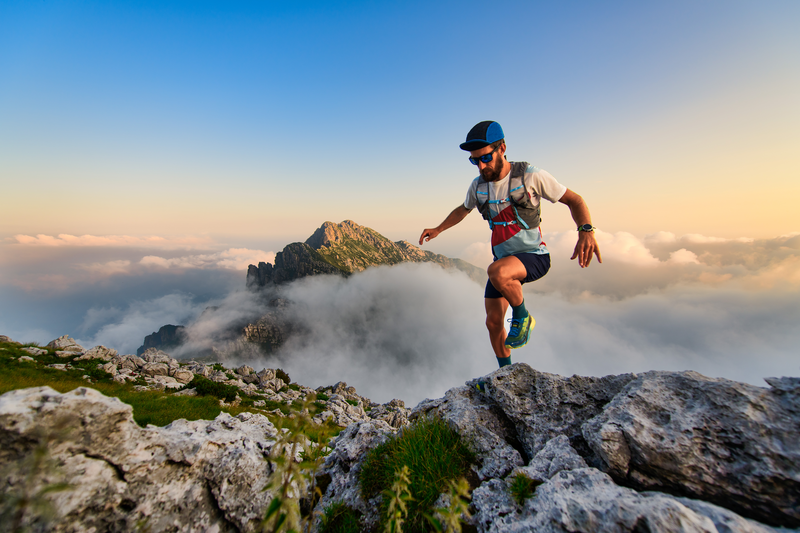
94% of researchers rate our articles as excellent or good
Learn more about the work of our research integrity team to safeguard the quality of each article we publish.
Find out more
BRIEF RESEARCH REPORT article
Front. Pediatr. , 19 November 2020
Sec. Pediatric Gastroenterology, Hepatology and Nutrition
Volume 8 - 2020 | https://doi.org/10.3389/fped.2020.591012
Background: Chronic low-grade inflammation and activation of the immune system are hallmark pathogenic mechanisms involved in metabolic dysfunction and are related to obesity. In particular, the involvement of regulatory and pro-inflammatory lymphocyte subpopulations has been reported in adults. We evaluated the Th17/Treg lymphocyte balance in obese and normal weight children, in relation with their metabolic status.
Methods: We enrolled 50 pediatric patients. According to metabolic status, subjects were classified into: metabolically healthy (MH) and metabolically unhealthy (MU) groups. MU phenotype was defined as the presence of at least one of the following risk factors: blood pressure >90th percentile, glycemia>100 mg/dl, HDL cholesterol <40 mg/dl, triglycerides>100 mg/dl (<10 years) or >130 mg/dl (>10 years), impaired insulin sensitivity with HOMA-IR>97.5th percentile. Patient Treg and Th17 profiles were also evaluated.
Results: Based on the presence of metabolic and/or cardiovascular pathological parameters, we classified 15 MU (30%) and 35 MH (70%) children; all MU children were obese. Analyzing the correlations between lymphocyte subpopulations and metabolic data, we noted a correlation between Th17 percentage and systolic hypertension (p = 0.01, r = −0.37); Treg/Th17 ratio and HOMA-IR (p = 0.02, r = 0.32) and systolic hypertension (p = 0.05, r = 0.30).
Conclusion: Children with obesity have a high risk of developing metabolic and cardiovascular complications. The Th17/Treg lymphocyte balance appears to be involved in glycemic homeostasis and blood pressure control. Careful and early monitoring of the immune system would facilitate new early preventive strategies in pediatric metabolic diseases.
The global obesity epidemic is worrying particularly considering the involvement of children and adolescents (1–5). Metabolic and cardiovascular complications linked to excess weight have been identified in young age groups, with important health and economic consequences (6–11).
Obesity is associated with systemic inflammation and is characterized by the presence of CD4 and CD8 T cell infiltration and altered immune homeostasis (12, 13). Compared to subcutaneous adipose, visceral adipose tissue has higher levels of acute phase proteins (14–17).
Importantly, this tissue turns out to be the main seat of immune cell production, and these cells in turn produce a considerable amount of pro-inflammatory cytokines (18–23), such as TNFα (16, 24), IL-1β (25, 26), IL-6 (16, 24), and interferon (IFN) γ (27–29).
It is largely recognized that chronic low-grade inflammation and activation of the immune system are involved in the pathogenic mechanisms of metabolic dysfunction (30). Data from the literature, both in experimental and human models, show the involvement of regulatory and pro-inflammatory lymphocyte subpopulations, such as T regulatory cells (Treg) and Th17. Th17 and Treg cells have reciprocal developmental pathways and opposing effects, proinflammatory, and immunosuppressive, respectively (30, 31). The factors controlling the Treg/Th17 balance and their reciprocal relationship have not been fully elucidated. However, the balance between these two compartments is central to the pathogenesis of various diseases and conditions including obesity-related complications (30–34). In particular, the role of Th17/Treg cell balance has been reported in the development and progression of insulin resistance (35), type 2 diabetes (36), non-alcoholic fatty liver disease (37–39), and atherosclerosis (40). To the best of our knowledge, no data on this topic have been described in the pediatric population.
The aim of this study was to evaluate the Th17/Treg lymphocyte balance in obese and normal weight children, in relationship with their metabolic status, in order to define its role in the development of metabolic complications.
We consecutively enrolled 50 Caucasian children and adolescents (24 M and 26 F; mean age 11.8 ± 3.6 years). These subjects were referred by their general practitioner or primary care pediatrician for auxological evaluation or obesity, between November 2018 and June 2019, to the outpatient clinic of the Pediatric Endocrinology Unit at the Fondazione IRCCS Policlinico S. Matteo. Exclusion criteria included: known secondary obesity conditions, use of any medications, and concomitant chronic or acute illnesses.
Subjects were divided into two groups, according to the World Health Organization criteria: (41)
- subjects with obesity (n = 33): BMI ≥97th percentile for the age and sex,
- subjects with normal weight (n = 17): BMI <85th percentile for the age and sex.
To assess dietary habits and physical activity, we used two sections of a previously validated questionnaire (42). Each section consisted of: 14 questions on dietary habits (breakfast consumption, daily water assumption, daily number of meals, and consumption of fruit, vegetables, and soft drinks or alcoholic beverages) and 5 questions on physical activity (walking, watching TV, listening, to music, using the computer, reading, practicing a sport, and shopping) with the following response categories: always, often, sometimes, never. The score assigned to each response ranged from 0 to 3, with the maximum score assigned to the healthiest answer and the minimum score to the least healthy response (42).
According to metabolic status, described in the Methods section, the patients were further classified into: metabolically healthy (MH) or metabolically unhealthy (MU).
The study protocol was approved by the Institutional Review Board of the Fondazione IRCCS Policlinico San Matteo (prot. 20190043635), and carried out in accordance with the Helsinki Declaration of 1975, as revised in 2008. All participants' parents or their responsible guardians were asked for and gave their written consent after being informed about the nature of the study.
The physical examination of the participants included anthropometric evaluation: height, weight, waist circumference, BMI calculation, pubertal stage determination according to Marshall and Tanner (prepubertal characteristics corresponding to Tanner stage 1) (43, 44) and blood pressure (BP) measurement. Height, weight, and waist circumference measurement, were performed as previously reported (45). BMI was calculated as body weight (kilograms) divided by height (meters squared) and waist to height ratio (WHtR) was also considered as a central adiposity index. Pubertal development was classified as: stage 1 = Tanner 1; stage 2 = Tanner stages 2–3; and stage 3 = Tanner stages 4–5. Systolic (SBP) and diastolic (DBP) blood pressures were measured twice with the patient sitting quietly; the second BP measurement was used for the analysis.
Blood samples were drawn in the morning, after an overnight fast. Metabolic blood assays included: glucose, insulin, total cholesterol, HDL-cholesterol, triglycerides (TGs), AST, ALT, GGT, PCR. Insulin resistance was calculated with the homeostasis model assessment for insulin resistance (HOMA-IR) formula. Plasma glucose was measured using the hexokinase-G-6-phosphate dehydrogenase method (Siemens Healthcare Diagnostics, UK) with a chemistry analyzer (Advia XPT, Siemens). Total cholesterol was determined with an enzymatic method (Advia XPT, Siemens Healthcare Diagnostics, UK). HDL cholesterol was measured with the selective detergent method, followed by enzymatic reactions (Siemens Healthcare Diagnostics). TG concentrations were measured with the glycerol phosphatase oxidase method (Siemens Healtcare Diagnostics, UK). Serum insulin was measured with a solid-phase, two-site chemiluminescent immunometric assay with an immunochemistry analyzer (Immulite 2000, Siemens Healtcare Diagnostics, UK). AST, ALT, and GGT were measured with a chemistry analyzer (Advia XPT, Siemens Healthcare) equipped with dedicated reagents; the method for the transaminase assay is based on NADH monitoring by ultraviolet (UV) detection without addition of P-5′-P. The GGT assay method is based on the transfer of the gamma-glutamyl group from L-gamma-glutamyl-3-carboxy-4-nitroaniline to the glycilglycine acceptor, to yield 3-carboxy-4-nitroaniline, which is measured. PCR was measured with clinical chemistry methods using the Advia XPT (Siemens Healthcare).
To define MH subjects, we applied the following criteria: SBP and DBP <90th percentile by gender, age and height percentile, glycemia <100 mg/dl, HDL-cholesterol >40 mg/dl, triglycerides <100 mg/dl (children <10 years) or <130 mg/dl (children >10 years), normal insulin sensitivity (ISI) with HOMA-IR ≤97.5 percentile for age and sex (46–48).
The MU phenotype was defined as the presence of at least one of the following risk factors: SBP or DBP >90th percentile, glycemia >100 mg/dl, HDL cholesterol <40 mg/dl, triglycerides >100 mg/dl (children <10 years) or >130 mg/dl (children >10 years), impaired insulin sensitivity (ISI) with HOMA-IR exceeding the 97.5th percentile for age and sex (48).
The criteria to define metabolic status were based on a consensus of the literature (≥80% agreement) (46). As markers of glycol metabolic derangement, a fasting blood glucose, and/or ISI was used. Impaired fasting glucose is rare in childhood and insulin resistance precedes glucose abnormalities and plays an important role in the transition from normal glucose tolerance to impaired glucose tolerance. Although the euglycemic-hyperinsulinemic clamp procedure is the gold standard method for the determination of insulin sensitivity, it is an impractical test in children as it is invasive and time intensive. For these reasons, we used HOMA-IR as a surrogate marker of insulin resistance/sensitivity (49, 50).
Peripheral blood mononuclear cells (PBMCs) were obtained by gradient density separation (Lympholyte, Cederlane). After two washes with saline solution (0.9% NaCl), viable PBMCs were cryopreserved (1% DMSO) in liquid nitrogen until analyses were performed.
After, thawing at 37°C, viable PBMCs were counted with trypan blue. Cells were suspended at a density of 1 × 106 cells/ml in RPMI supplemented with 10% heat-inactivated fetal calf serum (Euroclone) for cell surface and intracellular staining.
PBMCs were stained for Treg cell surface markers following standard procedures. In brief, after an incubation at +4°C for 30 min with antibodies specific for cell surface antigens (PC7 labeled anti-CD4, FITC labeled anti-CD127, APC labeled anti-CD25; Beckman Coulter), the cells were treated with fixation/permeabilization buffer (eBioscience) at +4°C for 40 min. PBMCs were then washed three times with permeabilization buffer to allow intracellular staining with PE-specific for forkhead box P3 transcription factor (FoxP3) antibody (eBioscience) at +4°C for 30 min.
In order to detect Th17, PBMCs were stimulated with phorbol 12-myristate l3-acetate (PMA) and ionomycin for 4 h at 37°C in the presence of brefeldin A. After one wash, cells were incubated for 30 min at +4°C with FITC labeled anti-CD3 and APC labeled anti-CD4 (Beckman Coulter). After the permeabilization procedure with fixation/permeabilization buffer (eBioscience) at +4°C for 40 min, PBMCs were washed three times with permeabilization buffer to allow intracellular staining with PE anti-IL17 (BD) at +4°C for 30 min.
For both staining procedures, appropriate isotype-matched controls were used. Acquisition and analysis of cell populations were performed by direct immunofluorescence with a Navios flow cytometer (Beckman Coulter, Milan, Italy). Typically, 100,000 events were acquired.
Tregs were defined as CD4+ CD127 neg CD25+ cells expressing FoxP3. Th17 were defined as CD4+ cells expressing intracellular IL-17. Representative flow cytometry analyses of Treg and Th17 cells, with gating algorithms, are reported in Figure 1.
Figure 1. (A,B) respectively, show representative flow cytometry analyses of Treg and Th17 subpopulations. (C) reports percentages of Treg, Th17 cells, and the Treg/Th17 ratio in enrolled subjects, according to BMI and metabolic status. Data are expressed as the mean ± SD (M) or median and IQR (MED). A p ≤ 0.05 was considered significant.
All analyses were performed using Stata 16 (StataCorp, College Station, TX, USA). Data were described with the mean, standard deviation (SD), median and 25th−75th percentiles if continuous and as counts and percent if categorical. Comparisons of quantitative variables between groups were made with the independent t-test or Mann-Whitney test. The association of categorical variables was assessed with the Fisher's exact test. For the purpose of this analysis, biomarkers were dichotomized at the local laboratory cut-off for normality. All tests were 2-sided. A p < 0.05 was considered statistically significant. Non-parametric correlations between continuous variables were assessed with the Spearman R-test. The magnitude of an effect size for correlation coefficients was evaluated as follows, as described by Cohen: small for correlation coefficients on the order of 0.1, medium for those on the order of 0.3, and large for those on the order of 0.5 (51).
The characteristics of enrolled obese children and normal weight subjects are reported in Table 1. No differences in age or sex were revealed between the groups. Children with obesity had average BMIs and HOMA-IR values, but significantly higher values when compared to normal weight subjects (p < 0.001). No other significant differences in mean values of metabolic parameters were noted. Based on the presence of metabolic and/or cardiovascular pathological parameters, 15 MU (30%) and 35 MH (70%) children were identified.
Table 1. Clinical and biochemical characteristics of enrolled subjects, according to BMI and metabolic status.
In detail, pathological cholesterol-HDL values were noted in 10 patients (30.3%), hypertriglyceridemia in four children (12.1%), systolic hypertension pressure in two (6.1%) and diastolic pressure in two (6.1%) subjects. All patients that presented with pathological parameters were obese. As reported in Table 1, BMI (p < 0.001), TG (p = 0.029), SBP (p = 0.005), and DBP (p = 0.02) were significantly higher in subjects with dysmetabolism, while HDL-cholesterol (p = 0.001) was significantly lower than that in metabolically normal subjects. Furthermore, only borderline significant (p = 0.07) HOMA was higher in the MH group when compared with the MU group.
Regarding immunological parameters, the percentages of lymphocyte subsets tested in normal weight and obese children and in metabolically normal and metabolically altered subjects are shown in Figure 1. We observed a decreasing circulating Th17 trend in children with obesity compared with normal weight children and an increasing Treg percentage trend in MH compared with MU children, without reaching significance, Figure 1.
Analyzing the correlations between lymphocyte subpopulations and clinical or metabolic data, we noted a significant correlation between Th17 percentage and systolic hypertension (p = 0.01, r = −0.37, Figure 2A); Treg/Th17 ratio and HOMA-IR (p = 0.02, r = 0.32, Figure 2B), systolic hypertension (p = 0.05, r = 0.30, Figure 2C) and physical activity level (p = 0.04, r = −0.30, Figure 2D). No other significant differences were detected.
Figure 2. Significant correlations between lymphocyte subpopulations and metabolic data. (A): correlation between Th17 percentage and systolic hypertension (p = 0.01, r = −0.3769); (B): correlation between Treg/Th17 ratio and HOMA-IR (p = 0.02, r = 0.32); (C): correlation between Treg/Th17 ratio and systolic hypertension (p = 0.05, r = 0.30); (D): correlation between Treg/Th17 ratio and physical activity (p = 0.04, r = −0.30).
Childhood obesity has reached the point where it should be considered a public health emergency (1–5). As confirmed by our results, a pathological BMI is the main risk factor for the development of metabolic and cardiovascular complications. Obese children should be evaluated for insulin resistance, dyslipidemia, and hypertension, which represent the most common early risk factors in children (6–11).
Obesity is associated with low-grade systemic inflammation; this state is well-defined by altered circulating levels of cytokines and acute phase reactants (11). During the development of an overweight or obese state, the adipose tissue undergoes morphological and secretive changes leading to a Th1 bias and a chronic state of inflammation (1, 11–17) that is involved in the development of numerous complications, such as insulin-resistance, hypertension, and dyslipidemia (18, 20, 21, 24).
Various T cell subpopulations regulate the cytokine balance of the body and secrete inflammatory factors, suggesting a link between T cell regulation and the pathogenesis of obesity related diseases. In particular, an imbalance in Th17 and Treg lymphocyte subsets has been proposed to play a role in obesity-dependent inflammation and related complications, including type 2 diabetes (T2DM) and its precursor IR (20, 24, 27, 52, 53) and early atherosclerosis process (18, 40, 54).
Even though we observed increasing or decreasing trends in Th17 and Treg lymphocytes, without reaching statistical significance, we noted a significant correlation between the Th17 and Treg distribution and the presence of IR and hypertension. These data support the influence that T cell imbalance has on systemic inflammation and dysmetabolism (11–17, 34, 55–60) and suggest a role for T cell subsets in obesity-related complications (27–39, 52–60). It has been shown that adipose tissue is a true endocrine organ able to produce and to release numerous inflammatory mediators, which play an important role in energetic homeostasis, in modulation of inflammation and the immunological response (52–60). In particular, IL-6 and TNFα seem to directly interfere with the normal transmission of insulin signals, promoting the onset of insulin resistance and later the onset of type 2 diabetes (61–64). The imbalance of lymphocyte subpopulations both in the presence of hypertension and IR confims that the two conditions may be related to each other and that inflammation is implicated in early angiogenetic processes (18, 34, 40, 54). In our study, pubertal changes may have influenced insulin sensitivity values. Moreover, considering that pathological HOMA-IR for age, sex, and pubertal stage, is accepted as a good marker of IR and IR confers risks for dyslipidemia and hypertension at a relatively early age in pediatrics (65), the role of immune system monitoring in prevention should be emphasized.
The correlation between Treg/Th17 ratio and physical activity levels confirms the importance of exercise on immune cell function, strengthening the crucial role of preventive programs.
We acknowledge that this study has some limitations, including the relatively small patient sample size that may have influenced the statistical power of the results. However, this is one of the few studies in the scientific literature evaluating this immunologic aspect in the pediatric age. Secondly, our results show an association, but do not demonstrate a direct cause-and-effect relationship, between Th17 and Treg imbalance and obesity related complications. Additionally, we only considered the role of Th17 and Tregs in metabolic disorders, confident that their cytokine expression would be useful to define the pathogenic mechanism in an inflammatory metabolically altered state. Finally, the role of fat distribution in cardiometabolic risk has been reported (66) and fat distribution at different body locations has been associated with changes in different types of cardiovascular risk biomarkers (67–69). In our patients, body composition was not recorded and the influence of fat phenotype on cardiometabolic and immunological profiles cannot be excluded.
Further studies are necessary to confirm our results and to define the role of inflammation in the complications related to obesity and the role regulatory T cells have in protection or predisposition, also considering different degrees of ponderal excess and fat distribution.
In conclusion, obese pediatric patients have a high risk of developing metabolic and cardiovascular diseases. Adipose tissue appears to be involved in T cell regulation of metabolic and tissue inflammatory responses. Careful and early monitoring of the immune system would offer new early preventive strategies in pediatric metabolic diseases.
The datasets generated for this study are available on request to the corresponding author.
The studies involving human participants were reviewed and approved by Fondazione IRCCS Policlinico San Matteo (prot. 20190043635). Written informed consent to participate in this study was provided by the participants' legal guardian/next of kin.
VC and MA designed the experiments, wrote, and supervised the writing of the manuscript. SC, FV, EC, and CR performed the experiments and wrote the manuscript. GVZ and CM supervised the writing of the manuscript. AD performed statistical analyses. RA performed the biochemical evaluations and wrote the manuscript. All of the authors have read and approved the manuscript.
This study was supported by a Current Research RC80652, Fondazione IRCCS Policlinico S. Matteo, Pavia, Italy.
The authors declare that the research was conducted in the absence of any commercial or financial relationships that could be construed as a potential conflict of interest.
The authors thank Dr. L. Kelly for English revision.
1. Kumar S, Kaufman T. Childhood obesity. Panminerva Med. (2018) 60:200–12. doi: 10.23736/S0031-0808.18.03557-7
2. Kumar S, Kelly AS. Review of childhood obesity: from epidemiology, etiology, and comorbidities to clinical assessment and treatment. Mayo Clin Proc. (2017) 92:251–65. doi: 10.1016/j.mayocp.2016.09.017
3. Gurnani M, Birken C, Hamilton J. Childhood obesity: causes, consequences, and management. Pediatr Clin North Am. (2015) 62:821–40. doi: 10.1016/j.pcl.2015.04.001
4. Güngör NK. Overweight and obesity in children and adolescents. J Clin Res Pediatr Endocrinol. (2014) 6:129–43. doi: 10.4274/jcrpe.1471
5. Nogrady B. Childhood obesity: a growing concern. Nature. (2017) 551:7681. doi: 10.1038/d41586-017-05868-y
6. Kelsey MM, Zaepfel A, Bjornstad P, Nadeau KJ. Age-related consequences of childhood obesity. Gerontology. (2014) 60:222–8. doi: 10.1159/000356023
7. Weihrauch-Blüher S, Wiegand S. Risk factors and implications of childhood obesity. Curr Obes Rep. (2018) 7:254–59. doi: 10.1007/s13679-018-0320-0
8. Bass R, Eneli I. Severe childhood obesity: an under-recognised and growing health problem. Postgrad Med J. (2015) 91:639–45. doi: 10.1136/postgradmedj-2014-133033
9. Umer A, Kelley GA, Cottrell LE, Giacobbi P Jr, Innes KE, Lilly CL. Childhood obesity and adult cardiovascular disease risk factors: a systematic review with meta-analysis. BMC Public Health. (2017) 17:683. doi: 10.1186/s12889-017-4691-z
10. Nehus E, Mitsnefes M. Childhood obesity and the metabolic syndrome. Pediatr Clin North Am. (2019) 66:31–43. doi: 10.1016/j.pcl.2018.08.004
11. Ferrante AW. Obesity-induced inflammation: a metabolic dialogue in the language of inflammation. J Intern Med. (2007) 262:408–14. doi: 10.1111/j.1365-2796.2007.01852.x
12. Nishimura S, Manabe I, Nagasaki M, Eto K, Yamashita H, Ohsugi M, et al. CD8+ effector T cells contribute to macrophage recruitment and adipose tissue inflammation in obesity. Nat Med. (2009) 15:914–20. doi: 10.1038/nm.1964
13. Jiang E, Perrard XD, Yang D, Khan IM, Perrard JL, Smith CW, et al. Essential role of CD11a in CD8+ T-cell accumulation and activation in adipose tissue. Arterioscler Thromb Vasc Biol. (2014) 34:34–43. doi: 10.1161/ATVBAHA.113.302077
14. Bernardo WM, Nobre MR, Jatene FB. Evidence-based clinical practice. part II–searching evidence databases. Rev Assoc Med Bras. (2004) 50:104–8. doi: 10.1590/S0104-42302004000100015
15. Pearson TA, Mensah GA, Alexander RW, Anderson JL, Cannon RO III, Criqui M, et al. Markers of inflammation and cardiovascular disease: application to clinical and public health practice: a statement for healthcare professionals from the centers for disease control and prevention and the American Heart Association. Circulation. (2003) 107:499–511. doi: 10.1161/01.CIR.0000052939.59093.45
16. Rodríguez-Hernández H, Simental-Mendía LE, Rodríguez-Ramírez G, Reyes-Romero MA. Obesity and inflammation: epidemiology, risk factors, and markers of inflammation. Int J Endocrinol. (2013) 2013:678159. doi: 10.1155/2013/678159
17. Coppack SW. Pro-inflammatory cytokines and adipose tissue. Proc Nutr Soc. (2001) 60:349–56. doi: 10.1079/PNS2001110
18. Lyon CJ, Law RE, Hsueh WA. Minireview: adiposity, inflammation, and atherogenesis. Endocrinology. (2003) 144:2195–200. doi: 10.1210/en.2003-0285
19. Han JM, Levings MK. Immune regulation in obesity-associated adipose inflammation. J Immunol. (2013) 191:527–32. doi: 10.4049/jimmunol.1301035
20. Phillips CM, Perry IJ. Does inflammation determine metabolic health status in obese and nonobese adults? J Clin Endocrinol Metab. (2013) 98:E1610–9. doi: 10.1210/jc.2013-2038
21. Perez-Martinez P, Alcala-Diaz J, Delgado-Lista J, Garcia-Rios A, Gomez-Delgado F, Marin-Hinojosa C, et al. Metabolical phenotypes of obesity influence triglyceride and inflammation homeostasis. Eur J Clin Invest. (2014) 44:1053–64. doi: 10.1111/eci.12339
22. Lackey DE, Burk DH, Ali MR, Mostaedi R, Smith WH, Park J, et al. Contributions of adipose tissue architectural and tensile properties toward defining healthy and unhealthy obesity. Am J Physiol Endocrinol Metab. (2014) 306:E233–46. doi: 10.1152/ajpendo.00476.2013
23. Grant RW, Dixit VD. Adipose tissue as an immunological organ. Obesity. (2015) 23:512–8. doi: 10.1002/oby.21003
24. Lee BC, Lee J. Cellular and molecular players in adipose tissue inflammation in the development of obesity-induced insulin resistance. Biochim Biophys Acta. (2014) 1842:446–62. doi: 10.1016/j.bbadis.2013.05.017
25. Negrin KA, Roth Flach RJ, DiStefano MT, Matevossian A, Friedline RH, Jung D, et al. IL-1 signaling in obesity-induced hepatic lipogenesis and steatosis. PLoS ONE. (2014) 9:e107265. doi: 10.1371/journal.pone.0107265
26. Alomar SY, Gentili A, Zaibi MS, Kepczyńska MA, Trayhurn P. IL-1β (interleukin-1β) stimulates the production and release of multiple cytokines and chemokines by human preadipocytes. Arch Physiol Biochem. (2016) 122:117–22. doi: 10.3109/13813455.2016.1156706
27. McLaughlin T, Ackerman SE, Shen L, Engleman E. Role of innate and adaptive immunity in obesity-associated metabolic disease. J Clin Invest. (2017) 127:5–13. doi: 10.1172/JCI88876
28. Guzik TJ, Skiba DS, Touyz RM, Harrison DG. The role of infiltrating immune cells in dysfunctional adipose tissue. Cardiovasc Res. (2017) 113:1009–23. doi: 10.1093/cvr/cvx108
29. O'Rourke RW, Metcalf MD, White AE, Madala A, Winters BR, Maizlin II, et al. Depot-specific differences in inflammatory mediators and a role for NK cells and IFN-gamma in inflammation in human adipose tissue. Int J Obes. (2009) 33:978–90. doi: 10.1038/ijo.2009.133
30. Xin Chen, Joost J. Oppenheim Th17 cells and Tregs: unlikely allies. J Leukoc Biol. (2014) 95:723–31. doi: 10.1189/jlb.1213633
31. Korn T, Oukka M, Kuchroo VE. Th17 cells: effector T cells with inflammatory properties. Semin Immunol. (2007) 19:362–71. doi: 10.1016/j.smim.2007.10.007
32. Cipolletta D. Adipose tissue-resident regulatory T cells: phenotypic specialization, functions and therapeutic potential. Immunology. (2014) 142:517–25. doi: 10.1111/imm.12262
33. Zúñiga LA, Shen WJ, Joyce-Shaikh B, Pyatnova EA, Richards AG, Thom C, et al. IL-17 regulates adipogenesis, glucose homeostasis, and obesity. J Immunol. (2010) 185:6947–59. doi: 10.4049/jimmunol.1001269
34. Mei W, Fuqiong C, Jingli W, Zeng Z, Yang Q, Shao S. Th17 and Treg lymphocytes in obesity and Type 2 diabetic patients. Clin Immunol. (2018) 197:77–85. doi: 10.1016/j.clim.2018.09.005
35. Tao L, Liu H, Gong Y. Role and mechanism of the Th17/Treg cell balance in the development and progression of insulin resistance. Mol Cell Biochem. (2019) 459:183–8. doi: 10.1007/s11010-019-03561-4
36. Zeng C, Shi X, Zhang B, Liu H, Zhang L, Ding W, et al. The imbalance of Th17/Th1/Tregs in patients with type 2 diabetes: relationship with metabolic factors and complications. J Mol Med. (2012) 90:175–86. doi: 10.1007/s00109-011-0816-5
37. He B, Wu L, Xie W, Shao Y, Jiang J, Zhao Z, et al. The imbalance of Th17/Treg cells is involved in the progression of nonalcoholic fatty liver disease in mice. BMC Immunol. (2017) 18:33. doi: 10.1186/s12865-017-0215-y
38. Jin Z, You J, Wang HT. The role of the balance between Th17 and Treg in liver disease. Zhonghua Gan Zang Bing Za Zhi. (2017) 25:637–40. doi: 10.3760/cma.j.issn.1007-3418.2017.08.017
39. Giles DA, Moreno-Fernandez ME, Divanovic S. IL-17 axis driven inflammation in non-alcoholic fatty liver disease progression. Curr Drug Targets. (2015) 16:1315–23. doi: 10.2174/1389450116666150531153627
40. Tarantino G, Costantini S, Finelli C, Capone F, Guerriero E, La Sala N, et al. Is serum Interleukin-17 associated with early atherosclerosis in obese patients? J Transl Med. (2014) 12:214. doi: 10.1186/s12967-014-0214-1
41. de Onis M, Onyango AW, Borghi E, Siyam A, Nishida C, Siekmann J. Development of a WHO growth reference for school-aged children and adolescents. Bull WHO. (2007) 85:660–7. doi: 10.2471/BLT.07.043497
42. Turconi G, Celsa M, Rezzani C, Biino G, Sartirana MA, Roggi C. Reliability of a dietary questionnaire on food habits, eating behavior and nutritional knowledge of adolescents. Eur J Clin Nutr. (2003) 57:753–63. doi: 10.1038/sj.ejcn.1601607
43. Marshall WA, Tanner JM. Variations in patterns of pubertal changes in boys. Arch Dis Child. (1969) 45:13–23. doi: 10.1136/adc.45.239.13
44. Marshall WA, Tanner JM. Variations in patterns of pubertal changes in girls. Arch Dis Child. (1969) 44:291–303. doi: 10.1136/adc.44.235.291
45. Calcaterra V, De Amici M, Leonard MM, De Silvestri A, Pelizzo G, Buttari N, et al. Serum calprotectin level in children: marker of obesity and its metabolic complications. Ann Nutr Metab. (2018) 73:177–83. doi: 10.1159/000492579
46. Damanhoury S, Newton AS, Rashid M, Hartling L, Byrne JLS, Ball GDC. Defining metabolically healthy obesity in children: a scoping review. Obes Rev. (2018) 19:1476–91. doi: 10.1111/obr.12721
47. Kwiterovich PO Jr. Recognition and management of dyslipidemia in children and adolescents. J Clin Endocrinol Metab. (2008) 93:4200–9. doi: 10.1210/jc.2008-1270
48. d'Annunzio G, Vanelli M, Pistorio A, Minuto N, Bergamino L, Iafusco D, et al. Insulin resistance and secretion indexes in healthy Italian children and adolescents: a multicentre study. Acta Biomedica. (2009) 80:21–8.
49. Calcaterra V, De Giuseppe R, Biino G, Mantelli M, Marchini S, Bendotti G, et al. Relation between circulating oxidized-LDL and metabolic syndrome in children with obesity: the role of hypertriglyceridemic waist phenotype. J Pediatr Endocrinol Metab. (2017) 30:1257–63. doi: 10.1515/jpem-2017-0239
50. The Expert Committee on the Diagnosis and Classification of Diabetes Mellitus: report of the expert committee on the diagnosis and classification of Diabetes mellitus. Diabetes Care. (1999) 22(Suppl. 1):S5–19. doi: 10.2337/diacare.21.1.S5
51. Cohen J. Statistical Power Analysis for the Behavioral Sciences. 2nd Edn. Hillsdale, NJ: Lawrence Erlbaum Associates (1988).
52. Sanshiro T, Francis K, Yoshikazu T. Recent advances in obesity-induced inflammation and insulin resistance. Front Endocrinol. (2013) 4:93. doi: 10.3389/fendo.2013.00093
53. Touch S, Clément K, André S. T cell populations and functions are altered in human obesity and type 2 diabetes. Curr Diab Rep. (2017) 17:81. doi: 10.1007/s11892-017-0900-5
54. Berg AH, Scherer PE. Adipose tissue, inflammation and cardiovascular disease. Circ Res. (2005) 96:939–49. doi: 10.1161/01.RES.0000163635.62927.34
55. Chehimi M, Vidal H, Eljaafari A. Pathogenic role of IL-17-producing immune cells in obesity, and related inflammatory diseases. J Clin Med. (2017) 6:68. doi: 10.3390/jcm6070068
56. Sumarac-Dumanovic M, Stevanovic D, Ljubic A, Jorga J, Simic M, Stamenkovic-Pejkovic D, et al. Increased activity of interleukin 23/interleukin-17 proinflammatory axis in obese women. Int J Obes. (2009) 33:151–56. doi: 10.1038/ijo.2008.216
57. Winer S, Paltser G, Chan Y, Tsui H, Engleman E, Winer D, et al. Obesity predisposes to Th17 bias. Eur J Immunol. (2009) 39:2629–35. doi: 10.1002/eji.200838893
58. Dalmas E, Venteclef N, Caer C, Poitou C, Cremer I, Aron-Wisnewsky J, et al. T cell-derived IL-22 amplifies IL-1beta-driven inflammation in human adipose tissue: relevance to obesity and type 2 diabetes. Diabetes. (2014) 63:196677. doi: 10.2337/db13-1511
59. Fabbrini E, Cella M, McCartney SA, Fuchs A, Abumrad NA, Pietka TA, et al. Association between specific adipose tissue CD4+ T-cell populations and insulin resistance in obese individuals. Gastroenterology. (2013) 145:366–74. doi: 10.1053/j.gastro.2013.04.010
60. Yusuke E, Koutaro Y, Toshinori N. The obesity-related pathology and Th17 cells. Cell Mol Life Sci. (2017) 74:1231–45. doi: 10.1007/s00018-016-2399-3
61. Maurizi G, Della Guardia L, Maurizi A, Poloni A. Adipocytes properties and crosstalk with immune system in obesity-related inflammation. J Cell Physiol. (2018) 233:88–97. doi: 10.1002/jcp.25855
62. Lainampetch J, Panprathip P, Phosat C, Chumpathat N, Prangthip P, Soonthornworasiri N, et al. Association of tumor necrosis factor alpha, interleukin 6, and c-reactive protein with the risk of developing type 2 diabetes: a retrospective cohort study of rural thais. J Diabetes Res. (2019) 2019:9051929. doi: 10.1155/2019/9051929
63. Arab Sadeghabadi Z, Abbasalipourkabir R, Mohseni R, Ziamajidi N. Investigation of oxidative stress markers and antioxidant enzymes activity in newly diagnosed type 2 diabetes patients and healthy subjects, association with IL-6 level. J Diabetes Metab Disord. (2019) 18:437–43. doi: 10.1007/s40200-019-00437-8
64. Sangoi MB, Carvalho JAM, Guarda NS, Duarte T, Duarte MMMF, Premaor MO, et al. Association between urinary levels of interleukin-6, interleukin-10 and tumor necrosis factor-alpha with glomerular and tubular damage Indicators in patients with type 2 diabetes. Clin Lab. (2019) 11:65. doi: 10.7754/Clin.Lab.2019.190410
65. Sakou II, Psaltopoulou T, Sergentanis TN, Karavanaki K, Karachaliou F, Ntanasis Stathopoulos I, et al. Insulin resistance and cardiometabolic risk factors in obese children and adolescents: a hierarchical approach. J Pediatr Endocrinol Metab. (2015) 28:589–96. doi: 10.1515/jpem-2014-0431
66. Stefan N. Causes, consequences, and treatment of metabolically unhealthy fatdistribution. Lancet Diabetes Endocrinol. (2020) 8:616–27. doi: 10.1016/S2213-8587(20)30110-8
67. Weil BR, Westby CM, van Guilder GP, Greiner JJ, Stauffer BL, DeSouza CA. Enhanced endothelin-1 system activity with overweight and obesity. Am J Physiol Heart Circ Physiol. (2011) 301:H689–95. doi: 10.1152/ajpheart.00206.2011
68. Suthahar N, Meijers WC, Ho JE, Gansevoort RT, Voors AA, van der Meer P, et al. Sex-specific associations of obesity and N-terminal pro-B-type natriuretic peptide levels in the general population. Eur J Heart Fail. (2018) 20:1205–14. doi: 10.1002/ejhf.1209
Keywords: obesity, children, Th17, Treg, metabolic status
Citation: Calcaterra V, Croce S, Vinci F, De Silvestri A, Cordaro E, Regalbuto C, Zuccotti GV, Mameli C, Albertini R and Avanzini MA (2020) Th17 and Treg Balance in Children With Obesity and Metabolically Altered Status. Front. Pediatr. 8:591012. doi: 10.3389/fped.2020.591012
Received: 03 August 2020; Accepted: 09 October 2020;
Published: 19 November 2020.
Edited by:
Steven Thomas Leach, University of New South Wales, AustraliaReviewed by:
Norbert Stefan, University of Tübingen, GermanyCopyright © 2020 Calcaterra, Croce, Vinci, De Silvestri, Cordaro, Regalbuto, Zuccotti, Mameli, Albertini and Avanzini. This is an open-access article distributed under the terms of the Creative Commons Attribution License (CC BY). The use, distribution or reproduction in other forums is permitted, provided the original author(s) and the copyright owner(s) are credited and that the original publication in this journal is cited, in accordance with accepted academic practice. No use, distribution or reproduction is permitted which does not comply with these terms.
*Correspondence: Valeria Calcaterra, dmFsZXJpYS5jYWxjYXRlcnJhQHVuaXB2Lml0
†These authors have contributed equally to this work
Disclaimer: All claims expressed in this article are solely those of the authors and do not necessarily represent those of their affiliated organizations, or those of the publisher, the editors and the reviewers. Any product that may be evaluated in this article or claim that may be made by its manufacturer is not guaranteed or endorsed by the publisher.
Research integrity at Frontiers
Learn more about the work of our research integrity team to safeguard the quality of each article we publish.