 92:607–13. doi: 10.1002/ajh.24734
94. Shukla P, Goswami S, Keizer RJ, Winger BA, Kharbanda S, Dvorak CC, et al. Assessment of a model-informed precision dosing platform use in routine clinical care for personalized busulfan therapy in the pediatric hematopoietic cell transplantation (HCT) population. Front Pharmacol. (2020) 11:888. doi: 10.3389/fphar.2020.00888
95. Trame MN, Bergstrand M, Karlsson MO, Boos J, Hempel G. Population pharmacokinetics of busulfan in children: increased evidence for body surface area and allometric body weight dosing of busulfan in children. Clin Cancer Res. (2011) 17:6867–77. doi: 10.1158/1078-0432.CCR-11-0074
96. Wu X, Xie H, Lin W, Yang T, Li N, Lin S, et al. Population pharmacokinetics analysis of intravenous busulfan in Chinese patients undergoing hematopoietic stem cell transplantation. Clin Exp Pharmacol Physiol. (2017) 44:529–38. doi: 10.1111/1440-1681.12735
97. Yuan J, Sun N, Feng X, He H, Mei D, Zhu G, et al. Optimization of busulfan dosing regimen in pediatric patients using a population pharmacokinetic model incorporating GST mutations. Pharmgenomics Pers Med. (2021) 14:253–68. doi: 10.2147/PGPM.S289834
98. Zwaveling J, Press RR, Bredius RGM, van derStraaten TR, den Hartigh J, Bartelink IH. Glutathione S-transferase polymorphisms are not associated with population pharmacokinetic parameters of busulfan in pediatric patients. Ther Drug Monit. (2008) 30:504. doi: 10.1097/FTD.0b013e3181817428
99. Buffery PJ, Allen KM, Chin PK, Moore GA, Barclay ML, Begg EJ. Thirteen years' experience of pharmacokinetic monitoring and dosing of busulfan: can the strategy be improved? Ther Drug Monit. (2014) 36:86–92. doi: 10.1097/FTD.0b013e31829dc940
100. Wall DA, Chan KW, Nieder ML, Hayashi RJ, Yeager AM, Kadota R, et al. Safety, efficacy, and pharmacokinetics of intravenous busulfan in children undergoing allogeneic hematopoietic stem cell transplantation. Pediatr Blood Cancer. (2010) 54:291–8. doi: 10.1002/pbc.22227
101. Zao JH, Schechter T, Liu WJ, Gerges S, Gassas A, Egeler RM, et al. Performance of busulfan dosing guidelines for pediatric hematopoietic stem cell transplant conditioning. Biol Blood Marrow Transplant. (2015) 21:1471–8. doi: 10.1016/j.bbmt.2015.05.006
102. Nava T, Rezgui MA, Uppugunduri CRS, Curtis PH-D, Théoret Y, Duval M, et al. GSTA1 genetic variants and conditioning regimen: missing key factors in dosing guidelines of busulfan in pediatric hematopoietic stem cell transplantation. Biol Blood Marrow Transplant. (2017) 23:1918–24. doi: 10.1016/j.bbmt.2017.07.022
103. Kishimoto K, Hasegawa D, Irie K, Okada A, Nakamura S, Tamura A, et al. Pharmacokinetic analysis for model-supported therapeutic drug monitoring of busulfan in Japanese pediatric hematopoietic stem cell transplantation recipients. Pediatr Transplant. (2020) 24:e13696. doi: 10.1111/petr.13696
104. O'Donnell PH, Artz AS, Undevia SD, Pai RK, Del Cerro P, Horowitz S, et al. Phase I study of dose-escalated busulfan with fludarabine and alemtuzumab as conditioning for allogeneic hematopoietic stem cell transplant: reduced clearance at high doses and occurrence of late sinusoidal obstruction syndrome/veno-occlusive disease. Leuk Lymphoma. (2010) 51:2240–9. doi: 10.3109/10428194.2010.520773
105. Abbasi N, Vadnais B, Knutson JA, Blough DK, Kelly EJ, O'Donnell PV, et al. Pharmacogenetics of intravenous and oral busulfan in hematopoietic cell transplant recipients. J Clin Pharmacol. (2011) 51:1429–38. doi: 10.1177/0091270010382915
106. Ansari M, Lauzon-Joset JF, Vachon MF, Duval M, Théoret Y, Champagne MA, et al. Influence of GST gene polymorphisms on busulfan pharmacokinetics in children. Bone Marrow Transplant. (2010) 45:261–7. doi: 10.1038/bmt.2009.143
107. Ansari M, Rezgui MA, Théoret Y, Uppugunduri CRS, Mezziani S, Vachon MF, et al. Glutathione S-transferase gene variations influence BU pharmacokinetics and outcome of hematopoietic SCT in pediatric patients. Bone Marrow Transplant. (2013) 48:939–46. doi: 10.1038/bmt.2012.265
108. Ansari M, Huezo-Diaz P, Rezgui MA, Marktel S, Duval M, Bittencourt H, et al. Influence of glutathione S -transferase gene polymorphisms on busulfan pharmacokinetics and outcome of hematopoietic stem-cell transplantation in thalassemia pediatric patients. Bone Marrow Transplant. (2016) 51:377–83. doi: 10.1038/bmt.2015.321
109. Bonifazi F, Storci G, Bandini G, Marasco E, Dan E, Zani E, et al. Glutathione transferase-A2 S112T polymorphism predicts survival, transplant-related mortality, busulfan and bilirubin blood levels after allogeneic stem cell transplantation. Haematologica. (2014) 99:172–9. doi: 10.3324/haematol.2013.089888
110. Bremer S, Fløisand Y, Brinch L, Gedde-Dahl T, Bergan S. Glutathione transferase gene variants influence busulfan pharmacokinetics and outcome after myeloablative conditioning. Ther Drug Monit. (2015) 37:493–500. doi: 10.1097/FTD.0000000000000180
111. Elhasid R, Krivoy N, Rowe JM, Sprecher E, Adler L, Elkin H, et al. Influence of glutathione S-transferase A1, P1, M1, T1 polymorphisms on oral busulfan pharmacokinetics in children with congenital hemoglobinopathies undergoing hematopoietic stem cell transplantation. Pediatr Blood Cancer. (2010) 55:1172–9. doi: 10.1002/pbc.22739
112. Gaziev J, Nguyen L, Puozzo C, Mozzi AF, Casella M, Perrone Donnorso M, et al. Novel pharmacokinetic behavior of intravenous busulfan in children with thalassemia undergoing hematopoietic stem cell transplantation: a prospective evaluation of pharmacokinetic and pharmacodynamic profile with therapeutic drug monitoring. Blood. (2010) 115:4597–604. doi: 10.1182/blood-2010-01-265405
113. Johnson L, Orchard PJ, Baker KS, Brundage R, Cao Q, Wang X, et al. Glutathione S-transferase A1 genetic variants reduce busulfan clearance in children undergoing hematopoietic cell transplantation. J Clin Pharmacol. (2008) 48:1052–62. doi: 10.1177/0091270008321940
114. Kim SD, Lee JH, Hur EH, Lee JH, Kim DY, Lim SN, et al. Influence of GST gene polymorphisms on the clearance of intravenous busulfan in adult patients undergoing hematopoietic cell transplantation. Biol Blood Marrow Transplant. (2011) 17:1222–30. doi: 10.1016/j.bbmt.2010.12.708
115. Nishikawa T, Yamaguchi H, Ikawa K, Nakayama K, Higashi E, Miyahara E, et al. Influence of GST polymorphisms on busulfan pharmacokinetics in Japanese children. Pediatr Int. (2019) 61:558–65. doi: 10.1111/ped.13859
116. Srivastava A, Poonkuzhali B, Shaji RV, George B, Mathews V, Chandy M, et al. Glutathione S-transferase M1 polymorphism: a risk factor for hepatic venoocclusive disease in bone marrow transplantation. Blood. (2004) 104:1574–7. doi: 10.1182/blood-2003-11-3778
117. ten Brink MH, van Bavel T, Swen JJ, van der Straaten T, Bredius RG, Lankester AC, et al. Effect of genetic variants GSTA1 and CYP39A1 and age on busulfan clearance in pediatric patients undergoing hematopoietic stem cell transplantation. Pharmacogenomics. (2013) 14:1683–90. doi: 10.2217/pgs.13.159
118. Uppugunduri CR, Rezgui MA, Diaz PH, Tyagi AK, Rousseau J, Daali Y, et al. The association of cytochrome P450 genetic polymorphisms with sulfolane formation and the efficacy of a busulfan-based conditioning regimen in pediatric patients undergoing hematopoietic stem cell transplantation. Pharmacogenomics J. (2014) 14:263–71. doi: 10.1038/tpj.2013.38
119. Yin J, Xiao Y, Zheng H, Zhang YC. Once-daily iv BU-based conditioning regimen before allogeneic hematopoietic SCT: a study of influence of GST gene polymorphisms on BU pharmacokinetics and clinical outcomes in Chinese patients. Bone Marrow Transplant. (2015) 50:696–705. doi: 10.1038/bmt.2015.14
120. Acosta-Martin AE, Antinori P, Uppugunduri CRS, Daali Y, Ansari M, Scherl A, et al. Detection of busulfan adducts on proteins. Rapid Commun Mass Spectrom. (2016) 30:2517–28. doi: 10.1002/rcm.7730
121. Czerwinski M, Gibbs JP, Slattery JT. Busulfan conjugation by glutathione S-transferases alpha, mu, and pi. Drug Metab Dispos. (1996) 24:1015–9.
122. Gibbs JP, Yang JS, Slattery JT. Comparison of human liver and small intestinal glutathione S-transferase-catalyzed busulfan conjugation in vitro. Drug Metab Dispos. (1998) 26:52–5.
123. Gibbs JP, Czerwinski M, Slattery JT. Busulfan-glutathione conjugation catalyzed by human liver cytosolic glutathione S-transferases. Cancer Res. (1996) 56:3678–81.
124. Mlakar V, Curtis PH-D, Armengol M, Ythier V, Dupanloup I, Hassine KB, et al. The analysis of GSTA1 promoter genetic and functional diversity of human populations. Sci Rep. (2021) 11:5038. doi: 10.1038/s41598-021-83996-2
125. Huezo-Diaz P, Uppugunduri Satyanarayana CR, Tyagi AK, Krajinovic M, Ansari Djaberi MG. Pharmacogenetic aspects of drug metabolizing enzymes in busulfan based conditioning prior to allogenic hematopoietic stem cell transplantation in children. Curr Drug Metab. (2014) 15:251–64. doi: 10.2174/1389200215666140202214012
126. Waespe N, Strebel S, Jurkovic Mlakar S, Krajinovic M, Kuehni CE, Nava T, et al. Genetic predictors for sinusoidal obstruction syndrome—a systematic review. J Pers Med. (2021) 11:347. doi: 10.3390/jpm11050347
127. Huezo-Diaz Curtis P, Uppugunduri CRS, Muthukumaran J, Rezgui MA, Peters C, Bader P, et al. Association of CTH variant with sinusoidal obstruction syndrome in children receiving intravenous busulfan and cyclophosphamide before hematopoietic stem cell transplantation. Pharmacogenomics J. (2018) 18:64–9. doi: 10.1038/tpj.2016.65
128. Uppugunduri CRS, Huezo-Diaz Curtis P, Nava T, Rezgui MA, Mlakar V, Mlakar SJ, et al. Association study of candidate DNA-repair gene variants and acute graft vs. host disease in pediatric patients receiving allogeneic hematopoietic stem-cell transplantation. Pharmacogenomics J. (2021) 2021:7. doi: 10.1038/s41397-021-00251-7
129. Ansari M, Petrykey K, Rezgui MA, Del Vecchio V, Cortyl J, Ameur M, et al. Genetic susceptibility to acute graft versus host disease in pediatric patients undergoing HSCT. Bone Marrow Transplant. (2021) 2021:8. doi: 10.1038/s41409-021-01386-8
130. Kielsen K, Enevold C, Heilmann C, Sengelov H, Pedersen AE, Ryder LP, et al. Donor genotype in the interleukin-7 receptor alpha-chain predicts risk of graft-versus-host disease and cytomegalovirus infection after allogeneic hematopoietic stem cell transplantation. Front Immunol. (2018) 9:109. doi: 10.3389/fimmu.2018.00109
131. Kim DDH, Yun J, Won H-H, Cheng L, Su J, Xu W, et al. Multiple single-nucleotide polymorphism-based risk model for clinical outcomes after allogeneic stem-cell transplantation, especially for acute graft-versus-host disease. Transplantation. (2012) 94:1250–7. doi: 10.1097/TP.0b013e3182708e7c
132. Hartley JA, O'Hare CC, Baumgart J. DNA alkylation and interstrand cross-linking by treosulfan. Br J Cancer. (1999) 79:264–6. doi: 10.1038/sj.bjc.6690043
133. Romanski M, Pogorzelska A, Glowka FK. Kinetics of in vitro guanine- N7-alkylation in calf thymus DNA by (2 S,3 S)-1,2-epoxybutane-3,4-diol 4-methanesulfonate and (2 S,3 S)-1,2:3,4-diepoxybutane: revision of the mechanism of DNA cross-linking by the prodrug treosulfan. Mol Pharm. (2019) 16:2708–18. doi: 10.1021/acs.molpharmaceut.9b00251
134. Bernardo ME, Zecca M, Piras E, Vacca A, Giorgiani G, Cugno C, et al. Treosulfan-based conditioning regimen for allogeneic haematopoietic stem cell transplantation in patients with thalassaemia major. Br J Haematol. (2008) 143:548–51. doi: 10.1111/j.1365-2141.2008.07385.x
135. Cutting R, Mirelman A, Vora A. Treosulphan as an alternative to busulphan for myeloablative conditioning in paediatric allogeneic transplantation. Br J Haematol. (2008) 143:748–51. doi: 10.1111/j.1365-2141.2008.07399.x
136. Greystoke B, Bonanomi S, Carr TF, Gharib M, Khalid T, Coussons M, et al. Treosulfan-containing regimens achieve high rates of engraftment associated with low transplant morbidity and mortality in children with non-malignant disease and significant co-morbidities. Br J Haematol. (2008) 142:257–62. doi: 10.1111/j.1365-2141.2008.07064.x
137. Burroughs LM, Nemecek ER, Torgerson TR, Storer BE, Talano JA, Domm J, et al. Treosulfan-based conditioning and hematopoietic cell transplantation for nonmalignant diseases: a prospective multicenter trial. Biol Blood Marrow Transplant. (2014) 20:1996–2003. doi: 10.1016/j.bbmt.2014.08.020
138. Morillo-Gutierrez B, Beier R, Rao K, Burroughs L, Schulz A, Ewins AM, et al. Treosulfan-based conditioning for allogeneic HSCT in children with chronic granulomatous disease: a multicenter experience. Blood. (2016) 128:440–8. doi: 10.1182/blood-2016-03-704015
139. Slatter MA, Rao K, Abd Hamid IJ, Nademi Z, Chiesa R, Elfeky R, et al. Treosulfan and fludarabine conditioning for hematopoietic stem cell transplantation in children with primary immunodeficiency: UK experience. Biol Blood Marrow Transplant. (2018) 24:529–36. doi: 10.1016/j.bbmt.2017.11.009
140. Mathews V, George B, Viswabandya A, Abraham A, Ahmed R, Ganapule A, et al. Improved clinical outcomes of high risk beta thalassemia major patients undergoing a HLA matched related allogeneic stem cell transplant with a treosulfan based conditioning regimen and peripheral blood stem cell grafts. PLoS ONE. (2013) 8:e61637. doi: 10.1371/journal.pone.0061637
141. Faraci M, Diesch T, Labopin M, Dalissier A, Lankester A, Gennery A, et al. Gonadal function after busulfan compared with treosulfan in children and adolescents undergoing allogeneic hematopoietic stem cell transplant. Biol Blood Marrow Transplant. (2019) 25:1786–91. doi: 10.1016/j.bbmt.2019.05.005
142. Wachowiak J, Sykora KW, Cornish J, Chybicka A, Kowalczyk JR, Gorczynska E, et al. Treosulfan-based preparative regimens for allo-HSCT in childhood hematological malignancies: a retrospective study on behalf of the EBMT pediatric diseases working party. Bone Marrow Transplant. (2011) 46:1510–8. doi: 10.1038/bmt.2010.343
143. Beier R, Schulz A, Honig M, Eyrich M, Schlegel PG, Holter W, et al. Long-term follow-up of children conditioned with Treosulfan: German and Austrian experience. Bone Marrow Transplant. (2013) 48:491–501. doi: 10.1038/bmt.2012.188
144. Boztug H, Sykora KW, Slatter M, Zecca M, Veys P, Lankester A, et al. European society for blood and marrow transplantation analysis of treosulfan conditioning before hematopoietic stem cell transplantation in children and adolescents with hematological malignancies. Pediatr Blood Cancer. (2016) 63:139–48. doi: 10.1002/pbc.25764
145. Kalwak K, Mielcarek M, Patrick K, Styczynski J, Bader P, Corbacioglu S, et al. Treosulfan-fludarabine-thiotepa-based conditioning treatment before allogeneic hematopoietic stem cell transplantation for pediatric patients with hematological malignancies. Bone Marrow Transplant. (2020) 55:1996–2007. doi: 10.1038/s41409-020-0869-6
146. Nemecek ER, Hilger RA, Adams A, Shaw BE, Kiefer D, Le-Rademacher J, et al. Treosulfan, fludarabine, and low-dose total body irradiation for children and young adults with acute myeloid leukemia or myelodysplastic syndrome undergoing allogeneic hematopoietic cell transplantation: prospective phase II trial of the pediatric blood and marrow transplant consortium. Biol Blood Marrow Transplant. (2018) 24:1651–6. doi: 10.1016/j.bbmt.2018.04.025
147. Shimoni A, Labopin M, Savani B, Hamladji RM, Beelen D, Mufti G, et al. Intravenous busulfan compared with treosulfan-based conditioning for allogeneic stem cell transplantation in acute myeloid leukemia: a study on behalf of the acute leukemia working party of European society for blood and marrow transplantation. Biol Blood Marrow Transplant. (2018) 24:751–7. doi: 10.1016/j.bbmt.2017.12.776
148. Nagler A, Labopin M, Beelen D, Ciceri F, Volin L, Shimoni A, et al. Long-term outcome after a treosulfan-based conditioning regimen for patients with acute myeloid leukemia: a report from the Acute Leukemia Working Party of the European Society for Blood and Marrow Transplantation. Cancer. (2017) 123:2671–9. doi: 10.1002/cncr.30646
149. van der Stoep M, Bertaina A, Ten Brink MH, Bredius RG, Smiers FJ, Wanders DCM, et al. High interpatient variability of treosulfan exposure is associated with early toxicity in paediatric HSCT: a prospective multicentre study. Br J Haematol. (2017) 179:772–80. doi: 10.1111/bjh.14960
150. Chiesa R, Standing JF, Winter R, Nademi Z, Chu J, Pinner D, et al. Proposed therapeutic range of treosulfan in reduced toxicity pediatric allogeneic hematopoietic stem cell transplant conditioning: results from a prospective trial. Clin Pharmacol Ther. (2020) 108:264–73. doi: 10.1002/cpt.1715
151. Mohanan E, Panetta JC, Lakshmi KM, Edison ES, Korula A, Na F, et al. Pharmacokinetics and pharmacodynamics of treosulfan in patients with thalassemia major undergoing allogeneic hematopoietic stem cell transplantation. Clin Pharmacol Ther. (2018) 104:575–83. doi: 10.1002/cpt.988
152. van der Stoep M, Zwaveling J, Bertaina A, Locatelli F, Guchelaar HJ, Lankester AC, et al. Population pharmacokinetics of treosulfan in paediatric patients undergoing hematopoietic stem cell transplantation. Br J Clin Pharmacol. (2019) 85:2033–44. doi: 10.1111/bcp.13995
153. ten Brink MH, Zwaveling J, Swen JJ, Bredius RG, Lankester AC, Guchelaar HJ. Personalized busulfan and treosulfan conditioning for pediatric stem cell transplantation: the role of pharmacogenetics and pharmacokinetics. Drug Discov Today. (2014) 19:1572–86. doi: 10.1016/j.drudis.2014.04.005
154. Danielak D, Twardosz J, Kasprzyk A, Wachowiak J, Kalwak K, Glowka F. Population pharmacokinetics of treosulfan and development of a limited sampling strategy in children prior to hematopoietic stem cell transplantation. Eur J Clin Pharmacol. (2018) 74:79–89. doi: 10.1007/s00228-017-2344-x
155. Curran MP, Perry CM. Clofarabine: in pediatric patients with acute lymphoblastic leukemia. Paediatr Drugs. (2005) 7:259–64. doi: 10.2165/00148581-200507040-00005
156. Jeha S, Gandhi V, Chan KW, McDonald L, Ramirez I, Madden R, et al. Clofarabine, a novel nucleoside analog, is active in pediatric patients with advanced leukemia. Blood. (2004) 103:784–9. doi: 10.1182/blood-2003-06-2122
157. Jeha S, Gaynon PS, Razzouk BI, Franklin J, Kadota R, Shen V, et al. Phase II study of clofarabine in pediatric patients with refractory or relapsed acute lymphoblastic leukemia. J Clin Oncol. (2006) 24:1917–23. doi: 10.1200/JCO.2005.03.8554
158. Hijiya N, Thomson B, Isakoff MS, Silverman LB, Steinherz PG, Borowitz MJ, et al. Phase 2 trial of clofarabine in combination with etoposide and cyclophosphamide in pediatric patients with refractory or relapsed acute lymphoblastic leukemia. Blood. (2011) 118:6043–9. doi: 10.1182/blood-2011-08-374710
159. O'Connor D, Sibson K, Caswell M, Connor P, Cummins M, Mitchell C, et al. Early UK experience in the use of clofarabine in the treatment of relapsed and refractory paediatric acute lymphoblastic leukaemia. Br J Haematol. (2011) 154:482–5. doi: 10.1111/j.1365-2141.2011.08752.x
160. Locatelli F, Testi AM, Bernardo ME, Rizzari C, Bertaina A, Merli P, et al. Clofarabine, cyclophosphamide and etoposide as single-course re-induction therapy for children with refractory/multiple relapsed acute lymphoblastic leukaemia. Br J Haematol. (2009) 147:371–8. doi: 10.1111/j.1365-2141.2009.07882.x
161. Andersson BS, de Lima M, Thall PF, Wang X, Couriel D, Korbling M, et al. Once daily iv busulfan and fludarabine (iv Bu-Flu) compares favorably with iv busulfan and cyclophosphamide (iv BuCy2) as pretransplant conditioning therapy in AML/MDS. Biol Blood Marrow Transplant. (2008) 14:672–84. doi: 10.1016/j.bbmt.2008.03.009
162. Andersson BS, Valdez BC, de Lima M, Wang X, Thall PF, Worth LL, et al. Clofarabine +/- fludarabine with once daily iv busulfan as pretransplant conditioning therapy for advanced myeloid leukemia and MDS. Biol Blood Marrow Transplant. (2011) 17:893–900. doi: 10.1016/j.bbmt.2010.09.022
163. Alatrash G, Thall PF, Valdez BC, Fox PS, Ning J, Garber HR, et al. Long-term outcomes after treatment with clofarabine +/- fludarabine with once-daily intravenous busulfan as pretransplant conditioning therapy for advanced myeloid leukemia and myelodysplastic syndrome. Biol Blood Marrow Transplant. (2016) 22:1792–800. doi: 10.1016/j.bbmt.2016.06.023
164. Kebriaei P, Basset R, Ledesma C, Ciurea S, Parmar S, Shpall EJ, et al. Clofarabine combined with busulfan provides excellent disease control in adult patients with acute lymphoblastic leukemia undergoing allogeneic hematopoietic stem cell transplantation. Biol Blood Marrow Transplant. (2012) 18:1819–26. doi: 10.1016/j.bbmt.2012.06.010
165. Kebriaei P, Bassett R, Lyons G, Valdez B, Ledesma C, Rondon G, et al. Clofarabine plus busulfan is an effective conditioning regimen for allogeneic hematopoietic stem cell transplantation in patients with acute lymphoblastic leukemia: long-term study results. Biol Blood Marrow Transplant. (2017) 23:285–92. doi: 10.1016/j.bbmt.2016.11.001
166. Versluys AB, Boelens JJ, Pronk C, Lankester A, Bordon V, Buechner J, et al. Hematopoietic cell transplant in pediatric acute myeloid leukemia after similar upfront therapy; a comparison of conditioning regimens. Bone Marrow Transplant. (2021) 56:1426–32. doi: 10.1038/s41409-020-01201-w
167. Tesfaye H, Branova R, Klapkova E, Prusa R, Janeckova D, Riha P, et al. The importance of therapeutic drug monitoring (TDM) for parenteral busulfan dosing in conditioning regimen for hematopoietic stem cell transplantation (HSCT) in children. Ann Transplant. (2014) 19:214–24. doi: 10.12659/AOT.889933
168. Ben-Barouch S, Cohen O, Vidal L, Avivi I, Ram R. Busulfan fludarabine vs. busulfan cyclophosphamide as a preparative regimen before allogeneic hematopoietic cell transplantation: systematic review and meta-analysis. Bone Marrow Transplant. (2016) 51:232–40. doi: 10.1038/bmt.2015.238
169. Bartelink IH, van Reij EML, Gerhardt CE, van Maarseveen EM, de Wildt A, Versluys B, et al. Fludarabine and exposure-targeted busulfan compares favorably with busulfan/cyclophosphamide-based regimens in pediatric hematopoietic cell transplantation: maintaining efficacy with less toxicity. Biol Blood Marrow Transplant. (2014) 20:345–53. doi: 10.1016/j.bbmt.2013.11.027
170. Harris AC, Boelens JJ, Ahn KW, Fei M, Abraham A, Artz A, et al. Comparison of pediatric allogeneic transplant outcomes using myeloablative busulfan with cyclophosphamide or fludarabine. Blood Advances. (2018) 2:1198–206. doi: 10.1182/bloodadvances.2018016956
171. Ishiwata Y, Nagata M, Tsuge K, Takahashi H, Suzuki S, Imai K, et al. Population pharmacokinetics of intravenous busulfan in Japanese pediatric patients with primary immunodeficiency diseases. J Clin Pharmacol. (2018) 58:327–31. doi: 10.1002/jcph.1027
172. Yeh RF, Pawlikowski MA, Blough DK, McDonald GB, O'Donnell PV, Rezvani A, et al. Accurate targeting of daily intravenous busulfan with 8-hour blood sampling in hospitalized adult hematopoietic cell transplant recipients. Biol Blood Marrow Transplant. (2012) 18:265–72. doi: 10.1016/j.bbmt.2011.06.013
173. Long-Boyle JR, Green KG, Brunstein CG, Cao Q, Rogosheske J, Weisdorf DJ, et al. High fludarabine exposure and relationship with treatment-related mortality after nonmyeloablative hematopoietic cell transplantation. Bone Marrow Transplant. (2011) 46:20–6. doi: 10.1038/bmt.2010.53
174. Ivaturi V, Dvorak CC, Chan D, Liu T, Cowan MJ, Wahlstrom J, et al. Pharmacokinetics and model-based dosing to optimize fludarabine therapy in pediatric hematopoietic cell transplant recipients. Biol Blood Marrow Transplant. (2017) 23:1701–13. doi: 10.1016/j.bbmt.2017.06.021
175. Chung H, Hong KT, Lee JW, Rhee SJ, Kim S, Yoon SH, et al. Pharmacokinetics of fludarabine and its association with clinical outcomes in paediatric haematopoietic stem cell transplantation patients. Bone Marrow Transplant. (2019) 54:284–92. doi: 10.1038/s41409-018-0260-z
176. Langenhorst JB, van Kesteren C, van Maarseveen EM, Dorlo TPC, Nierkens S, Lindemans CA, et al. Fludarabine exposure in the conditioning prior to allogeneic hematopoietic cell transplantation predicts outcomes. Blood Adv. (2019) 3:2179–87. doi: 10.1182/bloodadvances.2018029421
177. Saraceni F, Beohou E, Labopin M, Arcese W, Bonifazi F, Stepensky P, et al. Thiotepa, busulfan and fludarabine compared to busulfan and cyclophosphamide as conditioning regimen for allogeneic stem cell transplant from matched siblings and unrelated donors for acute myeloid leukemia. Am J Hematol. (2018) 93:1211–9. doi: 10.1002/ajh.25225
178. Saraceni F, Labopin M, Hamladji R-M, Mufti G, Socié G, Shimoni A, et al. Thiotepa-busulfan-fludarabine compared to busulfan-fludarabine for sibling and unrelated donor transplant in acute myeloid leukemia in first remission. Oncotarget. (2018) 9:3379–93. doi: 10.18632/oncotarget.23273
179. Sanz J, Boluda JC, Martin C, Gonzalez M, Ferra C, Serrano D, et al. Single-unit umbilical cord blood transplantation from unrelated donors in patients with hematological malignancy using busulfan, thiotepa, fludarabine and ATG as myeloablative conditioning regimen. Bone Marrow Transplant. (2012) 47:1287–93. doi: 10.1038/bmt.2012.13
180. Giannotti F, Labopin M, Shouval R, Sanz J, Arcese W, Angelucci E, et al. Haploidentical transplantation is associated with better overall survival when compared to single cord blood transplantation: an EBMT-Eurocord study of acute leukemia patients conditioned with thiotepa, busulfan, and fludarabine. J Hematol Oncol. (2018) 11:110. doi: 10.1186/s13045-018-0655-8
181. Ruggeri A, Sanz G, Bittencourt H, Sanz J, Rambaldi A, Volt F, et al. Comparison of outcomes after single or double cord blood transplantation in adults with acute leukemia using different types of myeloablative conditioning regimen, a retrospective study on behalf of Eurocord and the Acute Leukemia Working Party of EBMT. Leukemia. (2014) 28:779–86. doi: 10.1038/leu.2013.259
182. Di Bartolomeo P, Santarone S, De Angelis G, Picardi A, Cudillo L, Cerretti R, et al. Haploidentical, unmanipulated, G-CSF-primed bone marrow transplantation for patients with high-risk hematologic malignancies. Blood. (2013) 121:849–57. doi: 10.1182/blood-2012-08-453399
183. Fox ML, García-Cadenas I, Pérez AM, Villacampa G, Piñana JL, Ortí G, et al. Feasibility of thiotepa addition to the fludarabine-busulfan conditioning with tacrolimus/sirolimus as graft vs. host disease prophylaxis. Leuk Lymphoma. (2020) 61:1823–32. doi: 10.1080/10428194.2020.1788015
184. Saad AA, Sharma M, Higa GM. Treatment of multiple myeloma in the targeted therapy era. Ann Pharmacother. (2009) 43:329–38. doi: 10.1345/aph.1L428
185. Horwitz ME, Morris A, Gasparetto C, Sullivan K, Long G, Chute J, et al. Myeloablative intravenous busulfan/fludarabine conditioning does not facilitate reliable engraftment of dual umbilical cord blood grafts in adult recipients. Biol Blood Marrow Transplantat. (2008) 14:591–4. doi: 10.1016/j.bbmt.2008.02.016
Keywords: acute lymphoblastic leukaemia (ALL), hematopoietic stem cell transplant (HSCT), chemotherapy, pharmacokinetics, pharmacogenetics, pharmacodynamics (PD)
Citation: Ben Hassine K, Powys M, Svec P, Pozdechova M, Versluys B, Ansari M and Shaw PJ (2021) Total Body Irradiation Forever? Optimising Chemotherapeutic Options for Irradiation-Free Conditioning for Paediatric Acute Lymphoblastic Leukaemia. Front. Pediatr. 9:775485. doi: 10.3389/fped.2021.775485
Received: 14 September 2021; Accepted: 21 October 2021;
Published: 10 December 2021.
Edited by:
Christina Peters, St. Anna Children's Cancer Research Institute (CCRI), AustriaReviewed by:
Mary Slatter, Newcastle University, United KingdomJerry Stein, Schneider Children's Medical Center, Israel
Copyright © 2021 Ben Hassine, Powys, Svec, Pozdechova, Versluys, Ansari and Shaw. This is an open-access article distributed under the terms of the Creative Commons Attribution License (CC BY). The use, distribution or reproduction in other forums is permitted, provided the original author(s) and the copyright owner(s) are credited and that the original publication in this journal is cited, in accordance with accepted academic practice. No use, distribution or reproduction is permitted which does not comply with these terms.
*Correspondence: Peter J. Shaw, peter.shaw@health.nsw.gov.au
†These authors share first authorship
‡These authors share last authorship