- 1Department of Neonatology, Shanghai Children's Hospital, Shanghai Jiao Tong University, Shanghai, China
- 2Department of Respiratory, Shanghai Children's Hospital, Shanghai Jiao Tong University, Shanghai, China
Background: The production of intestinal gases and fecal short-chain fatty acids (SCFAs) by infant gut microbiota may have a significant impact on their health, but information about the composition and volume of intestinal gases and SCFA profiles in preterm infants is scarce.
Objective: This study examined the change of the composition and volume of intestinal gases and SCFA profiles produced by preterm infant gut microbiota in vitro during the first 4 weeks of life.
Methods: Fecal samples were obtained at five time points (within 3 days, 1 week, 2 weeks, 3 weeks, and 4 weeks) from 19 preterm infants hospitalized in the neonatal intensive care unit (NICU) of Shanghai Children's Hospital, Shanghai Jiao Tong University between May and July 2020. These samples were initially inoculated into four different media containing lactose (LAT), fructooligosaccharide (FOS), 2′-fucosyllactose (FL-2), and galactooligosaccharide (GOS) and thereafter fermented for 24 h under conditions mimicking those of the large intestine at 37.8°C under anaerobic conditions. The volume of total intestinal gases and the concentrations of individual carbon dioxide (CO2), hydrogen (H2), methane (CH4), and hydrogen sulfide (H2S) were measured by a gas analyzer. The concentrations of total SCFAs, individual acetic acid, propanoic acid, butyric acid, isobutyric acid, pentanoic acid, and valeric acid were measured by gas chromatography (GC).
Results: The total volume of intestinal gases (ranging from 0.01 to 1.64 ml in medium with LAT; 0–1.42 ml with GOS; 0–0.91 ml with FOS; and 0–0.44 ml with FL-2) and the concentrations of CO2, H2, H2S, and all six fecal SCFAs increased with age (p-trends < 0.05). Among them, CO2 was usually the predominant intestinal gas, and acetic acid was usually the predominant SCFA. When stratified by birth weight (<1,500 and ≥1,500 g), gender, and delivery mode, the concentration of CO2 was more pronounced among infants whose weight was ≥1,500 g than among those whose weight was <1,500 g (p-trends < 0.05).
Conclusions: Our findings suggested that the intestinal gases and SCFAs produced by preterm infant gut microbiota in vitro increased with age during the first 4 weeks of life.
Introduction
The abundant and diverse members of the human gut microbiota have intricate roles in maintaining health (1, 2). A growing number of studies have attempted to characterize the composition of the gut microbiota using rRNA and DNA technologies, while much less is known about the metabolism of the intestinal microbiota in situ and in real time. Their byproducts, including intestinal gases and short-chain fatty acids (SCFAs), may be unique biomarkers for specific gut microbiota (3–5). Measuring these byproducts may accelerate our understanding of the relationships among intestinal gases, SCFAs, the metabolic activity of the gut microbiota, and human health states.
Previous studies indicated that a significant portion of the intestinal gases are produced through fermentation in the gut instead of the human host. Intestinal gases mainly include carbon dioxide (CO2), hydrogen (H2), methane (CH4), and hydrogen sulfide (H2S) (3, 6). Michael et al. measured the volume and composition of intestinal gases from 11 healthy, young male subjects by means of an intestinal washout technique. They found that the bowel normally contains relatively small quantities of gas ranging from 30 to 200 ml, and nitrogen (N2) was usually the predominant gas, whereas oxygen (O2) was less (7). According to a study performed in 20 healthy individuals, the primary constituents of flatus are N2 (59%), H2 (20.9%), CO2 (9%), CH4 (7.2%), O2 (3.9%), and H2S (0.00028%) (8). H2 and CH4 can be detected using breath tests as markers of carbohydrate fermentation following a carbohydrate load or the occurrence of small intestinal bacterial overgrowth (SIBO) (9). Microbes colonize the neonatal gut immediately following birth (1, 10). The gut bacterial communities in adults are different from those in infants. The intestinal gases produced by gut microbiota are also different between them. The volume and composition of intestinal gases produced by the infant gut microbiota are unknown, especially in preterm infants.
SCFAs, important byproducts of the complex interplay between diet and the gut microbiota within the gut lumen environment, are regarded as key signaling molecules between the gut microbiome and host health states (11). It is well established that SCFAs play a profound role in mucosal maintenance and integrity, glucose homeostasis, lipid metabolism, and immune function (4). Previous analysis of fecal SCFAs showed their potential to be used to detect patients with colorectal cancer (CRC) and adenomatous polyposis (AP) as a noninvasive means (12). In infants, the reduced concentrations of acetic acid and propionic acid may be related to the risk of breast milk jaundice (BMJ) (13). In addition, fecal SCFAs may be biomarkers of cow's milk protein allergy (CMPA) (14). Until now, there has been a study reporting the average total fecal SCFA concentrations and fecal butyrate concentrations of eight healthy adults over 12 weeks (15). However, information on the change in fecal SCFAs from meconium to feces during the first month of life is scarce, particularly in relation to preterm infants.
Therefore, the objective of our work was to reveal the evolution of the production of intestinal gases and fecal SCFAs in vitro by preterm infant gut microbiota during the first month of life. In addition, we made the observations about the role of birth weight, gender, delivery mode, and type of feeding for intestinal gas production and SCFAs after preterm birth.
Materials and Methods
Participants and Sample Collection
This study included 19 preterm babies hospitalized in the neonatal intensive care unit (NICU) of Shanghai Children's Hospital, Shanghai Jiao Tong University (Shanghai, China) between May and July 2020. Written informed parental consent was obtained for each preterm infant before inclusion. To be eligible for enrollment, preterm infants needed to be born at a complete gestational age (GA) of <37 weeks and without obvious malformations or inherited or metabolic diseases.
Spontaneously evacuated meconium and fecal samples were collected from diapers by the medical staff into 10-ml stool containers. Meconium was collected within 3 days after birth, and fecal samples were collected weekly from preterm infants when they were in the hospital, immediately stored at 4°C, and transported within 12 h for further fermentation.
Fermentation in vitro
Fermentation in vitro was carried out as previously described (16). The four types of culture media consisted of the following common compounds: peptone water, 5.0 g/L; tryptone, 5.0 g/L; yeast extract, 2.5 g/L; L-aminothiopropionic acid, 1 g/L; hemin, 2 ml/L; NaCl, 0.9 g/L; CaCl2·6H2O, 0.09 g/L; KH2PO4, 0.45 g/L; K2HPO4, 0.45 g/L; MgSO4·7H2O, 0.09 g/L; resazurin (1 g/L), 1 ml; and vitamin I solution (including biotin, 0.05 g/L; cobalamin, 0.05 g/L; para-aminobenzoic acid, 0.15 g/L; folic acid, 0. 25 g/L; and pyridoxamine, 0. 75 g/L), 200 μl/L. We added LAT, 8 g/L; galactooligosaccharide (GOS), 8 g/L; fructooligosaccharide (FOS), 8 g/L; and 2′-fucosyllactose (FL-2), 8 g/L as the sole carbon source in the four types of media. Each medium was inoculated with 0.5 ml of 10% fresh fecal slurry prepared with prereduced phosphate-buffered saline (PBS) buffer (150 mmol/L of NaCl, 10 mmol/L of Na2HPO4, and 20 mmol/L of NaH2PO4, pH 7.2–7.4). Then, they were fermented for 24 h under conditions mimicking those of the large intestine at 37.8°C under complete anaerobic conditions.
Intestinal Gas Measurement
The gas produced from preterm infant intestinal flora fermentation was detected by a gas analyzer (HL-QT01), which consists of a gas sampler, valving module, vacuum generator, and gas detection chamber integrated with several gas sensors. The valving module was used to control the gas quantity introduced into the gas detection chamber with the help of the vacuum generator. The programmable working process control and the sensing results analysis were performed by the electronics mainboard with preset software. The detection steps were as follows: (1) the vacuum generator adjusted the gas detection chamber to a certain vacuum level through the valving module; (2) the gas entered the instrument from the sample bottle through the gas sampler, and the gas volume was adjusted through the valving module; (3) the gas entered into the gas detection chamber was measured by gas sensors; and (4) the measurement results were calculated by preset software to obtain the gas content and bacterial composition in the sample container.
We calibrated the gas analyzer by detecting the gas in the medium that was not inoculated with fecal slurry and ensuring that the detection result was zero.
Short-Chain Fatty Acid Measurement
The concentrations of SCFAs were measured by gas chromatography (GC). The amounts of acetic acid, propanoic acid, butyric acid, isobutyric acid, pentanoic acid, and valeric acid in the culture media were measured by GC (Shimadzu, GC-2010 Plus, Kyoto, Japan) equipped with a DB-FFAP column (0.32 mm * 30 m * 0.5 mm) (Agilent Technologies, Santa Clara, CA, USA) using an H2 flame ionization detector.
Statistical Analysis
Statistical analyses were conducted using SPSS 26.0 software (SPSS Inc., Chicago, IL, USA). Quantitative data are expressed as the mean and 95% confidence intervals (CIs) of the mean or, when they were not nonnormally distributed, as the median and interquartile range (IQR). When classified by age, the Kruskal–Wallis test was used for comparison between groups. When classified by sex, delivery mode, or weight, the Mann–Whitney U test was used for comparisons among groups. p < 0.05 was considered to indicate a statistically significant difference.
Results
Infant Characteristics
Nineteen preterm infants (12 males and seven females) enrolled in the study had a mean GA of 31.11 (95% CI: 30.01, 32.20) weeks and a mean birth weight of 1,385.53 (95% CI: 1,235.89, 1,535.17) g. Of these 19 infants, nine were delivered by cesarean section, and 10 infants were delivered vaginally. Six infants received mechanical ventilation. Eleven infants were both breastfed and formula-fed, seven infants were exclusively breastfed, and only one infant was exclusively formula-fed. All infants received antibacterial prophylaxis for at least the first 5 days of life. The sociodemographic characteristics of the infants are presented in Table 1.
Intestinal Gases
We collected 95 stool specimens from 19 newborns and measured intestinal gases in 380 media. In medium with LAT, the total volume of intestinal gases produced by preterm infant gut microbiota was 0.01 (IQR: 0–0.31) ml within 3 days after birth (D3); 0.02 (IQR: 0–0.66) ml at 1 week after birth (W1); 0.60 (IQR: 0.01–1.72) ml at 2 weeks after birth (W2); 0.71 (IQR: 0.06–1.97) ml at 3 weeks after birth (W3); and 1.64 (IQR: 0.07–2.48) ml at 4 weeks after birth (W4). In medium with GOS, the total volume of intestinal gases was 0 (IQR: 0–0.75) ml within D3; 0.13 (IQR: 0–0.58) ml at W1; 0.15 (IQR: 0–1.71) ml at W2; 0.58 (IQR: 0.16–1.54) ml at W3; and 1.42 (IQR: 0.18–1.75) ml at W4. In medium with FOS, the total volume of intestinal gases was 0 (IQR: 0–0.77) ml within D3; 0.03 (IQR: 0–0.60) ml at W1; 0.20 (IQR: 0–1.71) ml at W2; 0.64 (IQR: 0.13–1.55) ml at W3; and 0.91 (IQR: 0.25–1.56) ml at W4. In medium with FL-2, the total volume of intestinal gases was 0 (IQR: 0–0.08) ml within D3; 0.13 (IQR: 0–0.24) ml at W1; 0.14 (IQR: 0–0.44) ml at W2; 0.44 (IQR: 0.20–0.55) ml at W3; and 0.41 (IQR: 0.25–0.80) ml at W4. The total volume of intestinal gases produced by preterm infant gut microbiota in the four media all increased with age (p-trends < 0.05) (Figure 1). The concentrations of CO2, H2, and H2S increased with age in the four media (p-trends < 0.05) (Figure 2). However, there was no difference in the concentration of CH4 among the five time points (p-trends > 0.05). CO2 and H2 are the two major intestinal gases. H2S was detectable in several samples (36/95). The detectable frequency of CH4 (75/95) was higher than that of H2S. When stratified by birth weight (<1,500 and ≥1,500 g), the concentration of CO2 was greater among infants whose birth weight was ≥1,500 g (p-trends < 0.05) (Figure 3). When stratified by gender, type of feeding, or delivery mode, differences were not found between the two groups (p-trends > 0.05).
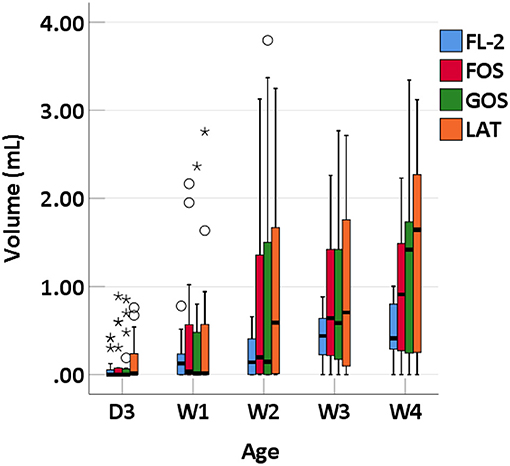
Figure 1. Total volume of intestinal gases of preterm infants of different ages. D3, 3 days after birth; W1, 1 week after birth; W2, 2 weeks after birth; W3, 3 weeks after birth; W4, 4 weeks after birth; LAT, lactose; FOS, fructooligosaccharide; FL-2, milk oligosaccharide; GOS, galactooligosaccharide; *, extreme value; °, discrete value.
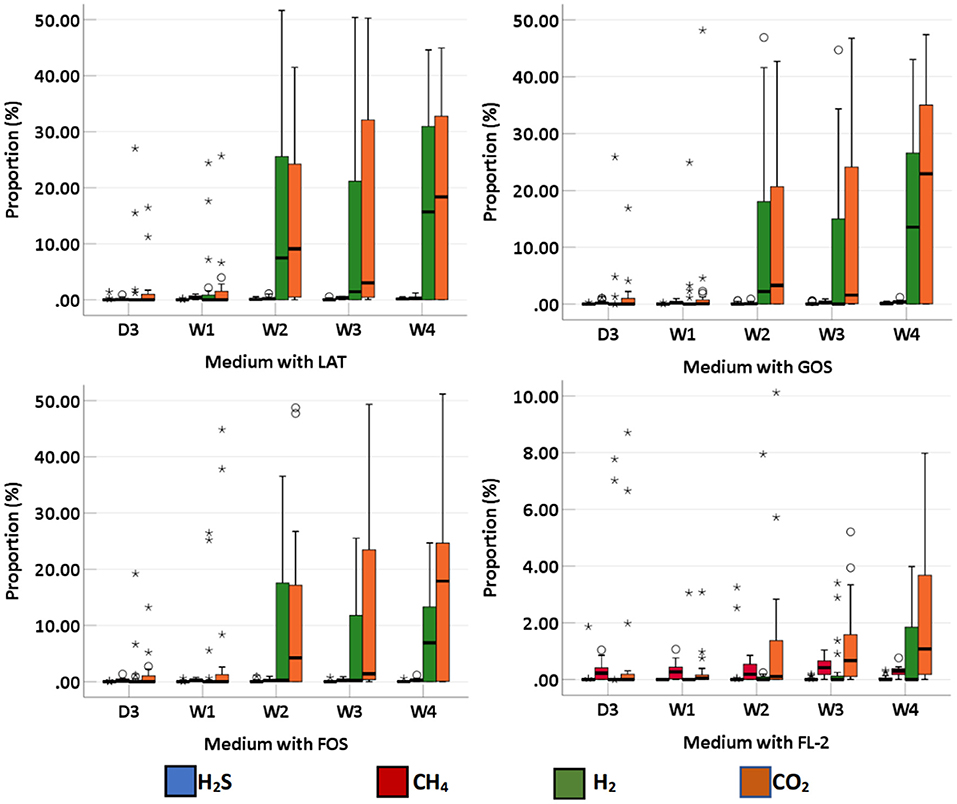
Figure 2. The proportion of intestinal gas composition (CO2, H2, CH4, and H2S) of preterm infants of different ages. D3, 3 days after birth; W1, 1 week after birth; W2, 2 weeks after birth; W3, 3 weeks after birth; W4, 4 weeks after birth; LAT, lactose; FOS, fructooligosaccharide; FL-2, milk oligosaccharide; GOS, galactooligosaccharide; *, extreme value; °, discrete value.
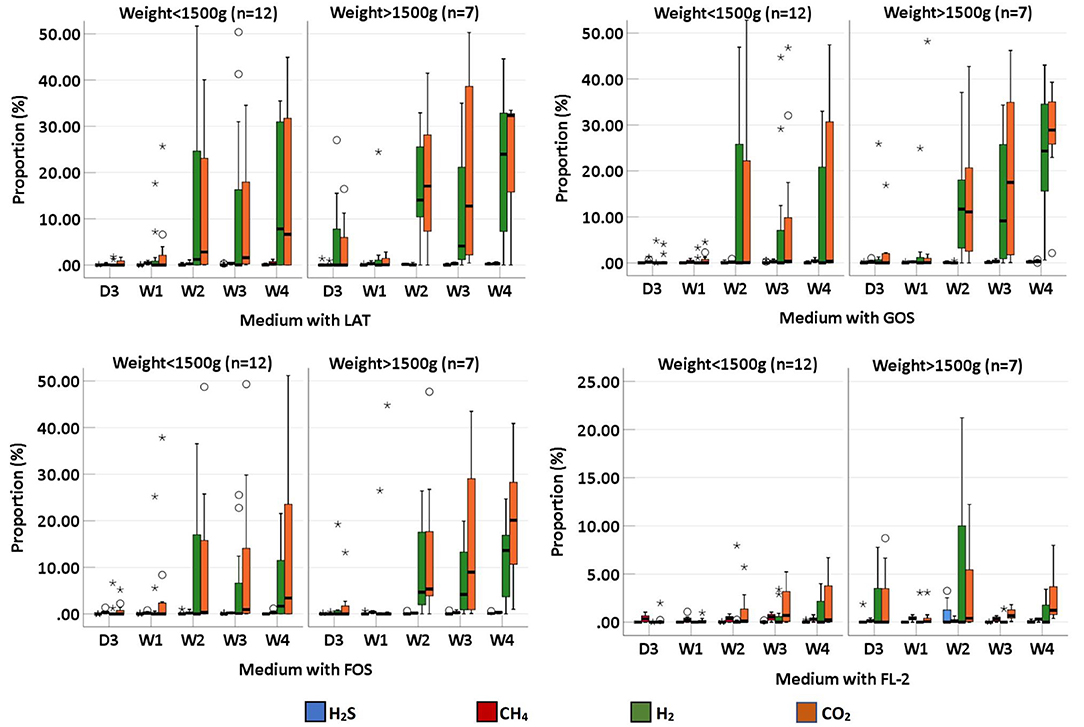
Figure 3. The proportion of intestinal gas composition (CO2, H2, CH4, and H2S) of preterm infants of different ages, stratified by weight (<1,500 and >1,500 g); D3, 3 days after birth; W1, 1 week after birth; W2, 2 weeks after birth; W3, 3 weeks after birth; W4, 4 weeks after birth; LAT, lactose; FOS, fructooligosaccharide; FL-2, milk oligosaccharide; GOS, galactooligosaccharide; *, extreme value; °, discrete value.
Short-Chain Fatty Acids
SCFAs were measured in a total of 95 fecal specimens. In medium with LAT, the concentration of total SCFAs produced by preterm infant gut microbiota was 1.14 (0.88–7.09) μmol/g within D3; 3.46 (2.37–5.13) μmol/g at W1; 6.71 (3.73–17.08) μmol/g at W2; 11.71 (5.86–23.77) μmol/g at W3; and 9.09 (2.11–23.24) μmol/g at W4. In medium with GOS, the concentration of total SCFAs was 1.11 (0.89–2.93) μmol/g within D3; 3.07 (2.04–5.54) μmol/g at W1; 5.88 (2.68–15.34) μmol/g at W2; 8.78 (5.38–17.66) μmol/g at W3; and 10.09 (2.56–19.25) μmol/g at W4. In medium with FOS, the concentration of total SCFAs was 1.01 (0.86–3.65) μmol/g within D3; 3.62 (2.56–5.40) μmol/g at W1; 5.57 (2.98–11.94) μmol/g at W2; 6.68 (4.53–13.15) μmol/g at W3; and 7.49 (2.93–19.19) μmol/g at W4. In medium with FL-2, the concentration of total SCFAs was 1.96 (1.06–7.09) μmol/g within D3; 6.95 (3.86–7.57) μmol/g at W1; 7.82 (4.38–12.75) μmol/g at W2; 12.03 (10.31–15.57) μmol/g at W3; and 10.93 (8.39–14.36) μmol/g at W4. The concentrations of total SCFAs, acetic acid, propanoic acid, butyric acid, isobutyric acid, pentanoic acid, and valeric acid in the four media usually increased with age (p-trends < 0.05) (Figures 4, 5). Among them, acetic acid was the predominant SCFA, and the concentration of pentanoic acid was the lowest. When stratified by birth weight (<1,500 and ≥1,500 g), gender, or delivery mode, differences in the concentrations of SCFAs were not significant (p-trends > 0.05).
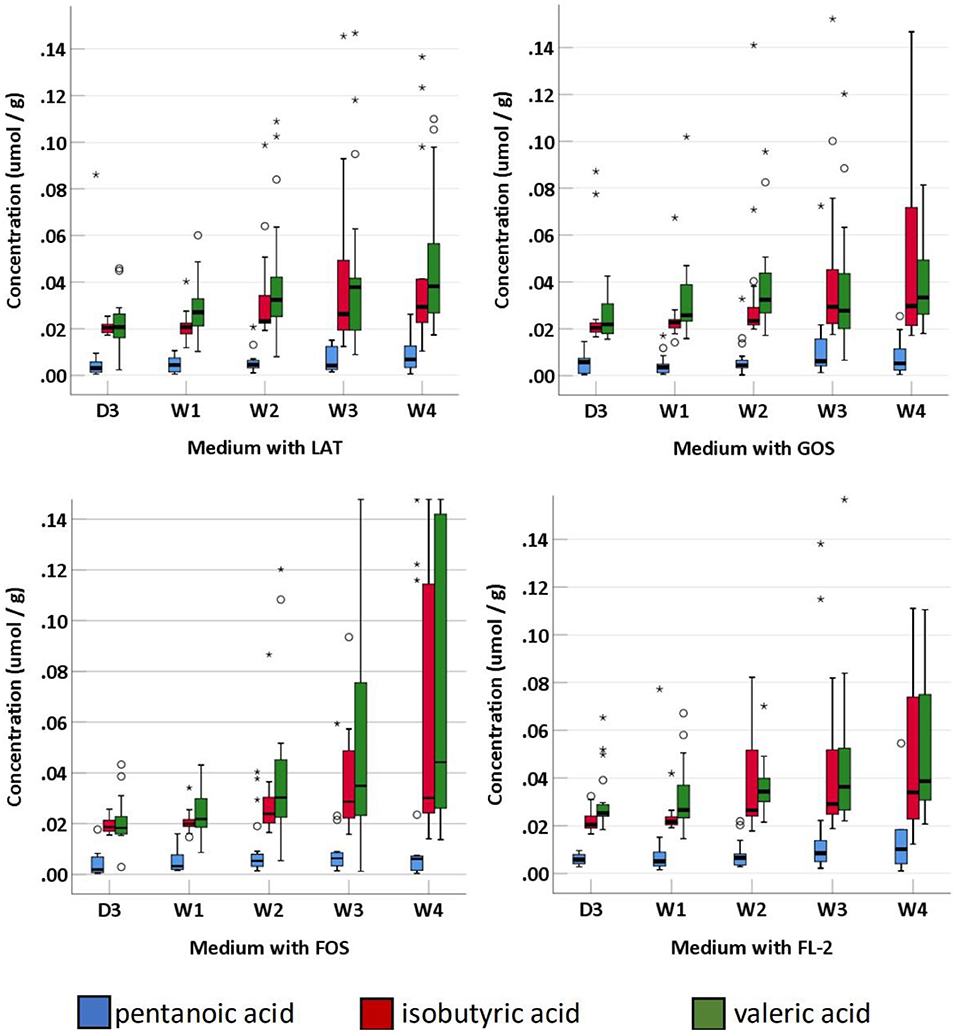
Figure 4. The concentration of fecal SCFAs (pentanoic acid, isobutyric acid, and valeric acid) of preterm infants of different ages. D3, 3 days after birth; W1, 1 week after birth; W2, 2 weeks after birth; W3, 3 weeks after birth; W4, 4 weeks after birth; LAT, lactose; FOS, fructooligosaccharide; FL-2, milk oligosaccharide; GOS, galactooligosaccharide; *, extreme value; °, discrete value; SCFAs, short-chain fatty acids.
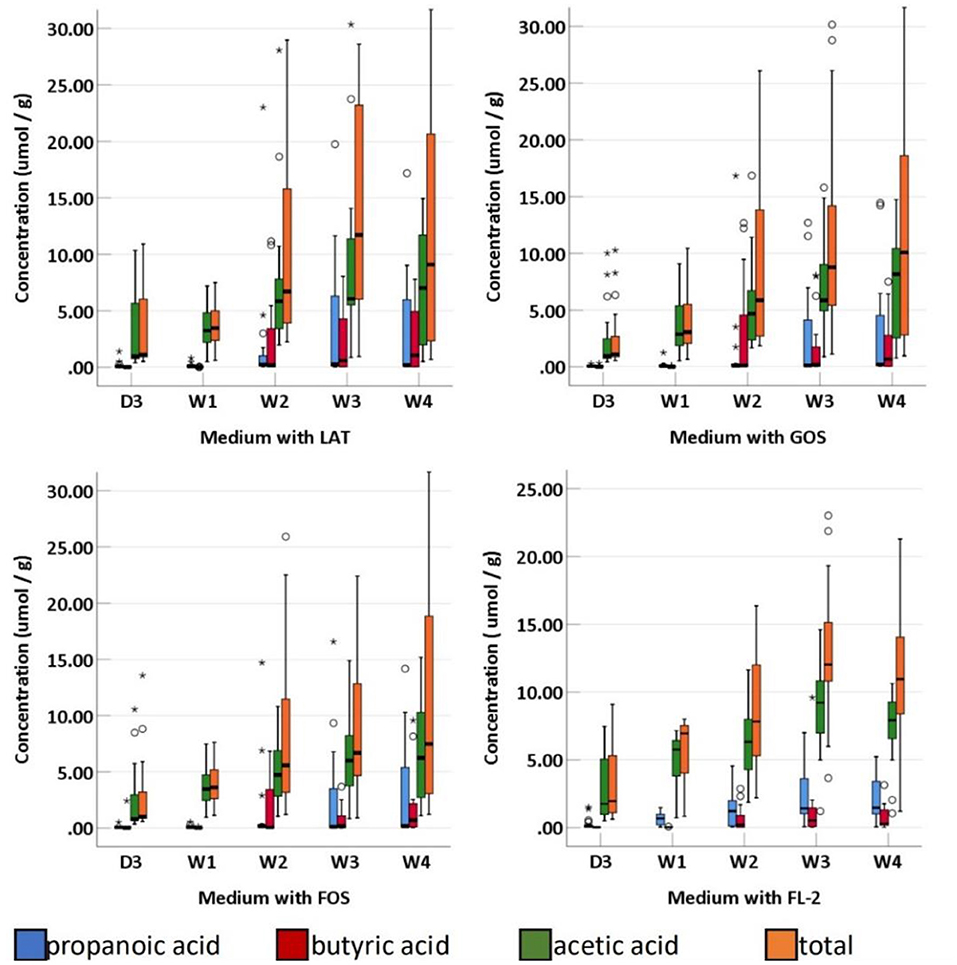
Figure 5. The concentration of fecal SCFAs (propanoic acid, butyric acid, acetic acid, and total) of preterm infants of different ages. D3, 3 days after birth; W1, 1 Week after birth; W2, 2 weeks after birth; W3, 3 weeks after birth; W4, 4 weeks after birth; LAT, lactose; FOS, fructooligosaccharide; FL-2, milk oligosaccharide; GOS, galactooligosaccharide; *, extreme value; °, discrete value; SCFAs, short-chain fatty acids.
Discussion
We followed the development of the intestinal gases and SCFAs produced by gut microbiota in vitro longitudinally in a cohort of 19 prematurely born infants from birth to 4 weeks. We examined the change in the composition and volume of intestinal gases and SCFA profiles produced by preterm infant gut microbiota in vitro during the first 4 weeks of life. We found that the intestinal gases and SCFAs produced by gut microbiota in vitro increased with age during the first 4 weeks of life. A number of H2 and CO2 began to be detected at 2 weeks. This change has a strong association with gut microbiota colonization, which develops over the course of host infancy to eventually reach its adult form (17, 18). The gut microbiota community occurs during the first 3 years of life. As the diversity and number of gut microbiota increase in the first several years, the volume and composition of intestinal gases change constantly.
CO2 can be produced after each food intake in the stomach or in the rest of the gut segments. CO2 is a major gas generated during the bacterial fermentation of carbohydrates in the distal small intestine and colon. Our results showed that CO2 was usually the predominant intestinal gas in preterm infants, which is consistent with previous studies (9). Additionally, we found that the concentration of CO2 increased sharply at 2 weeks after birth. This result indicated that the massive colonization of gut microbiota that produces CO2 may begin at 2 weeks after the birth of preterm infants.
Previous studies indicated that a broad assemblage of hydrogenogens must exist in the human colon. Most likely, it is most abundant in the right colon, the colonic region with the greatest extent of microbial fermentation (19). H2 production is integral to broad microbial fermentation. Our study found that the proportion of H2 produced by the gut microbiota within the 3-day and first-week fecal samples was almost zero. The time at which H2 began to be detected was 2 weeks after birth. A number of hydrogenogens may begin to colonize the gut of preterm infants at 2 weeks after birth. In addition, the concentration of H2 in the medium with LAT was greater than that in the other three media. According to a previous study, patients diagnosed with lactose (LAT) intolerance excreted more H2 via the lungs, which was detected by the LAT breath test (20, 21). It is apparent that the breath test is not suitable for neonates. The fermentation in vitro and gas analysis system may therefore be a useful tool for the diagnosis of LAT intolerance in neonates.
Peled et al. analyzed CH4 concentration in the expired air of 393 infants, children, and adolescents and found that there was no CH4 production below the age of 3 years (22). However, Methanobacteria were detected in fecal samples obtained from children at 27 months of age (23). Dridi's study indicated a high prevalence of Methanobrevibacter smithii and Methanosphaera stadtmanae in the human gut using an improved DNA detection protocol, and the youngest subject in this study was 1 month (24). In our study, CH4 was detected in the majority of samples. This result may prove that neonates may produce CH4, but only when the production reaches a threshold does it appear in the breath (25). In addition, we found that there was no difference in the proportion of CH4 among the groups classified by age. Previous studies showed that the quantities of M. smithii in human feces remained constant over time. Methanogenic activity seems to have remained unaltered over a period of many years with dietary and antibiotic changes (26). This trend of stability of M. smithii has also been reported over a 13-month period using fecal specimens from two adult individuals (27). Those studies can at least partly explain our results.
H2S is a notoriously toxic gas. Despite its lethal effects, H2S is a vital molecule for living organisms, including humans. It is involved in inflammation, gut motility, oxidative stress, ulcer healing, vascular tone, neuromodulation, cryoprotection, memory formation, hormone secretion, apoptosis, and many other vital biological functions (28). The concept was proposed decades before that H2S may increase the risk of mucosal inflammation (29). Recently, an increasing number of studies have suggested that a limited amount of H2S is necessary for reducing the risk of colonic mucosal inflammation (30–32). Our results showed that H2S was detectable in a small portion of samples. Among the preterm infants in our study, a high concentration of H2S was detected in the fecal samples of an infant diagnosed with neonatal necrotizing enterocolitis (NEC). Besides the intestinal gases, fecal volatile organic compounds (VOCs) are other products of metabolic pathways of intestinal microorganisms. Recently, more and more studies showed the potential of VOC analysis as an early diagnostic biomarker for NEC in preterm infants (33–35). Therefore, we hypothesized that the gas analyzer can be used as a new tool for exploring the relationship between H2S and NEC, which needs a large number of experiments to confirm.
A recent study has showed that VOC profiles, as measured by an eNose device, in preterm infants born at GA <30 weeks, are not influenced by GA or mode of delivery during the first 3 weeks of life (36). In this study, we evaluated the effects of mode of delivery, birth weight, gender, and type of feeding on intestinal gases. When stratified by birth weight (<1,500 and ≥1,500 g), only the concentration of CO2 was different between the two groups. When stratified by gender, type of feeding, or delivery mode, differences in the volume of total intestinal gases and the concentrations of CO2, H2, CH4, and H2S were not significant between the two groups. This result indicates that birth weight may influence the production of CO2 in the human gut. However, gender and delivery mode have little impact on intestinal gas production. More samples are needed to confirm our results.
SCFAs are the major end products of bacterial metabolism in the human large intestine. The principal SCFAs that result from both carbohydrate and amino acid fermentation are acetate, propionate, and butyrate (37), which are similar to the SCFA profiles of preterm infants. In addition, we found that the concentrations of total SCFAs, acetic acid, propanoic acid, butyric acid, isobutyric acid, pentanoic acid, and valeric acid in the four media usually increased with age. Previous findings showed that SCFA formation by intestinal bacteria was regulated by many different host, environmental, dietary, and microbiological factors (37). In the past decade, several studies have indicated that feeding types and perinatal antibiotics significantly affect the function of the gut microbiota and the content of SCFAs in the stool samples of premature infants (38–40). When stratified by birth weight (<1,500 and ≥1,500 g), gender, or delivery mode, differences in the concentrations of SCFAs in preterm infants were not significant. More samples and grouping are needed to confirm our findings. SCFAs represent the major carbon flux from the diet through the gut microbiota to the host, and evidence is emerging for a regulatory role of SCFAs in local, intermediary, and peripheral metabolism. Manipulating the diet-gut microbiome–host metabolism axis may be a useful prevention of some leading causes of morbidity and mortality (4). However, our understanding of the significance of SCFAs in human metabolic health is limited, especially in neonates. Thus, more well-designed and controlled human studies are needed.
The first strength of this study was the prospective and standardized collection and handling of fecal samples by which we reduce the risk of potential pre-analytical errors. Secondly, we excluded the measurement of disease-specific intestinal gases and SCFAs by excluding infants with obvious malformations or inherited or metabolic diseases. Lastly, the gas analyzer (HL-QT01) used in this study was noninvasive compared with the tonometric balloon technique and intestinal washout technique used in other studies (6, 41).
There are several limitations that need to be addressed. Firstly, the study sample size was relatively small to evaluate the effects of delivery mode, birth weight, gender, and feeding type on intestinal gases and SCFAs concentrations among these heterogeneous premature babies. Large studies are needed to confirm our findings. Secondly, there was no microbiome analysis. Although we did not directly conduct gut microbiome analysis, in vitro fermentation methodology was a sophisticated platform for studying the gut microbiota composition and functionality (42).
Lastly, this was an in vitro study. In a variety of studies, researchers have used in vitro fermentations to explore fermentation rate, SCFA production, and changes in microbiota in existence of dietary fibers (43). Batch in vitro fermentations, which were generally carried out in test tubes within a short period of time (usually 24–48 h), was the most convenient way for studying microbial degradation of specific dietary compounds and therefore to explore the metabolites released and how the host health could be affected (42). LAT, FOS, GOS, and FL-2 were generally thought to be fermented in the colon of infants and degraded into physiologically active metabolites and gases. Therefore, the capability of infant gut microbiota degrading oligosaccharides (including LAT, FOS, GOS, and FL-2) to produce intestinal gases and SCFAs was compared in our study. We found that in vitro batch fermentation could be further used as a valuable screening platform for initial examination of food stuffs or food components and could help optimize individualized nutrition in infants.
As in vitro models do not involve epithelial or immune cells, it is true that their applicability to study complexity of the human colon and host–gut microbiota interactions are limited. However, in vitro models have their own advantages. Firstly, they offer unique opportunities for quantitative measurements of particular microbial metabolites. As the majority of SCFAs are absorbed by colonic epithelial cells and metabolized by cells in different tissues including colon cells, only 5%−10% are excreted in the feces (43). Measurement of fecal SCFAs may lead to overestimation or underestimation of intestinal microbial metabolism. Secondly, in vitro models also provide tools to monitor the metabolic activities of the microbiota, rather than merely description of fecal bacterial composition or metabolite composition.
In summary, our study demonstrated that the intestinal gases and SCFAs produced by preterm infant gut microbiota in vitro increased with age during the first 4 weeks of life. The production of intestinal gases and SCFAs is strongly associated with gut microbiota colonization and health status. More well-designed and controlled human studies are needed to explore the associations between intestinal gases and SCFA production with gut microbiota colonization and diseases of preterm infants.
Data Availability Statement
The original contributions presented in the study are included in the article/Supplementary Materials, further inquiries can be directed to the corresponding author/s.
Ethics Statement
The studies involving human participants were reviewed and approved by Ethics Committee of Shanghai Children's Hospital. Written informed consent to participate in this study was provided by the participants' legal guardian/next of kin. Written informed consent was obtained from the minor(s)' legal guardian/next of kin for the publication of any potentially identifiable images or data included in this article.
Author Contributions
YH and GD: conceptualization and methodology. XW and JL: formal analysis and writing—original draft preparation. DY and HZ: collected clinical samples and performed experiments. NL and KG: data curation. YH: writing—review and editing and funding acquisition. All authors contributed to the article and approved the submitted version.
Funding
This work was supported by the Special Project of Clinical Research on Health Industry of Shanghai Health Commission (Grant Number 201940329) and Special Project of Clinical Research of Shanghai Children's Hospital (Grant Number 2020YLYZ01).
Conflict of Interest
The authors declare that the research was conducted in the absence of any commercial or financial relationships that could be construed as a potential conflict of interest.
Publisher's Note
All claims expressed in this article are solely those of the authors and do not necessarily represent those of their affiliated organizations, or those of the publisher, the editors and the reviewers. Any product that may be evaluated in this article, or claim that may be made by its manufacturer, is not guaranteed or endorsed by the publisher.
Supplementary Material
The Supplementary Material for this article can be found online at: https://www.frontiersin.org/articles/10.3389/fped.2021.726193/full#supplementary-material
Abbreviations
SCFAs, short-chain fatty acids; NICU, neonatal intensive care unit; LAT, lactose; FOS, fructooligosaccharide; FL-2, milk oligosaccharide; GOS, galactooligosaccharide; CO2, carbon dioxide; H2, hydrogen; CH4, methane; H2S, hydrogen sulfide; GC, gas chromatography; N2, nitrogen; SIBO, small intestinal bacterial over growth; CRC, colorectal cancer; AP, adenomatous polyposis; BMJ, breast milk jaundice; CMPA, cow's milk protein allergy; 95% CI, 95% confidence intervals; IQR, interquartile range; D3, three days after birth; W1, 1 week after birth; W2, 2 weeks after birth; W3, 3 weeks after birth; W4, 4 weeks after birth; NEC, necrotizing enterocolitis; VOC, fecal volatile organic compound.
References
1. Christian M, Sabrina D, Francesca B, Francesca T, Jennifer M, Silvia AM, et al. The first microbial colonizers of the human gut: composition, activities, and health implications of the infant gut microbiota. Microbiol Mol Biol Rev. (2017) 81:e00036–00017. doi: 10.1128/MMBR
2. Christopher LG, Tiffany LW. The gut microbiota at the intersection of diet and human health. Science. (2018) 362:776–80. doi: 10.1126/science.aau5812
3. Pimentel M, Mathur R, Chang C. Gas and the microbiome. Curr Gastroenterol Rep. (2013) 15:356. doi: 10.1007/s11894-013-0356-y
4. Morrison DJ, Preston T. Formation of short chain fatty acids by the gut microbiota and their impact on human metabolism. Gut Microbes. (2016) 7:189–200. doi: 10.1080/19490976.2015.1134082
5. Scaldaferri F, Nardone O, Lopetuso LR, Petito V, Bibbò S, Laterza L, et al. Intestinal gas production and gastrointestinal symptoms: from pathogenesis to clinical implication. Eur Rev Med Pharmacol Sci. (2013) 17:2–10
6. Ou JZ, Yao CK, Rotbart A, Muir JG, Gibson PR. Kalantar-zadeh K. Human intestinal gas measurement systems: in vitro fermentation and gas capsules. Trends Biotechnol. (2015) 33:208–13. doi: 10.1016/j.tibtech.2015.02.002
7. Levitt MD. Volume and composition of human intestinal gas determined by means of an intestinal washout technic. N Engl J Me. (1971) 284:1394–8. doi: 10.1056/NEJM197106242842502
8. Sahakian AB, Jee SR, Pimentel M. Methane and the gastrointestinal tract. Dig Dis Sci. (2010) 55:2135–43. doi: 10.1007/s10620-009-1012-0
9. Kalantar-Zadeh K, Berean KJ, Burgell RE, Muir JG, Gibson PR. Intestinal gases: influence on gut disorders and the role of dietary manipulations. Nat Rev Gastroenterol Hepatol. (2019) 16:733–47. doi: 10.1038/s41575-019-0193-z
10. Hill CJ, Lynch DB, Murphy K, Ulaszewska M, Jeffery IB, O'Shea CA, et al. Evolution of gut microbiota composition from birth to 24 weeks in the INFANTMET Cohort. Microbiome. (2017) 5:4. doi: 10.1186/s40168-016-0213-y
11. Sivaprakasam S, Prasad PD, Singh N. Benefits of short-chain fatty acids and their receptors in inflammation and carcinogenesis. Pharmacol Ther. (2016) 164:144–51. doi: 10.1016/j.pharmthera.2016.04.007
12. Niccolai E, Baldi S, Ricci F, Russo E, Nannini G, Menicatti M, et al. Evaluation and comparison of short chain fatty acids composition in gut diseases. World J Gastroenterol. (2019) 25:5543–58. doi: 10.3748/wjg.v25.i36.5543
13. Duan M, Han ZH, Huang T, Yang Y, Huang B. Characterization of gut microbiota and short-chain fatty acid in breastfed infants with or without breast milk jaundice. Lett Appl Microbiol. (2021) 72:60–7. doi: 10.1111/lam.13382
14. Thompson-Chagoyan OC, Fallani M, Maldonado J, Vieites JM, Khanna S, Edwards C, et al. Faecal microbiota and short-chain fatty acid levels in faeces from infants with cow's milk protein allergy. Int Arch Allergy Immunol. (2011) 156:325–32. doi: 10.1159/000323893
15. McOrist AL, Abell GC, Cooke C, Nyland K. Bacterial population dynamics and faecal short-chain fatty acid (SCFA) concentrations in healthy humans. Br J Nutr. (2008) 100:138–46. doi: 10.1017/S0007114507886351
16. Shen Q, Zhao L, Tuohy KM. High-level dietary fibre up-regulates colonic fermentation and relative abundance of saccharolytic bacteria within the human faecal microbiota in vitro. Eur J Nutr. (2012) 51:693–705. doi: 10.1007/s00394-011-0248-6
17. Yatsunenko T, Rey FE, Manary MJ, Trehan I, Dominguez-Bello MG, Contreras M, et al. Human gut microbiome viewed across age and geography. Nature. (2012) 486:222–7. doi: 10.1038/nature11053
18. Yassour M, Vatanen T, Siljander H, Hämäläinen AM, Härkönen T, Ryhänen SJ, et al. Natural history of the infant gut microbiome and impact of antibiotic treatment on bacterial strain diversity and stability. Sci Transl Med. (2016) 8:343ra81. doi: 10.1126/scitranslmed.aad0917
19. Carbonero F, Benefiel AC, Gaskins HR. Contributions of the microbial hydrogen economy to colonic homeostasis. Nat Rev Gastroenterol Hepatol. (2012) 9:504–18. doi: 10.1038/nrgastro.2012.85
20. Law D, Conklin J, Pimentel M. Lactose intolerance and the role of the lactose breath test. Am J Gastroenterol. (2010) 105:1726–8. doi: 10.1038/ajg.2010.146
21. Metz G, Jenkins DJ, Peters TJ, Newman A, Blendis LM. Breath hydrogen as a diagnostic method for hypolactasia. Lancet. (1975) 1:1155–7. doi: 10.1016/s0140-6736(75)93135-9
22. Peled Y, Gilat T, Liberman E, Bujanover Y. The development of methane production in childhood and adolescence. J Pediatr Gastroenterol Nutr. (1985) 4:575–9. doi: 10.1097/00005176-198508000-00013
23. Rutili A, Canzi E, Brusa T, Ferrari A. Intestinal methanogenic bacteria in children of different ages. New Microbiol. (1996) 19:227–43
24. Dridi B, Henry M, El Khéchine A, Raoult D, Drancourt M. High prevalence of Methanobrevibacter smithii and Methanosphaera stadtmanae detected in the human gut using an improved DNA detection protocol. PLoS ONE. (2009) 4:e7063. doi: 10.1371/journal.pone.0007063
25. McKay LF, Eastwood MA, Brydon WG. Methane excretion in man–a study of breath, flatus, and faeces. Gut. (1985) 26:69–74. doi: 10.1136/gut.26.1.69
26. Conway, de Macario E, Macario AJ. Methanogenic archaea in health and disease: a novel paradigm of microbial pathogenesis. Int J Med Microbiol. (2009) 299:99–108. doi: 10.1016/j.ijmm.2008.06.011
27. Miller TL, Wolin MJ. Stability of Methanobrevibacter smithii populations in the microbial flora excreted from the human large bowel. Appl Environ Microbiol. (1983) 45:317–8. doi: 10.1128/aem.45.1.317-318.1983
28. Singh SB, Lin HC. Hydrogen sulfide in physiology and diseases of the digestive tract. Microorganisms. (2015) 3:866–89. doi: 10.3390/microorganisms3040866
29. Levine J, Ellis CJ, Furne JK, Springfield J, Levitt MD. Fecal hydrogen sulfide production in ulcerative colitis. Am J Gastroenterol. (1998) 93:83–7. doi: 10.1111/j.1572-0241.1998.083_c.x
30. Wallace JL, Vong L, McKnight W, Dicay M, Martin GR. Endogenous and exogenous hydrogen sulfide promotes resolution of colitis in rats. Gastroenterology. (2009) 137:569–78. doi: 10.1053/j.gastro.2009.04.012
31. Wang R. Physiological implications of hydrogen sulfide: a whiff exploration that blossomed. Physiol Rev. (2012) 92:791–896. doi: 10.1152/physrev.00017.2011
32. Drucker NA, Jensen AR, Ferkowicz M, Markel TA. Hydrogen sulfide provides intestinal protection during a murine model of experimental necrotizing enterocolitis. J Pediatr Surg. (2018) 53:1692–8. doi: 10.1016/j.jpedsurg.2017.12.003
33. Berkhout DJC, Niemarkt HJ, de Boer NKH, Benninga MA, de Meij TGJ. The potential of gut microbiota and fecal volatile organic compounds analysis as early diagnostic biomarker for necrotizing enterocolitis and sepsis in preterm infants. Expert Rev Gastroenterol Hepatol. (2018) 12:457–70. doi: 10.1080/17474124.2018.1446826
34. Hosfield BD, Pecoraro AR, Baxter NT, Hawkins TB, Markel TA. The assessment of fecal volatile organic compounds in healthy infants: electronic nose device predicts patient demographics and microbial enterotype. J Surg Res. (2020)254:340–7. doi: 10.1016/j.jss.2020.05.010
35. de Meij TG, van der Schee MP, Berkhout DJ, van de Velde ME, Jansen AE, Kramer BW, et al. Early detection of necrotizing enterocolitis by fecal volatile organic compounds analysis. J Pediatr. (2015) 167:562–7.e1. doi: 10.1016/j.jpeds.2015.05.044
36. Deianova N, El Manouni El Hassani S, Niemarkt HJ, Cossey V, van Kaam AH, Jenken F, et al. Fecal volatile organic compound profiles are not influenced by gestational age and mode of delivery: a longitudinal multicenter cohort study. Biosensors (Basel). (2020) 10:50. doi: 10.3390/bios10050050
37. Macfarlane S, Macfarlane GT. Regulation of short-chain fatty acid production. Proc Nutr Soc. (2003) 62:67–72. doi: 10.1079/PNS2002207
38. Chen C, Yin Q, Wu H, Cheng L, Kwon JI, Jin J, et al. Different effects of premature infant formula and breast milk on intestinal microecological development in premature infants. Front Microbiol. (2020) 10:3020. doi: 10.3389/fmicb
39. Pourcyrous M, Nolan VG, Goodwin A, Davis SL, Buddington RK. Fecal short-chain fatty acids of very-low-birth-weight preterm infants fed expressed breast milk or formula. J Pediatr Gastroenterol Nutr. (2014) 59:725–31. doi: 10.1097/MPG.0000000000000515
40. Arboleya S, Sánchez B, Solís G, Fernández N, Suárez M, Hernández-Barranco AM, et al. Impact of prematurity and perinatal antibiotics on the developing intestinal microbiota: a functional inference study. Int J Mol Sci. (2016) 17:649. doi: 10.3390/ijms17050649
41. Sobko T, Elfström K, Navér L, Lundberg JO, Norman M. Intestinal hydrogen and nitric oxide gases in preterm infants–effects of antibiotic therapy. Neonatology. (2009) 95:68–73. doi: 10.1159/000151757
42. Pérez-Burillo S, Molino S, Navajas-Porras B, Valverde-Moya ÁJ, Hinojosa-Nogueira D, López-Maldonado A, et al. An in vitro batch fermentation protocol for studying the contribution of food to gut microbiota composition and functionality. Nat Protoc. (2021) 16:3186–209. doi: 10.1038/s41596-021-00537-x
Keywords: intestinal gas, fecal short-chain fatty acid, carbon dioxide, hydrogen, methane, hydrogen sulfide
Citation: Wang X, Li J, Li N, Guan K, Yin D, Zhang H, Ding G and Hu Y (2021) Evolution of Intestinal Gases and Fecal Short-Chain Fatty Acids Produced in vitro by Preterm Infant Gut Microbiota During the First 4 Weeks of Life. Front. Pediatr. 9:726193. doi: 10.3389/fped.2021.726193
Received: 16 June 2021; Accepted: 27 August 2021;
Published: 27 September 2021.
Edited by:
Jill L. Maron, Tufts Medical Center, United StatesReviewed by:
Corentin Babakissa, Université de Sherbrooke, CanadaEner Cagri Dinleyici, Eskişehir Osmangazi University, Turkey
Camilia Martin, Beth Israel Deaconess Medical Center and Harvard Medical School, United States
Copyright © 2021 Wang, Li, Li, Guan, Yin, Zhang, Ding and Hu. This is an open-access article distributed under the terms of the Creative Commons Attribution License (CC BY). The use, distribution or reproduction in other forums is permitted, provided the original author(s) and the copyright owner(s) are credited and that the original publication in this journal is cited, in accordance with accepted academic practice. No use, distribution or reproduction is permitted which does not comply with these terms.
*Correspondence: Yong Hu, aHV5b25nY25AMTYzLmNvbQ==; Guodong Ding, ZGluZ2d1b2RvbmdAc2hjaGlsZHJlbi5jb20uY24=
†These authors have contributed equally to this work