- 1Division of Neonatology, Careggi University Hospital of Florence, Florence, Italy
- 2Department of Medicine and Surgery, University of Parma, Parma, Italy
- 3Department of Molecular and Developmental Medicine, University of Siena, Siena, Italy
- 4Department of Neonatology, University Medical Center Utrecht and Utrecht University, Utrecht, Netherlands
Background and Aim: Preterm white matter is vulnerable to lipid peroxidation-mediated injury. F2-isoprostanes (IPs), are a useful biomarker for lipid peroxidation. Aim was to assess the association between early peri-postnatal IPs, white matter injury (WMI) at term equivalent age (TEA), and neurodevelopmental outcome in preterm infants.
Methods: Infants with a gestational age (GA) below 28 weeks who had an MRI at TEA were included. IPs were measured in cord blood (cb) at birth and on plasma (pl) between 24 and 48 h after birth. WMI was assessed using Woodward MRI scoring system. Multiple regression analyses were performed to assess the association between IPs with WMI and then with BSITD-III scores at 24 months corrected age (CA). Receiver operating characteristic (ROC) curve analysis was used to evaluate the predictive value of pl-IPs for the development of WMI.
Results: Forty-four patients were included. cb-IPs were not correlated with WMI score at TEA, whereas higher pl-IPs and lower GA predicted higher WMI score (p = 0.037 and 0.006, respectively) after controlling for GA, FiO2 at sampling and severity of IVH. The area under the curve was 0.72 (CI 95% = 0.51–0.92). The pl-IPs levels plotted curve indicated that 31.8 pg/ml had the best predictive threshold with a sensitivity of 86% and a specificity of 60%, to discriminate newborns with any WMI from newborns without WMI. IPs were not associated with outcome at 24 months.
Conclusion: Early measurement of pl-IPs may help discriminate patients showing abnormal WMI score at TEA, thus representing an early biomarker to identify newborns at risk for brain injury.
Introduction
Despite improved survival rates of preterm infants in recent years, these infants remain at risk of developing a wide range of complications both in the neonatal period and in the long term. The outcome, furthermore, depends on gestational age (GA) and neonatal morbidity (1, 2). The brain is particularly susceptible to the consequences of preterm birth, which leads to high rates of long-term neurological disabilities. White matter injury (WMI) is the primary type of brain injury in survivors of premature birth (3). Previous magnetic resonance imaging (MRI) studies to determine the spectrum of WMI showed that non-cystic WMI was the most common pattern of brain injury, whereas cystic periventricular leukomalacia (c-PVL) has become uncommon (4, 5).
Furthermore, it has been demonstrated that MRI performed at term equivalent age (TEA) can predict an adverse neurodevelopmental outcome (6). Together with the MRI, the use of specific biomarkers may help in the early diagnosis of WMI to promptly identify those infants who may benefit from early intervention. To date, various studies investigated different biomarkers of brain injury, however the optimal neuro-biomarker able to predict cerebral damage has not been identified (7, 8).
In the pathogenesis of WMI, ischemia and reperfusion, together with inflammation, seem to play a central role in producing reactive oxygen species (ROS) (9). Preterm white matter is highly exposed to oxidative damage because of the imbalance between free radical production and the immaturity of antioxidant enzymes, superoxide dismutases-1 and -2, catalase, and glutathione peroxidase (10–13). The human preterm white matter is especially vulnerable to lipid peroxidation-mediated injury. The aldehydes originated from lipid hydroperoxides can be identified in the glia quantifying F2-isoprostanes (IPs) in human cerebral tissue (14). In addition, IPs are significantly raised in infants with WMI at neuropathological examination (15).
Thus, our study aimed to assess the possible correlation between IPs, measured in cord blood (cb) at birth and plasma (pl) between 24 and 48 h after birth, and WMI evaluated with the MRI at TEA in a cohort of extremely preterm infants. The second aim was to determine the relationship between IPs and neurodevelopmental outcome at 2 years of age.
Materials and Methods
Patients
A prospective, observational, single-center study was carried out from September 2012 to September 2014 at the Neonatal Intensive Care Unit of the Wilhelmina Children's Hospital Utrecht, Utrecht, the Netherlands. The study was approved by the local ethics committee (10_365). Preterm infants with a gestational age (GA) below 28 weeks, enrolled after written parental informed consent, and for whom an MRI was successfully performed at TEA, were included in this study. Exclusion criteria were major congenital malformations, chromosomal disorders and inborn errors of metabolism.
Biomarkers
Blood samples were obtained from the vein of a double-clamped umbilical cord immediately after birth. Heparinized blood samples of 0.5 ml were drawn from the indwelling arterial catheter, inserted for clinical care, during routine tests between 24 and 48 h after birth. All samples were immediately centrifuged in order to obtain platelet-poor plasma and butylated hydroxytoluene (BHT) 1% w/v in methanol (5 μl per ml of plasma) was supplemented to prevent the in vitro lipid peroxidation (16). Samples were frozen at −80°C and stored until shipping to the laboratory of oxidative stress of the University of Siena, Italy, where the analysis was performed. F2-IPs were detected as markers of lipid OS-induced injury according to the LC-MS/MS methodology described by Casetta e al (17).
MRI Acquisition
The brain MRI was performed on a 3T MR system (Achieva, Philips Medical Systems, Best, Netherlands) at TEA. Infants were wrapped into a vacuum pillow in a SENSE head coil. MiniMuffs (Natus Medical Incorporated, San Carlos, CA, USA) and earmuffs (EM's 4 Kids, Everton Park, Australia) were used for hearing protection. The scanning protocol comprised T2 and T1 weighted imaging. Parameters of the scanning protocol included: axial 3D T1-weighted image (TR = 9.4 ms; TE = 4.6 ms; voxel size 0.94 × 0.94 × 2.0 mm; no gap), coronal 3D T1- weighted image (TR = 9.5ms; TE = 4.6 ms, voxel size 0.78 × 0.91 × 1.2 mm; no gap), axial T2-weighted image (TR = 6,293 ms; TE = 120 ms; voxel size 0.54 × 0.61 × 2.0 mm; no gap), and coronal T2-weighted image (TR = 4,847 ms; TE = 150 ms; voxel size 0.78 × 0.89 × 1.2 mm; no gap). Respiratory rate, heart rate and arterial oxygen saturation were monitored during MRI, and a neonatologist or physician assistant was present during the examination. When necessary, infants were sedated using oral chloral hydrate using a dose of 50 mg/kg through the gastric tube. The severity of brain injury was assessed by using conventional coronal T1- and T2-weighted spin-echo sequences. All MRI images were scored for the presence of brain abnormalities by two experienced neonatologists (LdV and MB) with more than 20 years' experience in neonatal neuroimaging, using the Woodward scoring system (6). This scoring system evaluates the presence/severity of white matter volume loss, white matter signal abnormality, the presence of cystic lesions, ventricular dilation, thinning of the corpus callosum and reduced myelination. The white matter score classes were then calculated based on the infant's total scores: normal, mildly abnormal, moderately and severely abnormal.
Clinical Data Collection
Clinical and demographic parameters were collected from patients' medical records: GA, birth weight (BW), BW <10th percentile, gender, mode of delivery, Apgar score at 5 min of life, fraction of inspired oxygen (FiO2) between 24 and 48 h after birth, occurrence and duration of non-invasive respiratory support and mechanical ventilation, sepsis, bronchopulmonary dysplasia (BPD), necrotizing enterocolitis (NEC), intraventricular hemorrhage (IVH), retinopathy of prematurity, and length of stay in the hospital. Sepsis was defined as positive blood culture. BPD was defined as oxygen requirement at 36 weeks of PMA (18). NEC was defined as Bell's stage >2 (19). IVH was classified according to Papile et al. (20). Socioeconomic status was determined based on maternal educational level (21).
Neurodevelopment Assessment
Neurodevelopmental outcome was assessed at 2 years corrected age (CA) using the Bayley Scales of Infant and Toddler Development, 3rd edition (BSITD-III). Cognitive and motor composite scores were used (mean in a normative population 100, standard deviation 15).
Statistical Analysis
Patients' characteristics were described as mean and standard deviation (SD), rate and percentage, or median and interquartile range (IQR). Changes in IPs levels were compared using Wilcoxon signed rank test. Univariate regression analyses were performed to assess the association between cb-IPs and pl-IPs with WMI. For the cb-IPs analysis the following variables were entered into the model: GA, BW (percentile) and severity of IVH. For the pl-IPs analysis mean FiO2 at sampling was entered into the model instead of BW (percentile). GA and birth weight were included into the regression model because an inverse correlation with IPs has been documented (22, 23). FiO2 was entered since previous studies reported an association between the use of higher oxygen concentrations and increased levels of oxidative stress biomarkers (24, 25). Then, IVH was considered because pl-IPs have been found associated with higher degree of IVH (26). A p < 0.05 was considered statistically significant. Results were presented as coefficients of the independent variables with the 95% confidence intervals (CI). Lastly, multivariate regression models were performed to examine the association between cb-IPs and pl-IPs with BSITD-III scores at 24 months CA. In these analyses, data was corrected for GA, WMI and socio-economic status. Since in our population only one infant showed severe IVH, we included WMI instead of IVH in the analysis. Condition indexes and the variance inflation factor (VIF) of the regression model were computed to detect multi-collinearity. Condition Index <30 and VIF variance inflation factor <5 values indicated that regression models did not have significant multicollinearity. Data analysis was performed using IBM SPSS Statistics version 20 (SPSS INC, Chicago, Illinois, USA). To analyse the impact of the pl-IPs level on the occurrence of any WMI, ROC (receiver operating characteristic) curve analysis was used. The test's ability to classify patients as those who will develop WMI or not is represented by the area under the ROC curve (AUC).
Results
Clinical characteristics of enrolled infants (n = 44) are summarized in Table 1. cb-IPs and pl-IPs levels were not significantly different [54.2 (36.4–79.2) vs. 58.6 (38.2–83.2)] (p = 0.424). Twenty-seven (61%) of patients had mild WMI, and eight (18%) developed moderate WMI. No infants had severe white matter abnormalities on MRI. Forty-two infants returned for follow up examination. The mean scores for cognitive (101 ± 12) and motor (106 ± 13) outcomes were well within the normal range.
Cord Blood Isoprostanes
Univariate regression analysis demonstrated that cb-IPs were not associated with WMI at TEA. After controlling for GA, BW (percentile) and severity of IVH, lower BW was associated with a higher WMI score (r = 0.65; p = 0.025) (Table 2). cb-IPs were not associated with cognitive and motor outcome at 24 months CA. After controlling for GA, maternal education and WMI, maternal educational level was associated with higher cognitive scores (r = 0.63; p = 0.001) (Table 3).
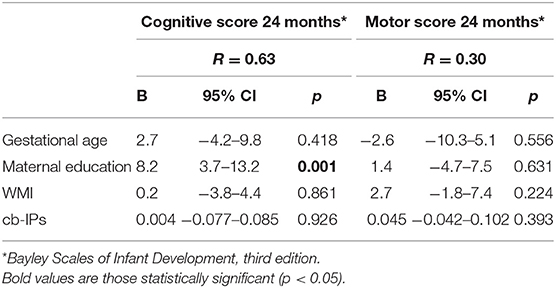
Table 3. Multivariable linear regression analysis between cb-IPs and neurological outcome at 24 months CA.
Plasma Isoprostanes Between 24 and 48 h After Birth
Univariate regression analysis demonstrated that pl-IPs were positively associated with WMI at TEA (r = 0.39; p = 0.02). After adjusting for GA, FiO2 at sampling and severity of IVH, higher pl-IPs concentration and lower GA were associated with a higher WMI score (r = 0.65; p = 0.037 and 0.006, respectively) (Table 4; Figure 1).
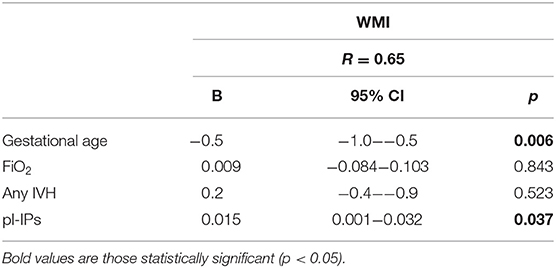
Table 4. Multivariable linear regression analysis correlating pl-IPs between 24 and 48 h after birth and WMI score at TEA.
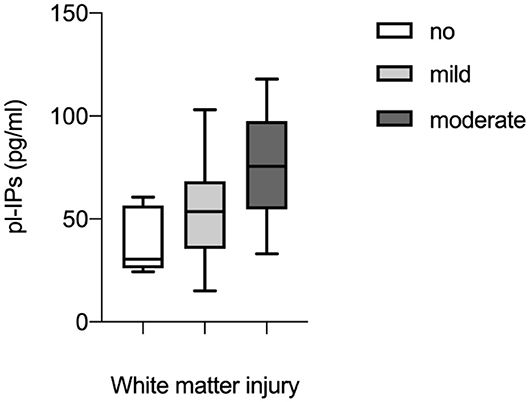
Figure 1. Correlation between plasma levels of isoprostanes (pl-IPs) between 24 and 48 h after birth and WMI evaluated according to the scoring system by Woodward et al. (6). Multivariable regression analysis (p = 0.037).
The area under the curve (AUC) was 0.72 (C.I. 95% = 0.51–0.92). The pl-IPs levels plotted curve indicated that 31.8 pg/ml had the best predictive threshold with a sensitivity of 86% and a lower specificity of 60% to discriminate newborns with and without WMI (Figure 2).
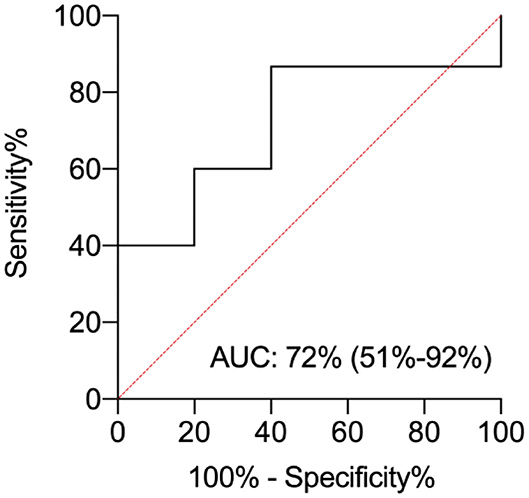
Figure 2. ROC curve analysis for plasma isoprostanes (pl-IPs) concentration between 24 and 48 h after birth. The area under the curve is 0.72 (C.I. 95%= 0.51–0.92). The pl-IPs levels plotted curve indicated 31.8 pg/ml as the best predictive threshold with a sensitivity of 86% and a specificity of 60%. ROC curve discriminates newborns with any WMI from newborns without WMI.
pl-IPs were not associated with cognitive and motor outcome at 24 months CA. After controlling for GA, maternal education and WMI, maternal education level was associated with higher cognitive scores (r = 0.52; p = 0.004) (Table 5).
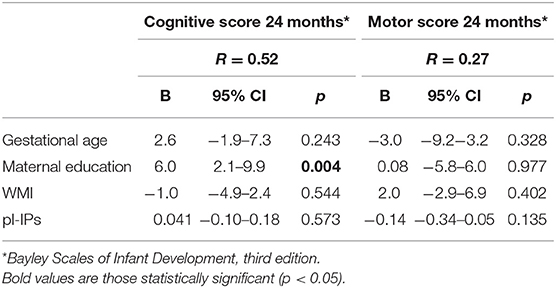
Table 5. Multivariable linear regression analysis correlating pl-IPs between 24 and 48 h after birth and neurological outcome at 24 months CA.
Discussion
Our study investigated the association between IPs levels in the early postnatal period in extremely preterm infants and WMI evaluated at TEA with MRI and neurological outcome at 24 months CA. In this cohort, 79% of the neonates had mild to moderate WMI visible on their MRI at TEA. Our results revealed that early pl-IPs concentration was higher in infants with WMI, and this correlation remained statistically significant after adjusting for potential confounding factors. Thus, pl-IPs levels might be a valuable early biomarker of later WMI.
In preterm infants, during the developmental window between 23 and 32 weeks PMA, the brain is at significant risk for WMI because the white matter is exposed to oxidative stress due to hypoxia-ischemia or maternal-fetal infection (28, 29). Pre-oligodendrocytes (OLs) dominate the white matter during this phase, with the first appearance of differentiated OLs around 30 to 35 gestational weeks (30). Studies of cultured oligodendroglial cells indicate that pre-OLs appear particularly prone to free radical-mediated injury because of the immaturity of antioxidant defenses, whereas differentiated OLs are more resistant to oxidative stress both in vitro and in vivo (10–12, 15, 31, 32). ROS released by activated microglia interact with cell structures like lipids and membranes, inducing lipid peroxidation that results in the release of highly reactive derivatives (33). IPs are the result of free radical-induced injury by peroxidation of lipids in cell membranes. They are formed in vivo by the peroxidation of arachidonic acid, mediated by free radical production by a non-cyclooxygenase mechanism (34, 35). IPs are chemically stable compounds and have been detected in fluids and tissues, such as in the brain, where IPs are enriched in glial cells and are sensitive and specific biomarkers of oxidative stress in adult human brain tissue from autopsy (36, 37). Furthermore, IPs are bioactive compounds that may exert vasoconstriction of different vascular beds, including the brain (38). Thus, it has been hypothesized that IPs may participate in neurovascular injury. To support this theory, IPs have been shown to induce neuromicrovascular endothelial cell death via thromboxane (TXA2) production in rat and piglet models of hypoxia-ischemia encephalopathy (39). Brault et al. demonstrated that IPs provokes pre-OLs death by oncosis, depending on increased production of TxA2 and inadequate antioxidant defenses, whereas little effect has been demonstrated on mature OLs (40).
Back et al. quantified oxidative damage directly in human preterm autopsy brains and found that the increase of IPs concentration generated during the early phases of WMI was associated with a marked depletion of the pre-OL. Moreover, the IPs levels were comparable to those found in the cerebral cortex after severe perinatal asphyxia in term infants (15). A further finding was that IPs concentration was not elevated in cerebral cortex, this is consistent with the concept that WMI develops from hypoxia–ischemia related to vascular end-zones. Hence, oxidative injury selectively targeted the white matter and spared the cerebral cortex during ischemic events. In a previous study, an increase in IPs concentration was demonstrated in the cerebrospinal fluid of 14 infants with WMI evaluated on MRI at TEA, with a strong increase in those with more severe cystic PVL with ventriculomegaly compared to infants with less severe forms of WMI such as white matter signal abnormality or ventriculomegaly alone (41). In addition, pl-IPs were significantly augmented during the first week after birth in preterm infants who went on to develop c-PVL and severe IVH (26).
We found that pl-IPs cutoff level of 31.8 pg/ml measured between 24 and 48 h after birth showed a good sensitivity for WMI (86%) but a low specificity (60%). The small number of subjects enrolled might have influenced this finding. Furthermore, previous studies correlated elevated levels of lipid peroxidation products and several complications of prematurity besides WMI. In fact, early pl-IPs concentration was found higher in infants who later developed BPD or died and severe IVH compared to survivors without (26). In addition, high urinary excretion of IPs has been associated with hemodynamically significant PDA in preterms <34 weeks GA (42). BPD, IVH, PDA, and WMI, all belong to the “free radicals disease” of prematurity, disorders caused by the harmful effect of free radicals (43, 44).
According to our results, pl-IPs predicted WMI at TEA, but not IPs measured on cord blood. cb-IPs reflect oxygen radical exposure during fetal life and placental metabolism of lipid peroxides. It has been reported that IPs concentration varied according to prenatal and precocious infants factors such as chorioamnionitis, fetal inflammatory response of the placenta, perinatal depression, fetal growth and birth weight (23, 45). On the other hand, early pl-IPs concentration might be influenced by other perinatal and postnatal factors, such as FiO2 during resuscitation in the delivery room, respiratory distress syndrome and mechanical ventilation, leading to an increased production of oxygen free radicals (24, 46–48).
In our model, lower BW was correlated to higher WMI score, probably due to lower GA. This result might be related to intrauterine growth restriction since this condition was diagnosed to the four infants small for gestational age (BW percentile <10th) enrolled in our study. Previous findings reported that fetal growth restriction has been associated with delayed oligodendrocyte maturation and myelination in animal models, and diffuse white matter abnormality in preterm infants (49, 50).
We did not find an association between IPs concentration in cord blood and plasma with neurological outcome. On the contrary, Matthews et al. demonstrated increased pl-IPs in preterm infants at risk for worse developmental outcomes adjusting for GA, maternal education and severe abnormalities on neuroimaging (51). The possible explanation for these conflicting results might be the higher incidence of severe abnormalities on neuroimaging [c-PVL, severe IVH (grade III or IV) and cerebellar hemorrhage], compared to our population. Furthermore, the different timing of sampling could also play a role as Matthews analyzed the change in pl-IPs concentration between 14 and 28 days after birth.
Additionally, we failed to find a correlation between WMI at TEA and BSITD-III at 2 years CA. Several studies have proven that MRI executed at term equivalent age (TEA) can predict an adverse neurodevelopmental outcome (6, 52, 53). The lack of this result might reflect the characteristic of our cohort, since none of the newborns showed severe brain injury and at follow up examination the scores were in a regular range. We found that maternal educational level was significantly correlated with cognitive development. This finding suggests that both genetic and environmental factors influence neurodevelopmental outcome. However, other covariates that were not analyzed due to the small sample size might also have influenced early development.
This study has some limitations. First, with the determination of IPs, we explore a specific type of brain injury, involving the prostaglandin metabolism. Other products of lipid peroxidation such as neuroprostanes, IPs-like molecules from docosahexanoic acid (DHA) might be an attractive and more specific biomarker of brain injury since DHA is more abundant than arachidonic acid in the brain (54, 55). The second limitation is the small sample size, which may have limited the possibility to detect a significant role of other potential mediating or interactive factors on outcome. Additional studies with a larger sample size, including infants with more severe brain injury, are needed in order to better define the most predictive cut-off value of pl-IPs for WMI detection.
Conclusion
Early measurement of pl-IPs may help to identify patients with a higher chance of an abnormal WMI score at TEA. The pl-IPs levels plotted curve indicated that 31.8 pg/ml had the best predictive threshold with a sensitivity of 86% and a lower specificity of 60%. pl-IPs might represent a reliable biomarker useful to identify newborns at high risk for brain injury. However, further researches on larger population of infants are needed to validate these findings.
Data Availability Statement
The raw data supporting the conclusions of this article will be made available by the authors, without undue reservation.
Ethics Statement
The studies involving human participants were reviewed and approved by Pediatric ethics committee of the Wilhelmina Children's Hospital Utrecht, Utrecht, Netherlands. Written informed consent to participate in this study was provided by the participants' legal guardian/next of kin.
Author Contributions
CC, SP, GB, SN, ML, CD, LV, FG, DV, MB, and MT have made substantial contributions to conception, design of the study, critically revised the article, and have given their final approval of the version to be published. CC and MT performed the data statistical analyses and wrote the manuscript. CC, MT, and SN collected data. All authors contributed to the article and approved the submitted version.
Funding
This study was supported by grants from the Tuscany Region and Italian Ministry of Health and General Directorate of Scientific and Technological Research for 2011–2014 (Project No. RF-2009-1499651).
Conflict of Interest
The authors declare that the research was conducted in the absence of any commercial or financial relationships that could be construed as a potential conflict of interest.
References
1. Buitendijk S, Zeitlin J, Cuttini M, Langhoff-Roos J, Bottu J. Indicators of fetal and infant health outcomes. Eur J Obstet Gynecol Reprod Biol. (2003) 111:66–77. doi: 10.1016/j.ejogrb.2003.09.007
2. Pierrat V, Marchand-martin L, Arnaud C, Kaminski M, Resche-rigon M, Lebeaux C, et al. Neurodevelopmental outcome at 2 years for preterm children born at 22 to 34 weeks ' gestation in France in 2011 : EPIPAGE-2 cohort study. BMJ. (2017) 358:j3448. doi: 10.1136/bmj.j3448
3. Volpe JJ. Brain injury in premature infants: a complex amalgam of destructive and developmental disturbances. Lancet Neurol. (2009) 8:110–24. doi: 10.1016/S1474-4422(08)70294-1
4. Inder TE, Wells SJ, Mogridge NB, Spencer C, Volpe JJ. Defining the nature of the cerebral abnormalities in the premature infant: a qualitative magnetic resonance imaging study. J Pediatr. (2003) 143:171–9. doi: 10.1067/S0022-3476(03)00357-3
5. Miller SP, Ferriero DM, Leonard C, Piecuch R, Gudden DV, Partridge JC, et al. Early brain injury in premature newborns detected with adverse early neurodevelopmental outcome. J Pediatr. (2005) 147:609–16. doi: 10.1016/j.jpeds.2005.06.033
6. Woodward LJ, Anderson PJ, Austin NC, Howard K, Sc. B, Inder TE. Neonatal MRI to predict neurodevelopmental outcomes in preterm infants. N Engl J Med. (2006) 685–94. doi: 10.1056/NEJMoa053792
7. Douglas-Escobar M, Weiss MD. Biomarkers of brain injury in the premature infant. Front Neurol. (2013) 3:185. doi: 10.3389/fneur.2012.00185
8. Bersani I, Pluchinotta F, Dotta A, Savarese I, Campi F, Auriti C, et al. Early predictors of perinatal brain damage: the role of neurobiomarkers. Clin Chem Lab Med. (2020) 58:471–86. doi: 10.1515/cclm-2019-0725
9. Volpe JJ. Cerebral white matter injury of the premature infant - more common than you think. Pediatrics. (2003) 112:176–80. doi: 10.1542/peds.112.1.176
10. Baud O, Greene AE, Li J, Wang H, Volpe JJ, Rosenberg PA. Glutathione peroxidase-catalase cooperativity is required for resistance to hydrogen peroxidase by mature rat oligodendrocytes. J Neurosci. (2004) 24:1531–40. doi: 10.1523/JNEUROSCI.3989-03.2004
11. Baud O, Haynes RF, Wang H, Folkerth RD, Li J, Volpe JJ, et al. Developmental up-regulation of MnSOD in rat oligodendrocytes confers protection against oxidative injury. Eur J Neurosci. (2004) 20:29–40. doi: 10.1111/j.0953-816X.2004.03451.x
12. Folkerth RD, Haynes RL, Borenstein NS, Belliveau RA, Trachtenberg F, Rosenberg PA, et al. Developmental lag in superoxide dismutases relative to other antioxidant enzymes in premyelinated human telencephalic white matter. J Neuropathol Exp Neurol. (2004) 63:990–9. doi: 10.1093/jnen/63.9.990
13. Panfoli I, Candiano G, Malova M, de Angelis L, Cardiello V, Buonocore G, et al. Oxidative stress as a primary risk factor for brain damage in preterm newborns. Front Pediatr. (2018) 6:369. doi: 10.3389/fped.2018.00369
14. Montine KS, Quinn JF, Zhang J, Fessel JP, Roberts LJ, Morrow JD, et al. Isoprostanes and related products of lipid peroxidation in neurodegenerative diseases. Chem Phys Lipids. (2004) 128:117–24. doi: 10.1016/j.chemphyslip.2003.10.010
15. Back SA, Luo NL, Mallinson RA, Malley JPO, Wallen LD, Frei B, et al. Selective vulnerability of preterm white matter to oxidative damage defined by F2-Isoprostanes. Ann Neurol. (2005) 58:108–20. doi: 10.1002/ana.20530
16. Milne GL, Dai Q RL. The isoprostanes−25 years later. Biochim Biophys Acta Mol Cell Biol Lipids. (2015) 1851:433–45. doi: 10.1016/j.bbalip.2014.10.007
17. Casetta B, Longini M, Proietti F, Perrone S, Buonocore G. Development of a fast and simple LC-MS / MS method for measuring the F2-isoprostanes in newborns. Matern Fetal Neonatal Med. (2012) 25:114–8. doi: 10.3109/14767058.2012.664856
18. Onland W, Debray T, Laughon M. Clinical prediction models for bronchopulmonary dysplasia: a systematic review and external validation study. BMC Pediatr. (2013) 13:207. doi: 10.1186/1471-2431-13-207
19. Bell MJ, Ternberg JL, Feigin R., Keating JP, Marshall R, Barton L. Neonatal necrotizing enterocolitis: therapeutic decisions based upon clinical staging. Ann Surg. (1987) 187:1–12. doi: 10.1097/00000658-197801000-00001
20. Papile LA, Burstein J, Burstein R KH. Incidence and evolution of subependymal and intraventricular hemorrhage: a study of infants with birth weights less than 1,500 gm. J Pediatr. (1978) 92:529–34. doi: 10.1016/S0022-3476(78)80282-0
21. Divisie Sociale en ruimtelijke statistieken SSt. Standaard Onderwijsindeling (2006). Ed 2013/'14 Den Haag/H:
22. Comporti M, Signorini C, Leoncini S, Buonocore G, Rossi V, Ciccoli L. Plasma F2-isoprostanes are elevated in newborns and inversely correlated to gestational age. Free Radic Biol Med. (2004) 37:724–32. doi: 10.1016/j.freeradbiomed.2004.06.007
23. Mestan K, Matoba N, Arguelles L, Harvey C, Ernst LM, Farrow K, et al. Cord blood 8-isoprostane in the preterm infant. Early Hum Dev. (2012) 88:683–9. doi: 10.1016/j.earlhumdev.2012.02.002
24. Vento M, Moro M, Escrig R, Arruza L, Villar G, Izquierdo I, et al. Preterm resuscitation with low oxygen causes less oxidative stress, inflammation, and chronic lung disease. Pediatrics. (2009) 124:e439–49. doi: 10.1542/peds.2009-0434
25. Kapadia VS, Chalak LF, Sparks JE, Allen JR, Savani RC, Wyckoff MH. Resuscitation of preterm neonates with limited versus high oxygen strategy. Pediatrics. (2013) 132:e1488–96. doi: 10.1542/peds.2013-0978
26. Ahola T, Fellman V, Kjellmer I, Raivio KO, Lapatto R. Plasma 8-isoprostane is increased in preterm infants who develop bronchopulmonary dysplasia or periventricular leukomalacia. Pediatr Res. (2004) 56:88–93. doi: 10.1203/01.PDR.0000130478.05324.9D
27. Visser GHA, Eilers PHC, Elferink-Stinkens PM, Merkus HMWM, Wit JM. New Dutch reference curves for birthweight by gestational age. Early Hum Dev. (2009) 85:737–44. doi: 10.1016/j.earlhumdev.2009.09.008
28. Ferriero DM, Miller SP. Imaging selective vulnerability in the developing nervous system. J Anat. (2010) 217:429–35. doi: 10.1111/j.1469-7580.2010.01226.x
29. Hagberg H, Peebles D, Mallard C. Models of white matter injury: comparison of infectious, hypoxic-Ischemic, and excitotoxic insults. Ment Retard Dev Disabil Res Rev. (2002) 8:30–8. doi: 10.1002/mrdd.10007
30. Back SA, Luo NL, Borenstein NS, Levine JM, Volpe JJ, Kinney HC. Late oligodendrocyte progenitors coincide with the developmental window of vulnerability for human perinatal white matter injury. J Neurosci. (2001) 21:1302–12. doi: 10.1523/JNEUROSCI.21-04-01302.2001
31. Haynes RL, Folkerth RD, Keefe RJ, Sung I, Swzeda LI, Rosenberg PA, et al. Nitrosative and oxidative injury to premyelinating oligodendrocytes in periventricular leukomalacia. J Neuropathol Exp Neurol. (2003) 62:441–50. doi: 10.1093/jnen/62.5.441
32. Back SA, Han BH, Luo NL, Chricton CA, Xanthoudakis S, Tam J, et al. Selective vulnerability of late oligodendrocyte progenitors to hypoxia-ischemia. J Neurosci. (2002) 22:455–63. doi: 10.1523/JNEUROSCI.22-02-00455.2002
33. Esterbauer H. Estimation of peroxidative damage. A critical review. Pathol Biol. (1996) 44:25–8.
34. Milne GL, Musiek ES, Morrow JD. F2-isoprostanes as markers of oxidative stress in vivo: an overview. Biomarkers. (2005) 10(Suppl. 1):10–23. doi: 10.1080/13547500500216546
35. Morrow JD, Hill KE, Burk RF, Nammour TM, Badr KF, Roberts LJ. A series of prostaglandin F2-like compounds are produced in vivo in humans by a non-cyclooxygenase, free radical-catalyzed mechanism. Proc Natl Acad Sci USA. (1990) 87:9383–7. doi: 10.1073/pnas.87.23.9383
36. Milne G, Huiyong Y, Klarissa D, Davies Ss, Jackson R. Isoprostane generation and function. Chem Rev. (2011) 111:5973–96. doi: 10.1021/cr200160h
37. Montine TJ, Morrow JD. Fatty acid oxidation in the pathogenesis of Alzheimer's disease. Am J Pathol. (2005) 166:1283–9. doi: 10.1016/S0002-9440(10)62347-4
38. Hou X, Roberts LJ, Gobeil F, Taber DF, Kanai K, Abran D, et al. Isomer-specific contractile effects of a series of synthetic F 2-isoprostanes on retinal and cerebral microvasculature. Free Radic Biol Med. (2004) 36:163–72. doi: 10.1016/j.freeradbiomed.2003.10.024
39. Brault S, Martinez-Bermudez AK, Marrache AM, Gobeil F, Hou X, Beauchamp M, et al. Selective neuromicrovascular endothelial cell death by 8-iso-prostaglandin F2α: possible role in ischemic brain injury. Stroke. (2003) 34:776–82. doi: 10.1161/01.STR.0000055763.76479.E6
40. Brault S, Martinez-Bermudez AK, Roberts J, Cui QL, Fragoso G, Hemdan S, et al. Cytotoxicity of the E2-isoprostane 15-E2t-IsoP on oligodendrocyte progenitors. Free Radic Biol Med. (2004) 37:358–66. doi: 10.1016/j.freeradbiomed.2004.05.007
41. Inder T, Mocatta T, Darlow B, Spencer C, Volpe JJ, Winterbourn C. Elevated free radical products in the cerebrospinal fluid of VLBW infants with cerebral white matter injury. Pediatr Res. (2002) 52:213–8. doi: 10.1203/00006450-200208000-00013
42. Coviello C, Tataranno ML, Corsini I, Leonardi V, Longini M, Bazzini F, et al. Isoprostanes as biomarker for patent ductus arteriosus in preterm infants. Front Pediatr. (2020) 8:3–6. doi: 10.3389/fped.2020.00555
43. Saugstad OD. Oxygen radical disease in neonatology. Semin Neonatol. (1998) 3:229–38. doi: 10.1016/S1084-2756(98)80008-0
44. Perrone S, Tataranno ML, Negro S, Longini M, Marzocchi B, Proietti F, et al. Early identification of the risk for free radical-related diseases in preterm newborns. Early Hum Dev. (2010) 86:241–4. doi: 10.1016/j.earlhumdev.2010.03.008
45. Hracsko Z Orvos H Novak Z Pal A Varga IS. Evaluation of oxidative stress markers in neonates with intra-uterine growth retardation. Redox Rep. (2008) 13:11–6. doi: 10.1179/135100008X259097
46. Tataranno ML, Oei JL, Perrone S, Wright IM, Smyth JP, Lui K, et al. Resuscitating preterm infants with 100% oxygen is associated with higher oxidative stress than room air. Acta Paediatr Int J Paediatr. (2015) 104:759–65. doi: 10.1111/apa.13039
47. Cho HY, Morgan DL, Bauer AK, Kleeberger SR. Signal transduction pathways of tumor necrosis factor-mediated lung injury induced by ozone in mice. Am J Respir Crit Care Med. (2007) 175:829–39. doi: 10.1164/rccm.200509-1527OC
48. Deng H, Mason SN, Auten RL. Lung inflammation in hyperoxia can be prevented by antichemokine treatment in newborn rats. Am J Respir Crit Care Med. (2000) 162:2316–23. doi: 10.1164/ajrccm.162.6.9911020
49. Tolcos M, Bateman E, O'Dowd R, Markwick R, Vrijsen K, Rehn A, et al. Intrauterine growth restriction affects the maturation of myelin. Exp Neurol. (2011) 232:53–65. doi: 10.1016/j.expneurol.2011.08.002
50. Barnett ML, Tusor N, Ball G, Chew A, Falconer S, Aljabar P, et al. Exploring the multiple-hit hypothesis of preterm white matter damage using diffusion MRI. NeuroImage Clin. (2018) 17:596–606. doi: 10.1016/j.nicl.2017.11.017
51. Matthews M, Aschner J, Stark A, Moore PE, Slaughter JC, Steele S, et al. Increasing F2-isoprostanes in the first month after birth predicts poor respiratory and neurodevelopmental outcomes in very preterm infants. Perinatol. (2016) 36:779–83. doi: 10.1038/jp.2016.74
52. Kidokoro H, Anderson PJ, Doyle LW, Woodward LJ, Neil JJ, Inder TE. Brain injury and altered brain growth in preterm infants: predictors and prognosis. Pediatrics. (2014) 134:E444–53. doi: 10.1542/peds.2013-2336
53. Martinez-Biarge M, Groenendaal F, Kersbergen KJ, Benders MJNL, Foti F, Van Haastert IC, et al. Neurodevelopmental outcomes in preterm infants with white matter injury using a new MRI classification. Neonatology. (2019) 116:227–35. doi: 10.1159/000499346
54. Roberts LJ, Montine TJ, Markesbery WR, Tapper AR, Hardy P, Chemtob S, et al. Formation of isoprostane-like compounds (neuroprostanes) in vivo from docosahexaenoic acid. J Biol Chem. (1998) 273:13605–12. doi: 10.1074/jbc.273.22.13605
Keywords: isoprostanes, white matter injury, preterm infants, oxidative stress, brain injury
Citation: Coviello C, Perrone S, Buonocore G, Negro S, Longini M, Dani C, de Vries LS, Groenendaal F, Vijlbrief DC, Benders MJNL and Tataranno ML (2021) Isoprostanes as Biomarker for White Matter Injury in Extremely Preterm Infants. Front. Pediatr. 8:618622. doi: 10.3389/fped.2020.618622
Received: 17 October 2020; Accepted: 14 December 2020;
Published: 15 January 2021.
Edited by:
Fiammetta Piersigilli, Cliniques Universitaires Saint-Luc, BelgiumReviewed by:
Donna M. Ferriero, University of California, San Francisco, United StatesFrancesca Campi, Bambino Gesù Children Hospital (IRCCS), Italy
Copyright © 2021 Coviello, Perrone, Buonocore, Negro, Longini, Dani, de Vries, Groenendaal, Vijlbrief, Benders and Tataranno. This is an open-access article distributed under the terms of the Creative Commons Attribution License (CC BY). The use, distribution or reproduction in other forums is permitted, provided the original author(s) and the copyright owner(s) are credited and that the original publication in this journal is cited, in accordance with accepted academic practice. No use, distribution or reproduction is permitted which does not comply with these terms.
*Correspondence: Caterina Coviello, caterinacoviello83@yahoo.it