- 1Neonatal Intensive Care Unit, Fondazione IRCCS Ca' Granda Ospedale Maggiore Policlinico, Milan, Italy
- 2Division of Biostatistics, Epidemiology and Public Health, Department of Statistics and Quantitative Methods, University of Milano-Bicocca, Milan, Italy
- 3Department of Clinical Sciences and Community Health, Università degli Studi di Milano, Milan, Italy
- 4Department of Pediatric Surgery, Fondazione IRCCS Ca' Granda Ospedale Maggiore Policlinico, Milan, Italy
- 5Pediatric Radiology Unit, Fondazione IRCCS Ca' Granda Ospedale Maggiore Policlinico, Milan, Italy
- 6Department of Obstetrics and Gynecology, Fondazione IRCCS Ca' Granda, Ospedale Maggiore Policlinico, Milan, Italy
- 7Cardiology Department, Fondazione IRCCS Ca' Granda Ospedale Maggiore Policlinico, Milan, Italy
- 8Neonatal Intensive Care Unit, Fondazione IRCCS Policlinico San Matteo, Pavia, Italy
Congenital diaphragmatic hernia is a rare disease with high mortality and morbidity due to pulmonary hypoplasia and pulmonary hypertension. The aim of the study is to investigate the relationship between radiographic lung area and systolic pulmonary artery pressure (sPAP) on the first day of life, mortality, and hernia recurrence during the first year of life in infants with a congenital diaphragmatic hernia (CDH). A retrospective data collection was performed on 77 CDH newborns. Echocardiographic sPAP value, deaths, and recurrence cases were recorded. Lung area was calculated by tracing the lung's perimeter, excluding mediastinal structures, and herniated organs, on the preoperative chest X-ray performed within 24 h after birth. Logistic and linear regression analyses were performed. Deceased infants showed lower areas and higher sPAP values. One square centimeter of rising in the total, ipsilateral, and contralateral area was associated with a 22, 43, and 24% reduction in mortality risk. sPAP values showed a decreasing trend after birth, with a maximum of 1.84 mmHg reduction per unitary increment in the ipsilateral area at birth. Recurrence patients showed lower areas, with recurrence risk decreasing by 14 and 29% per unit increment of the total and ipsilateral area. In CDH patients, low lung area at birth reflects impaired lung development and defect size, being associated with increased sPAP values, mortality, and recurrence risk.
Clinical Trial Registration: The manuscript is an exploratory secondary analysis of the trial registered at ClinicalTrials.gov with identifier NCT04396028.
Introduction
Congenital diaphragmatic hernia (CDH) is a severe congenital malformation with a wide outcome variability (1). Pulmonary hypoplasia and persistent pulmonary hypertension (PH) represent the two main determinants of outcomes in patients, with still high mortality and morbidity (2–12). The radiographic assessment of the lung area has been proposed as an alternative method to evaluate pulmonary hypoplasia soon after birth (13–15). In newborns with CDH, lung area is correlated to the functional residual capacity measured through the diluted helium technique, and its increase is associated with tidal volume improvement in the first year of life (16, 17). The chest radiographic thoracic area (CRTA) was found to be lower in patients with poor prognosis and to predict survival to discharge from the Neonatal Intensive Care Unit (NICU) better than lung-to-head ratio at diagnosis (LHR) (18). However, a possible association between lung area and pulmonary hypertension has never been investigated.
Hernia recurrence represents one of the most common complications, and a large diaphragmatic defect is one of the main independent risk factors (19–25). The recurrence could occur weeks, months, or even years after the primary surgery, and patients often remain asymptomatic for a long time or until complications arise. Therefore, the overall risk of recurrence during the lifespan remains unknown (8). To our knowledge, an association between lung area and hernia recurrence has never been reported so far.
Since lung hypoplasia and vascular development are strictly related, our hypothesis was that lower lung areas at birth could determine higher mortality and higher pulmonary artery pressure (25–27). Moreover, we supposed that low lung area could indirectly reflect a large diaphragmatic defect size and be therefore associated with hernia recurrence (16, 18).
Methods
The present study was carried out in accordance with the principles of good clinical practice and the Helsinki Declaration, as well as the national legislative and administrative provisions in force. This study was approved by the local Ethics Committee (Milan Area 2, Italy) with approval number OSMAMI-04/05/2020-0015998-U. Due to the retrospective nature of the study, the informed consent was waived by the Ethics Committee.
This study represents an exploratory secondary analysis of a previous retrospective cohort study called Assessment of the Pulmonary Area in Newborns with Congenital diaphragmatic HErnia (NeoAPACHE), performed at NICU of Fondazione IRCCS Ca' Granda Ospedale Maggiore Policlinico, Milan, Italy, on CDH patients over a 6-year period (January 2012–December 2018). A comprehensive description of the main study design has been previously published (NeoAPACHE I) (17).
In NeoAPACHE II, we aimed to evaluate the relationship between radiographic pulmonary area assessed on the first day of life and:
1. Pulmonary hypertension at birth, indirectly estimated by measuring the sPAP through tricuspid valve regurgitation gradient with echocardiogram;
2. Death during the first year of life; and
3. Recurrence of CDH among survivors at 1 year of life.
Moreover, the radiological features and outcomes of neonates candidate to FETO procedure were described and compared with those of the expectantly managed patients. At our Center, FETO was offered to fetuses with severe lung hypoplasia, defined as an O/E LHR <25 and <45% in left and right CDH, respectively, in the absence of major associated malformations and/or genetic anomalies known to have a significant impact on postnatal survival (28, 29).
Subjects
All CDH patients admitted to our NICU are managed according to the CDH EURO Consortium Consensus guidelines (30). As previously described, we enrolled all newborns having a preoperative chest X-ray performed within 24 h after birth at our NICU. Death within 1 h, rotated, and air leak radiographs were excluded (17). Surgery was performed as soon as the patient achieved the hemodynamic and respiratory stability through median laparotomy with either primary repair or Gore-Tex® patch insertion. According to CDHSG defect size classification, patching was performed in case of large defects (type C or D) (30–34). After discharge, all patients were included in a multidisciplinary follow-up program. In our Unit, we prefer to perform a chest X-ray at all time points during the first 2 years of life, then annually, aiming to detect asymptomatic recurrences early (8, 30, 35).
Assessment of Radiographic Pulmonary Area
Each patient's pulmonary area was assessed by freehand tracing of the diaphragm and rib cage's perimeter, excluding the mediastinal structures and herniated organs (17). If the anatomy was particularly disrupted, only the aerated portion of the lung was considered. The corresponding area was automatically calculated by the software Synapse PACS (FUJIFILM Medical Systems USA, Inc.). On each radiogram, three measurements were performed:
1. Ipsilateral pulmonary area (cm2);
2. Contralateral pulmonary area (cm2); and
3. Total pulmonary area (cm2), obtained as the sum of the preceding two.
Data Collection
Data regarding prenatal history, clinical, and surgical course were collected from each patient's electronic medical records. Hernia severity was defined according to the combined evaluation of observed/expected lung-to-head ratio (O/E LHR%), liver herniation, and side of the diaphragmatic defect (left, right, bilateral) (2, 28, 29). Echocardiograms performed after birth (T0), pre-surgery (T1), post-surgery (T2), and 7 days after surgery (T3) were reviewed, and reported sPAP values were recorded. CDH recurrence after surgical repair and the number of deaths in the first year of life were considered. Data acquisition was anonymous.
Statistical Analysis
Continuous variables were reported as mean (standard deviation) or median (interquartile range); categorical variables were presented as number and percentage. For the comparison between groups, Student's t-test, Mann-Whitney U-test, or Fisher exact test were applied as appropriate.
The reproducibility of the method has already been assessed in the primary analysis, using the Bland Altman plot and calculating the Pearson correlation index (17).
Logistic regression models were used to evaluate the relationship between the lung area and death or hernia recurrence risk. Linear regression models were used to assess the effects of lung area on sPAP values. The models were corrected for gestational age at birth, as this variable could independently influence the lung development and survival of patients.
The ROC curve was also calculated to assess the discriminatory capacity of the radiographic measurement, thus analyzing the sensitivity and specificity of the test.
Statistical analysis was performed using IBM SPSS®Statistics V26.0. A p-value of 0.05 or lower was considered to be statistically significant.
Data Availability
The manuscript illustrates the results of an exploratory secondary analysis of the principal study NeoAPACHE I, registered at the ClinicalTrials.gov with identifier NCT04396028. Datasets generated during and/or analyzed during the current study are available from the corresponding author.
Results
The radiographic pulmonary area was assessed on 77 patients, 49 of whom survived to discharge and were alive at the age of 1 year (36.4% mortality rate) (Figure 1). The majority of CDH were left-sided, with a high prevalence of severe forms and liver herniation. Fetal endoscopic tracheal occlusion (FETO) was performed in one-third of the cases, while extracorporeal membrane oxygenation (ECMO) was required in three patients. In more than half of cases, a diaphragmatic patch was needed for surgical repair, and in one patient, an abdominal patch was also used (Table 1).
Radiographic Pulmonary Area, Pulmonary Hypertension, and Mortality
The study population was divided into two groups, deceased (n = 28) and survived (n = 49). Compared with survivors, deceased patients showed a lower mean observed/expected lung-to-head ratio (O/E-LHR%) both at diagnosis and before birth, and the liver was herniated more frequently. Moreover, both gestational age and weight were lower, and patch insertion was significantly higher (Table 2).
At all times, deceased patients showed higher systolic pulmonary arterial pressure (sPAP) values compared with survivors (sPAP T0: 64.4 ± 17.2 vs. 54.4 ± 5.3 mmHg, p = 0.016; sPAP T1: 60.7 ± 10.9 vs. 50.6 ± 16.2 mmHg, p = 0.022; sPAP T2: 55.1 ± 17.9 vs. 46.4 ± 17.7 mmHg, p = 0.163; sPAP T3: 65.7 ± 16.5 vs. 38.8 ± 13.6 mmHg, p < 0.001; Figure 2A). They also showed lower mean total, ipsilateral, and contralateral pulmonary areas at birth (total pulmonary area: 8.1 ± 4.6 vs. 15.1 ± 6.9 cm2, p < 0.001; ipsilateral pulmonary area: 1.8 ± 2.6 vs. 5.1 ± 3.4 cm2, p < 0.001; contralateral pulmonary area, 6.2 ± 3.0 vs. 10.0 ± 4.3 cm2, p < 0.001; Figure 2B).
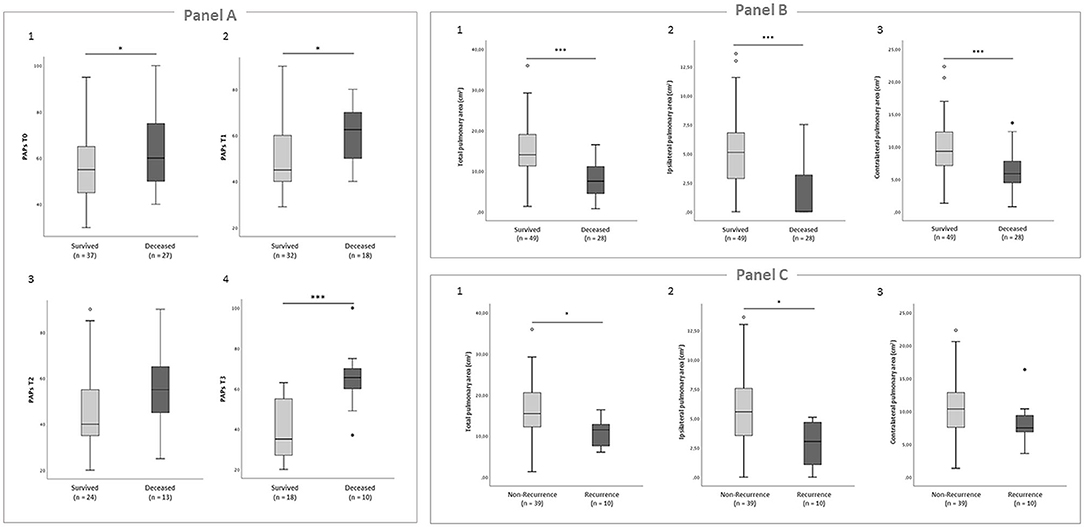
Figure 2. (A) Boxplots showing the comparison of sPAP values between survived and deceased patients. Student's t-test was performed to compare the two groups. (1) sPAP T0, p = 0.016; (2) sPAP T1, p = 0.022; (3) sPAP T2, p = 0.163; (4) sPAP T3, p < 0.001. (B) Boxplots showing the comparison of radiographic lung area on the first day of life between survived and deceased patients. Student's t-test was performed to compare the two groups. (1) Total pulmonary area, p < 0.001; (2) ipsilateral pulmonary area, p < 0.001; (3) contralateral pulmonary area, p < 0.001. (C) Boxplots showing the comparison of radiographic lung area on the first day of life between non-recurrence and recurrence patients. Student's t-test was performed to compare the two groups. (1) Total pulmonary area, p = 0.034; (2) ipsilateral pulmonary area, p = 0.011; (3) contralateral pulmonary area, p = 0.164.
At birth, pulmonary area and sPAP were significantly associated: as the three areas increased, sPAP at T0 significantly decreased, as shown by the linear regression model (Table 3A).
Following logistic regression analysis with death as the outcome variable, the increase in all radiographic parameters was also significantly related to improved survival in the first year of life (Table 3B).
Finally, with increasing sPAP at T0, the risk of death significantly increased as well (Table 3C).
The receiver operating characteristic (ROC) curve analysis showed that the total pulmonary area had an area under the curve (AUC) of 0.808, and a cut-off of 10.87 cm2 predicted survival with 77.6% sensitivity and 75% specificity (Figure 3A1). The ipsilateral pulmonary area had an AUC of 0.772, and a cut-off of 2.08 cm2 predicted survival with 81.6% sensitivity and 68% specificity (Figure 3A2). The contralateral pulmonary area had an AUC of 0.775, and a cut-off of 7.3 cm2 predicted survival with 75% sensitivity and 68% specificity (Figure 3A3).
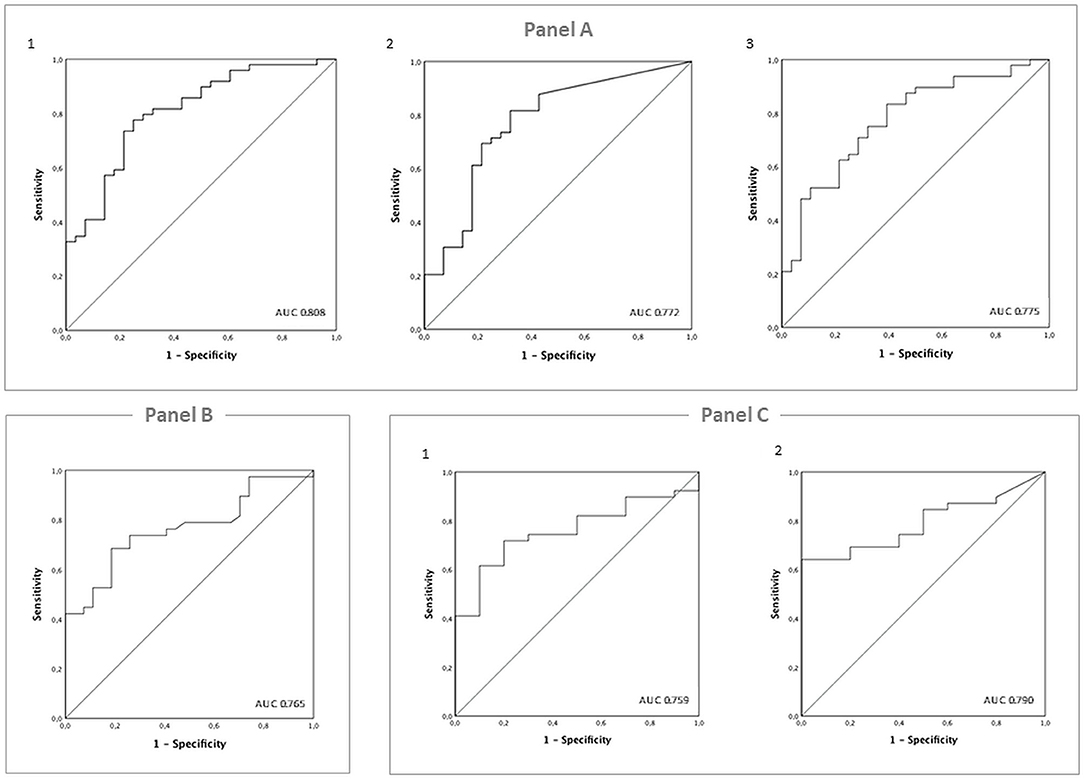
Figure 3. (A) ROC analysis and estimation of the corresponding area under the curve (AUC) for the radiographic pulmonary area's performance in predicting survival to 1 year of life. (1) Total pulmonary area; (2) ipsilateral pulmonary area; (3) contralateral pulmonary area. (B) ROC analysis and estimation of the corresponding area under the curve (AUC) for the O/E LHR% at diagnosis performance in predicting hernia recurrence in the first year of life. (C) ROC analysis and estimation of the corresponding area under the curve (AUC) for the radiographic performance of the pulmonary area in predicting hernia recurrence in the first year of life. (1) Total pulmonary area; (2) ipsilateral pulmonary area.
We finally performed aROC curve analysis using the O/E LHR at diagnosis. The total pulmonary area had an area AUC of 0.765, and a cut-off value of 31.7% showed 74% sensitivity and 74% specificity in predicting survival (Figure 3B).
Radiographic Pulmonary Area and Hernia Recurrence
Survivors at the end of the first year of life were divided into two groups based on hernia recurrence: recurrence (n = 10) and non-recurrence (n = 39; Table 4).
The recurrence group mainly included severe-moderate forms (80 vs. 28.2%), while most non-recurrence patients were mild (20 vs. 71.8%). Although the mean initial O/E-LHR% was not significantly different, the mean final O/E-LHR% was lower in the recurrence group (44.4 vs. 56.9%, p = 0.029). Even though diaphragmatic patching was higher in the recurrence group, this difference was not significant. Recurrence patients required longer intensive care (Table 4).
The mean total and ipsilateral pulmonary area were significantly lower in the recurrence compared with non-recurrence group (total pulmonary area: 11.0 ± 3.2 vs. 16.2 ± 7.2 cm2, p = 0.034; ipsilateral pulmonary area: 2.7 ± 2 vs. 5.7 ± 3.4 cm2, p = 0.011), while the mean contralateral area was not significantly different (8.3 ± 3.3 vs. 10.5 ± 4.5 cm2, p = 0.164; Figure 2C).
The logistic regression model showed that as the total and ipsilateral areas increased, CDH recurrence significantly decreased (Table 3D).
The ROC curve analysis showed that the total pulmonary area had an AUC of 0.759, and a cut-off of 13.07 cm2 predicted a 1-year follow-up free of hernia recurrence with 71.8% sensitivity and 80% specificity (Figure 3C1). The ipsilateral pulmonary area had an AUC of 0.790, and a cut-off of 3.75 cm2 had 74.4% sensitivity and 60% specificity (Figure 3C2).
Comparison Between FETO and Non-FETO Patients
Mild cases have been excluded from the non-FETO population to achieve a more homogeneous CDH population of moderate-severe cases, either treated in utero or expectantly managed. The new population was constituted 45 patients, divided into 28 FETO (100% severe) and 17 non-FETO (76.5% moderate and 23.5% severe) (Table 5).
FETO group was more severely affected, as showed by lower mean O/E-LHR% at diagnosis and a higher liver herniation rate. However, the mean O/E-LHR% before birth was higher.
No differences were found in the mean total and contralateral pulmonary area, while the mean ipsilateral pulmonary area was significantly increased in the FETO group.
Mean sPAP values, length of pharmacological treatments, and mechanical ventilation were not significantly different. Despite lower gestational age at birth, no significant difference in mortality rate was observed (FETO 57.1% vs. non-FETO 58.8%, p > 0.999). The recurrence rate among survivors did not reach statistical significance (p = 0.074).
Discussion
Our study showed an association between radiographic lung area, sPAP values, and death, confirming pulmonary hypoplasia and pulmonary hypertension as the two most important determinants of mortality (25, 26, 36). Among survivors, lung area was also associated with hernia recurrence. As previously reported, our findings suggest a possible role of the radiographic lung area assessment as an easy, non-invasive, and reproducible tool in the early prediction of mortality and morbidity among patients with CDH (17, 18).
In our cohort, lower O/E-LHR% in the deceased group indicated a more severe fetal lung impairment, which was then reflected in smaller pulmonary areas at birth. Consequently, lung area and death were inversely related: 1 cm2 of rising in the ipsilateral area was associated with a 43% reduction in mortality, while variations in the total and contralateral area determined a reduction of 22 and 24%, respectively.
Wide defects have been previously associated with worse survival and pulmonary hypertension, suggesting that small lung size depicts the link between these two elements (6). Similarly, in our cohort, deceased infants were characterized by persistently higher sPAP values than survivors. In particular, sPAP values at birth showed a decreasing trend by 1.84 mmHg, with each 1 cm2 increase in the ipsilateral area.
Our findings were consistent with previous literature (13, 16, 18). A significantly lower CRTA was reported in newborns with CDH who died compared with survivors, and a CRTA >12.99 cm2 was found to predict survival to discharge from NICU better than LHR at diagnosis, with 85% sensitivity and 73% specificity (18). In our study, we considered the O/E LHR% at diagnosis instead of the absolute ratio, but similarly, the lung area performed better in predicting mortality.
After surgical repair, persistently elevated pulmonary pressure carried the highest mortality risk, with a 16% increase in death risk for each sPAP unitary increment. Several studies have correlated the severity of PH with mortality. Dillon et al. evaluated mortality in a cohort of CDH patients and reported that all those with supra-systemic sPAP died (26). Coughlin et al. reported that patients with higher pulmonary pressure at 1 month had a higher incidence of post-operative complications and worse survival, and persistently severe PH at 1 month was associated with increased mortality (6). Similarly, looking at our results, we could assume that the most critical factor might not be the absolute value of sPAP or the presence of PH in the first hours after birth, rather its persistence over time (6).
We also observed a significant association between preoperative radiographic measurements and hernia recurrence among survivors during the first year of life. The overall recurrence rate of 20.4% in our cohort was in line with the literature reports (19, 24, 37). In particular, the recurrence rate was higher in those patients with lower final O/E LHR%, prolonged invasive respiratory support, and need for intensive care. Similarly, Al-Iede et al. found a longer duration of mechanical ventilation and hospitalization in children with recurrence (21). Notably, these patients showed a significantly lower mean total pulmonary area at birth than non-recurrence, mainly due to a significantly lower ipsilateral pulmonary area.
As a consequence, we respectively, observed a 14 and 29% reduction in recurrence risk in our cohort per unit increment of the total and ipsilateral area. The total radiographic area had the best specificity in discriminating those patients at risk of recurrence, while the ipsilateral area showed better sensitivity. Taken together, the lower ipsilateral area and O/E-LHR% reflected the presence of a large diaphragmatic defect as the cause of poor lung development, indirectly confirming defect size as the leading risk factor for hernia recurrence (19–22, 24). In other words, we speculate that recurrence patients were somehow “predisposed” to this complication since birth and could have been identified early in the postnatal course. The recurrence group's high patching rate suggested the presence of a wide defect, although this difference did not reach statistical significance. We cannot deduce any specific contribution of the patch in determining the recurrence risk due to the low sample size.
We observed that tracheal occlusion improved lung development and outcome through the descriptive comparison between FETO and non-FETO patients' characteristics. Since prenatal treatment is reserved for severe cases of CDH, the FETO group included only patients at one end of the spectrum of disease severity (2). Nevertheless, final O/E-LHR% dramatically improved after the procedure, and the ipsilateral lung area at birth was even significantly better so that the total pulmonary area did not differ between the two groups. Likewise, Dassios et al. observed that patients previously submitted to the FETO procedure had a CRTA comparable with untreated patients with a similar mortality rate, reflecting a lung catch-up growth favored by the prenatal procedure (18).
In our cohort, the non-FETO group, which was primarily constituted by moderate cases, showed a 41.2% survival rate, in line with what is generally expected for this category of CDH (2, 38, 39). As observed by Doneè et al., tracheal occlusion allowed improved outcomes in the operative group, similar to a moderate population expectantly managed (40). Finally, the recurrence risk was not significantly different between the two populations, despite higher patching in the FETO group, as previously observed by Ali et al. (41). Although patch repair is a leading risk factor, the low recurrence rate suggests other factors besides patch use as possible re-herniation determinants (20, 42). Tsai et al. reported a non-significant difference in recurrence rate between patching and primary repair, despite a higher disease severity in the first group (20). Jawaid et al. reported a low incidence of recurrence in patients in which Gore-Tex® patch was inserted (42). Although we cannot conclude on the patch's contribution to re-herniation, we can observe that lower radiographic area at birth could influence the risk of this complication and speculate that lung catch-up growth in FETO patients could confer the same recurrence risk as the untreated counterparts, which needs to be confirmed with further data (19, 21, 22, 24).
To the best of our knowledge, our study seems to be the first to evaluate the association between radiographic lung area and two important outcomes affecting newborns with CDH: pulmonary hypertension and hernia recurrence.
The radiographic measurement is easy, rapid, and can be performed soon after birth on the chest X-ray routinely performed at NICU admission. It would contribute to the early identification of infants at greater risk of developing higher sPAP values in the immediate postnatal period and a higher likelihood of long-term hernia recurrence and higher mortality. For example, the combined serial evaluation of lung area and sPAP over time could help to define trajectories related to the risk of persistently elevated sPAP and chronic pulmonary hypertension. Similarly, the preoperative radiographic assessment could help identify a subgroup of patients at higher risk of recurrence, directing them toward a tailored surgical follow-up.
The ipsilateral and contralateral areas were considered separately, evaluating the impact of hernia on each lung. We demonstrated that the ipsilateral area, which is more seriously affected by visceral herniation, has the most significant influence on patient outcomes.
Finally, focusing on FETO patients, we confirm the positive effects of the fetal procedure on lung catch-up growth and patient outcome.
Patients from our cohort showed a broad spectrum of disease severity, including infants requiring fetal surgery and ECMO support, and the standardization of treatment according to international guidelines guarantees uniformity of care.
A certain technical difficulty in tracing the lung perimeter in severe forms must be underlined as a limitation of the study. We arbitrarily decided to consider only those parts of the radiograms where a lung plot was present, corresponding to those regions effectively recruited and ventilated. However, the interference of mechanical compression exerted by the herniated organs plays a considerable role.
This methodological decision could constitute a bias leading to underestimating the lung dimensions since atelectasis areas had been excluded from the measurement. Therefore, after mechanical compression has been removed, the effective lung area evaluation could reliably define lung hypoplasia and associated outcome. For example, Dimitriou et al. calculated the difference between the pre- and post-operative radiographic measurements, showing that post-operative improvement was higher in patients with a good outcome. They concluded that poor prognosis was correlated to low post-operative rather than low preoperative values, which was probably more related to mechanical compression than lung hypoplasia (16). Therefore, the radiographic assessment of post-operative lung areas and the relative increase from preoperative values should be included in further analysis.
The neonatal ECMO Center was activated in 2016, with only three patients undergoing extracorporeal support during the study period. This could have had an impact on survival chances. In addition, team training and expertise plays a major role in favorable ECMO outcome. We expect a survival improvement in the very recent years, which we would like to confirm with additional analysis (43–45).
Another significant weakness is related to the retrospective design of the study, which limited the sample size. Some missing data regarding sPAP estimation could not be integrated with further hemodynamic assessments. Therefore, only an exploratory secondary analysis could be performed. Finally, we recognize that several factors could influence pulmonary vascular resistance and mortality throughout the hospital stay, such as pharmacological treatments, infections, patency of the ductus arteriosus, or surgery timing. Similarly, radiographic lung area could be influenced by factors such as quality of image, ventilator settings, under- or overinflation, which can then influence vascular resistance as well. The contribution of these factors cannot be completely assessed with single imaging performed at birth, which reflects the patient's conditions in a defining moment. Although the highest lung area can be considered a good approximation, it might not reflect the patient best clinical condition, and these factors should be taken into account in further analysis on a larger cohort. In addition, it would be of great importance to match lung area and sPAP values at T1, T2, and T3, to clarify if the association is still confirmed over time and define possible trajectories.
Conclusions
The radiographic pulmonary area on the first day of life reflects impaired lung development during fetal life and the extent of the diaphragmatic defect in CDH patients. Lower lung areas are associated with higher sPAP values at birth, death, and hernia recurrence. Further studies are needed to consolidate these results and define the possible role of the radiographic lung area for early risk assessment, monitoring, and outcome prediction in newborns with CDH.
Data Availability Statement
The datasets presented in this study can be found in online repositories. The names of the repository/repositories and accession number(s) can be found at: ClinicalTrials.gov n° NCT04396028.
Ethics Statement
The studies involving human participants were reviewed and approved by Milan Area 2, Italy. Written informed consent from the participants' legal guardian/next of kin was not required to participate in this study in accordance with the national legislation and the institutional requirements.
Author Contributions
IA, GC, GR, SGa, SGh, VC, GB, NPes, and FMo contributed to the study's conception and design. IA, GR, GC, VC, SGa, SGh, and FMa wrote the first draft of the manuscript. IA, NPes, and GC calculated the sample size. IA and NPes performed the statistical analysis. IA and IB assessed radiographic pulmonary areas. IB, NPer, IF, FMa, AC, MC, and FMo provided extensive critical revision. All authors contributed to the manuscript's critical revision and read and approved the submitted version.
Conflict of Interest
The authors declare that the research was conducted in the absence of any commercial or financial relationships that could be construed as a potential conflict of interest.
Acknowledgments
The authors would like to thank all the Neonatal ECMO Team Mangiagalli of the Fondazione IRCCS Ca' Granda Ospedale Maggiore Policlinico: nurses and neonatologists of the NICU, surgeons of the Department of Pediatric Surgery, anesthesiologists of the Pediatric Anesthesiology and Intensive Care Unit, and nurses of the operating room.
Abbreviations
AUC, area under the curve; CDH, congenital diaphragmatic hernia; CRTA, chest radiographic thoracic area; ECMO, extracorporeal membrane oxygenation; FETO, fetal endoscopic tracheal occlusion; NICU, neonatal intensive care unit; O/E LHR%, observed/expected lung-to-head ratio; PH, pulmonary hypertension; ROC, receiver operating characteristic; sPAP, systolic pulmonary artery pressure.
References
1. Grover TR, Murthy K, Brozanski B, Gien J, Rintoul N, Keene S, et al. Short-term outcomes and medical and surgical interventions in infants with congenital diaphragmatic hernia. Am J Perinatol. (2015) 32:1038–44. doi: 10.1055/s-0035-1548729
2. Deprest J, Brady P, Nicolaides K, Benachi A, Berg C, Vermeesch J, et al. Prenatal management of the fetus with isolated congenital diaphragmatic hernia in the era of the TOTAL trial. Semin Fetal Neonatal Med. (2014) 19:338–48. doi: 10.1016/j.siny.2014.09.006
3. Steinhorn RH, Abman SH. 52 - Persistent pulmonary hypertension. In: Gleason CA, Juul SE, editors. Avery's Diseases of the Newborn. 10th Ed. Philadelphia, PA: Elsevier (2018). p. 768–78.e3. doi: 10.1016/B978-0-323-40139-5.00052-8
4. Pierro M, Thebaud B. Understanding and treating pulmonary hypertension in congenital diaphragmatic hernia. Semin Fetal Neonatal Med. (2014) 19:357–63. doi: 10.1016/j.siny.2014.09.008
5. Snoek KG, Greenough A, Van Rosmalen J, Capolupo I, Schaible T, Ali K, et al. Congenital diaphragmatic hernia: 10-year evaluation of survival, extracorporeal membrane oxygenation, and foetoscopic endotracheal occlusion in four high-volume centres. Neonatology. (2018) 113:63–8. doi: 10.1159/000480451
6. Coughlin MA, Werner NL, Gajarski R, Gadepalli S, Hirschl R, Barks J, et al. Prenatally diagnosed severe CDH: mortality and morbidity remain high. J Pediatr Surg. (2016) 51:1091–5. doi: 10.1016/j.jpedsurg.2015.10.082
7. Bagolan P, Morini F. Long-term follow up of infants with congenital diaphragmatic hernia. Semin Pediatr Surg. (2007) 16:134–44. doi: 10.1053/j.sempedsurg.2007.01.009
8. American Academy of Pediatrics Section on Surgery; American Academy of Pediatrics Committee on Fetus and Newborn Lally KP Engle W. Postdischarge follow-up of infants with congenital diaphragmatic hernia. Pediatrics. (2008) 121:627–32. doi: 10.1542/peds.2007-3282
9. Rocha G, Azevedo I, Pinto JC, Guimarães H. Follow-up of the survivors of congenital diaphragmatic hernia. Early Hum Dev. (2012) 88:255–8. doi: 10.1016/j.earlhumdev.2011.08.025
10. Chen C, Jeruss S, Chapman JS, Terrin N, Tighiouart H, Glassman E, et al. Long-term functional impact of congenital diaphragmatic hernia repair on children. J Pediatr Surg. (2007) 42:657–65. doi: 10.1016/j.jpedsurg.2006.12.013
11. Patel N, Kipfmueller F. Cardiac dysfunction in congenital diaphragmatic hernia: pathophysiology, clinical assessment, and management. Semin Pediatr Surg. (2017) 26:154–8. doi: 10.1053/j.sempedsurg.2017.04.001
12. Rafat N, Schaible T. Extracorporeal membrane oxygenation in congenital diaphragmatic hernia. Front Pediatr. (2019) 7:336. doi: 10.3389/fped.2019.00336
13. Dassios T, Curley A, Krokidis M, Morley C, Ross-Russell R. Correlation of radiographic thoracic area and oxygenation impairment in bronchopulmonary dysplasia. Respir Physiol Neurobiol. (2016) 220:40–5. doi: 10.1016/j.resp.2015.09.009
14. May C, Prendergast M, Salman S, Rafferty GF, Greenough A. Chest radiograph thoracic areas and lung volumes in infants developing bronchopulmonary dysplasia. Pediatr Pulmonol. (2009) 44:80–5. doi: 10.1002/ppul.20952
15. Dimitriou G, Greenough A, Kavvadia V, Shute M, Karani J. A radiographic method for assessing lung area in neonates. Br J Radiol. (1999) 72:335–8. doi: 10.1259/bjr.72.856.10474492
16. Dimitriou G, Greenough A, Davenport M, Nicolaides K. Prediction of outcome by computer-assisted analysis of lung area on the chest radiograph of infants with congenital diaphragmatic hernia. J Pediatr Surg. (2000) 35:489–93. doi: 10.1016/S0022-3468(00)90219-7
17. Amodeo I, Raffaeli G, Pesenti N, Macchini F, Condò V, Borzani I, et al. The NeoAPACHE study protocol i: assessment of the radiographic pulmonary area and long-term respiratory function in newborns with congenital diaphragmatic hernia. Front Pediatr. (2020) 8:581809. doi: 10.3389/fped.2020.581809
18. Dassios T, Ali K, Makin E, Bhat R, Krokidis M, Greenough A. Prediction of mortality in newborn infants with severe congenital diaphragmatic hernia using the chest radiographic thoracic area. Pediatr Crit Care Med Soc Crit Care Med. (2019) 20:534–9. doi: 10.1097/PCC.0000000000001912
19. Putnam LR, Gupta V, Tsao K, Davis CF, Lally PA, Lally KP, et al. Factors associated with early recurrence after congenital diaphragmatic hernia repair. J Pediatr Surg. (2017) 52:928–32. doi: 10.1016/j.jpedsurg.2017.03.011
20. Tsai J, Sulkowski J, Adzick NS, Hedrick HL, Flake AW. Patch repair for congenital diaphragmatic hernia: is it really a problem? J Pediatr Surg. (2012) 47:637–41. doi: 10.1016/j.jpedsurg.2011.11.054
21. Al-Iede MM, Karpelowsky J, Fitzgerald DA. Recurrent diaphragmatic hernia: modifiable and non-modifiable risk factors. Pediatr Pulmonol. (2016) 51:394–401. doi: 10.1002/ppul.23305
22. Fisher JC, Haley MJ, Ruiz-Elizalde A, Stolar CJ, Arkovitz MS. Multivariate model for predicting recurrence in congenital diaphragmatic hernia. J Pediatr Surg. (2009) 44:1173–80. doi: 10.1016/j.jpedsurg.2009.02.043
23. Moss RL, Chen CM, Harrison MR. Prosthetic patch durability in congenital diaphragmatic hernia: a long-term follow-up study. J Pediatr Surg. (2001) 36:152–4. doi: 10.1053/jpsu.2001.20037
24. Nagata K, Usui N, Terui K, Takayasu H, Goishi K, Hayakawa M, et al. Risk factors for the recurrence of the congenital diaphragmatic hernia—report from the long-term follow-up study of Japanese CDH study group. Eur J Pediatr Surg. (2015) 25:9–14. doi: 10.1055/s-0034-1395486
25. Leeuwen L, Fitzgerald DA. Congenital diaphragmatic hernia. J Paediatr Child Health. (2014) 50:667–73. doi: 10.1111/jpc.12508
26. Dillon PW, Cilley RE, Mauger D, Zachary C, Meier A. The relationship of pulmonary artery pressure and survival in congenital diaphragmatic hernia. J Pediatr Surg. (2004) 39:307–12. doi: 10.1016/j.jpedsurg.2003.11.010
27. Kitagawa M, Hislop A, Boyden E, Reid L. Lung hypoplasia in congenital diaphragmatic hernia a quantitative study of airway, artery, and alveolar development. Br J Surg. (1971) 58:342–6. doi: 10.1002/bjs.1800580507
28. Russo FM, De Coppi P, Allegaert K, Toelen J, van der Veeken L, Attilakos G, et al. Current and future antenatal management of isolated congenital diaphragmatic hernia. Semin Fetal Neonatal Med. (2017) 22:383–90. doi: 10.1016/j.siny.2017.11.002
29. Van der Veeken L, Russo FM, De Catte L, Gratacos E, Benachi A, Ville Y, et al. Fetoscopic endoluminal tracheal occlusion and reestablishment of fetal airways for congenital diaphragmatic hernia. Gynecol Surg. (2018) 15:9. doi: 10.1186/s10397-018-1041-9
30. Snoek KG, Reiss IK, Greenough A, Capolupo I, Urlesberger B, Wessel L, et al. Standardized postnatal management of infants with congenital diaphragmatic hernia in Europe: the CDH EURO consortium consensus-2015 update. Neonatology. (2016) 110:66–74. doi: 10.1159/000444210
31. Ito M, Terui K, Nagata K, Yamoto M, Shiraishi M, Okuyama H, et al. Clinical guidelines for the treatment of congenital diaphragmatic hernia. Pediatr Int. (2021) 63:371–90. doi: 10.1111/ped.14473
32. Boloker J, Bateman DA, Wung JT, Stolar CJ. Congenital diaphragmatic hernia in 120 infants treated consecutively with permissive hypercapnea/spontaneous respiration/elective repair. J Pediatr Surg. (2002) 37:357–66. doi: 10.1053/jpsu.2002.30834
33. Rozmiarek AJ, Qureshi FG, Cassidy L, Ford HR, Hackam DJ. Factors influencing survival in newborns with congenital diaphragmatic hernia: the relative role of timing of surgery. J Pediatr Surg. (2004) 39:821–4. doi: 10.1016/j.jpedsurg.2004.02.010
34. Tsao K, Lally KP. The Congenital Diaphragmatic Hernia Study Group: a voluntary international registry. Semin Pediatr Surg. (2008) 17:90–7. doi: 10.1053/j.sempedsurg.2008.02.004
35. Hollinger LE, Buchmiller TL. Long term follow-up in congenital diaphragmatic hernia. Semin Perinatol. (2020) 44:151171. doi: 10.1053/j.semperi.2019.07.010
36. Harting MT. Congenital diaphragmatic hernia-associated pulmonary hypertension. Semin Pediatr Surg. (2017) 26:147–53. doi: 10.1053/j.sempedsurg.2017.04.008
37. Rowe DH, Stolar CJ. Recurrent diaphragmatic hernia. Semin Pediatr Surg. (2003) 12:107–9. doi: 10.1016/S1055-8586(02)00020-3
38. Jani J, Nicolaides K, Keller R, Benachi A, Peralta C, Favre R, et al. Observed to expected lung area to head circumference ratio in the prediction of survival in fetuses with isolated diaphragmatic hernia. Ultrasound Obstetr Gynecol. (2007) 30:67–71. doi: 10.1002/uog.4052
39. Jani J, Nicolaides KH, Gratacos E, Valencia C, Doné E, Martinez JM, et al. Severe diaphragmatic hernia treated by fetal endoscopic tracheal occlusion. Ultrasound Obstetr Gynecol. (2009) 34:304–10. doi: 10.1002/uog.6450
40. Doné E, Gratacos E, Nicolaides K, Allegaert K, Valencia C, Castañon M, et al. Predictors of neonatal morbidity in fetuses with severe isolated congenital diaphragmatic hernia undergoing fetoscopic tracheal occlusion. Ultrasound Obstetr Gynecol. (2013) 42:77–83. doi: 10.1002/uog.12445
41. Ali K, Dassios T, Khaliq SA, Williams EE, Tamura K, Davenport M, et al. Outcomes of infants with congenital diaphragmatic hernia by side of defect in the FETO era. Pediatr Surg Int. (2019) 35:743–7. doi: 10.1007/s00383-019-04484-3
42. Jawaid W, Qasem E, Jones M, Shaw N, Losty P. Outcomes following prosthetic patch repair in newborns with congenital diaphragmatic hernia. Br J Surg. (2013) 100:1833–7. doi: 10.1002/bjs.9306
43. Raffaeli G, Ghirardello S, Vanzati M, Baracetti C, Canesi F, Conigliaro F, et al. Start a neonatal extracorporeal membrane oxygenation program: a multistep team training. Front Pediatr. (2018) 6:151. doi: 10.3389/fped.2018.00151
44. Wild KT, Rintoul N, Kattan J, Gray B, Keene S, Best D, et al. Extracorporeal Life Support Organization (ELSO): guidelines for neonatal respiratory failure. Asaio Journal. (2020) 66:463–70.
Keywords: congenital diaphragmatic hernia, radiographic lung area, lung hypoplasia, pulmonary hypertension, mortality, recurrence of the hernia, FETO
Citation: Amodeo I, Pesenti N, Raffaeli G, Macchini F, Condò V, Borzani I, Persico N, Fabietti I, Bischetti G, Colli AM, Ghirardello S, Gangi S, Colnaghi M, Mosca F and Cavallaro G (2021) NeoAPACHE II. Relationship Between Radiographic Pulmonary Area and Pulmonary Hypertension, Mortality, and Hernia Recurrence in Newborns With CDH. Front. Pediatr. 9:692210. doi: 10.3389/fped.2021.692210
Received: 07 April 2021; Accepted: 14 June 2021;
Published: 12 July 2021.
Edited by:
Thomas Schaible, University of Heidelberg, GermanyReviewed by:
Carmen Mesas Burgos, Karolinska Institutet (KI), SwedenArno Van Heijst, Self-Employed, Millingen aan de Rijn, Netherlands
Copyright © 2021 Amodeo, Pesenti, Raffaeli, Macchini, Condò, Borzani, Persico, Fabietti, Bischetti, Colli, Ghirardello, Gangi, Colnaghi, Mosca and Cavallaro. This is an open-access article distributed under the terms of the Creative Commons Attribution License (CC BY). The use, distribution or reproduction in other forums is permitted, provided the original author(s) and the copyright owner(s) are credited and that the original publication in this journal is cited, in accordance with accepted academic practice. No use, distribution or reproduction is permitted which does not comply with these terms.
*Correspondence: Giacomo Cavallaro, giacomo.cavallaro@policlinico.mi.it
†These authors share first authorship