- 1Pediatric Unit, Department of Medical and Surgical Sciences of the Mothers, Children and Adults, University of Modena and Reggio Emilia, Modena, Italy
- 2Department of Medical and Surgical Sciences of the Mothers, Children and Adults, Post Graduate School of Pediatrics, University of Modena and Reggio Emilia, Modena, Italy
- 3Neonatal Intensive Care Unit, Department of Medical and Surgical Sciences of the Mothers, Children and Adults, University of Modena and Reggio Emilia, Modena, Italy
Hypothalamic-pituitary-gonadal (HPG) axis activation occurs three times in life: the first is during fetal life, and has a crucial role in sex determination, the second time is during the first postnatal months of life, and the third is with the onset of puberty. These windows of activation recall the three windows of the “Developmental Origin of Health and Disease” (DOHaD) paradigm and may play a substantial role in several aspects of human development, such as growth, behavior, and neurodevelopment. From the second trimester of pregnancy there is a peak in gonadotropin levels, followed by a decrease toward term and complete suppression at birth. This is due to the negative feedback of placental estrogens. Studies have shown that in this prenatal HPG axis activation, gonadotropin levels display a sex-related pattern which plays a crucial role in sex differentiation of internal and external genitalia. Soon after birth, there is a new increase in LH, FSH, and sex hormone concentrations, both in males and females, due to HPG re-activation. This postnatal activation is known as “minipuberty.” The HPG axis activity in infancy demonstrates a pulsatile pattern with hormone levels similar to those of true puberty. We review the studies on the changes of these hormones in infancy and their influence on several aspects of future development, from linear growth to fertility and neurobehavior.
Introduction
During embryogenesis, the pituitary gland begins synthesizing both Luteinizing Hormone (LH) and Follicle-Stimulating Hormone (FSH) at around 9 weeks of gestation (1). LH and FSH can be detected in fetal blood from 12 to 14 weeks (2, 3) and start to be GnRH-dependent after 31–32 weeks (4, 5).
Prenatal modulation of the HPG axis activity is also due to placental hormone production. In fact, the structure of hCG is an analog of LH and may bind to the LH receptor, with similar biological effects on gonadal tissues (2, 6). Moreover, the placenta produces Estrogens (E) and Progesterone (P), that rise during the third trimester. This has a negative effect on gonadotropin levels and results in a drop in LH and FSH in cord blood at birth in healthy infants of both sexes (6–8).
After birth, the removal of placental hormones from the neonate's circulation results in a lack of negative feedback on the GnRH pulse generator and reactivation of the HPG axis. This postnatal activation that starts in the first few days of life is known as “minipuberty” (9).
Studies on healthy term neonates indicate that the rise of LH and FSH begins at around 1 week of age. It achieves a peak, reaching the pubertal range, between 1 and 3 months of life and then declines toward the age of 6 months (6–8, 10–13). These postnatal hormonal changes have different trends in boys and girls. Particularly in males, it seems to be related to the development and maturation of the reproductive system. Furthermore, the impact of gonadotropins and sex-steroid hormones during this first period of life has been studied and relates to many different aspects of infant growth and behavior (14).
The aim of this review is to summarize the current understanding on minipuberty and its role as a temporary window of opportunity for diagnosis and possible treatment in babies with disorders of sex development (DSD). Moreover, we would like to highlight the extent of what happens (or not) during minipuberty in terms of hormonal changes and trends which may influence future neurobehavior.
Infants Born at Term
Males: In male neonates, both LH and FSH levels peak between 1 and 3 months of age and then gradually decrease to prepubertal levels at around 6-9 months (9, 12, 14). The LH peak is higher than the FSH level. Testosterone (T) starts to increase 1 week following the LH rise and declines to prepubertal values by 6 months of age (11, 12, 15). T levels, both in cord blood and in serum during the first postnatal months, are higher in boys than in girls (11–13, 15–17).
The number of Leydig cells in both testes increases considerably until the third month of life, which correlates with the T trend and then gradually decreases due to an apoptosis process (18, 19). Sertoli cells also grow during the first postnatal months under the stimulation of FSH (20) but, without the expression of androgen receptors (AR) during infancy, they do not complete their maturation and spermatogenesis does not occur (21). This leads to an increase of testicular volume during the first months after birth, which then gradually decreases until the second year of life due to the halt in cell proliferation, the reduction of AMH production, and the formation of the blood-testicular barrier (22, 23).
All these hormonal changes during the first months of life have a great impact on the urogenital system. This involves not only the testes but also the development and growth of the penis, prostate, and scrotal hair. In fact, the postnatal T surge within the first three months has been associated with penile growth in infancy (24). The increase in androgens has been associated with cutaneous manifestations, such as sebaceous gland hypertrophy and acne (25). There is also a link with the development of transient isolated scrotal hair between 3 and 6 months of life with a spontaneous disappearance within the first year of life (26).
Females: In female infants, FSH levels are higher than LH, following a different trend than in males. FSH shows the same gonadotropin peak as in males at 1–3 months of age but can remain elevated up to 3–4 years of life. In contrast, LH levels decrease at the same age as in boys (9, 12, 27).
E levels at birth are high, with similar values in the cord blood of both sexes (28), followed by a gradual decrease during the first days of life and a new increase after the first week only in girls.
E remains high until 6 months with fluctuating levels, probably related to the FSH trend, and decreases toward 2 years of life (29–31). The mammary glands and uterus are certainly E target tissues but evidence of minipuberty effects is not univocal. At birth, most full-term babies of both sexes have palpable breast tissue (32) that probably results from placental E effect. In the following months, breast tissue in females remains larger and persists longer due to HPG axis activity and its consequent E production (30). In contrast, uterine length increases in utero but, after birth, there is a steady decrease from day 7 toward the third month, after which the volume remains stable until the second year (30).
With little evidence from few studies, the biological role of minipuberty in girls is still controversial and partially unknown.
Babies born small for gestational age (SGA): The HPG axis activation in SGA infants born at term is not well defined and its short-term and long-term effects on growth and development are still controversial. Studies on SGA females found higher postnatal FSH levels compared with neonates born appropriate for gestational age (AGA). This different pattern of secretion in SGA females was also associated with reduced uterine and ovarian size that persisted into young adulthood (33, 34). Moreover, Anti Mullerian Hormone (AMH) levels have been reported to be higher in SGA girls at 2–3 months of life, suggesting possible altered follicular development (35).
In contrast with these findings, other studies have reported higher E in SGA females after the administration of a GnRH stimulation test, although the reported basal levels were not significantly different (35).
In male SGA term neonates, HPG axis activation has been linked both to lower (36) and higher (34) FSH and T (37) levels, with uncertain effects in adult life (38).
Further studies are necessary to clarify the pattern of minipuberty in SGA male and female infants, along with the clinical implications. It is important to bear in mind that SGA neonates are at increased risk of metabolic and endocrinological disorders. These include reduced insulin sensitivity and increased adrenal hyperandrogenism, with consequent precocious pubarche and reduced ovulation rate (39).
Preterm Infants
Little is known about the influence of prematurity on HPG axis activity and its effects. Fewer studies have investigated this pattern longitudinally in preterm (PT) babies compared with those born full-term (FT). Preterm birth does not seem to influence the postnatal HPG axis activation, as gonadotropin levels begin to rise after birth (whenever that is, as fetal-placental interruption) with the same timing as in FT infants.
Moreover, this hormonal surge might be even stronger and more prolonged than in FT infants (40, 41). However, these data are not univocal (40–42) in either the amplitude or the duration between different sexes. Immaturity of the hypothalamic feedback has been suggested as a possible mechanism for this strong and prolonged activation, although its biological significance is still not completely understood.
The most recent longitudinal data suggest that minipuberty declines at about the same post-term age in term neonates compared with premature infants, suggesting that the HPG activity is regulated in an evolutionarily way (13). In particular, Kuiri-Hanninen et al. (13) used spot urine samples in order to compare gonadotropin and testosterone levels in a small cohort of FT and PT male neonates with a gestational age (GA) between 24.7 and 36.6 weeks. From day 7 to 14 months of age they measured length, weight, penile length, and testicular volume. They simultaneously collected urine samples to detect urinary gonadotropins and testosterone levels until 6 months of age. Their findings revealed higher hormonal levels in PT babies with a positive association between testosterone levels and penile growth, as well as between FSH levels and testicular growth after birth until 5 months of age, when a subsequent decrease occurred. In addition, studies on PT female infants demonstrated higher gonadotropin levels than those in FT girls with a prolonged duration of the peak (43) but a sharp decrease around term age (30). In these PT girls, an amplified postnatal E surge was observed at around three months of corrected age and there was an association with increased growth of the mammary gland and uterine length. Possible clinical consequences of this intensive stimulation in premature females are evidenced by features of the ovarian hyperstimulation syndrome with edema of the vulva, solitary or multiple cysts in the ovaries on ultrasonography, breast growth, and occasional vaginal bleeding (44, 45).
The hormonal differences between boys and girls during minipuberty appear to be fundamental for later sexual differentiation and development. In particular, we think that increasing knowledge on minipuberty in girls may give us key information about the premature thelarche of girls below 2 years of age, and the early puberty that occurs before 8 years of age. In SGA neonates, results are still controversial and more studies are needed to clarify how gonadotrophins and sexual hormones change according to sex. This may be very useful, considering that SGA-born children may go through early puberty and/or precocious isolated pubarche. In preterm babies, we speculated that the prolonged activation of HPG axis may be one of the factors influencing the early re-activation of the HPG axis before puberty age.
Babies With Disorders of Sex Development
The development of internal and external genitalia is a complex balance between gene expression and hormonal influence and an anomaly at each stage can result in 46, XY DSD.
From this point of view, minipuberty can be considered as a window of sensitivity, because it may allow the clinician to come to an early diagnosis and possible treatment opportunity.
Studies on primates testing the effects of a reversible suppression of minipuberty using GnRH agonists or antagonists described lower testis volume and penile length in cases treated compared with controls (46–48). Male infants with congenital central hypogonadism (CHH) were found to have an absence of both fetal and postnatal FSH, LH, and T surges (49). This lack of postnatal FSH secretion seems to be the main reason for impaired germ cell differentiation with later infertility, especially if associated with cryptorchidism (50, 51). As a result, minipuberty may potentially provide a short window of time to make an early diagnosis and for treatment in male neonates that exhibit a micropenis with or without cryptorchidism (52, 53), improving the outcome of orchidopexy, and also reducing the long-term consequences of an absent minipuberty.
On the other hand, the finding of elevated gonadotropins during minipuberty in a 46, XY male neonate with undetectable testosterone levels may suggest congenital anorchism (vanishing testis or testicular regression syndrome). Infants with complete androgen insensitivity syndrome may present with lower-than-normal postnatal LH and T levels, whereas these hormones may be normal or high in cases of partial androgen insensitivity syndrome (54).
We have a unique opportunity to evaluate the spontaneous function of the HPG hormone axis during minipuberty. It is therefore recommended that serum FSH, LH, and testosterone are measured during the first 6 months of life in infants with DSD or suspected CHH. In particular, we would suggest checking for these hormones at seven days of life, and one, three, and, if possible, 6 months of life, to detect the minipuberty trend. The use of the LH/FSH ratio may provide important information in the workup of infants suspected of DSD, especially regarding the sex specific ratio detected in literature (55).
Minipuberty: A Window for Treatment?
Neonates affected by cryptorchidism, micropenis, and CHH must receive timely treatment to optimize genital development. The current recommendation for a micropenis is brief therapy with low-dose testosterone delivered by intramuscular injection or by topical application to induce penile growth. This treatment was also assumed to work for cryptorchidism. However, while exogenous T stimulates penile growth, it does not affect testicular development. In fact, current recommendations advocate surgical correction for undescended testes during the first year of life (56). Nevertheless, there are limitations to this treatment. Small testes augment the risk of testicular trauma and tissue loss during orchidopexy (57), increasing the likelihood of a negative impact on future fertility. Moreover, successful scrotal repositioning of testes does not prevent infertility. However, a normal minipuberty after successful surgery may lead to the presence of Ad spermatogonia (58). The role of Ad spermatogonia is to maintain the supply of stem cells for spermatogenesis. In 178 testicular biopsies after orchidopexy the authors found three groups of high, intermediate, and low risk of infertility depending on the presence of Ad spermatogonia. After puberty, sperm concentrations were analyzed and correlated positively with plasma gonadotropin and testosterone levels. For all these reasons, recreating the hormonal milieu of minipuberty with gonadotrophin treatment could be beneficial for these patients.
In 2002, Main et al. (59) published the first case of CHH and micropenis treated with short-term recombinant human LH and FSH. The outcome of this case was successful; the penile length increased by 50% and the testicular volume almost tripled. Similar results were described in other recent cases (60, 61).
The REMAP study (62) investigated the use of recombinant LH plus FSH preparations in neonates and infants with a micropenis and/or cryptorchidism due to hypogonadotropic hypogonadism. During therapy, all ten patients increased their height velocity: LH levels increased from undetectable to high-normal; FSH reached supranormal levels; and Inibin-b, AMH, and T reached normal levels. Penile length normalized among all children and intriguingly confirms the emerging evidence that testicular descendance is induced by gonadotrophin treatment (61, 63). Furthermore, in this study the therapy may have induced high/normal activation of Sertoli and Leydig cells, restoring testicular endocrine function and improving future fertility.
Vincel et al. (64) analyzed testicular biopsies before and after orchidopexy or hormonal treatment in patients with isolated bilateral cryptorchidism with a high infertility risk. Their results showed how the number of Ad spermatogonia and the number of germ cells per at least 100 tubular cross-sections increased or decreased post-surgery. Indeed, patients who received hormonal treatment showed an important increase in the number of cells and the complete transition of gonocyte and fetal spermatogonia to Ad spermatogonia. These findings support the hypothesis that GnRH induces LH release; LH increases testosterone levels acting directly on Leyding cells, mimicking minipuberty (50, 65).
Finally, studies have focused on the molecular mechanisms that explain the ability of GnRH to rescue fertility. The analysis demonstrates that several IncRNAs involved in epigenetic programming were responsive to GnRH treatment, helping in the preparation of Ad spermatogonial stem cells for commitment to differentiation. In particular, the authors found that DMRTC2, PAX7, BRACHYURY/T, and TERT were associated with defective minipuberty and were responsive to GnRHa (66). Minipuberty may represent a “window of opportunity” to evaluate the HPG axis by measuring basal hormone concentrations with no need for stimulation tests in infants with suspected reproductive disorders. Minipuberty provides a unique opportunity to evaluate the spontaneous function of the HPG axis which is lost thereafter for approximately another 10 years until the HPG axis is reactivated in puberty (67).
Is Our Environment Influencing Minipuberty in Humans and Predisposing Them to DSD?
Endocrine Disruptor Chemicals (EDC) are compounds detectable in every setting of daily life. These chemical compounds are found in a range of products such as those containing pesticides, metals, additives or food contaminants, and personal care products (68). In fact, EDCs are so common that it is almost impossible for individuals to avoid them during everyday activities. These substances may cause adverse health effects, disrupting endocrine function. In particular, they interfere with the endocrine and reproductive systems through nuclear receptors, non-nuclear steroid hormone receptors, non-steroid receptors, orphan receptors, enzymatic pathways, and other mechanisms (69). Children may be exposed both directly and indirectly to ECDs, especially during the three main temporal windows of the DOHaD paradigm and during breastfeeding (70, 71). Concerning breastfed children, Ortega-Garcia et al. detected a linear positive correlation between anogenital distance (AGD) in male infants and the duration of breastfeeding (72). The results of this study, called MALAMA, suggested breastfeeding to be a protective factor against the reduction of the AGD of 2-year-old boys. The authors hypothesized this could be related to early exposure to EDCs through baby formula milk (72).
Moreover, EDCs may interfere with HPG activation (73), both during fetal life or immediately after birth, throughout minipuberty. EDCs during minipuberty in males could impair testicular descent (74). Focusing on some of the most analyzed compounds in this research area, several studies have demonstrated that Bisphenol A (BPA) has an anti-androgen function, decreasing testosterone levels, an event that impacts sex differentiation during fetal life and modifies the AGD length (75, 76). In particular, Sun et al. showed how maternal exposure to BPA was associated with shortened AGD in boys at 12 months of age, highlighting a gender specific effect (77). Another family of EDCs influencing minipuberty are phthalates. Maternal exposure to phthalates during pregnancy showed a reduced T level in males at minipuberty and, because of the antiandrogenic effect of these compounds, the testosterone-luteinizing hormone ratio (T/LH) is also lower in the same period (78). This is probably due to compensated Leydig cell function, requiring higher levels of LH to maintain the necessary level of T for embryo differentiation. It was demonstrated in animal models that phthalates can inhibit Insl3 production and consequently modify the gubernaculum growth necessary for the testes' transabdominal descent (79, 80). However, the effects of these compounds on Insl3 and T in the human testes were less attenuated than in rodents. Table 1 summarizes the most recent studies on the effect of EDC on HPG axis during minipuberty in humans. The impact of EDCs is not limited to the postnatal period. Indeed, alongside this phase there are two more windows of development: fetal and puberty. In these phases, cells are promptly proliferating, and epigenetic changes are more likely to occur (81). All this may lead to additional effects in later stages of life including delayed or precocious puberty (82–85), small testes and high levels of follicle-stimulating hormone (FSH) (86, 87), polycystic ovary syndrome (88), and breast cancer (89).
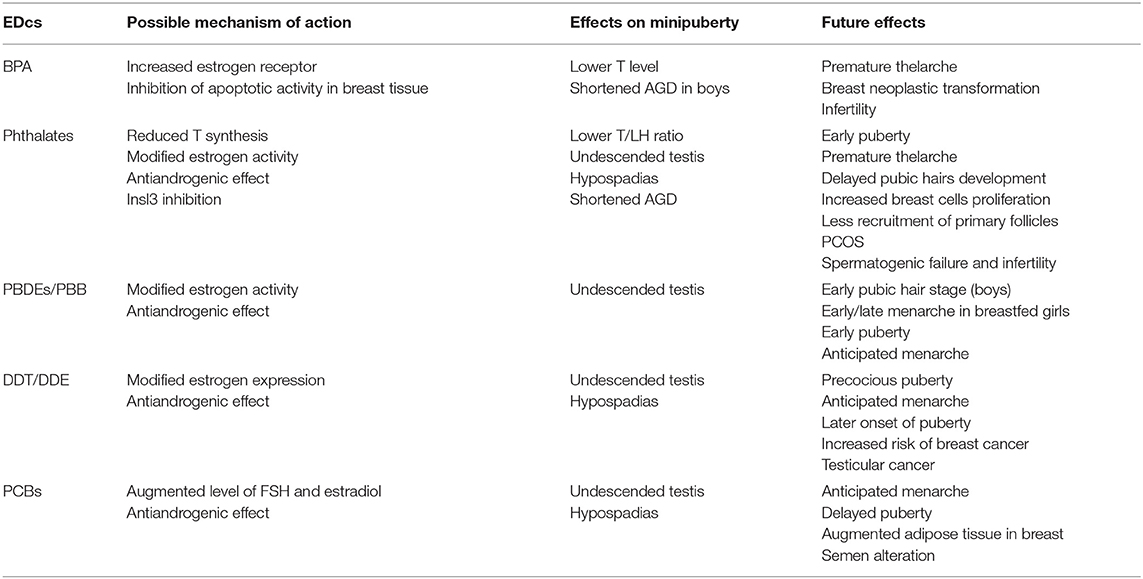
Table 1. Possible effects in humans of the main EDCs on minipuberty and long-term consequences (68–89).
Our environment plays a crucial role in developmental programming. The influence of EDCs on minipuberty may predispose an individual to undescended testis, AGD modifications, or reduction of T surge. We should always keep an eye on the appearance of the external genitalia in neonates and on the possible maternal exposure to phthalates and BPA through a specific interview.
How Minipuberty Influences Linear Growth During the First 6 Months of Life
During minipuberty, the transient HPG axis activation results in a sex steroid surge. Some studies have indicated a higher growth velocity and a faster increase in weight (and lean body mass) associated with somatic changes in boys when compared with girls during the first 6 months of life (90–93). Based on these results, studies have tested the hypothesis of an association with minipuberty, particularly with the peak of testosterone production. Kiviranta et al. (94) evaluated the precise timing and the magnitude of this sexual dimorphism in growth among a large cohort of full-term healthy boys and girls during the first years of life. In a smaller sample of healthy neonates, serial measurements of urinary and blood hormones were assessed. Results from this study demonstrated that linear growth was significantly faster in boys than in girls, especially when comparing the first three months of age. Interestingly, this observation occurred simultaneously with the peak of postnatal gonadal activation and the authors found a positive correlation between T levels and growth velocity in both sexes, elucidating a possible novel biological role of minipuberty as an engine of growth velocity during the first months of life. Differences in sex hormones during minipuberty between boys and girls are important for the sex differentiation in linear growth and body composition, with males having a higher growth velocity and accumulating more lean mass compared to females.
How Minipuberty Modulates Neurobehavioral Development
As for sexual development, the human brain is also shaped by a combination of genetic, epigenetic, environmental, and hormonal exposure. Sex steroid hormones are among one of the strongest biological factors influencing neural and behavioral development. Over the past two decades, there has been a growing interest in understanding how sex determination and sexual hormones may affect structural and functional brain development (95, 96). The cellular and molecular mechanisms induced by T (converted to estradiol in the brain) are multifaceted and include neurogenesis, cellular differentiation, axon guidance, synaptic pruning, apoptosis, and phagocytosis. Several studies on mammalian brains, including humans, have demonstrated that early androgen exposure has an influence on sex differences in juvenile behavior (54, 96, 97). Indeed, manipulating androgens prenatally in non-human primates alters brain regions and behaviors (98). Many studies have been performed in girls prenatally exposed to high levels of androgens because of congenital adrenal hyperplasia (CAH) where there is strong evidence of male typical play behavior, suggesting a similar hormonal influence on human brain development (98–104). This influence of androgen levels on the brain has been identified not only among affected girls but also in the general population. Fetal T measured from amniotic fluid positively correlates with male typical play in preschool girls and boys assessed with a standardized questionnaire (105). This prenatal period of HPG axis activation is therefore critical for the sexual differentiation that drives the different organization in circuitry and neuroanatomy between the male and female brain. The early postnatal surge of gonadotropins and T in boys during minipuberty can potentially provide a window of opportunity in understanding the effects of sex steroid hormones on human gender development (106). Emerging evidence suggests that T levels during minipuberty have an influence not only on male genitalia and reproductive function but also on later gender-typical behavior. Indeed, minipuberty occurs during a period of huge and rapid brain development in terms of volume, cortical thickness, surface, and cortical network development (107–109). Lamminmäki et al. (110) found a positive correlation between T levels in FT infants from day 7 to 6 months and future sex-typed behavior at 14 months of life. In this study, the Pre-School Activities Inventory (PSAI) (111, 112) playroom was used during an observation of toy choices. In boys, T levels correlated significantly with PSAI scores and playing with trains. Conversely, playing with dolls was significantly correlated with a negative trend. In addition, Pasterski et al. (113) used AGD at birth and penile growth from birth to 3 months of age to estimate prenatal and postnatal androgen exposure. They re-evaluated children included in the study at 3 to 4 years of age using the PSAI suggesting that T levels in both periods, prenatal and postnatal, are independent contributors to later gender-related behavior. Language development is another area of investigation of a possible correlation with early postnatal HPG axis activation. Results based on small samples suggest a correlation between T levels and a different expressive vocabulary in boys and girls (114, 115). We have summarized some of the clearest studies of the last decade in Table 2 in order to better understand the influence of hormonal changes happening during minipuberty on sex-related behavior. All these emerging results may support a role for the imprinting of T during early infancy in human neurobehavioral sexual differentiation, although its effects are still largely unknown in both the short and long-term.
Conclusion
Although further studies are needed, pre- and postnatal activation of the HPG axis could be considered an important window of prediction on how each newborn will grow and develop. Measurement of LH, FSH, and testosterone at 7 days, one and three months, and, when possible, six months, may help the clinician to better understand how minipuberty develops in different neonates. This will give us important information once the baby approaches puberty or when he or she shows impaired linear growth. Moreover, minipuberty must be considered a fundamental moment for possible therapeutic intervention in DSD. Therapeutic interventions may be able to change the natural history of some DSD or, at least, to improve prognosis in terms of fertility and quality of life.
Author Contributions
LL, VT, ABo, and LM analyzed the existing literature and wrote the draft of the review. ABe and LI critically reviewed the manuscript. All authors read and approved the final version of the manuscript. All authors contributed to the article and approved the submitted version.
Conflict of Interest
The authors declare that the research was conducted in the absence of any commercial or financial relationships that could be construed as a potential conflict of interest.
References
1. Hagen C, McNeilly AS. The gonadotrophins and their subunits in foetal pituitary glands and circulation. J Steroid Biochem. (1977) 8:537–44. doi: 10.1016/0022-4731(77)90259-X
2. Clements JA, Reyes FI, Winter JSD, Faiman C. Studies on human sexual development. III. fetal pituitary and serum, and amniotic fluid concentrations of LH. CG, and FSH. J Clin Endocrinol Metab. (1976) 42:9–19. doi: 10.1210/jcem-42-1-9
3. Kaplan SL, Grumbach MM. The onotogenesis of human foetal hormones. II. Luteinizing hormone (LH) and follicle stimulating hormone (FSH). Acta Endocrinologica. (1976) 81:808–29. doi: 10.1530/acta.0.0810808
4. Guimiot F, Chevrier L, Dreux S, Chevenne D, Caraty A, Delezoide AL. Negative fetal FSH/LH regulation in late pregnancy is associated with declined kisspeptin/KISS1R expression in the tuberal hypothalamus. J Clin Endocrinol Metab. (2012) 97:E2221–9. doi: 10.1210/jc.2012-2078
5. Pilavdzic D, Kovacs K, Asa SL. Pituitary morphology in anencephalic human fetuses. Neuroendocrinology. (1997) 65:164–72. doi: 10.1159/000127177
6. Varvarigou AA, Liatsis SG, Vassilakos P, Decavalas G, Beratis NG. Effect of maternal smoking on cord blood estriol, placental lactogen, chorionic gonadotropin, FSH, LH, and cortisol. J Perin Med. (2009) 37:28. doi: 10.1515/JPM.2009.028
7. Winter JSD, Faiman C, Hobson WC, Prasad AV, Reyes FI. Pituitary-Gonadal relations in infancy. I. patterns of serum gonadotropin concentrations from birth to four years of age in man and chimpanzee. J Clin Endocrinol Metab. (1975) 40:545–51. doi: 10.1210/jcem-40-4-545
8. Winter JSD, Hughes IA, Reyes FI, Faiman C. Pituitary-Gonadal relations in infancy: 2. Patterns of serum gonadal steroid concentrations in man from birth to two years of age. J Clin Endocrinol Metab. (1976) 42:679–86. doi: 10.1210/jcem-42-4-679
9. Kuiri-Hänninen T, Sankilampi U, Dunkel L. Activation of the hypothalamic-Pituitary-Gonadal axis in infancy: minipuberty. Horm Res Paediatr. (2014) 82:73–80. doi: 10.1159/000362414
10. Schmidt H, Schwarz H. Serum concentrations of LH and FSH in the healthy newborn. Eur J Endocrinol. (2000) 2000:213–5. doi: 10.1530/eje.0.1430213
11. Bergadá I, Milani C, Bedecarrás P, Andreone L, Ropelato MG, Gottlieb S, et al. Time course of the serum gonadotropin surge, inhibins, and anti-Müllerian hormone in normal newborn males during the first month of life. J Clin Endocrinol Metab. (2006) 91:4092–8. doi: 10.1210/jc.2006-1079
12. Andersson A-M. Longitudinal reproductive hormone profiles in infants: peak of inhibin b Levels in infant boys exceeds levels in adult men. J Clin Endocrinol Metab. (1998) 83:675–81. doi: 10.1210/jc.83.2.675
13. Kuiri-Hänninen T, Seuri R, Tyrväinen E, Turpeinen U, Hamalainen E, Stenman U-H, et al. Increased activity of the hypothalamic-Pituitary-Testicular axis in infancy results in increased androgen action in premature boys. J Clin Endocrinol Metab. (2011) 96:98–105. doi: 10.1210/jc.2010-1359
14. Zegher FD, Devlieger H, Veldhuis JD. Pulsatile and sexually dimorphic secretion of luteinizing hormone in the human infant on the day of birth. Pediatr Res. (1992) 32:605–7. doi: 10.1203/00006450-199211000-00025
15. Forest MG, Cathiard AM, Bertrand JA. Evidence of testicular activity in early infancy. J Clin Endocrinol Metab. (1973) 37:148–51. doi: 10.1210/jcem-37-1-148
16. Barry JA, Hardiman PJ, Siddiqui MR, Thomas M. Meta-analysis of sex difference in testosterone levels in umbilical cord blood. J Obst Gyn. (2011) 31:697–702. doi: 10.3109/01443615.2011.614971
17. Garagorri JM, Rodríguez G, Lario-Elboj ÁJ, Olivares JL, Lario-Muñoz Á, Orden I. Reference levels for 17-hydroxyprogesterone, 11-desoxycortisol, cortisol, testosterone, dehydroepiandrosterone sulfate and androstenedione in infants from birth to six months of age. Eur J Pediatr. (2008) 167:647–53. doi: 10.1007/s00431-007-0565-1
18. Nistal M, Paniagua R, Regadera J, Santamaria L, Amat P. A quantitative morphological study of human leydig cells from birth to adulthood. Cell Tissue Res. (1986) 246:229–36. doi: 10.1007/BF00215884
19. Chemes HE. Infancy is not a quiescent period of testicular development. Int J Androl. (2001) 24:2–7. doi: 10.1046/j.1365-2605.2001.00260.x
20. Cortes D, Müller J, Skakkebæk NE. Proliferation of sertoli cells during development of the human testis assessed by stereological methods. Int J Androl. (1987) 10:589–96. doi: 10.1111/j.1365-2605.1987.tb00358.x
21. Chemes HE, Rey RA, Nistal M, Regadera J, Musse M, Gonzàles-Peramato P, et al. Physiological androgen insensitivity of the fetal, neonatal, and early infantile testis is explained by the ontogeny of the androgen receptor expression in sertoli cells. J Clin Endocrinol Metab. (2008) 93:4408–12. doi: 10.1210/jc.2008-0915
22. Kuijper EAM, van Kooten J, Verbeke JIML, van Rooijen M, Lambalk CB. Ultrasonographically measured testicular volumes in 0- to 6-year-old boys. Hum Reprod. (2008) 23:792–6. doi: 10.1093/humrep/den021
23. Cassorla FG, Golden SM, Johnsonbaugh RE, Heroman WM, Loriaux DL, Sherins RJ. Testicular volume during early infancy. J Pediat. (1981) 99:742–3. doi: 10.1016/S0022-3476(81)80398-8
24. Boas M, Boisen KA, Virtanen HE, Kaleva M, Suomi AM, Schmidt IM, et al. Postnatal penile length and growth rate correlate to serum testosterone levels: a longitudinal study of 1962 normal boys. Eur J Endocrinol. (2006) 154:125–9. doi: 10.1530/eje.1.02066
25. Kuiri-Hänninen T, Haanpää M, Turpeinen U, Hämäläinen E, Dunkel L, Sankilampi U. Transient postnatal secretion of androgen hormones is associated with acne and sebaceous gland hypertrophy in early infancy. J Clin Endocrinol Metab. (2013) 98:199–206. doi: 10.1210/jc.2012-2680
26. Janus D, Wojcik M, Tyrawa K, Starzyk J. Transient isolated scrotal hair development in infancy. Clin Pediatr. (2013) 52:628–32. doi: 10.1177/0009922813480845
27. Kuiri-Hänninen T, Kallio S, Seuri R, Tyvairen E, Liakka A, Tapanainen J, et al. Postnatal developmental changes in the pituitary-ovarian axis in preterm and term infant girls. J Clin Endocrinol Metab. (2011) 96:3432–9. doi: 10.1210/jc.2011-1502
28. Troisi R, Potischman N, Roberts J, Siiteri P, DAftary A, Sims C, et al. Associations of maternal and umbilical cord hormone concentrations with maternal, gestational and neonatal factors (United States). Cancer Causes Control. (2003) 14:347–55. doi: 10.1023/a:1023934518975
29. Schmidt IM, Chellakooty M, Haavisto A-M, Boisen KA, Damgaard IN, Steendahl U, et al. Gender difference in breast tissue size in infancy: correlation with serum estradiol. Pediatr Res. (2002) 52:682–6. doi: 10.1203/00006450-200211000-00012
30. Kuiri-Hänninen T, Haanpää M, Turpeinen U, Hamalainen E, Seuri R, Tyrvainen E, et al. Postnatal ovarian activation has effects in estrogen target tissues in infant girls. J Clin Endocrinol Metab. (2013) 98:4709–16. doi: 10.1210/jc.2013-1677
31. Chellakooty M, Schmidt IM, Haavisto AM, Boisen KA, Damgaard IN, Petersen JH, et al. Inhibin a, inhibin b, follicle-Stimulating hormone, luteinizing hormone, estradiol, and sex hormone-Binding globulin levels in 473 healthy infant girls. J Clin Endocrinol Metab. (2003) 88:3515–20. doi: 10.1210/jc.2002-021468
32. Jayasinghe Y, Cha R, Horn-Ommen J, O'Brien P, Simmons PS. Establishment of normative data for the amount of breast tissue present in healthy children up to two years of age. J Pediat Adolesc Gynecol. (2010) 23:305–11. doi: 10.1016/j.jpag.2010.03.002
33. Ibáñez L, Potau N, Enriquez G, Marcos MV, de Zegher F. Hypergonadotrophinaemia with reduced uterine and ovarian size in women born small-for-gestational-age. Hum Reprod. (2003) 18:1565–9. doi: 10.1093/humrep/deg351
34. Ibáñez L, Valls C, Cols M, Ferrer A, Marcos MV, de Zegher F. Hypersecretion of fSH in infant boys and girls born small for gestational age. J Clin Endocrinol Metab. (2002) 87:1986–8. doi: 10.1210/jcem.87.5.8459
35. Sir-Petermann T, Hitchsfeld C, Codner E, Maliqueo M, Iniguez G, Echiburù B, et al. Gonadal function in low birth weight infants: a Pilot study. J Pediat Endocrinol Metab. (2007) 20:405. doi: 10.1515/JPEM.2007.20.3.405
36. Nagai S, Kawai M, Myowa-Yamakoshi M, Morimoto T, Matsukura T, Heike T. Gonadotropin levels in urine during early postnatal period in small for gestational age preterm male infants with fetal growth restriction. J Perinatol. (2017) 37:843–7. doi: 10.1038/jp.2017.55
37. Forest MG, de Peretti E, Bertrand J. Testicular and adrenal androgens and their binding to plasma proteins in the perinatal period: developmental patterns of plasma testosterone, 4-androstenedione, dehydroepiandrosterone and its sulfate in premature and small for date infants as compared with that of full-term infants. J Steroid Biochem. (1980) 12:25–36. doi: 10.1016/0022-4731(80)90247-2
38. Cicognani A, Alessandroni R, Pasini A, Pirazzoli P, Cassio A, Barbieri E, et al. Low birth weight for gestational age and subsequent male gonadal function. J Pediat. (2002) 141:376–80. doi: 10.1067/mpd.2002.126300
39. Ibáñez L, de Zegher F. Puberty after prenatal growth restraint. Horm Res. (2006) 65(Suppl. 3):112–5 doi: 10.1159/000091515
40. Tapanainen J, Koivisto M, Vihko R, Huhtaniemi I. Enhanced activity of the pituitary-Gonadal axis in premature human infants. J Clin Endocrinol Metab. (1981) 52:235–8. doi: 10.1210/jcem-52-2-235
41. Shinkawa O, Furuhashi N, Fukaya T, Suzuki M, Kono H, Tachibana Y. Changes of serum gonadotropin levels and sex differences in premature and mature infant during neonatal life. J Clin Endocrinol Metab. (1983) 56:1327–31. doi: 10.1210/jcem-56-6-1327
42. Greaves RF, Hunt RW, Chiriano AS, Zacharin MR. Luteinizing hormone and follicle-Stimulating hormone levels in extreme prematurity: development of reference intervals. Pediatrics. (2008) 121:e574–e80. doi: 10.1542/peds.2007-1327
43. de Jong M, Rotteveel J, Heijboer AC, Cranendonk A, Twisk JWR, van Weissenbruch MM. Urine gonadotropin and estradiol levels in female very-low-birth-weight infants. Early Hum Dev. (2013) 89:131–5. doi: 10.1016/j.earlhumdev.2012.09.007
44. Sedin G, Bergquist C, Lindgren PG. Ovarian hyperstimulation syndrome in preterm infants. Pediatr Res. (1985) 19:548–52. doi: 10.1203/00006450-198506000-00009
45. Poon WYS, Tung JYL. Vaginal bleeding in an infant with extreme prematurity. Case Rep Pediatr. (2020) 2020:8881634. doi: 10.1155/2020/8881634
46. Lunn SF, Cowen GM, Fraser HM. Blockade of the neonatal increase in testosterone by a gnRH antagonist: the free androgen index, reproductive capacity and postmortem findings in the male marmoset monkey. J Endocrinol. (1997) 154:125–31. doi: 10.1677/joe.0.1540125
47. Nevison B, Dixson F. Manipulation of postnatal testosterone levels affects phallic and clitoral development in infant rhesus monkeys. Int J Androl. (1999) 22:119–28. doi: 10.1046/j.1365-2605.1999.00158.x
48. Liu L, Cristiano AM, Southers JL. Effects of pituitary-Testicular axis suppression in utero and during the early neonatal period with a long-Acting luteinizing hormone-Releasing hormone analog on genital development, somatic growth, and bone density in male cynomolgus monkeys in the first 6 months of life. J Clin Endocrinol Metab. (1991) 73:1038–43. doi: 10.1210/jcem-73-5-1038
49. Grumbach MM. A window of opportunity: the diagnosis of gonadotropin deficiency in the male infant 1. J Clin Endocrinol Metab. (2005) 90:3122–7. doi: 10.1210/jc.2004-2465
50. Hadziselimovic F, Zivkovic D, Bica DTG, Emmons LR. The importance of mini-puberty for fertility in cryptorchidism. J Urol. (2005) 174(4 Part 2):1536–9. doi: 10.1097/01.ju.0000181506.97839.b0
51. Hadziselimovic F, Emmons LR, Buser MW. A diminished postnatal surge of ad spermatogonia in cryptorchid infants is additional evidence for hypogonadotropic hypogonadism. Swiss Med Wkly. (2004) 134:381–4.
52. Quinton R, Mamoojee Y, Jayasena CN, Young J, Howard S, Dunkel L, et al. Society for endocrinology UK guidance on the evaluation of suspected disorders of sexual development: emphasizing the opportunity to predict adolescent pubertal failure through a neonatal diagnosis of absent minipuberty. Clin Endocrinol. (2017) 86:305–6. doi: 10.1111/cen.13257
53. Dwyer AA, Jayasena CN, Quinton R. Congenital hypogonadotropic hypogonadism: implications of absent mini-puberty. Min Endocrinol. (2016) 2016:10 doi: 10.3389/fendo.2019.00353
54. Bouvattier C, Carel J-C, Lecointre C, David A, Sultan C, Berrand A-M, et al. Postnatal changes of t, lH, and fSH in 46,XY infants with mutations in the AR gene. J Clin Endocrinol Metab. (2002) 87:29–32. doi: 10.1210/jcem.87.1.7923
55. Johannsen TH, Main KM, Ljubicic ML, Jensen TK, Andersen HR, Andersen MS, et al. Sex differences in reproductive hormones during mini-Puberty in infants with normal and disordered sex development. J Clin Endocrinol Metab. (2018) 103:3028–37. doi: 10.1210/jc.2018-00482
56. Kolon TF, Herndon CDA, Baker LA, Baskin LS, Baxter CG, Cheng EY, et al. Evaluation and treatment of cryptorchidism: aUA guideline. J Urol. (2014) 192:337–45 doi: 10.1016/j.juro.2014.05.005
57. Bouvattier C, Maione L, Bouligand J, Dodé C, Guiochon-Mantel A, Young J. Neonatal gonadotropin therapy in male congenital hypogonadotropic hypogonadism. Nat Rev Endocrinol. (2012) 8:172–82 doi: 10.1038/nrendo.2011.164
58. Hadziselimovic F, Hoecht B. Testicular histology related to fertility outcome and postpubertal hormone status in cryptorchidism. Klinische Padiatrie. (2008) 220:302–7 doi: 10.1055/s-2007-993194
59. Main KM, Schmidt IM, Toppari J, Skakkebaek NE. Early postnatal treatment of hypogonadotropic hypogonadism with recombinant human FSH and LH. Eur J Endocrinol. (2002) 146:75–9 doi: 10.1530/eje.0.1460075
60. Bougnères P, François M, Pantalone L, Rodrigue D, Bouvattier C, Demesteere E, et al. Effects of an early postnatal treatment of hypogonadotropic hypogonadism with a continuous subcutaneous infusion of recombinant follicle-stimulating hormone and luteinizing hotmone. J Clin Endocrinol Metab. (2008) 93:2202–5 doi: 10.1210/jc.2008-0121
61. Stoupa A, Samara-Boustani D, Flechtner I, Pinto G, Jourdon I, Gonzaléz-Briceno L, et al. Efficacy and safety of continuous subcutaneous infusion of recombinant human gonadotropins for congenital micropenis during early infancy. Horm Res Paediatr. (2017) 87:103–10 doi: 10.1159/000454861
62. Papadimitriop DT, Chrysis D, Nyktari G, Zoupanos G, Liakou E, Papadimitriou A, et al. Replacement of male mini-Puberty. J Endocr Society. (2019) 3:1275–82 doi: 10.1210/js.2019-00083
63. Lambert A, Bougnères P. Growth and descent of the testes in infants with hypognadotropic hypogonadism receiving subcoutaneous gonadotropin infusion. Int J Pediatr Endocrinol. (2016) 2016:13 doi: 10.1186/s13633-016-0031-9
64. Vincel B, Verkauskas G, Bilius V, Dasevicius D, Malcius D, Jones B, et al. Gonadotropin-Releasing hormone agonist corrects defective mini-Puberty in boys with cryptorchidism: a Prospective randomized study. BioMed Research International. (2018) 46:51218 doi: 10.1155/2018/4651218
65. Zivkovic D, Bica DTG, Hadziselimovic F. Relationship between adult dark spermatogonia and secretory capacity of leydig cells in cryptorchidism. BJU Int. (2007) 100:1147–9. doi: 10.1111/j.1464-410X.2007.07034.x
66. Gegenschatz-Schmid K, Verkauskas G, Demougin P, Bilius V, Dasevicius D, Stadler MB, et al. DMRTC2, PAX7, BRACHYURU/T and tert are implicated in male germ cell development following curative hormone treatment for cryptorchidism-induced infertility. Genes. (2017) 8:267. doi: 10.3390/genes8100267
67. Renault CH, Aksglaede L, Wøjdemann D, Hansen AB, Jensen RB, Juul A. Minipuberty of human infancy - a window of opportunity to evaluate hypogonadism and differences of sex development? Ann Pediatr Endocrinol Metab. (2020) 25:84–91 doi: 10.6065/apem.2040094.047
68. https://www.endocrine.org/advocacy/position-statements/endocrine-disrupting-chemicals (Accessed September 19, 2020).
69. Phillips KP, Foster WG. Key developments in endocrine disrupter research and human health. J Toxicol Environ Health B Crit Rev. (2008) 11:322–44 doi: 10.1080/10937400701876194
70. Watkins DJ, Sánchez BN, Téllez-Rojo MM, Lee JM, Mercado-García A, Blank-Goldenberg C, et al. Phthalate and bisphenol a exposure during in utero windows of susceptibility in relation to reproductive hormones and pubertal development in girls. Environ Res. (2017) 159:143–51 doi: 10.1016/j.envres.2017.07.051
71. Bowman JD, Choudhury M. Phthalates in neonatal health: friend or foe? J Dev Orig Health Dis. (2016) 7:652–64 doi: 10.1017/S2040174416000349
72. Ortega-Garcia JA, Olano-Soler HA, Martinez-Alvarez A, Campillo-Lopez F, Gomariz-Penalver V, Mendiola-Olivares J, et al. Breastfeeding duration and anogenital distance in 2-year-old infants. Breastfeed Med. (2016) 1:350–5. doi: 10.1089/bfm.2016.0034
73. Winneke G, Ranft U, Wittsiepe J, Kasper-Sonnenberg M, Furst P, Kramer U, et al. Behavioral sexual dimorphism in school-age children and early developmental exposure to dioxins and pCBs: a follow-up study of the duisburg cohort. Environ Health Perspect. (2014) 122:292–8. doi: 10.1289/ehp.1306533
74. Ünüvar T, Büyükgebiz A. Fetal and neonatal endocrine disruptors. J Clin Res Pediatr Endocrinol. (2012) 4:51–60 doi: 10.4274/Jcrpe.569
75. Liu C, Xu X, Zhang Y, Li W, Huo X. Associations between maternal phenolic exposure and cord sex hormones in male newborns. Hum. Reprod. (2016) 31:648–56. doi: 10.1093/humrep/dev327
76. Steckler T, Wang J, Bartol FF, Roy SK, Padmanabhan V. Fetal programming: prenatal testosterone treatment causes intrauterine growth retardation, reduces ovarian reserve and increases ovarian follicular recruitment. Endocrinology. (2015) 146:3185–93. doi: 10.1210/en.2004-1444
77. Sun X, Li D, Liang H, Miao M, Song X, Wang Z, et al. Maternal exposure to biphenol a and anogenital distance troughout infancy: a longitudinal study from Shangai, China. Environ Int. (2018) 121(Pt 1):269–75. doi: 10.1016/j.envint.2018.08.055
78. Muerköster A-P, Frederiksen H, Juul A, Andersson A-M, Jensen RC, Glintborg D, et al. Maternal phthalate exposure associated with decreased testosterone/LH ratio in male offspring during mini-puberty. Odense Child Cohort. Environ Int. (2020) 144:106025 doi: 10.1016/j.envint.2020.106025
79. Howdeshell KL, Furr J, Lambright CR, Rider CV, Wilson VS, Gray LE. Cumulative effects of dibutyl phthalate and diethylhexyl phthalate on male rat reproductive tract development: altered fetal steroid hormones and genes. Toxicological Sciences. (2007) 99:190–202. doi: 10.1093/toxsci/kfm069
80. Borch J, Metzdorff SB, Vinggaard AM, Brokken L, Dalgaard M. Mechanisms underlying the anti-androgenic effects of diethylhexyl phthalate in fetal rat testis. Toxicology. (2006) 223:144–55 doi: 10.1016/j.tox.2006.03.015
81. Gore AC, Chappell VA, Fenton SE, Flaws JA, Nadal A, Prins GS, et al. EDC-2: the endocrine society's second scientific statement on endocrine-Disrupting chemicals. Endocr Rev. (2015) 36:E1–E50 doi: 10.1210/er.2015-1010
82. Vasiliu O, Muttineni J, Karmaus W. In utero exposure to organochlorines and age at menarche. Hum Reprod. (2004) 19:1506–12 doi: 10.1093/humrep/deh292
83. Windham GC, Pinney SM, Voss RW, Sjödin A, Biro FM, Greenspan LC, et al. Brominated flame retardants and other persistent organohalogenated compounds in relation to timing of puberty in a longitudinal study of girls. Environ Health Perspect. (2015) 123:1046–52 doi: 10.1289/ehp.1408778
84. Hashemipour M, Kelishadi R, Amin MM, Ebrahim K. Is there any association between phthalate exposure and precocious puberty in girls? Environ Sci Pollut Res Int. (2018) 25:13589–96 doi: 10.1007/s11356-018-1567-4
85. Chen Y, Wang Y, Ding G, Tian Y, Zhou Z, Wang X, et al. Association between bisphenol a exposure and idiopathic central precocious puberty (ICPP) among school-aged girls in shanghai, china. Environ Int. (2018) 115:410–6 doi: 10.1016/j.envint.2018.02.041
86. Hsu PC, Lai TJ, Guo NW, Lambert GH, Leon Guo Y. Serum hormones in boys prenatally exposed to polychlorinated biphenyls and dibenzofurans. J Toxicol Environ Health A. (2005) 68:1447–56. doi: 10.1080/15287390590967360
87. Yang CY, Yu ML, Guo HR, Lai TJ, Hsu CC, Lambert G, et al. The endocrine and reproductive function of the female yucheng adolescents prenatally exposed to pCBs/PCDFs. Chemosphere. (2005) 61:355–6 doi: 10.1016/j.chemosphere.2005.02.089
88. Palioura E, Diamanti-Kandarakis E. Polycystic ovary syndrome (PCOS) and endocrine disrupting chemicals (EDCs). Rev Endocr and Met Disorders. (2016) 16:365–71 doi: 10.1007/s11154-016-9326-7
89. Burks H, Martin E, Mclachlan J, Bunnell B, Burow M. Endocrine disruptors and the tumor microenvironment: a new paradigm in breast cancer biology. Mol Cell Endocrinol. (2017) 457:13–9 doi: 10.1016/j.mce.2016.12.010
90. Roche AF, Guo S, Moore WM. Weight and recumbent length from 1 to 12 mo of age: reference data for 1-mo increments. Am J Clin Nutr. (1989) 49:599–607. doi: 10.1093/ajcn/49.4.599
91. Rosen O, Cohen A. Analysis of growth curves via mixtures. Statist Med. (2003) 22:3641–54. doi: 10.1002/sim.1582
92. Gasser T, Sheehy Al. Molinari. Sex dimorphism in growth. Ann Hum Biol. (2000) 27:187–97. doi: 10.1080/030144600282299
93. Becker M, Oehler K, Partsch C-J, Ulmen U, Schmutzler R, Cammann H, et al. Hormonal 'minipuberty' influences the somatic development of boys but not of girls up to the age of 6 years. Clin Endocrinol. (2015) 83:694–701. doi: 10.1111/cen.12827
94. Bizzarri C, Cappa M. Ontogeny of hypotalamus-Pituitary gonadal axis and minipuberty: an ongoing debate? Front Endocrinol. (2020) 11:187 doi: 10.3389/fendo.2020.00187
95. Kiviranta P, Kuiri-Hanninen T, Saari A, Lamidi M-L, Dunkel L, Sankilampi U. Transient postnatal gonadal activation and growth velocity in infancy. Pediatrics. (2016) 138:e20153561–e20153561. doi: 10.1542/peds.2015-3561
96. Lenroot RK, Gogtay N, Greenstein DK, Molloy Wells E, Wallace GL, Clasen LS, et al. Sexual dimorphism of brain developmental trajectories during childhood and adolescence. NeuroImage. (2007) 36:1065–73. doi: 10.1016/j.neuroimage.2007.03.053
97. Goddings A-L, Beltz A, Peper JS, Crone EA, Braams BR. Understanding the role of puberty in structural and functional development of the adolescent brain. J Res Adolesc. (2019) 29:32–53. doi: 10.1111/jora.12408
98. Hines M, Brook C, Conway GS. Androgen and psychosexual development: core gender identity, sexual orientation, and recalled childhood gender role behavior in women and men with congenital adrenal hyperplasia (CAH). J Sex Res. (2004) 41:75–81. doi: 10.1080/00224490409552215
99. Ehrhardt A, Meyer-Bahlburg H. Effects of prenatal sex hormones on gender-related behavior. Science. (1981) 211:1312–8. doi: 10.1126/science.7209510
100. New MI. Diagnosis and management of congenital adrenal hyperplasia. Annu Rev Med. (1998) 49:311–28. doi: 10.1146/annurev.med.49.1.311
101. Berenbaum SA, Hines M. Early androgens are related to childhood sex-Typed toy preferences. Psychol Sci. (1992) 3:203–6. doi: 10.1111/j.1467-9280.1992.tb00028.x
102. Pasterski VL, Geffner ME, Brain C, Hindmarsh P, Brook C, Hines M. Prenatal hormones and postnatal socialization by parents as determinants of male-typical toy play in girls with congenital adrenal hyperplasia. Child Dev. (2005) 76:264–78. doi: 10.1111/j.1467-8624.2005.00843.x
103. Pasterski V, Zucker KJ, Hindmarsh PC, Hughes IA, Acerini C, Spencer D, et al. Increased cross-Gender identification independent of gender role behavior in girls with congenital adrenal hyperplasia: results from a standardized assessment of 4- to 11-Year-Old children. Arch Sex Behav. (2015) 44:1363–75. doi: 10.1007/s10508-014-0385-0
104. Frisén L, Nordenström A, Falhammar H, Flipsson H, Holmdahl G, Janson PO, et al. Gender role behavior, sexuality, and psychosocial adaptation in women with congenital adrenal hyperplasia due to CYP21A2 deficiency. J Clin Endocrinol Metab. (2009) 94:3432–9. doi: 10.1210/jc.2009-0636
105. Auyeung B, Baron-Cohen S, Ashwin E, Knickmeter R, Taylor K, Hackett G, et al. Fetal testosterone predicts sexually differentiated childhood behavior in girls and in boys. Psychol Sci. (2009) 20:144–8. doi: 10.1111/j.1467-9280.2009.02279.x
106. Hines M, Spencer D, Kung KT, Browne WV, Constantinescu M, Noorderhaven RM. The early postnatal period, mini-puberty, provides a window on the role of testosterone in human neurobehavioural development. Curr Opin Neurobiol. (2016) 38:69–73. doi: 10.1016/j.conb.2016.02.008
107. Knickmeyer RC, Gouttard S, Kang C, Evans D, Wilber K, Smith JK, et al. A structural MRI study of human brain development from birth to 2 years. J Neurosci. (2008) 28:12176–82. doi: 10.1523/JNEUROSCI.3479-08.2008
108. Lyall AE, Shi F, Geng X, Woolson S, Wang L, Hamer RM, et al. Dynamic development of regional cortical thickness and surface area in early childhood. Cereb Cortex. (2015) 25:2204–12. doi: 10.1093/cercor/bhu027
109. Li G, Lin W, Gilmore JH, Shen D. Spatial patterns, longitudinal development, and hemispheric asymmetries of cortical thickness in infants from birth to 2 years of age. J Neurosci. (2015) 35:9150–62. doi: 10.1523/JNEUROSCI.4107-14.2015
110. Lamminmäki A, Hines M, Kuiri-Hänninen T, Kilpeläinen L, Dunkel L, Sankilampi U. Testosterone measured in infancy predicts subsequent sex-typed behavior in boys and in girls. Horm Behav. (2012) 61:611–6. doi: 10.1016/j.yhbeh.2012.02.013
111. Golombok S, Rust J. The measurement of gender role behaviour in pre-School children: a research note. J Child Psychol Psychiat. (1993) 34:805–11. doi: 10.1111/j.1469-7610.1993.tb01072.x
112. Golombok S, Rust J, Zervoulis K, Croudace T, Golding J, Hines M. Developmental trajectories of sex-Typed behavior in boys and girls: a Longitudinal general population study of children aged 2.5-8 years. Child Dev. (2008) 79:1583–93. doi: 10.1111/j.1467-8624.2008.01207.x
113. Pasterski V, Acerini CL, Dunger DB, Ong KK, Hughes IA, Thankamony A, et al. Postnatal penile growth concurrent with mini-puberty predicts later sex-typed play behavior: evidence for neurobehavioral effects of the postnatal androgen surge in typically developing boys. Horm Behav. (2015) 69:98–105. doi: 10.1016/j.yhbeh.2015.01.002
114. Kung KTF, Browne WV, Constantinescu M, Noorderhaven RM, Hines M. Early postnatal testosterone predicts sex-related differences in early expressive vocabulary. Psychoneuroendocrinology. (2016) 68:111–6. doi: 10.1016/j.psyneuen.2016.03.001
115. Schaadt G, Hesse V, Friederici AD. Sex hormones in early infancy seem to predict aspects of later language development. Brain Lang. (2015) 141:70–6. doi: 10.1016/j.bandl.2014.11.015
116. Constantinescu M, Moore DS, Johnson SP, Hines M. Early contributions to infants' mental rotation abilities. Dev Sci. (2018) 21:e12613. doi: 10.1111/desc.12613
117. Fenson L, Bates E, Dale P, Goodman J, Reznick JS, Thal D. Measuring variability in early child language: don't shoot the messenger. Child Dev. (2000) 71:323–8. doi: 10.1111/1467-8624.00147
Keywords: minipuberty, neurobehavior, neonate, gonadotrophins, hypothalamic-pituitary-gonadal axis, hypogonadism
Citation: Lucaccioni L, Trevisani V, Boncompagni A, Marrozzini L, Berardi A and Iughetti L (2021) Minipuberty: Looking Back to Understand Moving Forward. Front. Pediatr. 8:612235. doi: 10.3389/fped.2020.612235
Received: 30 September 2020; Accepted: 14 December 2020;
Published: 18 January 2021.
Edited by:
Amanda Lesley Ogilvy-Stuart, Cambridge University Hospitals NHS Foundation Trust, United KingdomReviewed by:
Sasha Howard, Queen Mary University of London, United KingdomAlan David Rogol, University of Virginia, United States
Copyright © 2021 Lucaccioni, Trevisani, Boncompagni, Marrozzini, Berardi and Iughetti. This is an open-access article distributed under the terms of the Creative Commons Attribution License (CC BY). The use, distribution or reproduction in other forums is permitted, provided the original author(s) and the copyright owner(s) are credited and that the original publication in this journal is cited, in accordance with accepted academic practice. No use, distribution or reproduction is permitted which does not comply with these terms.
*Correspondence: Laura Lucaccioni, laura.lucaccioni@unimore.it