- Pediatric Pulmonology and Cystic Fibrosis Unit, Hippokration Hospital, Aristotle University of Thessaloniki, Thessaloniki, Greece
As Cystic Fibrosis (CF) treatment advances, research evidence has highlighted the value and applicability of Lung Clearance Index and Cardiopulmonary Exercise Testing as endpoints for clinical trials. In the context of these new endpoints for CF trials, we have explored the use of these two test outcomes for routine CF care. In this review we have presented the use of these methods in assessing disease severity, disease progression, and the efficacy of new interventions with considerations for future research.
Introduction
Cystic Fibrosis (CF) treatment and patient management has improved dramatically over the last 20 years. The rise of new therapies and effective newborn screening has led to an extraordinary increase in overall survival and quality of life. Despite these improvements in clinical practice, the need for methods that can detect early pathological alterations and direct management remains important.
Spirometry is considered the gold standard endpoint for evaluating CF lung disease. Despite its catholic implication for routine CF assessment, it is not feasible from an early age. In children who are able to perform effort dependent lung function tests, spirometry has low sensitivity in detecting early structural and functional alterations (1). This inability to detect early-stage disease results in a “diagnostic gap” (2, 3) that can be crucial to detect if subsequent patient deterioration is to be avoided.
Cystic fibrosis clinicians and researchers have tried to fill this gap with methods that provide early and reliable deterioration assessment. The Multiple Breath Washout (MBW) test with Lung Clearance Index (LCI) and Cardio-Pulmonary Exercise Testing (CPET) have shown promise in detecting early changes in respiratory physiology.
Lung Clearance Index, LCI
Lung Clearance Index (LCI) is a measure of ventilation distribution inhomogeneity. It can be calculated with the Multiple Breath Washout Method (MBW). The above method measures the clearance of an inert gas (N2 or SF6) from the lungs during tidal breathing. The greater inhomogeneity of ventilation, the higher LCI values are calculated (4). LCI sensitively detects pathology within the peripheral airways (5). This means it can provide information about the “silent lung zone,” the lung compartments whose pathology is not detectable by conventional lung function tests such as spirometry (5, 6). LCI testing is of great value for lung diseases such as CF, for which early detection of structural damages and immediate interventions are crucial to prevent disease progression (7).
MBW is feasible from a young age to adulthood. It requires only passive cooperation with tidal breathing (8, 9). The success rate of the test is 72–99% except for infants and young children below 3.5 years of age, who might need sedation to achieve acceptable measurements. In experienced centers the test can still be used successfully in the majority of these younger children. In experienced centers, over 90% of children aged over 6 year can successfully complete this test and the success rate is even higher amongst adults (10–14).
Kent et al. have reviewed the reliability of LCI and found that the mean coefficient of variation (CV) and the intra-class correlation coefficient (ICC) for LCI measurements within one session were acceptable in both healthy controls and CF cases (15). Few studies have assessed the validity of LCI. Those that have been published demonstrated that LCI was able to distinguish CF subjects from healthy control subjects (16–21). Additionally, LCI could differentiate the disease severity among CF patients with mild to moderate lung disease as revealed by structural changes on high-resolution computed tomography (HRCT) and the patient's microbiological status (3, 7, 22–25). LCI is not well-tolerated by individuals with very severe lung damage, because of the time it takes to complete a measurement. For such individuals, LCI values do not accurately reflect the disease severity because of either totally obstructed or poorly ventilated lung units that do not participate in ventilation distribution. For such patients, spirometry may be a preferable option although this can also plateau at low levels in those with more severe disease (6).
When considering reference values, while LCI was considered an age-independent measure, the current literature suggests a different upper limit of normal (ULN) that is age dependent. The ULN in recent studies has been variably suggested as being; (i) 7.8–8.2 for infants (21, 26–28), (ii) 7.4–7.8 for preschoolers (26, 29), and (iii) 7.2–7.9 for school-aged children (23, 30, 31). Such values might well-depend on the particular equipment used.
Assessment Tool for Disease Severity
Spirometry has been a routine method for assessing lung function but is insensitive in detecting early airway damage (3, 32). In contrast, HRCT detects structural lung damage even in asymptomatic infants and children with normal spirometry (3, 33). Its use however is limited because of the necessary radiation exposure that occurs with scanning.
The superiority of MBW compared to spirometry regarding the sensitivity in detecting early demonstration of lung disease has been demonstrated in a large number of studies (3, 21, 23, 29, 31, 34, 35). In early stages of CF lung disease, many cases with normal FEV1% had abnormal LCI (3).
LCI also showed high sensitivity (92.3%) and positive predictive value (97.3%) in detecting structural damage as revealed by HRCT. A normal LCI can, in most cases, exclude the presence of HRCT abnormalities (3, 22). LCI has been found to relate in particular to the extent of bronchiectasis, and the presence of mucus plugging and emphysema (36). Such findings suggest that routine use of LCI is a reliable alternative to CT as a first line investigation to exclude lung damage whilst minimizing radiation exposure. Some studies recently investigated the performance of Magnetic Resonance Imaging (MRI) using hyperpolarized gas among patients with CF and also found a strong association between abnormalities detected and elevations in LCI (37–41).
LCI values have been investigated in relation to cough swab and sputum culture results among CF patients (16, 29, 42, 43). Patients with chronic lung infection with Aspergillus fumigatus, Pseudomonas aeruginosa, and Stenotrophomonas maltophilia had higher LCI levels than those with normal oral flora (44, 45). LCI values were associated with chronic infection with known CF pathogens (16) and pathogen load (42). Spirometry, LCI, and CT measures were all worse in patients colonized with P. aeruginosa compared to Staphylococcus aureus. It would appear that infection with the latter did not affect the subject's airways as significantly (44, 46, 47).
Ventilation inhomogeneity has been used to assess impacts on the daily life of CF patients. LCI has been shown to be predictive of exercise intolerance as assessed by cardiopulmonary exercise testing (48, 49). Chelabi et al. supported the utility of LCI to predict exercise limitation in children with normal FEV1 (50). Recently, Papale et al. evaluated the ability of LCI to predict nocturnal hypoxemia among CF patients and found that LCI was predictive of such abnormalities (AUC: 0.96, Youden index: 0.79) in stable patients with mild to moderate disease, FEV1 was predictive only in patient with more severe airways disease (AUC: 0.71) (51).
Assessment Tool for Disease Progression
LCI can be used to assess airway disease in infancy. Higher LCI, measured shortly after birth, correlated with a greater respiratory rate during the first year of life (52). Additionally, LCI values at the age of 3 months were predictive of LCI measurements during the first year of life (53). Normal LCI tended to remain stable (53) whereas those with raised levels tended to track at a higher level (54) despite there being minimal structural changes in this age group (55). Newly diagnosed patients discovered because of clinical symptoms had higher LCI values than those diagnosed through newborn screening (53). Abnormal LCI in preschool children is associated with many clinical parameters including F508del homozygous genotype, P. aeruginosa colonization and nebulised dornase alpha use. It is also predictive of spirometry in later childhood (56), as well as LCI during school age (57) and adolescence (58).
A significant benefit of LCI has been predicting the risk of first isolation of P. aeruginosa in previously non-colonized patients. An increase of LCI by 1.18 could predict first colonization (sensitivity 52%, specificity 70%), despite 81% of such patients being free of respiratory symptoms and an almost stable FEV1% (59). LCI values have also been shown to be predictive of the risk of pulmonary exacerbations with a cut-off of LCI change of 1.37 predicting the likelihood of such clinical events (sensitivity: 47.8%, specificity: 79.6%) (44). Studies suggest that a larger proportion of exacerbation events had a deterioration of LCI compared to a decline in FEV1% (41.7 vs. 30.0%, p:0.012) (60). Vermeulen et al. showed that the baseline LCI value of a patient could also predict the risk of developing a pulmonary exacerbation during the following year. Higher baseline LCI values were associated with earlier pulmonary exacerbation (61).
Assessment Tool of Interventions Efficacy
A remarkable role of LCI is its utility in estimating the effectiveness of therapeutic interventions. LCI improved significantly in CF patients after receiving IV antibiotic treatment for a pulmonary exacerbation. The improvement of LCI was greater than the change in spirometry (25 vs. 15%), but neither LCI nor FEV1 recovered to baseline values (60, 62, 63). In contrast there were no significant changes in LCI in studies assessing the effects of nebulized tobramycin (28-day on/off regime) (64, 65). The positive impact of inhalation of dornase alpha (66) and hypertonic Saline (67–69) has been demonstrated using LCI measurements. More recently LCI has been used as a primary outcome measure to assess the efficacy of CFTR potentiators (ivacaftor, lumacaftor/ivacaftor, tezacaftor/ivacaftor) (70–72).
Although the value of LCI has been established in an increasing number of CF services, the main limitations on its more widespread use is that is a time-consuming test requiring specialist equipment and appropriately trained personnel.
Cardio-Pulmonary Exercise Testing
Cardio-pulmonary exercise testing (CPET) provides a comprehensive assessment of pulmonary, cardiovascular and muscular function. Its principle is quite simple; implementing maximal exercise in a patient while monitoring their cardiac function, pulmonary gas exchange, and progressive muscle oxidation. The test can provide useful data about early pathological alterations in each of these compartments and information about the extent to which these abnormalities impact on exercise performance.
Exercise Testing on a cycle ergometer or treadmill is feasible from the age of around 6 years (73). The test procedure varies in terms of duration depending on the protocol used and the information that need to be obtained (74). The suggested protocol for routine clinical practice does not usually last more than 10–15 min (73, 75) and results are immediately available for interpretation. The test protocol for CF patients has been standardized and reference value equations are available even for young children (76).
CPET's use in CF was mainly research-oriented until, in early 1992, when Nixon et al. demonstrated that peak oxygen uptake during a maximal cardiopulmonary exercise test (the amount of oxygen a CF patient's lung can absorb during maximal exertion) is a prognostic factor for survival. Patients with VO2peak >80% predicted showed excellent 8-year survival, whereas patients with low maximal oxygen uptake (VO2 peak <60%) had worse outcomes (77).
CPET has since been used for clinical and research purposes in CF with studies highlighting its role in assessing overall disease progression. CPET parameters have also been shown to reflect structural and functional abnormalities and in particular, disease prognosis.
Assessment Tool of Structural, Functional Abnormalities, and Disease Severity
Cystic fibrosis is characterized by progressive inflammation and impaired mucus excretion (78). This vicious cycle leads to airway remodeling and impaired gas exchange (79). Exercise testing is more sensitive than spirometry in detecting structural abnormalities, as shown by High Resolution Computed Tomography (HRCT) both in adults (80) and CF adolescents (81). CPET is also indicative of functional alterations taking place in CF lungs. CPET parameters have been shown to be associated with ventilation inhomogeneity (48) and reflect increased dead space ventilation in CF patients as the disease progresses (82). Van de Weert et al. found that low exercise capacity was associated with chronic P. aeruginosa colonization and changes in immunoglobulin levels in CF adolescents (83).
Assessment Tool for Disease Progression and Survival
As Cystic Fibrosis lung disease progresses and pseudomonas colonization is established, exercise testing parameters also deteriorate (84). However, the most clinically relevant contribution of CPET to clinical practice is the implications of test results for long term survival. Aerobic fitness as indicated by VO2 Max has a key role in determining prognosis (85, 86). In addition other indices including VE/VO2 and VE/VCO2 have also proved useful as predictors of death or lung transplantation at 10-year follow-up (87, 88).
Assessment Tool of Intervention Efficacy
Exercise testing has shown additional benefits over spirometry in characterizing for example the efficacy of therapeutic interventions to treat pulmonary exacerbations (89, 90). It has also been used as an outcome measure for assessing the effectiveness of CFTR modulators (87, 91, 92) and as an incentive for as well as measure of the effectiveness of pulmonary rehabilitation (93–96). It has also been used for exercise prescription (97–100), pre- and post- transplantation assessment (101–103) and as a primary and secondary endpoint measure in clinical trials (95, 104, 105).
Conclusion
LCI and CPET parameters are sensitive measures for detecting early lung disease, and predicting outcomes including colonization with infecting pathogens, pulmonary exacerbations and long term survival, Table 1. These findings have led to the use of these methods for routine patient assessment in many CF centers. Whilst data confirming the sensitivity of LCI and CPET in the early detection of CF lung disease are increasing, there is still a need for more information about validity, age specific reference values and their best use as tools to measure the benefits of clinical interventions. Future research might usefully afford greater insight into the best use of these measures in assessing the needs of increasingly patients with CF.
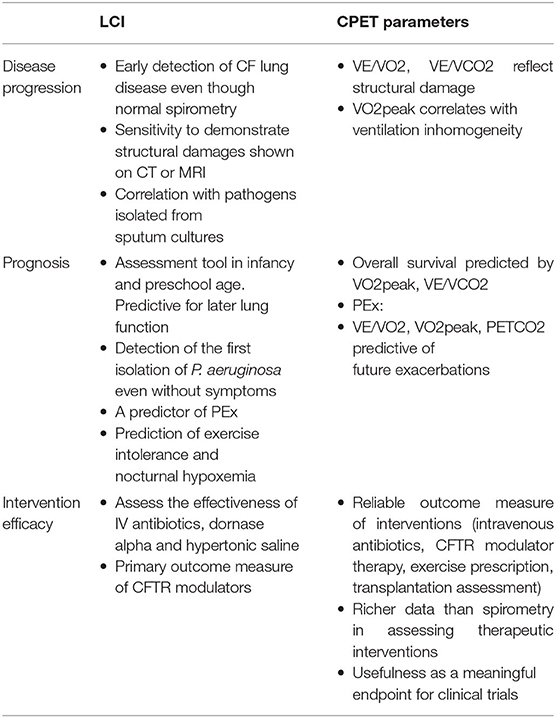
Table 1. The role of LCI and CPET parameters in disease progression, prognosis, and intervention efficacy.
Author Contributions
EH, AK, VA, IT, E-AC, MG, FK, and JT have made contributions to the design, editing, and writing of this manuscript. All authors contributed to the article and approved the submitted version.
Conflict of Interest
The authors declare that the research was conducted in the absence of any commercial or financial relationships that could be construed as a potential conflict of interest.
References
1. Taylor-Robinson D, Whitehead M, Diderichsen F, Olesen HV, Pressler T, Smyth RL, et al. Understanding the natural progression in %FEV1 decline in patients with cystic fibrosis: a longitudinal study. Thorax. (2012) 67:860–6. doi: 10.1136/thoraxjnl-2011-200953
2. Breuer O, Caudri D, Stick S, Turkovic L. Predicting disease progression in cystic fibrosis. Expert Rev Respir Med. (2018) 12:905–17. doi: 10.1080/17476348.2018.1519400
3. Ellemunter H, Fuchs SI, Unsinn KM, Freund MC, Waltner-Romen M, Steinkamp G, et al. Sensitivity of lung clearance index and chest computed tomography in early CF lung disease. Respir Med. (2010) 104:1834–42. doi: 10.1016/j.rmed.2010.06.010
4. Robinson PD, Latzin P, Verbanck S, Hall GL, Horsley A, Gappa M, et al. Consensus statement for inert gas washout measurement using multiple- and single- breath tests. Eur Respir J. (2013) 41:507–22. doi: 10.1183/09031936.00069712
5. Robinson PD, Goldman MD, Gustafsson PM. Inert gas washout: theoretical background and clinical utility in respiratory disease. Respiration. (2009) 78:339–55. doi: 10.1159/000225373
6. Horsley A. Lung clearance index in the assessment of airways disease. Respir Med. (2009) 103:793–9. doi: 10.1016/j.rmed.2009.01.025
7. Hall GL, Logie KM, Parsons F, Schulzke SM, Nolan G, Murray C, et al. Air trapping on chest CT is associated with worse ventilation distribution in infants with cystic fibrosis diagnosed following newborn screening. PLoS ONE. (2011) 6:e23932. doi: 10.1371/journal.pone.0023932
8. Beydon N DS, Lombardi E, Allen JL, Arets HG, Aurora P, Bisgaard H, et al. An official American Thoracic Society/European Respiratory Society statement: pulmonary function testing in preschool children. Am J Respir Crit Care Med. (2007) 175:1304–45. doi: 10.1164/rccm.200605-642ST
9. Gustafsson P. Inert gas washout in preschool children. Paediatr Respir Rev. (2005) 6:239–45. doi: 10.1016/j.prrv.2005.09.009
10. Armstrong D, Bayfield K, Alton E, Boyd A, Cunningham S, Elgmati H, et al. P209 standardisation of lung clearance index in a multicentre clinical trial. Thorax. (2014) 69(Suppl. 2):A168–9. doi: 10.1136/thoraxjnl-2014-206260.338
11. Downing B, Irving S, Bingham Y, Fleming L, Bush A, Saglani S. Feasibility of lung clearance index in a clinical setting in pre-school children. Eur Respir J. (2016) 48:1074–80. doi: 10.1183/13993003.00374-2016
12. O'Neill K, Saunders C. New directions on lung clearance index variability and feasibility. J Cyst Fibros. (2018) 17:137–9. doi: 10.1016/j.jcf.2018.02.010
13. Stahl M, Graeber SY, Joachim C, Barth S, Ricklefs I, Diekmann G, et al. Three-center feasibility of lung clearance index in infants and preschool children with cystic fibrosis and other lung diseases. J Cyst Fibros. (2018) 17:249–55. doi: 10.1016/j.jcf.2017.08.001
14. Stanojevic S, Davis SD, Retsch-Bogart G, Webster H, Davis M, Johnson RC, et al. Progression of lung disease in preschool patients with cystic fibrosis. Am J Respir Crit Care Med. (2017) 195:1216–25. doi: 10.1164/rccm.201610-2158OC
15. Kent L, Reix P, Innes JA, Zielen S, Le Bourgeois M, Braggion C, et al. Lung clearance index: evidence for use in clinical trials in cystic fibrosis. J Cyst Fibros. (2014) 13:123–38. doi: 10.1016/j.jcf.2013.09.005
16. Belessis Y, Dixon B, Hawkins G, Pereira J, Peat J, MacDonald R, et al. Early cystic fibrosis lung disease detected by bronchoalveolar lavage and lung clearance index. Am J Respir Crit Care Med. (2012) 185:862–73. doi: 10.1164/rccm.201109-1631OC
17. Fuchs SI, Ellemunter H, Eder J, Mellies U, Grosse-Onnebrink J, Tümmler B, et al. feasibility and variability of measuring the lung clearance index in a multi-center setting. Pediatr Pulmonol. (2012) 47:649–57. doi: 10.1002/ppul.21610
18. Gustafsson PM. Peripheral airway involvement in CF and asthma compared by inert gas washout. Pediatr Pulmonol. (2007) 42:168–76. doi: 10.1002/ppul.20554
19. Hoo AF, Thia LP, Nguyen TT, Bush A, Chudleigh J, Lum S, et al. Lung function is abnormal in 3-month-old infants with cystic fibrosis diagnosed by newborn screening. Thorax. (2012) 67:874–81. doi: 10.1136/thoraxjnl-2012-201747
20. Horsley AR, Gustafsson PM, Macleod KA, Saunders C, Greening AP, Porteous DJ, et al. Lung clearance index is a sensitive, repeatable and practical measure of airways disease in adults with cystic fibrosis. Thorax. (2008) 63:135–40. doi: 10.1136/thx.2007.082628
21. Lum S, Gustafsson P, Ljungberg H, Hülskamp G, Bush A, Carr SB, et al. Early detection of cystic fibrosis lung disease: multiple-breath washout versus raised volume tests. Thorax. (2007) 62:341–7. doi: 10.1136/thx.2006.068262
22. Gustafsson PM, De Jong PA, Tiddens HAWM, Lindblad A. Multiple-breath inert gas washout and spirometry versus structural lung disease in cystic fibrosis. Thorax. (2008) 63:129–34. doi: 10.1136/thx.2007.077784
23. Gustafsson PM AP, Lindblad A. Evaluation of ventilation maldistribution as an early indicator of lung disease in children with cystic fibrosis. Eur Respir J. (2003) 22:972–9. doi: 10.1183/09031936.03.00049502
24. Hatziagorou E, Avramidou V, Georgopoulou V, Kirvasillis F, Tsanakas JN. WS20.4 is lung clearance index (LCI) affected by the severity of lung disease in CF? J Cyst Fibros. (2014) 13:S39. doi: 10.1016/S1569-1993(14)60123-5
25. Owens CM, Aurora P, Stanojevic S, Bush A, Wade A, Oliver C, et al. Lung Clearance Index and HRCT are complementary markers of lung abnormalities in young children with CF. Thorax. (2011) 66:481–8. doi: 10.1136/thx.2010.150375
26. Aurora P KW, Stocks J. Gas mixing efficiency from birth to adulthood measured by multiple-breath washout. Respir Physiol Neurobiol. (2005) 148:125–39. doi: 10.1016/j.resp.2005.05.027
27. Fuchs O, Latzin P, Thamrin C, Stern G, Frischknecht P, Singer F, et al. Normative data for lung function and exhaled nitric oxide in unsedated healthy infants. Eur Respir J. (2011) 37:1208–16. doi: 10.1183/09031936.00125510
28. Hülskamp G, Lum S, Stocks J, Wade A, Hoo AF, Costeloe K, et al. Association of prematurity, lung disease and body size with lung volume and ventilation inhomogeneity in unsedated neonates: a multicentre study. Thorax. (2009) 64:240–5. doi: 10.1136/thx.2008.101758
29. Aurora P, Bush A, Gustafsson P, Oliver C, Wallis C, Price J, et al. Multiple-breath washout as a marker of lung disease in preschool children with cystic fibrosis. Am J Respir Crit Care Med. (2005) 171:249–56. doi: 10.1164/rccm.200407-895OC
30. Anagnostopoulou P, Latzin P, Jensen R, Stahl M, Harper A, Yammine S, et al. Normative data for multiple breath washout outcomes in school-aged Caucasian children. Eur Respir J. (2020) 55:1901302. doi: 10.1183/13993003.01302-2019
31. Aurora P, Gustafsson P, Bush A, Lindblad A, Oliver C, Wallis CE, et al. Multiple breath inert gas washout as a measure of ventilation distribution in children with cystic fibrosis. Thorax. (2004) 59:1068–73. doi: 10.1136/thx.2004.022590
32. Macklem PT. The physiology of small airways. Am J Respir Crit Care Med. (1998) 157:S181–3. doi: 10.1164/ajrccm.157.5.rsaa-2
33. Long FR WR, Castile RG. Structural airway abnormalities in infants and young children with cystic fibrosiss. J Pediatr. (2004) 144:154–61. doi: 10.1016/j.jpeds.2003.09.026
34. Gangell CL, Hall GL, Stick SM, Sly PD. Lung function testing in preschool-aged children with cystic fibrosis in the clinical setting. Pediatr Pulmonol. (2010) 45:419–33. doi: 10.1002/ppul.21192
35. Kraemer R, Blum A, Schibler A, Ammann RA, Gallati S. Ventilation inhomogeneities in relation to standard lung function in patients with cystic fibrosis. Am J Respir Crit Care Med. (2005) 171:371–8. doi: 10.1164/rccm.200407-948OC
36. Avramidou V, Hatziagorou E, Kampouras A, Georgopoulou V, Kirvasilis F, Tsanakas J. Is Lung Clearance Index (LCI) affected by the severity of lung disease in CF?. J Pulm Respir Med. (2017) 6:392.
37. Nyilas S, Bauman G, Sommer G, Stranzinger E, Pusterla O, Frey U, et al. Novel magnetic resonance technique for functional imaging of cystic fibrosis lung disease. Eur Respir J. (2017) 50:1701464. doi: 10.1183/13993003.01464-2017
38. Rayment JH, Couch MJ, McDonald N, Kanhere N, Manson D, Santyr G, et al. Hyperpolarised (129)Xe magnetic resonance imaging to monitor treatment response in children with cystic fibrosis. Eur Respir J. (2019) 53:1802188. doi: 10.1183/13993003.02188-2018
39. Smith L, Marshall H, Aldag I, Horn F, Collier G, Hughes D, et al. Longitudinal assessment of children with mild cystic fibrosis using hyperpolarized gas lung magnetic resonance imaging and lung clearance index. Am J Respir Crit Care Med. (2018) 197:397–400. doi: 10.1164/rccm.201705-0894LE
40. Stahl M, Wielputz MO, Graeber SY, Joachim C, Sommerburg O, Kauczor HU, et al. Comparison of lung clearance index and magnetic resonance imaging for assessment of lung disease in children with cystic fibrosis. Am J Respir Crit Care Med. (2017) 195:349–59. doi: 10.1164/rccm.201604-0893OC
41. Verbanck S, Vanderhelst E. The respective roles of lung clearance index and magnetic resonance imaging in the clinical management of patients with cystic fibrosis. Am J Respir Crit Care Med. (2018) 197:409. doi: 10.1164/rccm.201706-1137LE
42. Singer F, Kieninger E, Abbas C, Yammine S, Fuchs O, Proietti E, et al. Practicability of nitrogen multiple-breath washout measurements in a pediatric cystic fibrosis outpatient setting. Pediatr Pulmonol. (2013) 48:739–46. doi: 10.1002/ppul.22651
43. O'Neill K, Bradley JM, Johnston E, McGrath S, McIlreavey L, Rowan S, et al. reduced bacterial colony count of anaerobic bacteria is associated with a worsening in lung clearance index and inflammation in cystic fibrosis. PLoS ONE. (2015) 10:e0126980. doi: 10.1371/journal.pone.0126980
44. Hatziagorou E, Avramidou V, Kampouras A, Kirvasillis F, Tsanakas J. Clinical value of lung clearance index (LCI) among patients with cystic fibrosis. Eur Respir J. (2014) 44:P1218. doi: 10.1183/13993003.congress-2015.OA3502
45. Walicka-Serzysko K, Postek M, Milczewska J, Sands D. Change in lung clearance index with microbiological status in children with cystic fibrosis. Pediatr Pulmonol. (2019) 54:729–36. doi: 10.1002/ppul.24278
46. Folescu TW, Marques EdA, Boechat MCB, Daltro P, Higa LYS, Cohen RWF. Escore tomográfico em pacientes com fibrose cística colonizados por Pseudomonas aeruginosa ou Staphylococcus aureus. J Bras Pneumol. (2012) 38:41–9. doi: 10.1590/S1806-37132012000100007
47. Kraemer R, Baldwin DN, Ammann RA, Frey U, Gallati S. Progression of pulmonary hyperinflation and trapped gas associated with genetic and environmental factors in children with cystic fibrosis. Respir Res. (2006) 7:138. doi: 10.1186/1465-9921-7-138
48. Avramidou V, Hatziagorou E, Kampouras A, Hebestreit H, Kourouki E, Kirvassilis F, et al. Lung clearance index (LCI) as a predictor of exercise limitation among CF patients. Pediatr Pulmonol. (2017) 53:81–7. doi: 10.1002/ppul.23833
49. Gambazza S, Guarise R, Carta F, Ambrogi F, Mirabella M, Brivio A, et al. Exercise capacity and ventilation inhomogeneity in cystic fibrosis: A cross-sectional study. Pediatr Pulmonol. (2020) 55:394–400. doi: 10.1002/ppul.24525
50. Chelabi R, Soumagne T, Guillien A, Puyraveau M, Degano B. In cystic fibrosis, lung clearance index is sensitive to detecting abnormalities appearing at exercise in children with normal spirometry. Respir Physiol Neurobiol. (2018) 247:9–11. doi: 10.1016/j.resp.2017.08.017
51. Papale M, Parisi GF, Spicuzza L, Licari A, Bongiovanni A, Mulè E, et al. Lung clearance index evaluation in detecting nocturnal hypoxemia in cystic fibrosis patients: toward a new diagnostic tool. Respir Med. (2020) 164:105906. doi: 10.1016/j.rmed.2020.105906
52. Korten I, Kieninger E, Yammine S, Cangiano G, Nyilas S, Anagnostopoulou P, et al. Respiratory rate in infants with cystic fibrosis throughout the first year of life and association with lung clearance index measured shortly after birth. J Cyst Fibros. (2019) 18:118–26. doi: 10.1016/j.jcf.2018.07.002
53. Nguyen TT-D, Thia LP, Hoo A-F, Bush A, Aurora P, Wade A, et al. Evolution of lung function during the first year of life in newborn screened cystic fibrosis infants. Thorax. (2014) 69:910–7. doi: 10.1136/thoraxjnl-2013-204023
54. Linnane BM, Hall GL, Nolan G, Brennan S, Stick SM, Sly PD, et al. Lung function in infants with cystic fibrosis diagnosed by newborn screening. Am J Respir Crit Care Med. (2008) 178:1238–44. doi: 10.1164/rccm.200804-551OC
55. Davies G, Thia LP, Stocks J, Bush A, Hoo AF, Wade A, et al. Minimal change in structural, functional and inflammatory markers of lung disease in newborn screened infants with cystic fibrosis at one year. J Cyst Fibros. (2020) 19:896–901. doi: 10.1016/j.jcf.2020.01.006
56. Hardaker KM, Panda H, Hulme K, Wong A, Coward E, Cooper P, et al. Abnormal preschool Lung Clearance Index (LCI) reflects clinical status and predicts lower spirometry later in childhood in cystic fibrosis. J Cyst Fibros. (2019) 18:721–7. doi: 10.1016/j.jcf.2019.02.007
57. Aurora P, Stanojevic S, Wade A, Oliver C, Kozlowska W, Lum S, et al. Lung clearance index at 4 years predicts subsequent lung function in children with cystic fibrosis. Am J Respir Crit Care Med. (2011) 183:752–8. doi: 10.1164/rccm.200911-1646OC
58. Davies G, Stanojevic S, Raywood E, Duncan JA, Stocks J, Lum S, et al. An observational study of the lung clearance index throughout childhood in cystic fibrosis: early years matter. Eur Respir J. (2020) 56:2000006. doi: 10.1183/13993003.00006-2020
59. Hatziagorou E, Avramidou V, Kouroukli E, Nousia L, Parisi M, Galogavrou M, et al. Can LCI predict new Pseudomonas aeruginosa colonization among CF patients?>. Eur Respir J. (2017) 50(Suppl. 61):OA1793. doi: 10.1183/1393003.congress-2017.OA1793
60. Perrem L, Stanojevic S, Shaw M, Jensen R, McDonald N, Isaac SM, et al. Lung clearance index to track acute respiratory events in school-age children with cystic fibrosis. Am J Respir Crit Care Med. (2020). doi: 10.1164/rccm.202006-2433OC. [Epub ahead of print].
61. Vermeulen F, Proesmans M, Boon M, Havermans T, De Boeck K. Lung clearance index predicts pulmonary exacerbations in young patients with cystic fibrosis. Thorax. (2014) 69:39–45. doi: 10.1136/thoraxjnl-2013-203807
62. Hatziagorou E, Avramidou V, Kirvassilis F, Tsanakas J. Use of lung clearance index to assess the response to intravenous treatment in cystic fibrosis. Hippokratia. (2015) 19:47–52.
63. Sanders DB, Bittner RCL, Rosenfeld M, Hoffman LR, Redding GJ, Goss CH. Failure to recover to baseline pulmonary function after cystic fibrosis pulmonary exacerbation. Am J Respir Crit Care Med. (2010) 182:627–32. doi: 10.1164/rccm.200909-1421OC
64. Hatziagorou E, Avramidou V, Kampouras A, Kirvasillis F, Tsanakas J. Lung Clearance Index (LCI) and FEV1 on the evaluation of the effect of tobramycin solution for inhalation (TIS) among patients with cystic fibrosis. Eur Respir J. (2014) 44(Suppl. 58):91–101.
65. Sutharsan S, Naehrig S, Mellies U, Sieder C, Ziegler J. An 8 week open-label interventional multicenter study to explore the lung clearance index as endpoint for clinical trials in cystic fibrosis patients ≥8 years of age, chronically infected with Pseudomonas aeruginosa. BMC Pulm Med. (2020) 20:167. doi: 10.1186/s12890-020-01201-y
66. Amin R, Subbarao P, Lou W, Jabar A, Balkovec S, Jensen R, et al. The effect of dornase alfa on ventilation inhomogeneity in patients with cystic fibrosis. Eur Respir J. (2011) 37:806–12. doi: 10.1183/09031936.00072510
67. Amin R, Subbarao P, Jabar A, Balkovec S, Jensen R, Kerrigan S, et al. Hypertonic saline improves the LCI in paediatric patients with CF with normal lung function. Thorax. (2010) 65:379–83. doi: 10.1136/thx.2009.125831
68. Subbarao P, Stanojevic S, Brown M, Jensen R, Rosenfeld M, Davis S, et al. Lung clearance index as an outcome measure for clinical trials in young children with cystic fibrosis. a pilot study using inhaled hypertonic saline. Am J Respir Crit Care Med. (2013) 188:456–60. doi: 10.1164/rccm.201302-0219OC
69. Stahl M, Wielpütz MO, Ricklefs I, Dopfer C, Barth S, Schlegtendal A, et al. Preventive Inhalation of Hypertonic Saline in Infants with Cystic Fibrosis (PRESIS). a randomized, double-blind, controlled study. Am J Respir Crit Care Med. (2019) 199:1238–48. doi: 10.1164/rccm.201807-1203OC
70. Davies J, Sheridan H, Bell N, Cunningham S, Davis SD, Elborn JS, et al. Assessment of clinical response to ivacaftor with lung clearance index in cystic fibrosis patients with a G551D-CFTR mutation and preserved spirometry: a randomised controlled trial. Lancet Respir Med. (2013) 1:630–8. doi: 10.1016/S2213-2600(13)70182-6
71. Davies JC, Sermet-Gaudelus I, Naehrlich L, Harris RS, Campbell D, Ahluwalia N, et al. A phase 3, double-blind, parallel-group study to evaluate the efficacy and safety of tezacaftor in combination with ivacaftor in participants 6 through 11 years of age with cystic fibrosis homozygous for F508del or heterozygous for the F508del-CFTR mutation and a residual function mutation. J Cyst Fibros. (2021) 20:68–77. doi: 10.1016/j.jcf.2020.07.023
72. Shaw M, Khan U, Clancy JP, Donaldson SH, Sagel SD, Rowe SM, et al. Changes in LCI in F508del/F508del patients treated with lumacaftor/ivacaftor: results from the prospect study. J Cyst Fibros. (2020) 19:931–3. doi: 10.1016/j.jcf.2020.05.010
73. Radtke T, Crook S, Kaltsakas G, Louvaris Z, Berton D, Urquhart DS, et al. ERS statement on standardisation of cardiopulmonary exercise testing in chronic lung diseases. Eur Respir J. (2019) 28:180101. doi: 10.1183/16000617.0101-2018
74. Fletcher GF, Ades PA, Kligfield P, Arena R, Balady GJ, Bittner VA, et al. Exercise standards for testing and training: a scientific statement from the American Heart Association. Circulation. (2013) 128:873–934. doi: 10.1161/CIR.0b013e31829b5b44
75. Hebestreit H, Arets HG, Aurora P, Boas S, Cerny F, Hulzebos EH, et al. Statement on exercise testing in cystic fibrosis. Respiration. (2015) 90:332–51. doi: 10.1159/000439057
76. Orenstein D. Assessment of Exercise Pulmonary Function. RT ed. Champaign, IL: Human Kinetics (1993).
77. Nixon PA, Orenstein DM, Kelsey SF, Doershuk CF. The prognostic value of exercise testing in patients with cystic fibrosis. N Engl J Med. (1992) 327:1785–8. doi: 10.1056/NEJM199212173272504
78. Brown SD, White R, Tobin P. Keep them breathing: cystic fibrosis pathophysiology, diagnosis, and treatment. Jaapa. (2017) 30:23–7. doi: 10.1097/01.JAA.0000515540.36581.92
79. Regamey N, Jeffery PK, Alton EW, Bush A, Davies JC. Airway remodelling and its relationship to inflammation in cystic fibrosis. Thorax. (2011) 66:624–9. doi: 10.1136/thx.2009.134106
80. Dodd JD, Barry SC, Barry RB, Gallagher CG, Skehan SJ, Masterson JB. Thin-section CT in patients with cystic fibrosis: correlation with peak exercise capacity and body mass index. Radiology. (2006) 240:236–45. doi: 10.1148/radiol.2401050502
81. Hatziagorou E, Kampouras A, Avramidou V, Georgopoulou V, Kirvasilis F, Kontouli K, et al. Exercise responses are related to structural lung damage in CF pulmonary disease. Pediatr Pulmonol. (2016) 51:914–20. doi: 10.1002/ppul.23474
82. Kampouras A, Hatziagorou E, Avramidou V, Georgopoulou V, Kirvassilis F, Hebestreit H, et al. Ventilation efficiency to exercise in patients with cystic fibrosis. Pediatr Pulmonol. (2019) 54:1584–90. doi: 10.1002/ppul.24438
83. van de Weert-van Leeuwen PB, Slieker MG, Hulzebos HJ, Kruitwagen CL, van der Ent CK, Arets HG. Chronic infection and inflammation affect exercise capacity in cystic fibrosis. Eur Respir J. (2012) 39:893–8. doi: 10.1183/09031936.00086211
84. Kampouras A, Hatziagorou E, Avramidou V, Georgopoulou V, Kirvassilis F, Tsanakas J. Does pseudomonas aeruginosa colonization affect exercise capacity in CF? Pulm Med. (2019) 2019:3786245. doi: 10.1155/2019/3786245
85. Vendrusculo FM, Heinzmann-Filho JP, da Silva JS, Perez Ruiz M, Donadio MVF. Peak oxygen uptake and mortality in cystic fibrosis: systematic review and meta-analysis. Respir Care. (2019) 64:91–8. doi: 10.4187/respcare.06185
86. Pianosi P, Leblanc J, Almudevar A. Peak oxygen uptake and mortality in children with cystic fibrosis. Thorax. (2005) 60:50–4. doi: 10.1136/thx.2003.008102
87. Hebestreit H, Hulzebos EHJ, Schneiderman JE, Karila C, Boas SR, Kriemler S, et al. Cardiopulmonary exercise testing provides additional prognostic information in cystic fibrosis. Am J Respir Crit Care Med. (2019) 199:987–95. doi: 10.1164/rccm.201806-1110OC
88. Moorcroft AJ, Dodd ME, Webb AK. Exercise testing and prognosis in adult cystic fibrosis. Thorax. (1997) 52:291–3. doi: 10.1136/thx.52.3.291
89. Robinson PD, Cooper P, Van Asperen P, Fitzgerald D, Selvadurai H. Using index of ventilation to assess response to treatment for acute pulmonary exacerbation in children with cystic fibrosis. Pediatr Pulmonol. (2009) 44:733–42. doi: 10.1002/ppul.20956
90. Cox NS, Follett J, McKay KO. Modified shuttle test performance in hospitalized children and adolescents with cystic fibrosis. J Cyst Fibros. (2006) 5:165–70. doi: 10.1016/j.jcf.2006.02.004
91. Ejiofor LCK, Mathiesen IHM, Jensen-Fangel S, Olesen HV, Skov M, Philipsen LKD, et al. Patients with cystic fibrosis and advanced lung disease benefit from lumacaftor/ivacaftor treatment. Pediatr Pulmonol. (2020) 55:3364–70. doi: 10.1002/ppul.25059
92. Saynor ZL, Barker AR, Oades PJ, Williams CA. The effect of ivacaftor in adolescents with cystic fibrosis (G551D mutation): an exercise physiology perspective. Pediatr Phys Ther. (2014) 26:454–61. doi: 10.1097/PEP.0000000000000086
93. Radtke T, Nevitt SJ, Hebestreit H, Kriemler S. Physical exercise training for cystic fibrosis. Cochrane Database Syst Rev. (2017) 11:Cd002768. doi: 10.1002/14651858.CD002768.pub4
94. Wark PAB, Cookson K, Thiruchelvam T, Brannan J, Dorahy DJ. Lumacaftor/ Ivacaftor improves exercise tolerance in patients with Cystic Fibrosis and severe airflow obstruction. BMC Pulm Med. (2019) 19:106. doi: 10.1186/s12890-019-0866-y
95. Edgeworth D, Keating D, Ellis M, Button B, Williams E, Clark D, et al. Improvement in exercise duration, lung function and well-being in G551D-cystic fibrosis patients: a double-blind, placebo-controlled, randomized, cross-over study with ivacaftor treatment. Clin Sci. (2017) 131:2037–45. doi: 10.1042/CS20170995
96. Ledger SJ, Owen E, Prasad SA, Goldman A, Willams J, Aurora P. A pilot outreach physiotherapy and dietetic quality improvement initiative reduces IV antibiotic requirements in children with moderate-severe cystic fibrosis. J Cyst Fibros. (2013) 12:766–72. doi: 10.1016/j.jcf.2013.01.003
97. Stevens D, Williams CA. Exercise testing and training with the young cystic fibrosis patient. J Sci Med Sport. (2007) 6:286–91.
98. Stevens D, Oades PJ, Armstrong N, Williams CA. A survey of exercise testing and training in UK cystic fibrosis clinics. J Cyst Fibros. (2010) 9:302–6. doi: 10.1016/j.jcf.2010.03.004
99. Selvadurai HC, Blimkie CJ, Meyers N, Mellis CM, Cooper PJ, Van Asperen PP. Randomized controlled study of in-hospital exercise training programs in children with cystic fibrosis. Pediatr Pulmonol. (2002) 33:194–200. doi: 10.1002/ppul.10015
100. Ward N, Stiller K, Holland AE. Exercise as a therapeutic intervention for people with cystic fibrosis. Expert Rev Respir Med. (2019) 13:449–58. doi: 10.1080/17476348.2019.1598861
101. Nguyen S, Leroy S, Cracowski C, Perez T, Valette M, Neviere R, et al. [Prognostic value of clinical exercise testing in adult patients with cystic fibrosis]. Revue des maladies Respiratoires. (2010) 27:219–25. doi: 10.1016/j.rmr.2010.01.009
102. Radtke T, Faro A, Wong J, Boehler A, Benden C. Exercise testing in pediatric lung transplant candidates with cystic fibrosis. Pediatr Transplant. (2011) 15:294–9. doi: 10.1111/j.1399-3046.2010.01471.x
103. Castellani C, Duff AJA, Bell SC, Heijerman HGM, Munck A, Ratjen F, et al. ECFS best practice guidelines: the 2018 revision. J Cyst Fibros. (2018) 17:153–78. doi: 10.1016/j.jcf.2018.02.006
104. Vendrusculo FM, Johnstone Z, Dhouieb E, Donadio MVF, Cunningham S, Urquhart DS. Airway clearance physiotherapy improves ventilatory dynamics during exercise in patients with cystic fibrosis: a pilot study. Arch Dis Child. (2019) 104:37–42. doi: 10.1136/archdischild-2017-314365
Keywords: lung clearance index (LCI), cardiopulmonary exercise testing (CPET), endpoints, cystic fibrosis, disease severity, disease progression
Citation: Hatziagorou E, Kampouras A, Avramidou V, Toulia I, Chrysochoou E-A, Galogavrou M, Kirvassilis F and Tsanakas J (2021) Toward the Establishment of New Clinical Endpoints for Cystic Fibrosis: The Role of Lung Clearance Index and Cardiopulmonary Exercise Testing. Front. Pediatr. 9:635719. doi: 10.3389/fped.2021.635719
Received: 30 November 2020; Accepted: 03 February 2021;
Published: 25 February 2021.
Edited by:
Gary James Connett, University Hospital Southampton NHS Foundation Trust, United KingdomReviewed by:
Zoe Louise Saynor, University of Portsmouth, United KingdomFrancis Gilchrist, University Hospitals of North Midlands NHS Trust, United Kingdom
Copyright © 2021 Hatziagorou, Kampouras, Avramidou, Toulia, Chrysochoou, Galogavrou, Kirvassilis and Tsanakas. This is an open-access article distributed under the terms of the Creative Commons Attribution License (CC BY). The use, distribution or reproduction in other forums is permitted, provided the original author(s) and the copyright owner(s) are credited and that the original publication in this journal is cited, in accordance with accepted academic practice. No use, distribution or reproduction is permitted which does not comply with these terms.
*Correspondence: Elpis Hatziagorou, aGF0emlhZ29yb3VAYXV0aC5ncg==