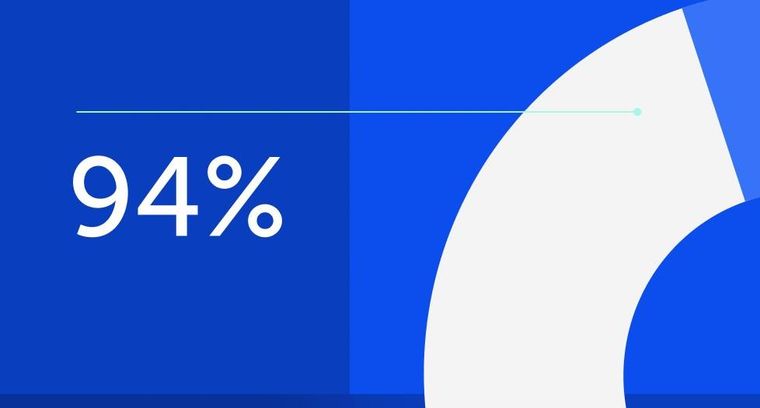
94% of researchers rate our articles as excellent or good
Learn more about the work of our research integrity team to safeguard the quality of each article we publish.
Find out more
MINI REVIEW article
Front. Pediatr., 29 October 2021
Sec. Neonatology
Volume 9 - 2021 | https://doi.org/10.3389/fped.2021.765306
This article is part of the Research TopicTransfusions in the Neonatal PeriodView all 5 articles
Red blood cell (RBC) transfusions are a life-saving intervention, with nearly 14 million RBC units transfused in the United States each year. However, the safety and efficacy of this procedure can be influenced by variations in the collection, processing, and administration of RBCs. Procedures or manipulations that increase potassium (K+) levels in stored blood products can also predispose patients to hyperkalemia and transfusion-associated hyperkalemic cardiac arrest (TAHCA). In this mini review, we aimed to provide a brief overview of blood storage, the red cell storage lesion, and variables that increase extracellular [K+]. We also summarize cases of TAHCA and identify potential mitigation strategies. Hyperkalemia and cardiac arrhythmias can occur in pediatric patients when RBCs are transfused quickly, delivered directly to the heart without time for electrolyte equilibration, or accumulate extracellular K+ due to storage time or irradiation. Advances in blood banking have improved the availability and quality of RBCs, yet, some patient populations are sensitive to transfusion-associated hyperkalemia. Future research studies should further investigate potential mitigation strategies to reduce the risk of TAHCA, which may include using fresh RBCs, reducing storage time after irradiation, transfusing at slower rates, implementing manipulations that wash or remove excess extracellular K+, and implementing restrictive transfusion strategies.
Of the nearly 14 million blood transfusions in the United States each year, over 400,000 of these were administered to pediatric patients (1, 2). A recent multicenter study found that 79% of children in the intensive care unit for cardiac conditions received at least one red blood cell (RBC) transfusion during their stay (3). Neonatal and pediatric patients are physiologically different from adults, and as such, transfusion indications and clinical management can present unique challenges (4, 5). For example, younger patients are more susceptible to electrolyte and metabolic consequences of transfusions (e.g., hyperkalemia, hypocalcemia), since the amount of blood given during surgery or massive transfusion can equal the total blood volume of a neonate, and the glomerular filtration rate of newborns does not reach maturity until near the first year of life (4, 6, 7). Moreover, many children's hospitals follow a dedicated donor strategy, wherein a single-donor unit is split into multiple pediatric packs for use in a designated patient (8–10), up to the date of expiration. This practice reduces the risk of multiple donor exposures, but with the consequence that older RBCs are then used for subsequent transfusions. Additionally, institutions that lack irradiation facilities may procure RBCs that are already irradiated off-site, which prolongs the time from irradiation to transfusion and increases the risk of transfusion-associated hyperkalemia. Accordingly, the collection, processing, and administration of RBC transfusions can influence the safety and efficacy of transfusions and contribute to adverse events (11). Indeed, a recent multicenter study found that nearly 1% of pediatric patients experience transfusion-associated hyperkalemia, which coincides with a 20% 1-day mortality rate (7) – thus, further highlighting the importance of additional study.
RBCs develop storage-induced damage and undergo a series of biochemical, metabolic, and structural changes that are collectively termed “the RBC storage lesion” [previously reviewed (12–14)]. Briefly, prolonged RBC storage results in glucose depletion and a reduction in ATP production, which impairs RBC membrane stability and increases hemolysis. Storage lesion severity can result in downstream physiological consequences that diminish both the safety and efficacy of RBC transfusions (12, 15–18). Accordingly, numerous biomarkers have been identified to monitor the pathobiological changes that red cells undergo during storage, including (but not limited to) measurements of free hemoglobin and non-transferrin bound iron, microvesicle production, 2, 3-diphosphoglyceric acid, lactate, and extracellular [K+] (14, 19–23). Although metabolic processes are slowed during refrigeration, hypothermic storage impairs cation transporters which alters the electrolyte composition of the RBC unit. As an example, Na+/K+ ATPase dysfunction results in K+ leak and accumulation within the extracellular solution of stored RBC units. Extracellular K+ levels increase linearly at a rate of approximately 1 mEq/L each day during refrigerated storage – until an equilibrium point is reached at ~ 60 mEq/L (24). Experimental studies by Bennett-Guerrero et al., reported a 376% increase in extracellular [K+] within 3-weeks of storage, exceeding the maximum level of instrument detection (20 mmol/L) (14). Clinical studies have also observed high [K+] in RBC units stored for longer periods of time, including a case report on the death of an infant after cardiac surgery that involved the rapid transfusion of a 32-day RBC unit with high K+ levels (60 mEq/L) (25, 26). The latter highlights the risk of electrolyte disturbances in transfused patients. Irradiation further exacerbates RBC K+ leak, as increases in oxidative stress and red cell permeability result in a two-fold increase in [K+] following blood product irradiation (27–29). Upon transfusion, an acute spike in serum [K+] can shift the myocardial resting membrane potential and trigger lethal arrhythmias (30). Indeed, transfusion-associated hyperkalemic cardiac arrest (TAHCA) is a recognized transfusion complication (31).
Both the RBC storage lesion and the method used to transfuse RBCs can contribute to transfusion-associated complications. RBC transfusions are administered to neonatal, infant, and pediatric patients via a variety of methods, including: intravenous (IV) catheter, handheld syringe infusion, or extracorporeal circulation (32–34). Conventionally, RBC transfusions are performed using a catheter, of variable bore size, placed peripherally in an accessible vein or via a central line (35). Accordingly, the route of administration can influence electrolyte homeostasis and the safety margin of this procedure. For instance, central lines are more commonly associated with hyperkalemia and cardiac arrest (25, 36). Whereas peripheral IV infusions provide ample time for transfused blood to ionically equilibrate and redistribute K+ with surrounding tissues before reaching the heart (35). Notably, patients with low cardiac output may still present with clinical hyperkalemia even in instances of peripheral venous administration (35, 37).
The flow rate of the transfusion can also influence the likelihood of developing transfusion-associated hyperkalemia and/or TAHCA. Transfusions with rapid flow rates can increase red cell hemolysis (32, 38) and also elevate K+ levels quickly with inadequate time for equilibration. Miller et al., observed that hemolysis increases with handheld syringes, resulting in a serum K+ concentration that exceeds the threshold for arrhythmogenicity (32). While there are guidelines for the maximum transfusion rate in pediatrics, in clinical practice, the rate of infusion is largely determined by the rate of blood loss and indication for transfusion. In the operating room or emergency setting, the use of handheld syringes is a common practice for neonatal and infant transfusions (32, 38), but can introduce considerable variability in the speed of administration compared to automated pumps. Emergency circumstances that require fast rates of transfusion also decrease the allowable time for K+ redistribution, which further increases the risk of TAHCA (37).
Transfusion-associated complications can also occur in pediatric populations following large volume RBC transfusions. Pediatric patients with congenital cardiac malformations often undergo reconstructive surgery that requires the use of extracorporeal membrane oxygenation (ECMO). Although adult patients can usually accommodate the volume of blood required to prime an ECMO circuit, this volume can exceed the total blood volume of a neonatal or infant patient. Consequently, pediatric ECMO patients receive large volumes of RBCs (42–105 ml/kg/day), which increases the risk of circulatory overload and hyperkalemia (34, 39). Parshuram et al., reported a case in which a 3-week old ECMO patient experienced TAHCA within a few min of infusing RBC's irradiated 10 days prior as part of the circuit prime; a blood gas measurement denoted 9 mmol/L [K+] at the time of cardiac arrest (40). Hyperkalemia has also been observed in cardiopulmonary bypass (CPB) procedures, especially when irradiated blood products are used in smaller patients (<5kg) (41, 42). Indeed, a single RBC transfusion can double an infant's plasma [K+] (26, 43). Further, both ECMO and CPB circuits place additional mechanical stress on circulating RBCs from the tubing, connectors, cannulas, and/or roller pump. This mechanical stress can alter the membrane properties of RBCs, by increasing red cell fragility and hemolysis (44–47). A study by the Naval Blood Research Laboratory found that 15% of red cells were damaged within 24-h of extracorporeal circulation (47). Saline washing of RBC's is yet another form of mechanical stress that has been shown to increase hemolysis and osmotic fragility (48). Accordingly, fresh RBCs are preferred for neonatal and infant patients undergoing cardiac surgery, ECMO, or an exchange transfusion (49–51).
Blood transfusions are life-saving procedures; nevertheless, RBC transfusions are associated with a wide range of complications. The latter includes increased postoperative complications and infections, impaired postoperative recovery, longer hospital stay, and increased morbidity following cardiac surgical procedures (52–60). As previously discussed, RBC transfusions can also precipitate hyperkalemia (26), as the extracellular solution of stored RBC units can reach 40–70 mM [K+] (19, 25). Indeed, more than 75% of critically ill (adult) patients and 18–23% of trauma (pediatric) patients experience elevated serum K+ following RBC transfusion (61, 62). Upon transfusion, high K+ levels can shift the myocardial resting membrane potential and precipitate adverse electrophysiological outcomes, from a short-lasting atrial flutter to protracted ventricular fibrillation (30, 63). An acute increase in serum [K+] can delay electrical conduction in the atrioventricular node, His bundle, and Purkinje system, which manifests as a prolonged PR interval and widened QRS complex (64). Hyperkalemia also predisposes the heart to pathological conditions, including ventricular tachycardia, ventricular fibrillation, atrioventricular block, and asystole (30, 63, 64). In some cases, cardiac electrical instabilities may be detectable in electrocardiogram recordings (65, 66) before the onset of severe events (Figure 1), such as cardiac arrest that require immediate intervention (25, 67). As highlighted above, risk factors for TAHCA may include the volume and rate of RBC transfusion, storage age of blood products, and irradiation of red cells – although the perceived risk of TAHCA remains debated (68).
Figure 1. Transfusion-associated hyperkalemia can precipitate cardiac electrical disturbances, which can present with peaked T-waves in the electrocardiogram. Further increase in serum [K+] can result in cardiac arrest. Graphic generated in BioRender. ECG traces kindly provided by Dr. Elizabeth Sherwin.
Recently, our laboratory conducted the first experimental study to show a direct correlation between red cell storage duration, hyperkalemia, and cardiac electrophysiology (69). Using intact heart preparations and human induced pluripotent stem cell-derived cardiomyocytes, we reported that RBC storage age was associated with increased extracellular [K+], reduced cardiac automaticity, and slowed electrical conduction. Notably, cardiac electrophysiology parameters remained stable following exposure to fresh RBC units, but older products precipitated bradycardia, impaired sinus node function, and delayed atrioventricular conduction. The latter suggests that the storage age of blood products may be a modifiable risk factor for hyperkalemic cardiac arrest and warrants further investigation.
Although TAHCA is an adverse event that is anticipated by anesthesiologists, it can still prove difficult to manage. In a simulation study (70), only one-fourth of anesthesia residents suspected hyperkalemia as an underlying cause of pulseless electrical activity, and only one-third of residents correctly resuscitated patients using the correct pediatric dose of epinephrine. Further, a separate case series found that serum [K+] during or immediately after cardiac arrest ranged from 5.9–9.2 mEq/L and that TAHCA had poor in-hospital survival of only 12.5% (71). To improve patient outcomes, it is important to examine and understand how the composition of transfused blood product(s), the method of transfusion administration, and patient demographics (31, 72) can increase susceptibility to TAHCA. Unfortunately, transfusion recipients often have several comorbidities, which may result in underreporting of transfusion-associated adverse events (73).
The prevalence of transfusion-associated hyperkalemia and/or TAHCA is currently unknown, as reports are limited to case studies and case reports. Nevertheless, hyperkalemia from blood transfusion is the second most common cause of perioperative cardiac arrest in neonates, infants, and children as documented by the perioperative cardiac arrest registry, second only to hypovolemia from blood loss (74). In Table 1, we have summarized 24 case studies and reports of TAHCA in neonatal, infant, and pediatric patients – with data reported as indicated in the original article (25, 37, 39, 40, 66, 67, 71, 75–92). We also highlight a case report in which TAHCA was avoided by pre-transfusion K+ filtration (87). Briefly, our literature search identified TAHCA case reports and case series using the following search query: “hyperkalemia or hyperkalemic” AND “transfusion” AND “cardiac arrest” AND “neonate OR neonatal OR infant OR pediatric” in both PubMed and Google Scholar databases. Only reports on patients ≤18 years old were included. Studies were excluded if transfusion-associated hyperkalemia was not explicitly associated with cardiac arrest, or if cardiac arrest was attributed to another condition. A manual search of the cited references was also performed.
Within the identified TAHCA case reports, patient's age ranged from 1 h to 18 years old; with 24 patients <1 year old, and 16 reports of TAHCA in neonatal patients. Roughly half of these studies included transfusions that were carried out during surgical procedures such as cardiac surgery, trauma, spinal surgery, hip surgery, organ transplant, and tracheoesophageal fistula repair. The remaining studies included medical transfusions such as ECMO and exchange transfusion. Fifteen reports included transfusions that were initiated centrally through a venous catheter and thirteen reports included transfusion via a peripheral vein. As observed in Table 1, there was a non-uniform method of reporting lab values between case studies and reports. In the case of Morray et.al., the authors reported that there were three cases of TAHCA, but did not provide any additional details beyond two of the three patients suffering fatal arrhythmias (86).
A number of clinical case reports have documented TAHCA in patients receiving large volume transfusions, although we have also highlighted reports of TAHCA following rapid, small volume transfusions of older and/or irradiated blood products (66, 67, 82, 84, 88, 89, 91). Inoue et al., observed peaked T-waves immediately after administering 10 mL of blood via handheld syringe to a 1.4kg newborn (82). In this specific case, blood was administered via a central venous catheter, which can facilitate the delivery of a higher K+ load to the heart without allowing adequate time for redistribution. Similarly, Taylor et al., reported that an infant developed hyperkalemia and cardiac arrhythmia after administering 5-day old blood in small 10 mL aliquots (91). One possible explanation for developing clinical hyperkalemia after small volume injections is the higher rate of hemolysis when smaller needles are used and/or fast rates of transfusion (32, 93). Other cases included transfused patients who received fresh blood without incident, but later developed TAHCA after the administration of older and/or irradiated RBCs that were stored for >24 h (25, 78, 79, 91). As an example, Hall et al. reported the successful transfusion of 4 units of fresh blood to a 2-week-old male undergoing cardiac surgery without incident; upon transfusion of 60 mL of 32-day old blood delivered via a central line, the patient suddenly developed cardiac arrest and died (25). In this case, the older blood products were administered due to a shortage of fresh units. Similarly, Buntain et al., reported successful transfusion of 1.5 units of RBCs in a craniofacial surgery, but, later the patient developed cardiac arrest following the administration of 60 mL of 22-day old blood that had been irradiated 14-days prior (78). Kim et al., reported a case of a 9-month old patient with severe hypoxia receiving ECMO support without incident for several days (39). In this case, the ECMO circuit was primed with RBCs <24 h post-irradiation. However, after an oxygenator failed, a new ECMO circuit was primed with donor blood that had been stored 3-days post-irradiation. Immediately after restarting ECMO, the patient went into cardiac arrest. ECMO is the equivalent of a massive transfusion for pediatric patients, who are vulnerable to large fluctuations in electrolyte concentrations; moreover, in this clinical case a catheter was inserted directly into the right atrium of the patient, which minimized the diffusion potential of the K+ load. In each of the described clinical cases, prolonged blood storage time (with or without irradiation) may have contributed to the development of TAHCA, as the extracellular [K+] within the RBC unit increases linearly with time and doubles 24-h post-irradiation (27).
We also noted clinical practices to avoid TAHCA using pre-transfusion manipulations. Nakagawa et al., reported the avoidance of a hyperkalemic event by using a K+ adsorption filter (87). In this case, a 10-month old patient was undergoing a liver transplant that required a massive transfusion, which was initiated with an in-line filter to reduce the [K+] from 16.3 to 1.9 mEq/L. Additionally, Sohn et al., demonstrated the applicability of a continuous autotransfusion system (CATS) to reduce the K+ level in donor blood before transfusion (90). In this case, the patient received 1 unit of non-irradiated blood, followed by another unit of blood that was 5-days post-irradiation. The patient went into cardiac arrest shortly after the transfusion. After restoring normal cardiac function, the surgical team utilized CATS to prevent TAHCA during the transfusion of another 26 RBC units. These strategies may be useful in situations where fresh RBC units are in short supply.
Strategies to reduce the incidence of TAHCA include the use of fresh blood products, reducing storage time post-irradiation, slower rates of transfusion, and other manipulation techniques. Current standard of practice dictates that blood units <5 days old and within 24 h of irradiation are employed in massive transfusion (50, 94); although older RBC units are administered if fresh units are not readily available. Fresh RBC units are also preferred for neonatal and pediatric cardiac surgery (95). Fresh whole blood (<48 h) has been used for cardiac surgery at a few select institutions (96), although whole blood is not routinely available to most transfusion services. For children receiving ECMO, consensus panels recommend the use of fresh RBCs within 5-days of collection and irradiated RBCs are used within 24-h (50). This recommendation stems from the lack of evidence-based data required to reach consensus on the safety of older RBCs in the context of critically ill children (34, 97). Finally, blood sparing and blood conservation procedures are recommended to reduce the number and volume of RBCs transfused to neonatal, infant, and pediatric patients (98).
RBC washing with a non-plasma solution is another potential mitigation strategy, which reduces the extracellular [K+] to a physiologically-compatible level (99, 100). This strategy has been adopted in cardiac surgery cases requiring cardiopulmonary bypass. Swindell et al., reported that pre-washing irradiated RBCs reduced [K+] from >20 to 0.8 mmol/L (42). Further, 36% of patients receiving unwashed, irradiated RBCs had a serum [K+] >6 mmol/L during cardiopulmonary bypass compared to 0% of patients receiving pre-washed RBCs. Pre-bypass ultrafiltration can also normalize electrolyte levels and reduce the risk of hyperkalemia. Delaney et al., reported that ultrafiltration significantly reduced [K+] from 10.9 to 6.0 mEq/L in ECMO circuits primed with fresh RBCs (101). Utilization of K+ adsorption filters to normalize electrolyte levels during extracorporeal support is another potential mitigation strategy, as in-line filters can remove >90% of extracellular K+ while maintaining flow rates up to 50 mL/min (81, 87, 102).
Transfusion-associated hyperkalemia is a recognized complication of massive and rapid RBC transfusions in neonates, infants, and children (103). Based on available case reports, clinical hyperkalemia can occur when aged and/or irradiated blood products are transfused, and when blood products are administered via a central line vs. a peripheral site (25, 36, 71). Further, the use of handheld syringes with small bore needles can increase hemolysis at the injection site and is a potential cause of transfusion-associated hyperkalemia in emergency situations (32, 38). Although there is debate around whether transfusion-associated hyperkalemia causes cardiac arrest and increases the incidence of TAHCA, there is no doubt that an increased serum [K+] can exacerbate a precarious physiological state in transfused patients (26, 83). While the prevalence of TAHCA is not known and may be underreported (73); we have highlighted 24 case reports and case series that identify potentially fatal outcomes if appropriate precautions are not taken to remedy electrolyte imbalance. TAHCA is not unique to neonatal and pediatric populations (61, 71), but these patients have increased susceptibility due to their unique physiology. There is a 20% 1-day mortality rate in pediatric patients who experience TAHCA (7), and hyperkalemia after transfusion is the second most common cause of perioperative cardiac arrest in neonates, infants, and children (74). Potential strategies to mitigate the risk of TAHCA include the use of fresh RBCs for pediatric cardiac surgery and ECMO, limiting the duration of storage post-irradiation, washing RBCs and/or pre-bypass filtration to remove extracellular K+, slower rates of transfusion, and the use of specialized pre-transfusion filters to reduce [K+] (42, 81, 87, 93, 101, 102, 104). To abrogate TAHCA in the future, additional studies are warranted to evaluate the safety and efficacy of these mitigation strategies and/or implementation of restrictive transfusion strategies (97, 105). Indeed, restrictive transfusion strategies can also help to mitigate blood supply shortages that have resulted from the COVID-19 pandemic, due in part to fewer blood donations (106).
MB and NP performed a literature search and generated Table 1. MB, NP, NL, and PS drafted and approved the manuscript. All authors contributed to the article and approved the submitted version.
This work was supported by the National Institutes of Health (R01HL139472 to NP), Children's National Heart Institute, Sheikh Zayed Institute for Pediatric Surgical Innovation, and the Children's National Research Institute.
The authors declare that the research was conducted in the absence of any commercial or financial relationships that could be construed as a potential conflict of interest.
All claims expressed in this article are solely those of the authors and do not necessarily represent those of their affiliated organizations, or those of the publisher, the editors and the reviewers. Any product that may be evaluated in this article, or claim that may be made by its manufacturer, is not guaranteed or endorsed by the publisher.
The authors gratefully acknowledge Devon Guerrelli and Dr. Elizabeth Sherwin for assistance with Figure 1.
1. Department of Health and Human Services. The 2011 National Blood Collection and Utilization Survey Report. Department of Health and Human Services (2011)
2. Jones JM, Sapiano MRP, Savinkina AA, Haass KA, Baker ML, Henry RA, et al. Slowing decline in blood collection and transfusion in the United States - 2017. Transfusion. (2020) 60(suppl 2):S1–9. doi: 10.1111/trf.15604
3. Mazine A, Rached-D'Astous S, Ducruet T, Lacroix J, Poirier N. Pediatric acute lung injury and sepsis investigators network. Blood transfusions after pediatric cardiac operations: a north american multicenter prospective study. Ann Thorac Surg. (2015) 100:671–7. doi: 10.1016/j.athoracsur.2015.04.033
4. Barcelona SL, Thompson AA, Coté CJ. Intraoperative pediatric blood transfusion therapy: a review of common issues. Part I: hematologic and physiologic differences from adults; metabolic and infectious risks. Paediatr Anaesth. (2005) 15:716–26. doi: 10.1111/j.1460-9592.2005.01548.x
5. Goel R, Cushing MM, Tobian AARR. Pediatric patient blood management programs: not just transfusing little adults. Transfus Med Rev. (2016) 30:235–41. doi: 10.1016/j.tmrv.2016.07.004
6. Bharadwaj A, Khandelwal M, Bhargava SK. Perioperative neonatal and paediatric blood transfusion. Indian J Anaesth. (2014) 58:652–7. doi: 10.4103/0019-5049.144679
7. Yamada C, Edelson M, Lee A, Saifee NH, Bahar B, Delaney M. Transfusion-associated hyperkalemia in pediatric population: prevalence, risk factors, survival, infusion rate, and RBC unit features. Transfusion. (2021) 61:1093–101. doi: 10.1111/trf.16300
8. Girelli G, Antoncecchi S, Casadei AM, del Vecchio A, Isernia P, Motta M, et al. Recommendations for transfusion therapy in neonatology. Blood Transfus. (2015) 13:484–97. doi: 10.2450/2015.0113-15
9. Jain R, Jarosz CR, Myers TF. Decreasing blood donor exposure in the neonates by using dedicated donor transfusions. Transfus Sci. (1997) 18:199–203. doi: 10.1016/S0955-3886(97)00010-6
10. Arora S, Marwaha N, Dhawan H, Rao KLN. Dedicated donor unit transfusions reduces donor exposure in pediatric surgery patients. Asian J Transfus Sci. (2017) 11:124–30. doi: 10.4103/ajts.AJTS_57_16
11. D'Alessandro A, Culp-Hill R, Reisz JA, Anderson M, Fu X, Nemkov T, et al. Heterogeneity of blood processing and storage additives in different centers impacts stored red blood cell metabolism as much as storage time: lessons from REDS-III-Omics. Transfusion. (2019) 59:89–100. doi: 10.1111/trf.14979
12. Yoshida T, Prudent M, D'alessandro A. Red blood cell storage lesion: causes and potential clinical consequences. Blood Transfus. (2019) 17:27–52. doi: 10.2450/2019.0217-18
13. Hess J. Red cell changes during storage. Transfus Apher Sci. (2010) 43:51–9. doi: 10.1016/j.transci.2010.05.009
14. Bennett-Guerrero E, Veldman TH, Doctor A, Telen MJ, Ortel TL, Reid TS, et al. Evolution of adverse changes in stored RBCs. Proc Natl Acad Sci. (2007) 104:17063–8. doi: 10.1073/pnas.0708160104
15. Glynn SA, Klein HG, Ness PM. The red blood cell storage lesion: the end of the beginning. Transfusion. (2016) 56:1462–8. doi: 10.1111/trf.13609
16. Donadee C, Raat NJH, Kanias T, Tejero J, Lee JS, Kelley EE, et al. Nitric oxide scavenging by red blood cell microparticles and cell-free hemoglobin as a mechanism for the red cell storage lesion. Circulation. (2011) 124:465–76. doi: 10.1161/CIRCULATIONAHA.110.008698
17. D'Alessandro A, Reisz JA, Zhang Y, Gehrke S, Alexander K, Kanias T, et al. Effects of aged stored autologous red blood cells on human plasma metabolome. Blood Adv. (2019) 3:884–96. doi: 10.1182/bloodadvances.2018029629
18. Koch CG, Li L, Sessler DI, Figueroa P, Hoeltge GA, Mihaljevic T, et al. Duration of red-cell storage and complications after cardiac surgery. N Engl J Med. (2008) 358:1229–39. doi: 10.1056/NEJMoa070403
19. Wallas CH. Sodium and potassium changes in blood bank stored human erythrocytes. Transfusion. (1979) 19:210–5. doi: 10.1046/j.1537-2995.1979.19279160297.x
20. D'Alessandro A, Kriebardis AG, Rinalducci S, Antonelou MH, Hansen KC, Papassideri IS, et al. An update on red blood cell storage lesions, as gleaned through biochemistry and omics technologies. Transfusion. (2015) 55:205–19. doi: 10.1111/trf.12804
21. Nguyen DB, Thuy Ly TB, Wesseling MC, Hittinger M, Torge A, Devitt A, et al. Characterization of microvesicles released from human red blood cells. Cell Physiol Biochem. (2016) 38:1085–99. doi: 10.1159/000443059
22. Rapido F, Brittenham GM, Bandyopadhyay S, La Carpia F, L'Acqua C, McMahon DJ, et al. Prolonged red cell storage before transfusion increases extravascular hemolysis. J Clin Invest. (2017) 127:375–82. doi: 10.1172/JCI90837
23. Hod EA, Zhang N, Sokol SA, Wojczyk BS, Francis RO, Ansaldi D, et al. Transfusion of red blood cells after prolonged storage produces harmful effects that are mediated by iron and inflammation. Blood. (2010) 115:4284–92. doi: 10.1182/blood-2009-10-245001
24. Zimrin AB, Hess JR. Current issues relating to the transfusion of stored red blood cells. Vox Sang. (2009) 96:93–103. doi: 10.1111/j.1423-0410.2008.01117.x
25. Hall TL, Barnes A, Miller JR, Bethencourt DM, Nestor L. Neonatal mortality following transfusion of red cells with high plasma potassium levels. Transfusion. (1993) 33:606–9. doi: 10.1046/j.1537-2995.1993.33793325059.x
26. Vraets A, Lin Y, Callum JL. Transfusion-associated hyperkalemia. Transfus Med Rev. (2011) 25:184–96. doi: 10.1016/j.tmrv.2011.01.006
27. Winter KM, Johnson L, Kwok M, Reid S, Alarimi Z, Wong JKL, et al. Understanding the effects of gamma-irradiation on potassium levels in red cell concentrates stored in SAG-M for neonatal red cell transfusion. Vox Sang. (2015) 108:141–50. doi: 10.1111/vox.12194
28. Serrano K, Chen D, Hansen AL, Levin E, Turner TR, Kurach JDR, et al. The effect of timing of gamma-irradiation on hemolysis and potassium release in leukoreduced red cell concentrates stored in SAGM. Vox Sang. (2014) 106:379–81. doi: 10.1111/vox.12112
29. Davey RJ, McCoy NC, Yu M, Sullivan JA, Spiegel DM, Leitman SF. The effect of prestorage irradiation on posttransfusion red cell survival. Transfusion. (1992) 32:525–8. doi: 10.1046/j.1537-2995.1992.32692367195.x
30. Weiss JN, Qu Z, Shivkumar K. Electrophysiology of hypokalemia and hyperkalemia. Circ Arrhythm Electrophysiol. (2017) 10:e004667. doi: 10.1161/CIRCEP.116.004667
31. Lee AC, Reduque LL, Luban NLC, Ness PM, Anton B, Heitmiller ES. Transfusion-associated hyperkalemic cardiac arrest in pediatric patients receiving massive transfusion. Transfusion. (2014) 54:244–54. doi: 10.1111/trf.12192
32. Miller MA, Schlueter AJ. Transfusions via hand-held syringes and small-gauge needles as risk factors for hyperkalemia. Transfusion. (2004) 44:373–81. doi: 10.1111/j.1537-2995.2004.00653.x
33. Goel R, Josephson CD. Recent advances in transfusions in neonates/infants. Research. (2018) 7:F1000. doi: 10.12688/f1000research.13979.1
34. Bembea MM, Cheifetz IM, Fortenberry J, Bunchman T, Valentine S, Bateman S, et al. Recommendations on the indications for red blood cell transfusion for the critically ill child receiving support from extracorporeal membrane oxygenation, ventricular assist, and renal replacement therapy devices from the pediatric critical care transfusi. Pediatr Crit Care Med. (2018) 19(9S Suppl 1):S157–62. doi: 10.1097/PCC.0000000000001600
35. Sloan SR. Neonatal transfusion review. Pediatr Anesth. (2011) 21:25–30. doi: 10.1111/j.1460-9592.2010.03458.x
36. Carvalho B, Quiney NF. ‘Near-miss' hyperkalaemic cardiac arrest associated with rapid blood transfusion. Anaesthesia. (1999) 54:1094–6. doi: 10.1046/j.1365-2044.1999.01109.x
37. Brown KA, Bissonnette B, McIntyre B. Hyperkalaemia during rapid blood transfusion and hypovolaemic cardiac arrest in children. Can J Anaesth. (1990) 37:747–54. doi: 10.1007/BF03006533
38. de Villiers WL, Murray AA, Levin AI. Expediting red blood cell transfusions by syringing causes significant hemolysis. Transfusion. (2017) 57:2747–51. doi: 10.1111/trf.14283
39. Kim DW, Cheon KR, Cho D, Lee KS, Cho HJ, Jeong IS. Transfusion associated hyperkalemia and cardiac arrest in an infant after extracorporeal membrane oxygenation. Korean J Crit Care Med. (2015) 30:132–4. doi: 10.4266/kjccm.2015.30.2.132
40. Parshuram CS, Cox PN. Neonatal hyperkalemic-hypocalcemic cardiac arrest associated with initiation of blood-primed continuous venovenous hemofiltration. Pediatr Crit Care Med. (2002) 3:67–9. doi: 10.1097/00130478-200201000-00015
41. Vohra HA, Adluri K, Willets R, Horsburgh A, Barron DJ, Brawn WJ, et al. Changes in potassium concentration and haematocrit associated with cardiopulmonary bypass in paediatric cardiac surgery. Perfusion. (2007) 22:87–92. doi: 10.1177/0267659107077951
42. Swindell CG, Barker TA, McGuirk SP, Jones TJ, Barron DJ, Brawn WJ, et al. Washing of irradiated red blood cells prevents hyperkalaemia during cardiopulmonary bypass in neonates and infants undergoing surgery for complex congenital heart disease. Eur J Cardio-Thoracic Surg. (2007) 31:659–64. doi: 10.1016/j.ejcts.2007.01.014
43. Ibrahim R, Mohamed D, Abdelghaffar S, Mansi Y. Red blood transfusion in preterm infants: changes in glucose, electrolytes and acid base balance. Asian J Transfus Sci. (2012) 6:36. doi: 10.4103/0973-6247.95049
44. Vercaemst L. Hemolysis in cardiac surgery patients undergoing cardiopulmonary bypass: a review in search of a treatment algorithm. J Extra Corpor Technol. (2008) 40:257–67.
45. Kameneva M V, Ündar A, Antaki JF, Watach MJ, Calhoon JH, Borovetz HS. Decrease in red blood cell deformability caused by hypothermia, hemodilution, and mechanical stress: factors related to cardiopulmonary bypass. ASAIO J. (1999) 45:307–10. doi: 10.1097/00002480-199907000-00010
46. Watanabe N, Arakawa Y, Sou A, Kataoka H, Ohuchi K, Fujimoto T, et al. Deformability of human red blood cells exposed to a uniform shear stress as measured by a cyclically reversing shear flow generator. Physiol Meas. (2007) 28:531–45. doi: 10.1088/0967-3334/28/5/007
47. Valeri C, Macgregor H, Ragno G, Healey N, Fonger J, Khuri SF. Effects of centrifugal and roller pumps on survival of autologous red cells in cardiopulmonary bypass surgery. Perfusion. (2006) 21:291–6. doi: 10.1177/0267659106073976
48. Masalunga C, Cruz M, Porter B, Roseff S, Chui B, Mainali E. Increased hemolysis from saline pre-washing RBCs or centrifugal pumps in neonatal ECMO. J Perinatol. (2007) 27:380–4. doi: 10.1038/sj.jp.7211748
49. Redlin M, Habazettl H, Schoenfeld H, Kukucka M, Boettcher W, Kuppe H, et al. Red blood cell storage duration is associated with various clinical outcomes in pediatric cardiac surgery. Transfus Med Hemotherapy. (2014) 41:146–51. doi: 10.1159/000357998
50. Boulton F. Transfusion guidelines for neonates and older children. Br J Haematol. (2004) 124:433–53. doi: 10.1111/j.1365-2141.2004.04815.x
51. Keidan I, Amir G, Mandel M, Mishali D. The metabolic effects of fresh versus old stored blood in the priming of cardiopulmonary bypass solution for pediatric patients. J Thorac Cardiovasc Surg. (2004) 127:949–52. doi: 10.1016/S0022-5223(03)01316-3
52. Iyengar A, Scipione CN, Sheth P, Ohye RG, Riegger L, Bove EL, et al. Association of complications with blood transfusions in pediatric cardiac surgery patients. Ann Thorac Surg. (2013) 96:910–6. doi: 10.1016/j.athoracsur.2013.05.003
53. Redlin M, Boettcher W, Kukucka M, Kuppe H, Habazettl H. Blood transfusion during versus after cardiopulmonary bypass is associated with postoperative morbidity in neonates undergoing cardiac surgery. Perfusion. (2014) 29:327–32. doi: 10.1177/0267659113517922
54. Kipps AK, Wypij D, Thiagarajan RR, Bacha EA, Newburger JW. Blood transfusion is associated with prolonged duration of mechanical ventilation in infants undergoing reparative cardiac surgery. Pediatr Crit Care Med. (2011) 12:52–6. doi: 10.1097/PCC.0b013e3181e30d43
55. Colognesi C, Maia R, Hajjar L, Galas F. Red blood transfusion is a predictor of poor outcome in pediatric cardiac surgery. Crit Care. (2012) 16:P443. doi: 10.1186/cc11050
56. Murphy GJ, Reeves BC, Rogers CA, Rizvi SIA, Culliford L, Angelini GD. Increased mortality, postoperative morbidity, and cost after red blood cell transfusion in patients having cardiac surgery. Circulation. (2007) 116:2544–52. doi: 10.1161/CIRCULATIONAHA.107.698977
57. Koch CG, Li L, Duncan AI, Mihaljevic T, Cosgrove DM, Loop FD, et al. Morbidity and mortality risk associated with red blood cell and blood-component transfusion in isolated coronary artery bypass grafting*. Crit Care Med. (2006) 34:1608–16. doi: 10.1097/01.CCM.0000217920.48559.D8
58. Ranucci M, Carlucci C, Isgrò G, Boncilli A, de Benedetti D, de la Torre T, et al. Duration of red blood cell storage and outcomes in pediatric cardiac surgery: an association found for pump prime blood. Crit Care. (2009) 13:R207. doi: 10.1186/cc8217
59. Cholette JM, Pietropaoli AP, Henrichs KF, Alfieris GM, Powers KS, Phipps R, et al. Longer RBC storage duration is associated with increased postoperative infections in pediatric cardiac surgery. Pediatr Crit Care Med. (2015) 16:227–35. doi: 10.1097/PCC.0000000000000320
60. Savinkina AA, Haass KA, Sapiano MRP, Henry RA, Berger JJ, Basavaraju S V, et al. Transfusion-associated adverse events and implementation of blood safety measures - findings from the 2017 national blood collection and utilization survey. Transfusion. (2020) 60:S10–6. doi: 10.1111/trf.15654
61. Raza S, Ali Baig M, Chang C, Dabas R, Akhtar M, Khan A, et al. A prospective study on red blood cell transfusion related hyperkalemia in critically ill Patients. J Clin Med Res. (2015) 7:417–21. doi: 10.14740/jocmr2123w
62. Livingston MH, Singh S, Merritt NH. Massive transfusion in paediatric and adolescent trauma patients: incidence, patient profile, and outcomes prior to a massive transfusion protocol. Injury. (2014) 45:1301–6. doi: 10.1016/j.injury.2014.05.033
63. Montford JR, Linas S. How dangerous is hyperkalemia? J Am Soc Nephrol. (2017) 28:3155–65. doi: 10.1681/ASN.2016121344
64. El-Sherif N, Turitto G. Electrolyte disorders and arrhythmogenesis. Cardiol J. (2011) 18:233−45.
65. Dittrich KL, Walls RM. Hyperkalemia: ECG manifestations and clinical considerations. J Emerg Med. (1986) 4:449–55. doi: 10.1016/0736-4679(86)90174-5
66. Kang BV, Kallmeyer SM, Casta A. Intraoperative alertness of a rise in serum potassium by double counting of the heart rate during blood transfusion in an infant. Pediatr Anesth. (2008) 18:798–800. doi: 10.1111/j.1460-9592.2008.02548.x
67. Baz EMK, Kanazi GE, Mahfouz RAR, Obeid MY. An unusual case of hyperkalaemia-induced cardiac arrest in a paediatric patient during transfusion of a “fresh” 6-day-old blood unit. Transfus Med. (2002) 12:383–6. doi: 10.1046/j.1365-3148.2002.00402.x
68. Strauss RG. Red blood cell storage and avoiding hyperkalemia from transfusions to neonates and infants. Transfusion. (2010) 50:1862–5. doi: 10.1111/j.1537-2995.2010.02789.x
69. Reilly M, Bruno C, Prudencio T, Ciccarelli N, Guerrelli D, Nair R, et al. Potential consequences of the red blood cell storage lesion on cardiac electrophysiology. J Am Heart Assoc. (2020) 9:e017748. doi: 10.1161/JAHA.120.017748
70. Howard-Quijano KJ, Stiegler MA, Huang YM, Canales C, Steadman RH. Anesthesiology residents performance of pediatric resuscitation during a simulated hyperkalemic cardiac arrest. Anesthesiology. (2010) 112:993–7. doi: 10.1097/ALN.0b013e3181d31fbe
71. Smith HM, Farrow SJ, Ackerman JD, Stubbs JR, Sprung J. Cardiac arrests associated with hyperkalemia during red blood cell transfusion: a case series. Anesth Analg. (2008) 106:1062–9. doi: 10.1213/ane.0b013e318164f03d
72. Vossoughi S, Perez G, Whitaker BI, Fung MK, Stotler B. Analysis of pediatric adverse reactions to transfusions. Transfusion. (2018) 58:60–9. doi: 10.1111/trf.14359
73. Whitaker BI, Belov A, Anderson SA. Progress in US hemovigilance: can we still learn from others? Transfusion. (2019) 59:433–6. doi: 10.1111/trf.15082
74. Bhananker SM, Ramamoorthy C, Geiduschek JM, Posner KL, Domino KB, Haberkern CM, et al. Anesthesia-related cardiac arrest in children: update from the pediatric perioperative cardiac arrest registry. Anesth Analg. (2007) 105:344–50. doi: 10.1213/01.ane.0000268712.00756.dd
75. Bolande RP, Traisman HS, Philipsborn HF. Electrolyte considerations in exchange transfusions for erythroblastosis fetalis. J Pediatr. (1956) 49:401–6. doi: 10.1016/S0022-3476(56)80225-4
76. Bolton DT. Hyperkalaemia, donor blood and cardiac arrest associated with ECMO priming. Anaesthesia. (2000) 55:825–6. doi: 10.1046/j.1365-2044.2000.01629-29.x
77. Bostic O, Duvernoy WFC. Hyperkalemic cardiac arrest during transfusion of stored blood. J Electrocardiol. (1972) 5:407–9. doi: 10.1016/S0022-0736(72)80054-2
78. Buntain SG, Pabari M. Massive transfusion and hyperkalaemic cardiac arrest in craniofacial surgery in a child. Anaesth Intensive Care. (1999) 27:530–3. doi: 10.1177/0310057X9902700518
79. Chen CKH, Hong CL, Kau YC, Lee HL, Chen CKH, Shyr MH. Fatal hyperkalemia during rapid and massive blood transfusion in a child undergoing hip surgery–a case report. Acta Anaesthesiol Sin. (1999) 37:163–6.
80. Galligan B, Cairns R, Schifano J, Selbing A, Bervil S. Preparation of packed red cells suitable for intravascular transfusion in utero. Transfusion. (1989) 29:179–81. doi: 10.1046/j.1537-2995.1989.29289146839.x
81. Inaba S, Nibu K, Takano H, Maeda Y, Uehara K, Oshige T, et al. Potassium-adsorption filter for RBC transfusion: a phase III clinical trial. Transfusion. (2000) 40:1469–74. doi: 10.1046/j.1537-2995.2000.40121469.x
82. Inoue S, Terada Y, Furuya H. Reproducible peaked T wave due to transfusion via central venous catheter in an infant. J Clin Anesth. (2010) 22:387–9. doi: 10.1016/j.jclinane.2009.10.012
83. Ivens D, Camu F. Sudden hyperkalemia during cardiopulmonary bypass with hypothermic cardiac arrest in an infant. J Cardiothorac Vasc Anesth. (1996) 10:258–60. doi: 10.1016/S1053-0770(96)80250-3
84. Kavčič A, Avčin S, Grosek Š. Severe hyperkalemia immediately after birth. Am J Case Rep. (2019) 20:1471–5. doi: 10.12659/AJCR.916368
85. Martin DP, Gomez D, Tobias JD, Schechter W, Cusi C, Michler R. Severe hyperkalemia during cardiopulmonary bypass: etiology and effective therapy. World J Pediatr Congenit Hear Surg. (2013) 4:197–200. doi: 10.1177/2150135112467213
86. Morray JP, Geiduschek JM, Ramamoorthy C, Haberkern CM, Hackel A, Caplan RA, et al. Anesthesia-related cardiac arrest in children: Initial findings of the pediatric perioperative cardiac arrest (POCA) registry. Anesthesiology. (2000) 93:6–14. doi: 10.1097/00000542-200007000-00007
87. Nakagawa M, Kubota M, Endo I, Inoue S, Seo N. Use of a K+-adsorption filter for the massive transfusion of irradiated red blood cells in a child. Can J Anesth. (2004) 51:639–40. doi: 10.1007/BF03018413
88. Pew WL. Cardiac arrest during exchange transfusion; successfully handled with thoracotomy and cardiac massage J Pediatr. (1955) 47:645–7. doi: 10.1016/S0022-3476(55)80087-X
89. Scanlon J, Krakaur R. Hyperkalemia following exchange transfusion. J Pediatr. (1980) 96:108–10. doi: 10.1016/S0022-3476(80)80342-8
90. Sohn H-M, Park Y-H, Byon H-J, Kim J-T, Kim H-S, Kim CS. Application of the continuous autotransfusion system (CATS) to prevent transfusion-related hyperkalemia following hyperkalemic cardiac arrest in an infant -a case report. Korean J Anesthesiol. (2012) 62:281–4. doi: 10.4097/kjae.2012.62.3.281
91. Taylor WC, Grisdale LC, Stewart AG. Unexplained death from exchange transfusion. J Pediatr. (1958) 52:694–700. doi: 10.1016/S0022-3476(58)80268-1
92. Woodforth IJ. Resuscitation from transfusion-associated hyperkalaemic ventricular fibrillation. Anaesth Intensive Care. (2007) 35:110–3. doi: 10.1177/0310057X0703500117
93. Harm SK, Raval JS, Cramer J, Waters JH, Yazer MH. Haemolysis and sublethal injury of RBCs after routine blood bank manipulations. Transfus Med. (2012) 22:181–5. doi: 10.1111/j.1365-3148.2011.01127.x
94. New HV, Berryman J, Bolton-Maggs PHB, Cantwell C, Chalmers EA, Davies T, et al. Guidelines on transfusion for fetuses, neonates and older children. Br J Haematol. (2016) 175:784–828. doi: 10.1111/bjh.14233
95. New HV, Stanworth SJ, Engelfriet CP, Reesink HW, McQuilten ZK, Savoia HF, et al. Neonatal transfusions. Vox Sang. (2009) 96:62–85. doi: 10.1111/j.1423-0410.2008.01105.x
96. Jobes DR, Sesok-Pizzini D, Friedman D. Reduced transfusion requirement with use of fresh whole blood in pediatric cardiac surgical procedures. Ann Thorac Surg. (2015) 99:1706–11. doi: 10.1016/j.athoracsur.2014.12.070
97. Valentine SL, Bembea MM, Muszynski JA, Cholette JM, Doctor A, Spinella PC, et al. Consensus recommendations for RBC transfusion practice in critically ill children from the pediatric critical care transfusion and anemia expertise initiative. Pediatr Crit Care Med. (2018) 19:884–98. doi: 10.1097/PCC.0000000000001613
98. Cholette JM, Willems A, Valentine SL, Bateman ST, Schwartz SM, Pediatric Critical Care Transfusion and Anemia Expertise Initiative (TAXI), Pediatric Critical Care Blood Research Network (Bloodnet), et al. Recommendations on RBC transfusion in infants and children with acquired and congenital heart disease from the pediatric critical care transfusion and anemia expertise initiative. Pediatr Crit Care Med. (2018) 19:S137–48. doi: 10.1097/PCC.0000000000001603
99. Grabmer C, Holmberg J, Popovsky M, Amann E, Schönitzer D, Falaize S, et al. Up to 21-day banked red blood cells collected by apheresis and stored for 14 days after automated wash at different times of storage. Vox Sang. (2006) 90:40–4. doi: 10.1111/j.1423-0410.2005.00719.x
100. Hansen A, Yi QL, Acker JP. Quality of red blood cells washed using the ACP 215 cell processor: assessment of optimal pre- and postwash storage times and conditions. Transfusion. (2013) 53:1772–9. doi: 10.1111/trf.12170
101. Delaney M, Axdorff-Dickey RL, Crockett GI, Falconer AL, Levario MJ, McMullan DM. Risk of extracorporeal life support circuit-related hyperkalemia is reduced by prebypass ultrafiltration. Pediatr Crit Care Med. (2013) 14:e263–7. doi: 10.1097/PCC.0b013e31828a70c5
102. Cid J, Villegas V, Carbassé G, Alba C, Perea D, Lozano M. Transfusion of irradiated red blood cell units with a potassium adsorption filter: a randomized controlled trial. Transfusion. (2016) 56:1046–51. doi: 10.1111/trf.13536
103. Brown KA, Bissonnette B, MacDonald M, Poon AO. Hyperkalaemia during massive blood transfusion in paediatric craniofacial surgery. Can J Anaesth. (1990) 37:401–8. doi: 10.1007/BF03005615
104. Weiskopf RB, Schnapp S, Rouine-Rapp K, Bostrom A, Toy P. Extracellular potassium concentrations in red blood cell suspensions after irradiation and washing. Transfusion. (2005) 45:1295–301. doi: 10.1111/j.1537-2995.2005.00220.x
105. Lacroix J, Hébert PC, Hutchison JS, Hume HA, Tucci M, Ducruet T, et al. Transfusion strategies for patients in pediatric intensive care units. N Engl J Med. (2007) 356:1609–19. doi: 10.1056/NEJMoa066240
Keywords: transfusion, hyperkalemia, cardiac arrest, pediatric, neonate, red blood cell storage lesion, red blood cell
Citation: Burke M, Sinha P, Luban NLC and Posnack NG (2021) Transfusion-Associated Hyperkalemic Cardiac Arrest in Neonatal, Infant, and Pediatric Patients. Front. Pediatr. 9:765306. doi: 10.3389/fped.2021.765306
Received: 26 August 2021; Accepted: 29 September 2021;
Published: 29 October 2021.
Edited by:
Begum Atasay, Ankara University Medical School, TurkeyReviewed by:
Tuuli Metsvaht, University of Tartu, EstoniaCopyright © 2021 Burke, Sinha, Luban and Posnack. This is an open-access article distributed under the terms of the Creative Commons Attribution License (CC BY). The use, distribution or reproduction in other forums is permitted, provided the original author(s) and the copyright owner(s) are credited and that the original publication in this journal is cited, in accordance with accepted academic practice. No use, distribution or reproduction is permitted which does not comply with these terms.
*Correspondence: Nikki Gillum Posnack, bnBvc25hY2tAY2hpbGRyZW5zbmF0aW9uYWwub3Jn
Disclaimer: All claims expressed in this article are solely those of the authors and do not necessarily represent those of their affiliated organizations, or those of the publisher, the editors and the reviewers. Any product that may be evaluated in this article or claim that may be made by its manufacturer is not guaranteed or endorsed by the publisher.
Research integrity at Frontiers
Learn more about the work of our research integrity team to safeguard the quality of each article we publish.